Abstract
Schisandrin C, a dibenzocyclooctadiene lignan isolated from the fruits of Schisandra chinensis (Turcz.) Baill. (Schisandraceae), was investigated for cytotoxicity and influence on three human cancer cell lines. Among human hepatocelluar carcinoma cells (Bel-7402), human breast cancer cells (Bcap37) and human nasopharyngeal carcinoma cells (KB-3-1), Bel-7402 cells were most sensitive with IC50 equal to 81.58 ± 1.06 μ M after treatment with schisandrin C for 48 h. Cytotoxicity of schisandrin C on tumor cells depends on cellular accumulation of the drug. The intracellular schisandrin C concentration was assayed by high-performance liquid chromatography (HPLC) and showed that schisandrin C could permeate the cell membrane. Staining with Hoechst 33258 showed fragmentation and condensation of chromatin in the cell treated with 75 μ M schisandrin C for 24 h. Flow cytometric analysis was performed to determine hypodiploid cells. The results of flow cytometry indicated that the percentage of hypodiploid Bel-7402 cells was 40.61 ± 1.43% after 24 h treatment with 100 μ M schisandrin C. The treatment resulted in the appearance of a hypodiploid peak (sub-G0/G1 region), probably due to the presence of apoptosing cells and apoptotic bodies with DNA content less than 2n. To our knowledge, this is the first report against human hepatocelluar carcinoma cells (Bel-7402) of schisandrin C.
Introduction
Schisandra chinensis (Turcz.) Baill. (Beiwuweizi in Chinese), belonging to the Schisandraceae family, is a well-known traditional Chinese medicine whose fruits have commonly been used as a tonic, a sedative, an antitussive, and an antiaging drug for centuries in China (CitationPharmacopoeia Commission of PRC, 2005). In clinical situations it has been used for the treatment of patients suffering from viral and chemical hepatitis since the 1970s (CitationHancke et al., 1999). Analysis of the chemical composition of Schisandra chinensis demonstrated that the biologically active compounds are dibenzocyclooctadiene lignans, which have revealed a wide variety of active effects including antihepatotoxic (CitationChiu et al., 2003; CitationIp et al., 2000; CitationHikino et al., 1984), antioxidant and detoxificant (CitationIp et al., 1995; CitationIp & Ko, 1996; CitationMak et al., 1997; CitationChiu et al., 2005), anticancer (CitationWu et al., 2004), and anti-amnestic activities (CitationKim et al., 2004, Citation2006). Recently, dibenzocyclooctadiene lignans were reported to reverse cancer drug resistance by targeting P-glycoprotein (P-gp) (CitationPan et al., 2005, Citation2006; CitationWan et al. 2006), multidrug resistance-associated protein 1 (MRP1) (CitationLi et al., 2007; CitationSun et al., 2007), and enhancing apoptosis (CitationLi et al., 2006).
Schisandrin C, as one of the important active dibenzocyclooctadiene lignans of Schisandra chinensis (), has been used as a liver protective drug (CitationKiso et al., 1985). Schisandrin C can afford protection against carbon tetrachloride-induced hepatic damage (CitationIp et al., 1997), myocardial ischemia-reperfusion injury (CitationYim & Ko, 1999), and glutamate-induced neurotoxicity (CitationKim et al., 2004). Moreover, schisandrin C has inhibitory activity against NFAT transcription (CitationLee et al., 2003). Therefore, schisandrin C has an extensive application possibility in clinical medicine. However, there are few reports focusing on its anti-tumor activity. To search a new candidate drug for treatment of human cancer, we investigated the antitumor activity of schisandrin C isolated from the traditional Chinese medicine Schisandra chinensis against human cancer cells.
Materials and Methods
Materials
The dried fruits of Schisandra chinensis were purchased from Shanghai Huayu Chinese Herbs Co., Ltd., Shanghai, China. The voucher specimen, identified by Professor Yan-hua Lu, was deposited at the Institute of Biochemistry, East China University of Science and Technology, Shanghai, China. 3-(4,5-Dimethylthiazol-2-yl)-2,5-diphenyltetrazolium bromide (MTT) was purchased from Bioseen Technology, Inc, Shanghai, China. Rosewell Park Memorial Institute (RPMI) Medium 1640 was purchased from GIBCO BRL, Grand Island, NY, USA. Fetal bovine serum (FBS) was purchased from Hangzhou Sijiqing Co., Ltd, Zhejiang, China. The other reagents are analytic reagents (AR).
Cell and cell culture
The human cell lines used included human hepatocellular carcinoma cells (Bel-7402), human breast cancer cells (Bcap37), human nasopharyngeal carcinoma cells (KB-3-1), human endothelial cells (ECV-304), and human liver cells (QSG-7701). All cells were purchased from the Cell Bank of Type Culture Collection of Chinese Academy of Sciences (Shanghai, China) and were cultured in RPMI 1640 medium with 100 mL/L FBS, penicillin (100 U/ml) and streptomycin (100 μ g/ml) at 37°C in a humidified atmosphere of 95% air and 5% CO2. Schisandrin C is dissolved in dimethylsulfoxide (DMSO). The control cells were treated with the same amount of vehicle alone. The final DMSO concentration never exceeded 0.5% (v/v), in either control or treated samples. Previous experiments have shown that DMSO at this concentration does not modify the cellular activities that were analyzed.
Separation and purification of schisandrin C from Schisandra chinensis
The powdered fruits of Schisandra chinensis (1 kg) were refluxed with hot ethanol twice at 70°C. The ethanol extract was filtered and concentrated to remove ethanol at 60°C by a rotary evaporator under reduced pressure. The residue was first separated by solvent extraction using petroleum ether and water and the petroleum ether portion exhibited strong anti-tumor activity. Then the petroleum ether portion was separated on a silica gel column (50 × 8 cm inside diameter (id)) eluted with 6.0 L 15% ethyl acetate in petroleum ether to obtain fraction 5. At last fraction 5 was re-crystallized with ethyl acetate to obtain 300 mg of schisandrin C.
The identification of schisandrin C separated in our laboratory () was accomplished by spectroscopic methods (UV, IR, ESI-MS, 1H-NMR and 13C-NMR). The spectral data of schisandrin C corresponded to literature values. The purity of schisandrin C was determined to be higher than 98% by normalization of the peak area detected by HPLC experiments.
Figure 3. Cytotoxic effects of schisandrin C in human cancer cells and human normal cells. Effect of schisandrin C on the viability of human cancer cells and human normal cells. The cells were treated with various concentrations of schisandrin C for 48 h, and cell viability was determined by MTT assay and was expressed as the mean ± SD of three separate experiments (n = 3 each in the three experiments). Significant differences from untreated control are indicated by *P < 0.01; ** P < 0.001.
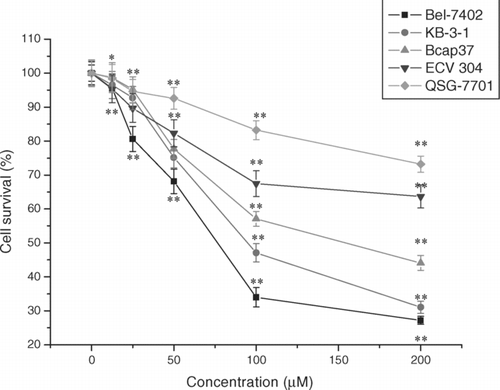
Cytotoxicity assay
The MTT (3-(4,5-dimethylthiazol-2-yl)-2,5-diphenyl tetrazolium bromide) colorimetric assay was performed as described by CitationMosmann (1983). Cells were placed within 96-well culture plates (104 cells/well) respectively, and allowed to attach for 24 h before treatment. The cells were treated with schisandrin C ranging from 12.5 to 200 μ m or without (vehicle control, 0.5% DMSO) schisandrin C for cell lines. Schisandrin C cytotoxicity was measured after 48 h of culture using the MTT assay. Absorbance in control and drug-treated wells was measured in an automated microplate reader (Bio-Rad 550) at 550 nm. The cytotoxicity of schisandrin C was expressed as IC50 (concentration of 50% cytotoxicity, which was extrapolated from linear regression analysis of experimental data).
HPLC assay of intracellular and extracellular schisandrin C concentration
Schisandrin C was dissolved in RPMI-1640 medium (100 μ M), and then incubated at 37°C in a humidified atmosphere of 95% air and 5% CO2 for 5 days. The solution was centrifuged at 12,000 rpm for 5 min at 4°C. The supernatant was assayed by HPLC to test the stability of schisandrin C.
The Bel-7402 cells were seeded at a density of 1.0 × 106 cells/ml and the cells were pretreated with 80 μ M schisandrin C for 12 h, then the medium was collected and centrifuged at 12,000 rpm for 5 min at 4°C. The supernatant was filtrated through a 0.45 μ m membrane. The supernatant was assayed by HPLC to determine the concentration of schisandrin C. In order to investigate the intracellular schisandrin C concentration, that cells were rinsed with PBS three times, and then a known number cells in PBS received freeze-thawing twice and were homogenized on ice. The cell homogenate was centrifuged at 12,000 rpm for 15 min at 4°C. The supernatant was stored on ice, and then was filtrated through a 0.45 μ m membrane. The supernatant was assayed by HPLC to examine the permeability of schisandrin C.
The chromatographic data were recorded and processed with Agilent Chromatographic Work Station software. Analysis was carried out at 30°C on an Agilent Eclipse SB-C 18 column (5 μ m, 250 × 4.6 mm, i.d.), which was protected by a guard column (5 μ m, 12.5 × 4.6 mm, i.d.). The mobile phase contained water and acetonitrile (20:80, v/v) at a flow rate of 1.0 ml/min. It was detected at 254 nm.
Fluorescent staining of nuclei of Bel-7402 cells
Bel-7402 cells from exponentially growing cultures were seeded within 24-well culture plates and were allowed to attach for 24 h before treatment. The cells were treated with 75 μ M schisandrin C or without (vehicle control, 0.5% DMSO) schisandrin C for 24 h. After treatment, cells were washed with phosphate-buffered saline (PBS), and were fixed in MeOH-HAc (3:1, v/v) for 10 min at 4°C. Cells were stained with Hoechst 33258 (5 μ M in PBS) for 5 min at room temperature and then examined in a Leica DMIRB fluorescent microscope at 356 nm (CitationYe et al., 2004).
Flow cytometry assay of Bel-7402 cells
To determine apoptosis, cells were stained with propidium iodide (PI) as described (CitationLee et al., 2004). The Bel-7402 cells, pretreated with various concentrations of schisandrin C, were collected by centrifugation 1,000 rpm for 15 min, and thoroughly rinsed with PBS. The pellets were resuspended in ice-cold 70% ethanol and fixed at −20°C for 30 min. The cells were then centrifuged (1,000 rpm for 15 min), and ethanol was removed by rinsing thoroughly with PBS. The cell pellets were resuspended in 1 mL DNA staining reagent containing 50 μ M RNase, 0.1% Triton X-100, 0.1 mM EDTA (pH = 7.4), and 50 μ mol/L PI at 4°C for 30 min in the dark. The DNA contents of stained cells were analyzed by a FACScan (Becton Dickinson, USA) with Cell Fit software. Hypodiploid cells have less DNA than that of diploid cells at G1 phase because of apoptosis-induced DNA fragmentation.
Statistical analysis
The results were expressed as means ± standard deviation (SD) and were analyzed by pair t-test. The scientific statistic software Origin version 7.0 was used to evaluate the significance of differences between groups. The criterion of statistical significance: *P < 0.01 and ** P < 0.001 versus control group.
Results
Cytotoxic effects of schisandrin C on human cancer cells and human normal cells
In our study we used the MTT assay to test the cytotoxic effects of schisandrin C. MTT is a tetrazolium salt which forms purple-colored, water-insoluble formazan upon reduction. Because only living cells can reduce MTT, MTT reduction has been developed into one of the most widely used methods for measuring cell proliferation and viability. It was widely assumed that MTT is reduced by active mitochondria in living cells because an earlier study showed that the mitochondria-specific substrate succinate supported MTT reduction (CitationLiu et al., 1999). In our report, cultured cancer cells were treated with schisandrin C (12.5–200 μ M) for 48 h, and the cytotoxicity of schisandrin C on cancer cells using MTT assay is shown in . The data indicated that cytotoxicity of schisandrin C on cancer cells was dose-dependent. The IC50 values on cytotoxicity were 81.58 ± 1.06 μ M for Bel-7402 cells, 108.00 ± 1.13 μ M for KB-3-1 cells, and 136.97 ± 1.53 μ M for Bcap37 cells, respectively. Therefore, further studies to assess the apoptotic activity of schisandrin C were performed with Bel-7402 cells.
To investigate whether schisandrin C has selective toxicity toward neoplastic rather than normal cells, the effects of schisandrin C on human normal cells were investigated. shows treatment of human liver QSG-7701 cells, human endothelial ECV-304 cells with schisandrin C (12.5–200 μ M) for 48 h resulted in a dose-dependent cytotoxicity. But human liver QSG-7701 cells were more resistant to the schisandrin C-mediated cytotoxicity than ECV-304 cells. When the concentration of schisandrin C was 200 μ M the cell survival for QSG-7701 was 73.2 ± 2.4%, compared with the cell survival for Bel-7402 cells which was 27.2 ± 1.2%. From this we can see that schisandrin C showed lower toxicity on human normal liver cells.
HPLC assay of intracellular and extracellular schisandrin C concentration
Based on the standard curve of schisandrin C, the stability of schisandrin C is shown in . Although schisandrin C showed a little instability in RPMI-1640 medium at 37°C in a humidified atmosphere of 95% air and 5% CO2, it was very stable in DMSO at 4°C. Schisandrin C was detected at 254 nm, and showed a retention time of 10.62 min under the chromatographic conditions shown in . The regression equation of the calibration curve was y = 4.5907 x + 1.0391 (r = 0.9999) for schisandrin C at the linear concentration range from 11.44 to 572.3 μ M (x, contents of compound (μ M); y, peak areas). When the cells were treated with 80 μ M schisandrin C for 12 h, the extracellular () and intracellular () concentration of schisandrin C were 76.77 μ mol and 4.04 μ mol/106 cells, respectively. The study showed schisandrin C could permeate the cell membrane into the cell. When schisandrin C permeated the cell membrane, it needed further research to investigate whether schisandrin C was converted to other derivatives.
Figure 4. Half-life of schisandrin C in DMSO at 4°C and RPMI-1640 medium (without cells). Schisandrin C was dissolved in RPMI-1640 medium, and then incubated for 5 days at 37°C, 5% CO2 and 95% humidity conditions. The solution was centrifuged at 12,000 rpm for 10 min. The supernatant was assayed by HPLC. Control group: schisandrin C incubated in RPMI-1640 medium for 0 h.
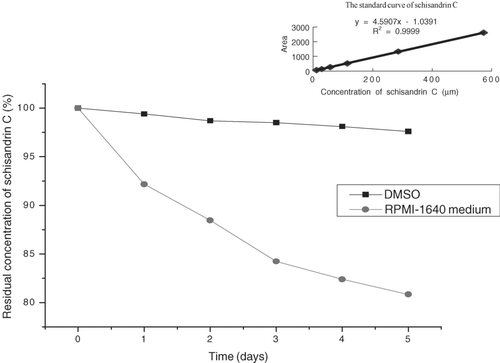
Figure 5. Intracellular and extracellular concentration of schisandrin C as quantified by HPLC method. Schisandrin C (80 μ M) was administered into RPMI-1640 medium with an incubation time of 12 h. The solution was centrifuged with 12,000 rpm for 10 min. The supernatant was assayed by HPLC. Under chromatographic conditions employed, schisandrin C was shown by a retention time of 10.623 min. (A) The standard of schisandrin C. (B) Extracellular concentration of schisandrin C (incubated in RPMI-1640 medium for 12 h). (C) Intracellular concentration of schisandrin C (incubated in RPMI-1640 medium for 12 h). (D) Blank cell lysis solution.
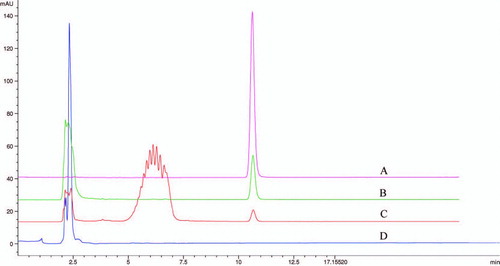
Effects of schisandrin C on nuclear morphology in Bel-7402 cells
Hoechst 33258 staining was applied to investigate whether Bel-7402 cells underwent cell death via apoptosis or necrosis. As shown in , after staining with Hoechst 33258, Bel-7402 cells treated with 75 μ M schisandrin C for 24 h revealed marked nuclear condensation, membrane blebbing, nuclear fragmentation and apoptotic bodies (as illustrated by arrows), all of which are the characteristics of apoptotic programmed cell death. However, the control cells () exhibited a normal nuclear morphology characterized by a diffuse chromatin structure and therefore light staining.
Figure 6. Effects of schisandrin C on the nuclear morphology in Bel-7402 cells. Fluorescent staining of nuclei in schisandrin C-treated Bel-7402 cells by Hoechst 33258. Cells were treated with 75 μ M schisandrin C for 24 h. Cells with condensed and fragmented nuclei and apoptotic bodies (arrows) are seen in the schisandrin C-treated cells (B), but not in the control treatment (A). Magnification x 200.
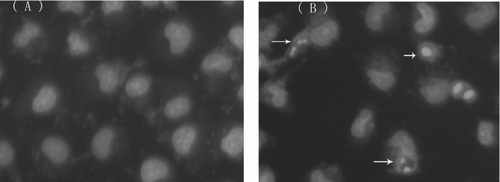
Effects of schisandrin C on the population of hypodiploid cells in Bel-7402 cells
To test whether schisandrin C-induced cell death via apoptosis, the percentage of apoptotic cells was measured by flow cytometry. Ten thousand cells in each sample were analyzed and the percentage of apoptotic cell accumulation in the sub-G1 peak was calculated. The sub-G1 peak in flow cytometry detection is considered as an indicator of cell apoptosis. Cells with DNA content less than G1 in the cell cycle distribution were counted as hypodiploid cells. As shown in , sub-G0/G1 population in drug-treated groups increased with the concentration of the schisandrin C increment. After a 24-h incubation with 50, 75, and 100 μ M, the percentage of hypodiploid cells were 7.21 ± 0.85, 10.32 ± 1.08, 40.61 ± 1.43%, respectively. The percentage of hypodiploid cells was significantly increased at the concentration of 50–100 μ M, which was in agreement with MTT results. The treatment resulted in the appearance of a hypodiploid peak (sub-G0/G1 region), probably due to the presence of cells in apoptosis and apoptotic bodies with DNA content less than 2n.
Figure 7. Analysis of the population of hypodiploid cells in Bel-7402 cells by flow cytometry. (A) Representative of the profiles of cell cycle distribution: Bel-7402 cells were not treated (a), 50 μ M (b), 75 μ M (c), 100 μ M (d) schisandrin C for 24 h. (B) The percentage of hypodiploid cells after staining with propidium iodide. Data are presented as the mean ± SD and are representative of an average of three independent experiments per concentration. Significant differences from control **P < 0.001.
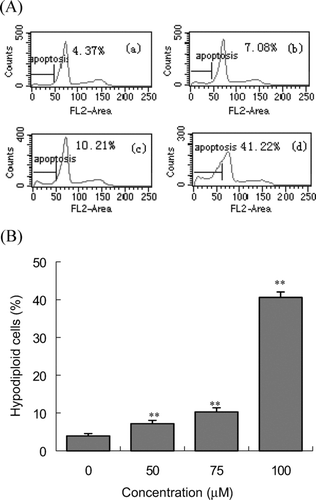
Discussion
Although the efficacy of chemotherapy and other standard therapies for the majority of cancer types has been improved during the last decades, the treatment of most human malignancies is still facing high mortality rates. Therefore, the development of novel potent, but non-toxic anti-cancer reagents is worth a continuous effort. In the last decade, dibenzocyclooctadiene lignans (e.g., deoxyschisandrin, γ -schisandrin, schisandrin C, schisandrin, gomisin A, schisantherin A) (CitationHe et al., 1997) has attracted considerable attention as a potential anti-cancer drug or an adjuvant to the conventional therapeutic modalities for human hepatocellular cancer (CitationWu et al., 2004; CitationPan et al., 2006; CitationLi et al., 2007).
The present study was undertaken to assess the anti-tumor activity of schisandrin C against three established human cancer cell lines. Among human hepatocelluar carcinoma cells (Bel-7402), human breast cancer cells (Bcap37) and human nasopharyngeal carcinoma cells (KB-3-1), Bel-7402 cells were the most sensitive one in these tested cell lines. The cytotoxic effects of schisandrin C showed dose-dependency against Bel-7402 cells, with IC50 equal to 81.58 ± 1.06 μ M for incubation of 48 h. In particular, we found that most of the Bel-7402 cells were detached from the surface of the culture dish and were floating in the medium after treatment with relatively high concentration of schisandrin C.
Safety and potency are the two most important criteria to evaluate a drug. Schisandra chinensis has been applied as a medicinal herb in China for several thousand years without reports of side effects, and is indexed as a tonic and sedative in Chinese pharmacopeias. Schisandrin C, as one of the major dibenzocyclooctadiene lignans isolated from Schisandra chinensis, had an lower cytocidal effect against QSG-7701. When the concentration of schisandrin C was 200 μ M, the cell survival for QSG-7701 was 73.2 ± 2.4%. The other data regarding the low toxicities related to this compound are referred to in a review article by CitationHancke et al. (1999). Therefore, with the safety and activities against human hepatocelluar carcinoma cells, schisandrin C has potential clinical application.
Furthermore, cytotoxicity of the schisandrin C on tumor cells depends on cellular accumulation of the drug. The intracellular schisandrin C concentration was assayed by HPLC and showed that schisandrin C could permeate the cell membrane into the cell. After schisandrin C permeation of the cell membrane, it needed further research to investigate whether schisandrin C was converted to other derivatives. In this study, we did not observe any metabolites of schisandrin C by HPLC assay, compared with few reports about schisandrin C converted to other derivatives based on our available literature knowledge.
Apoptosis, characterized by morphological changes such as membrane blebbing, cell shrinkage, chromatin condensation and nuclear fragmentation with formation of apoptotic bodies, is a form of programmed cell death that occurs naturally in cells and can be beneficial to cancer therapy as previously studied (CitationChinkwo, 2005; CitationKroemer & Martin, 2005). In this study, we showed that schisandrin C induced Bel-7402 cell apoptosis was confirmed by Hoechst 33258 staining and cell cycle analyses. Staining with Hoechst 33258 showed condensed and fragmented nuclei and apoptotic bodies in the cells treated with 75 μ M schisandrin C for 24 h. The FCM results indicated a percentage of hypodiploid Bel-7402 cells of about 40.61 ± 1.43%, after treatment with 100 μ M for 24 h, and the appearance of a hypodiploid peak (sub-G0/G1 region), probably due to the presence apoptosis cells and apoptotic bodies with DNA content less than 2n. These results suggested that schisandrin C could induce Bel-7402 cells apoptosis in vitro.
Although the mechanism of tumor inhibiting activity of schisandrin C is not clarified, there are some reports about dibenzocyclooctadiene lignans inducing cancer cell apoptosis. Schisandrin B induced apoptosis through caspase-3-dependent pathways accompanied with the down-regulation of heat shock protein 70 protein expression at an early event in human hepatoma SMMC-7721 cells (CitationWu et al., 2004). Schisandrin B can also substantially enhance caspase-9 accompanied with a significant elevation of mitochondrial breakdown in SMMC-7721 cells (CitationLi et al., 2006). Further investigations are needed to determine in more detail the molecular mechanism of apoptosis in Bel-7402 cells induced by schisandrin C, such as the dependent pathway of this apoptotic process, the signal transduction pathways involved and the possible changes in expression of other apoptosis-related genes.
Besides its low toxicity to healthy cells and cytotoxicity on human hepatocellular cancer cell (Bel-7402), schisandrin C has the following advantages. First of all, dibenzocyclooctadiene lignans were reported to reverse cancer drug resistance by targeting P-gp (CitationPan et al., 2006), MRP1 (CitationLi et al., 2007), and enhancing apoptosis (CitationLi et al., 2006). Schisandrin C as one of the important dibenzocyclooctadiene lignans may have similar activities; secondly, schisandrin C as a strong liver protective agent can prevent liver injuries (CitationKiso et al, 1985) and inhibit lipid peroxidation (CitationLu & Liu, 1991; CitationLiu & Lesca, 1982); finally, its activities on enhancement of mitochondrial glutathione antioxidant system and the induction of heat shock protein 70 (CitationIp et al., 1997). Bearing the multiple functions and high safety, schisandrin C could be promising as a potent apoptosis-inducing drug or an adjuvant to the conventional therapeutic modalities for human hepatocellular cancer.
In conclusion, in this paper we investigated in vitro the antitumor activity of schisandrin C in human cancer cell lines and made an attempt to support that schisandrin C had cytotoxicity, anti-proliferation, and apoptosis-inducing effect on Bel-7402 cells. To our knowledge, this is the first report against Bel-7402 cells of schisandrin C.
Acknowledgements
This work is supported by the key disciplinary foundation of Shanghai (03DZ19546).
Declaration of interest: The authors report no conflicts of interest. The authors alone are responsible for the content and writing of the paper.
References
- KA Chinkwo. (2005). Sutherlandia frutescens extracts can induce apoptosis in cultured carcinoma cells. J Ethnopharmacol 98:163–170.
- PY Chiu, DHF Mak, MKT Poon, and KM Ko. (2005). Role of cytochrome P-450 in schisandrin B-induced antioxidant and heat shock responses in mouse liver. Life Sci 77:2887–2895.
- PY Chiu, MH Tang, DHF Mak, MKT Poon, and KM Ko. (2003). Hepatoprotective mechanism of schisandrin B: Role of mitochondrial glutathione antioxidant status and heat shock proteins. Free Radical Bio Med 35:368–380.
- JL Hancke, RA Burgos, and F Ahumada. (1999). Schisandra chinensis (Turcz.) Baill. Fitoterapia 70:451–471.
- XG He, LZ Lian, and LZ Lin. (1997). Analysis of lignan constituents from Schisandra chinensis by liquid chromatography-electrospray mass spectrometry. J Chromatogr A 757:81–87.
- H Hikino, Y Kiso, H Taguchi, and Y Ikeya. (1984). Antihepatotoxic actions of lignoids from Schizandra chinensis fruits. Planta Med 50:213–218.
- SP Ip, and KM Ko. (1996). The crucial antioxidant action of schisandrin B in protecting against carbon tetrachloride hepatotoxicity in mice: A comparative study with butylated hydroxytoluene. Biochem Pharmacol 52:1687–1693.
- SP Ip, CY Ma, CT Che, and KM Ko. (1997). Methylenedioxy group as determinant of schisandrin in enhancing hepatic mitochondrial glutathione in carbon tetrachloride-intoxicated mice. Biochem Pharmacol 54:317–319.
- SP Ip, MKT Poon, SS Wu, CT Che, KH Ng, YC Kong, and KM Ko. (1995). Effect of schisandrin B on hepatic glutathione antioxidant system in mice: Protection against carbon tetrachloride toxicity. Planta Med 61:398–401.
- SP Ip, HY Yiu, and KM Ko. (2000). Differential effect of schisandrin B and dimethyl diphenyl bicarboxylate (DDB) on hepatic mitochondrial glutathione redox status in carbon tetrachloride intoxicated mice. Mol Cell Biochem 205:111–114.
- DH Kim, TM Hung, KH Bae, JW Jung, S Lee, BH Yoon, JH Cheong, KH Ko, and JH Ryu. (2006). Gomisin A improves scopolamine-induced memory impairment in mice. Eur J Pharmacol 542:129–135.
- SR Kim, MK Lee, KA Koo, SH Kim, SH Sung, NG Lee, GJ Markelonis, TH Oh, JH Yang, and YC Kim. (2004). Dibenzocyclooctadiene lignans from Schizandra chinensis protect primary cultures of rat cortical cells from glutamate-induced toxicity. J Neurosci Res 76:397–405.
- Y Kiso, M Tohkin, H Hikino, Y Ikeya, and H Taguchi. (1985). Mechanism of antihepatotoxic activity of wuweizisu C and gomisin A. Planta Med 51:331–334.
- G Kroemer, and SJ Martin. (2005). Caspase-independent cell death. Nature Med 11:725–730.
- EJ Lee, HY Min, HJ Park, HJ Chung, S Kim, YN Han, and SK Lee. (2004). G2/M cell cycle arrest and induction of apoptosis of by a stilbenoid, 3,4,5-trimethoxy-4′-bromo-cis-stilbene, in human lung cancer cells. Life Sci 75:2829–2839.
- IS Lee, HK Lee, NT Dat, MS Lee, JW Kim, DS Na, and YH Kim. (2003). Lignans with inhibitory activity against NFAT transcription from Schisandra chinensis. Planta Med 69:63–64.
- L Li, Q Lu, and X Hu. (2006). Schisandrin B enhances doxorubicin-induced apoptosis of cancer cells but not normal cells. Biochem Pharmacol 71:584–595.
- L Li, QR Pan, M Sun, QH Lu, and X Hu. (2007). Dibenzocyclooctadiene lignans—A class of novel inhibitors of multidrugresistance-associated protein 1. Life Sci 80:741–748.
- KT Liu, and P Lesca. (1982). Pharmacological properties of dibenzo[a c]cyclooctene derivatives isolated from Fructus Schizandrae chinensis III. Inhibitory effects on carbon tetrachloride-induced lipid peroxidation, metabolism and covalent binding of carbon tetrachloride to lipids. Chem-Biol Interact 41:39–47.
- YB Liu. (1999). Understanding the biological activity of amyloid proteins in vitro: From inhibited cellular MTT reduction to altered cellular cholesterol homeostatis. Prog Neuro-Psycho 23:377–395.
- H Lu, and GT Liu. (1991). Effect of dibenzo[ac]cyclooctene lignans isolated from Fructus schizandrae on lipid peroxidation and antioxidative enzyme activity. Chem-Biol Interact 78:77–84.
- DHF Mak, and KM Ko. (1997). Alterations in susceptibility to carbon tetrachloride toxicity and hepatic antioxidant/detoxification system in streptozotocin-induced short-term diabetic rats: Effects of insulin and schisandrin B treatment. Mol Cell Biochem 175:225–232.
- T Mosmann. (1983). Rapid colorimetric assay for cellular growth and survival: Application to proliferation and cytotoxicity assays. J Immunol Methods 65:55–63.
- Pharmacopoeia Commission of PRC (2005): Pharmacopoeia of the People's Republic of China Version 2005, Vol. 1. Beijing, Chemical Industry Press.
- Q Pan, Q Lu, K Zhang, and X Hu. (2006). Dibenzocyclooctadiene lignans-A class of novel inhibitors of P-glycoproteins. Cancer Chemoth Pharm 58:99–106.
- QR Pan, T Wang, and X Hu. (2005). Schisandrin B-a novel inhibitor of pglycoprotein. Biochem Bioph Res Co 335:406–411.
- M Sun, XL Xu, Q Lu, Q Pan, and X Hu. (2007). Schisandrin B-A dual inhibitor of P-glycoprotein and multidrug resistance-associated protein 1. Cancer Lett 246:300–307.
- CK Wan, GY Zhu, XL Shen, A Chattopadhyay, S Dey, and WF Fong. (2006). Gomisin A alters substrate interaction and reverses P-glycoprotein-mediated multidrug resistance in HepG2-DR cells. Biochem Pharmacol 72:824–837.
- YF Wu, MF Cao, YP Gao, F Chen, T Wang, EP Zumbika, and KX Qian. (2004). Down-modulation of heat shock protein 70 and up-modulation of caspase-3 during schisandrin B-induced apoptosis in human hepatoma SMMC-7721 cells. World J Gastroenterol 10:2944–2948.
- CL Ye, JW Liu, DZ Wei, YH Lu, and F Qian. (2004). In vitro anti-tumor activity of 2′,4′ -dihydroxy-6′ -methoxy-3′,5′ -dimethylchalcone against six established human cancer cell lines. Pharmacol Res 50:505–510.
- TK Yim, and KM Ko. (1999). Methylenedioxy group and cyclooctadiene ring as structural determinants of schisandrin in protecting against myocardial ischemia-reperfusion injury in rats. Biochem Pharmacol 57:77–81.