Abstract
Intraocular neovascularization is a complication in a variety of eye diseases, and is a leading cause of visual loss. The purpose of this study was to design and synthesize three novel codrugs of the antiangiostatic steroid, 3α, 17α, 21-trihydroxy-5β-pregnan-20-one (trihydroxy steroid, THS) with the cytotoxic agent 5-fluorouracil (5FU) which incorporates either one or two molecules of 5FU attached through carbonate ester linkages at positions O3, and/or O21 of the THS molecule. Furthermore, a kinetic study of the O3α-, O21-di-(N1-methyloxycarbonyl-2, 4-dioxo-5-fluoropyrimidinyL)17α-hydroxy-5β-pregnan-20-one (THS-BIS-5FU) codrug was carried out. The overall goal of this codrug strategy was to improve sustained drug delivery of both compounds by overcoming their individual solubility problems, and to thus enhance their bioavailability. The codrug was found to be optimal with superior angiostatic activity using the CAM assay compared to the activity of the parent compounds alone. In the hydrolysis studies 5FU was released at a faster rate than THS with an unknown intermediate observed by HPLC, a rationale and proposed structure and mechanism of the unknown THS derivative is provided.
Introduction
Angiogenesis is defined as the growth of new blood vessels from pre-existing vessels or capillaries [Citation1]. Under physiological conditions, this process is tightly regulated by both inhibitory and stimulatory factors [Citation2,Citation3]. An imbalance of these regulatory factors can lead to unabated neovascularization and the subsequent pathology associated with angiogenesis [Citation4,Citation5]. Unabated angiogenesis is the central pathological mechanism for the formation of childhood hemangiomas [Citation6,Citation7], in the development of new vessels to support tumor growth and metastasis [Citation8,Citation9], and in many ocular disorders which lead to blindness [Citation3,Citation4,Citation10,Citation11].
In 1985, Folkman and colleagues identified a group of steroids that exhibited angiostatic activity, but were devoid of glucocorticoid or mineralocorticoid activity; they classified these steroids as “Angiostatic Steroids” [Citation12] The most potent angiostatic steroid, trihydroxy steroid (THS) or 3α, 17α, 21-trihydroxy-5β-pregnan-20-one, had angiostatic properties similar to cortisone. THS suppresses the protease activity of the angiogenic protein, basic fibroblast growth factor (bFGF) [Citation13], and thus, has the capacity of inhibiting angiogenesis without the usual side effects associated with corticosteroids. However, the aqueous solubility of THS is only 12 μg/mL; thus, affording poor in vivo bioavailability. Because of its low aqueous solubility, THS is a strong candidate for prodrug or mutual prodrug (codrug) formulation. Mutual prodrugs (Codrugs) are bi-partate or tri-partate compounds that contain a covalent linker moiety between two or more synergistic compounds. Like prodrugs, codrugs are bioreversible derivatives of the component active parent compounds.
5-Fluorouracil (5FU) is a known cytostatic, antineoplastic agent; it is effective in the management of proliferative vitreoretinopathy (PVR), an infrequent complication of retinal reattachment surgery, which causes approximately 1600 cases of blindness each year in the United States [Citation14]. However, due to the high aqueous solubility (11 mg/mL) and rapid clearance of 5FU from the vitreous (t1/2 = 2.45 hr) [Citation15], multiple intravitreal injections of this drug are needed to maintain therapeutic levels of 5FU.
Taking into account the above information the co-administration of THS and 5FU in a sustained released formulation may be of therapeutic value by acting through mutually non-exclusive mechanisms in vivo. However, due to the pronounced difference in water-solubility of these two compounds, they cannot be formulated in a physical mixture that would allow for concomitant sustained release of both compounds in stoichiometric ratios. This is because if THS and 5FU were compressed into a pellet as a physical mixture, the more soluble 5FU would rapidly leach out of the pellet, leaving behind the less water-soluble THS.
An interesting approach for solving the above formulation problem is the synthesis of a novel mutual prodrug (codrug), which could be designed to provide a hydrolysable sustained release formulation of covalently linked THS and 5FU for the intra-ocular delivery of these two synergistic drugs that may have value in the treatment of chronic retinal angiogenesis. Once synthesized, the codrugs could be compressed into pellets. Upon dissolution, the codrug would be designed to rapidly hydrolyze to produce a concomitant and quantitative sustained release of THS and 5FU.
Covalent linkage of a lipophilic angiostatic steroid such as THS to an antiproliferative compound of high aqueous solubility, such as 5FU, would afford a hydrolysable codrug with improved physicochemical properties (i.e. intermediate between the water-insoluble THS molecule and the highly water-soluble 5FU molecule) leading to an increase in the bioavailability of both parent drugs with an expected increase in angiostatic efficacy. Ideally, the THS-5FU codrug implant would be administered for sustained delivery to prevent occurrence of angiogenesis and/or retinal detachments resulting from proliferation of fibrovascular networks.
Carbonate ester modification of active drug substances is a useful mechanism for the development of novel prodrugs and was found to exhibit beneficial pharmacological properties [Citation16,Citation17,Citation18]. It is expected that the proposed THS-5FU codrug with carbonate ester linkage would improve ophthalmic drug delivery by enhancing drug penetration, and reducing systemic or ocular side effects. Such codrugs would benefit from their aqueous stability in vitro, coupled with their high susceptibility to esterase-mediated hydrolysis [Citation19].
In designing linker units to covalently link the THS molecule to the 5FU molecule, a careful consideration of which functional groups are to be modified should be made, to determine if they are amenable to derivatization; this will also allow for an appropriate ratio of the two parent drugs to be linked together. THS contains three hydroxyl functionalities that could be possible sites of attachment for 5FU moieties via a carbonate linker. However, the 17α-hydroxyl functionality is sterically hindered, and not amenable to derivatization. The 3α and 21 hydroxyls can both be derivatized. These considerations prompted us to design new codrugs where it would be possible to covalently link either 1 or 2 molar equivalents of 5FU to 1 molar equivalent of THS, to afford novel THS-5FU codrugs.
In this study we describe the synthesis of three novel codrugs of THS and 5FU, in addition to a primary pharmacological evaluation, and an in vitro kinetic study on stoichiometrically the most attractive codrug: O3α-, O21-di-(N1-methyloxycarbonyl-2, 4-dioxo-5-fluoropyrimidinyL)17α-hydroxy-5β-pregnan-20-one, THS-BIS-5FU codrug, which was carried out in order to determine that the rate of hydrolysis of the codrug to the component parent drugs was appropriate for efficient drug delivery.
Materials and methods
Materials and reagents
Chicken embryos were obtained from Sunrise Farms, Catskill, NY. Bovine vitreous was obtained from the Bourbon County Meat Locker, and human serum was obtained from the Clinical Research Center at the University of Kentucky.
5-Fluorouracil (5FU), 5-Fluorocytosine (5FC), Reichstein's Substance S (RSS), formaldehyde, phosgene, hydrochloric acid, ethanol, benzene, tetrahydrofuran, triethylamine, and other chemicals and solvents used in the synthesis procedure were obtained from Aldrich Chemical Co. (Milwaukee, WI). Sodium phosphate (monohydrate, monobasic), acetonitrile, and HCl were purchased from Fisher Scientific.
NMR instrumentation
1H-NMR and 13C-NMR spectra were carried out on a Varian VXR-300 MHz spectrometer (Varian, Palo Alto, CA); spectra were run at 21°C in CDCl3 or DMSO-d6 using tetramethyl silane (TMS) as an internal standard. High resolution electron impact ionization mass spectra were recorded at 70 eV on a JEOL JMS-700T M Station (magnetic sector instrument) at a resolution of greater than 10000. Samples were introduced via heatable direct probe inlet. Perfluorokerosene (pfk) was used to produce reference masses.
HPLC instrumentation
The HPLC analytical system used for the codrug kinetic studies consisted of an L-4000 UV detector, an L-6000 intelligent pump, an AS-2000 autosampler, and a D-2500 chromato-integrator, Hitachi (Tokyo, Japan).
For the analysis of THS and THS-BIS-5FU, the column utilized was an Axxion C-18 reverse phase ODS column (25 cm × 4 mm × 5 μm) preceded by a Rainin C-18 pre-column (Hamilton, USA). The mobile phase consisted of 50% acetonitrile and 50% water (pH 6.0), at a flow rate of 1 mL/min and UV detection was at 208 nm. For the analysis of 5FU, the column utilized was a Sphereclone 2 ODS 5 (25 cm × 0.46 cm I.D.) purchased from Phenomenex (Torrence, CA, USA), preceded by a Hitachi C-18 guard column (Tokyo, Japan). The mobile phase consisted of phosphate buffer (pH 3.5, 1.0 M) at a flow rate of 1 ml/min and UV detection was at 270 nm.
Synthesis of THS-5FU codrugs
17α,20:20,21-Bis-(methylenedioxy)pregn-4-ene-3-one (2)
Reichstein's Substance S (1, 1 g, 2.9 mmol) was dissolved with stirring in CH2Cl2 (30 mL), and a solution of 37% w/v formalin (2.4 eq., 20 mL) and conc. HCl (2.5 eq., 20 mL) was added. The resulting mixture was stirred at room temperature for 24 h, after which the organic and aqueous phases were separated. The organic phase was washed with aqueous NaHCO3 solution (2 × 50 mL), water (2 × 50 mL), and then with saturated aqueous NaCl solution (50 mL). The aqueous phase was washed once with CH2Cl2 (50 mL), and the combined CH2Cl2 solutions were dried over anhydrous Na2SO4 for 12 h. The solution was then filtered and the solvent removed on a rotary evaporator, in vacuo, to afford a white powder. This product was dried under high vacuum (0.01 mm Hg) overnight, and recrystallized from a mixture of CH2Cl2 and diethyl ether. Upon standing at ambient temperature, a product crystallized out and was identified as 17α, 20:20, 21-bis-(methylenedioxy)pregn-4-ene-3-one (2), m.p. 191°C (lit. 192–195°C) [Citation20]. 1H-NMR (CDCl3): δ 5.72 (s, 1H, COCHC), 5.12 (m, 4H, COCH2OCOCH2OCH2), 3.95 (d of d, 2H, J = 18.6 Hz, COCH2OCOCH2OCH2), 2.51-0.91 (complex mixture of multiplets, 19-H), 1.19 (s, 3H, CH3), 0.88 (s, 3H, CH3) ppm.
17α,20:20,21-Bis-(methylenedioxy)5β-pregnan-3-one (3)
A modification of the previous reported synthesis was utilized [Citation21]. A suspension of 2 (0.7 g, 1.8 mmol) in a mixture of 95% ethanol (40 mL), KOH (90 mg), and 5% Pd-CaCO3 (100 mg) was hydrogenated at 50 psig and 25°C for 17 h. The suspension was then filtered through celite, and the celite pad was washed with CH2Cl2 (30 mL). The filtrate and washings were collected, combined, and the organic liquors were removed by rotary evaporation in vacuo. The resulting crude product was obtained as an amorphous foam with a light yellow tint. The product was dissolved in ethyl acetate (50 mL), the solution was washed with water (2 × 50 mL), and then with aqueous NaCl solution (2 × 50 mL). The ethyl acetate phase was separated and dried over anhydrous Na2SO4 for 12 h. The solution was then filtered and the solvent was removed by rotary evaporation in vacuo, to afford 17α, 20:20, 21-bis-(methylenedioxy)5βpreg-nan-3-one (3), as an amorphous foam, in an overall yield of 0.7 g (91%). 1H-NMR (CDCl3): δ 5.12 (m, 4H, COCH2OCOCH2OCH2), 3.95 (d of d, 2H, 19.1 Hz, COCH2OCOCH2OCH2), 2.85-1.04 (complex mixture of multiplets, 22-H), 1.01 (s, 3H, CH3), 0.82 (s, 3H, CH3) ppm.
17α,20:20,21-Bis-(methylenedioxy)3α-hydroxy-5β-pregnane (4)
A solution of 3 (0.7 g, 1.8 mmol) in tetrahydrofuran (10 mL) and absolute ethanol (15 mL) was cooled to 0°C. Sodium borohydride (200 mg) was added to the cooled solution. The mixture was stirred at 0°C for 10 min, and then at room temperature for 2 h. The resultant mixture was evaporated to half its volume by rotary evaporation in vacuo. Diethyl ether (100 mL) was then added to the mixture, and the resulting solution was washed with water (3 × 50 mL), and then with aqueous NaCl solution (50 mL). The organic phase was separated and dried over anhydrous MgSO4 for 12 h. The solution was filtered and the solvent was removed by rotary evaporation in vacuo. The resulting crude product was purified by column chromatography using Al2O3 and 6% water in benzene:acetone (15:1) as eluent. The overall yield of 17α, 20:20, 21-bis-(methylenedioxy)3α-hydroxy-5β-pregnane (4) was 0.28 g (40%). The purified product was obtained as white amorphous foam. 1H-NMR(CDCl3): δ 5.12 (m, 4H, COCH2OCOCH2OCH2), 3.95 (d of d, 2H, J = 19.2 Hz, COCH2OCOCH2OCH2), 2.85-1.04 (complex mixture of multiplets, 22-H), 3.64 (m, 1H, CHOH), 0.91 (s, 3H, CH3), 0.80 (s, 3H, CH3) ppm.
3α,17α,21-trihydroxy-5β-pregnan-20-one (THS) (5)
A solution of 4 (0.27 g, 0.7 mmol) in tetrahydrofuran (5 mL) was added to 48% hydrofluoric acid (10 mL) in a plastic container and stirred for 2.5 h. To the reaction mixture was added water (150 mL), then sodium bicarbonate (40 g). The resulting aqueous mixture was extracted with ethyl acetate (2 × 200 mL). The ethyl acetate phases were combined, washed with water (2 × 50 mL), then with saturated aqueous NaCl solution (2 × 50 mL), and dried over anhydrous Na2SO4 for 12 h. The solution was filtered and the solvent removed by rotary evaporation in vacuo. The resulting crude product was purified by silica gel column chromatography using a benzene:acetone (15:1) gradient as the eluent. The overall yield of 3α, 17α, 21-trihydroxy-5β-pregnan-20-one (THS) (5) was 0.17 g (70%). The purified product was obtained as a white powder, m.p. 215°C (lit. 212-216°C) [Citation22]. 1H-NMR (CDCl3): δ 4.51 (d of d, J = 19.0 Hz, 2H, COCH2OH), 3.64 (m, 1H, CHOH), 2.69-1.09 (complex mixture of multiplets 22H), 0.90 (s, 3H, CH3), 0.62 (s, 3H, CH3) ppm. 13C-NMR (CDCl3): δ 212.5 (CO), 89.8 (COH), 71.9 (CHOH), 67.8 (CH2OH), 51.1 (CH), 49.1 (CH2), 42.4 (CH), 40.2 (CH), 38.2 (CH2), 38.0 (CH), 35.2 (CH2), 34.6 (CH2), 31.3 (CH2) 31.1 (CH2), 29.0 (CH2), 28.7 (CH2), 26.3 (CH2), 23.9 (C), 23.8 (CH3), 21.1 (C), 15.7 (CH3) ppm.
O3α,O21-Di-(chloroformyl)-17α-hydroxy-5β-pregnan-20-one (6)
THS (5) (400 mg, 1.14 mmol) was placed into a reaction flask and the flask was purged with dry nitrogen gas. Anhydrous tetrahydrofuran (15 mL) was added to the flask and the resulting solution was cooled to 0°C in an ice bath. Phosgene (20% in toluene) (85 mL, containing 2.2 equivalents of phosgene) was placed into a separate flask and cooled to 0°C. The THF solution of 5 was then added drop-wise to the phosgene solution over a period of 10 min using the double ended needle technique. The resulting reaction mixture was stirred at 0°C for 30 min, then at room temperature overnight. The mixture was evaporated on a rotary evaporator in vacuo, and the resulting product, O3α, O21-di(chloroformyl)17α-hydroxy-5β-pregnan-20-one (6), was obtained as a white powder in an overall yield of 494 mg (91%). 1H-NMR (CDCl3): δ 5.12 (d of d, 2H, J = 18.9 Hz, CH2OCOCl), 4.83 (m, 1H, CHOCOCl), 2.06-1.00 (complex mixture of multiplet, 22-H), 0.92 (s, 3H, CH3), 0.63 (s, 3H, CH3) 13C-NMR (CDCl3): δ 202.5 (CO), 151.0 (OCOCl), 149.7 (OCOCl), 90.3 (COH), 83.5 (CHOCOCl), 73.7 (CH2OCOCl), 51.3 (CH), 48.9 (C), 41.7 (CH), 40.1 (CH), 35.7 (CH2), 35.5 (CH2), 34.7 (CH2), 34.5 (CH2), 31.6 (CH2), 30.5 (CH2), 26.7 (C), 26.3 (CH2), 26.1 (CH2), 23.5 (CH2), 23.0 (CH3), 20.4 (CH2), 14.6 (CH3) ppm; exact mass: 474.4020, high resolution MS: 474.4031.
N1,N3-Di-(hydroxymethyl)-5-fluorouracil (8)
This compound was prepared utilizing a modification of the method of Ahmad et al [Citation23]. A 37% aqueous solution of formalin (0.137 g, 4.6 mmol) was added to 5FU (7) (100 mg) and the mixture was stirred at 60°C for 2 h. The solution was evaporated to dryness, in vacuo, on a rotary evaporator. The resulting product, N1, N3-di-(hydroxymethyl)-5-fluorouracil (8) was obtained as a clear, viscous liquid in an overall yield of 176 mg (92%). 1H-NMR (DMSO-d6): δ 8.22 (d, 1H, JHF = 5.4 Hz, CH), 5.0-5.8 9 (d, 4H, J = 18.5 Hz, CH2OH) ppm.
O3α-,O21-Di-(N1-methyloxycarbonyl-2,4-dioxo-5-fluoropyrimidinyl)17α-hydroxy-5β-pregnan-20-one (11)
O3α, O21-Di-(chloroformyl)17α-hydroxy-5β-pregnan-20-one (6) (400 mg, 0.84 mmol) was placed into a reaction flask, and the flask was purged with dry nitrogen gas. Anhydrous acetonitrile (30 mL) was added to the reaction flask to afford a solution of 6. N1, N3-di-(hydroxymethyl)-5-fluorouracil (8) (271 mg; 2.2 equivalents) was placed into a second reaction flask, and the flask was purged with dry nitrogen gas. Anhydrous acetonitrile (5 mL) was added to the reaction flask to afford a solution of 8. Anhydrous triethylamine (210 μL, 2.2 equivalents) was added to the solution of 8. Both solutions of 6 and 8 were cooled to 0°C for 10 min and the solution of 8 was then added drop-wise to the solution of 6 over a period of 10 min. The resulting solution was stirred for 24 h at room temperature. The reaction products were fractionated and purified by silica gel column chromatography using 100% chloroform as the mobile phase, yielding the following pure products: O3α-(N1-methyloxycarbonyl-2,4-dioxo-5-fluoropyrimidinyl)17α-hydroxy-5β-pregnan-20-one (9), O21-(N1-methyloxycarbonyl-2,4-dioxo-5-fluoropyrimidinyl)17α-hydroxy-5β-pregnan-20-one (10), and O3-, O21-di(N1-methyloxycarbonyl-2,4-dioxo-5-fluoropyrimidinyl)17α-hydroxy-5β-pregnan-20-one (THS-BIS-5FU) (11).
For 9: 1H-NMR (DMSO-d6): δ 8.13 [d, 1H, JHF = 4.5 Hz, CH(5FU)], 5.58 (s, 2H, NCH2O), 4.58 (d of d, 2H, J = 19.0 Hz, COCH2O), 4.53 (m, 1H, CHOCO2), 2.22-0.92 (complex mixture of multiplets, 22H), 1.71 (s, 3H, CH3), 1.58 (s, 3H, CH3) ppm. 13C-NMR (DMSO-d6): δ 205.6 (CO), 157.2 [CO (5FU-C2)], 152.9 (CO (5FU-C4)), 149.1 (COOCH2N), 138.5 (F), 129.5 (5FU-CH), 88.8 (COH), 78.4 (CHOCOOCH2), 73.1 (COOCH2N), 67.1 (CH2OH), 50.5 (CH), 47.3 (C), 41.0 (CH), 35.4 (CH), 36.1 (CH2), 34.3 (CH2), 34.3 (CH2), 33.6 (CH2), 31.5 (CH2), 30.0 (C), 26.3 (CH2), 26.1 (CH2), 23.4 (CH2), 23.3 (CH3), 20.1 (CH2), 14.1 (CH3) ppm; exact mass: 536.5898, high resolution MS: 536.5891.
For 10: 1H-NMR (DMSO-d6): δ 8.12 [d, 1H, JHF = 5.6 Hz, CH(5FU)], 5.61 (d, 2H, J = 18 Hz, NCH2O), 4.99 (d of d, 2H, J = 19.1 Hz, COCH2O), 3.68 (m, 1H, CHOCO2), 2.22-0.92 (complex mixture of multiplets 22H), 1.71 (s, 3H, CH3), 1.50 (s, 3H, CH3) ppm. 13C-NMR (DMSO-d6): δ 205.8 (CO), 157.4 [CO (5FU-C2)], 153.4 [CO (5FU-C4)], 149.2 (COOCH2N), 140.9 (F), 129.3 (5FU-CH), 90.1 (COH), 74.2 (CHOH), 73.5 (COOCH2N), 71.2 (CH2OCOO), 50.3 (CH), 47.8 (C), 41.0 (CH), 35.4 (CH), 36.6 (CH2), 34.1 (CH2), 39.9 (CH2), 33.6 (CH2), 31.5 (CH2), 27.4 (C), 26.4 (CH2), 26.1 (CH2), 23.1 (CH2), 23.3 (CH3), 20.6 (CH2), 14.1 (CH3) ppm; exact mass: 536.5898, high resolution MS: 536.5888.
For 11: 1H-NMR (DMSO-d6): δ 8.13 [d, 1H, JHF = 4.5 Hz, CH(5FU)], 8.12 [d, 1H, JHF = 5.6 Hz, CH(5FU)], 5.61 (d, 2H, J = 18.0 Hz, NCH2O), 5.58 (s, 2H, NCH2O), 4.99 (d of d, 2H, J = 18.5 Hz, COCH2O), 4.53 (m, 1H, CHOCO2), 2.22-0.92 (complex mixture of multiplets, 22H), 1.71 (s, 3H, CH3), 1.50 (s, 3H, CH3) ppm. 13C-NMR (DMSO-d6): δ 205.6 (CO), 157.5-157.2 [d, CO (5FU-C2)], 153.2-152.9 [d, CO (5FU-C4)], 149.2 (COOCH2N), 149.1 (COOCH2N), 141.0 (F), 137.9 (F), 129.8 (5FU-CH), 129.3 (5FU-CH), 88.8 (COH), 78.4 (CHOCOO), 73.5 (COOCH2N), 73.1 (COOCH2N), 71.2 (CH2OCOO), 50.3 (CH), 47.8 (C), 41.0 (CH), 35.4 (CH), 36.1 (CH2), 34.2 (CH2), 34.1 (CH2), 33.6 (CH2), 31.6 (CH2), 30.0 (C), 26.4 (CH2), 26.1 (CH2), 23.3 (CH2), 23.3 (CH3), 20.0 (CH2), 14.1 (CH3) ppm; exact mass: 722.6873, high resolution MS: 722. 6865.
Efficacy studies using the chick chorioallantoic membrane (CAM) assay
Preliminary studies of the angiostatic properties of THS, 5FU, THS:5FU physical mixtures, and the codrug THS-BIS-5FU were assessed using a modification of the chick chorioallantoic membrane (CAM) assay described by Crum [Citation12]. Briefly, the chicken embryos were cracked and then placed in petri dishes in a humidified CO2 incubator (3% CO2/air) at 37°C on day 3. At day 6, chick embryos were removed from the incubator and each received a methylcellulose implant on the CAM. At least 20 embryos were implanted with pellets for each combination of the two drugs. The embryos were then placed in the incubator for a further 48 h. On day 8, chick embryos were removed from the incubator and the angiostatic activity of each combination of pelleted drugs was evaluated. An avascular zone of ≥ 4 mm at the site of implantation indicated a positive response; otherwise a negative response was indicated. Results were tabulated as the number of positive responses over the total embryos counted for each combination of pelleted drugs. The percent positive response was also determined.
Pellets of THS, 5FU, THS:5FU physical mixtures in a 1:1 and a 1:2 ratio were prepared, and the bis-codrug at two concentrations, each of these was suspended separately in 700 μL of 10% aqueous methylcellulose containing 2% DMSO. A 10 μL volume of each of the aqueous methylcellulose suspensions was pipetted onto a sterile teflon surface and allowed to air-dry for 30 min, this produced methylcellulose disks approximately 2 mm in diameter. The methylcellulose disks were removed from the teflon surface with sterile forceps and placed onto the 6-day old chick chorioallantoic membrane (CAM).
Samples of THS, 5FU were prepared at concentrations of 2.5 μg/mL, 5.0 μg/mL, and 10 μg/mL. Thus, 3.5 mg of THS was mixed with 1.3 mg of 5FU, and 3.5 mg of THS was mixed with 2.6 mg of 5FU, to afford physical mixtures of THS:5FU in a 1:1, and 1:2 molar ratio, respectively. In addition 7.0 mg of THS-BIS-5FU was utilized to simulate a high dose of the physical mixture of THS:5FU at the 1:2 molar ratio (high dose), and 3.5 mg of THS-BIS-5FU was utilized to simulate a lower dose of the physical mixture of THS:5FU at the 1:2 molar ratio (low dose).
Hydrolysis studies
Hydrolysis studies on the THS-BIS-5FU codrug were performed in 0.1 M phosphate buffer (pH 7.4), human serum, and bovine vitreous humor.
A 200 μL volume of the THS-BIS-5FU codrug stock solution (1 mg/mL acetonitrile) was placed into a separate conical test tube containing 1.8 mL of either 0.1 M phosphate buffer (pH 7.4), human serum, or bovine vitreous humor and placed in water bath at 37°C. 200 μL aliquots of the phosphate buffer incubate were removed for analysis at 0, 10, 30, 60, 120, 180, and 240 min and were analyzed directly in triplicate by HPLC.
Plasma and vitreous humor samples were treated in the following manner except that aliquots of these incubates were removed at 0, 10, 30, 60 and 120 min. Briefly, a 200 μl volume of either plasma or vitreous humor was placed into a 1.5 ml Eppendorf tube. The sample was precipitated with 800 μl of acetonitrile and brought to a final volume of 1 ml. The 1 ml sample was vortexed and centrifuged at 8,000 g for 2 min. A 200 μl aliquot part of the organic supernatant was removed and treated with a mixture of 200 μl of acetonitrile and 600 μl of ethyl acetate. The sample was vortexed and centrifuged for 2 min. An 800 μl portion of the organic layer was then transferred to a 1.5 ml Eppendorf tube and evaporated to dryness in vacuo. The residue was then reconstituted with 200 μl of mobile phase, and the solution was injected onto the HPLC column and analyzed in triplicate.
Isolation and identification of an unknown intermediate from the hydrolysis of O3α-,O21-di-(N1-methyloxycarbonyl-2,4-dioxo-5-fluoropyrimidinyL)17α-hydroxy-5β-pregnan-20-one codrug [THS-BIS-5FU]
A 1 mg sample of O3α-, O21-di-(N1-methyloxycarbonyl-2, 4-dioxo-5-fluoropyrimidinyL)17α-hydroxy-5β-pregnan-20-one codrug THS-BIS-5FU was placed in a 15 mL conical test tube. A volume of 10 mL of human serum was added to the test tube. The serum sample was vortexed and placed in a water-bath at 37°C. The sample was vortexed every 5 min for 1 h. After 1 h, the serum was deproteinated by addition of 10 mL of acetonitrile. The sample was vortexed and centrifuged at 14,000 rpm for 10 min. The supernatant was separated and extracted with 50 mL of ethyl acetate, and the organic layer was removed and evaporated to dryness by rotary evaporation. The residue was reconstituted in mobile phase and purified by preparative HPLC, using the instrumentation and conditions described for the purification of THS and THS-BIS-5FU codrug. The mobile phase consisted of 50% v/v acetonitrile and 50% v/v water, pH 6.0. The unknown intermediate was collected in the mobile phase, and a 200 μL sample was removed and re-injected onto the HPLC analytical column, to test for purity. The remaining sample was flash-frozen, and then lyophilized. The residue was dissolved in CDCl3 for 1H-NMR spectroscopic analysis and structural identification. Analysis of the 1H-NMR spectrum indicated that the unknown intermediate was not stable to the lyophilization process and partially degraded to THS; this was verified by HPLC analysis. The 1H-NMR analysis of the unknown intermediate had: 1H-NMR (CDCl3): δ 4.48 (d of d, 2H, J = 18 Hz, COCH2O), 4.29 (d:d, 2H, COCH2O), 4.05 (m, 1H, CHOH), 3.61 (m, 1H, CHOH), 2.69-1.09 (fingerprint region containing 44-H's by integration), 0.90 (s, 3H, CH3), 0.89 (s, 3H, CH3), 0.62 (s, 6H, CH3), ppm.
Results
In the CAM assay 5FU exhibited an unexpected decrease in angiostatic activity with increasing dose. This inverse dose-response inhibition of angiogenesis in the chick embryo experiments (the CAM assay) might result from cytotoxic effects of the higher concentrations of 5FU on embryonic normal tissues [Citation24]. In this respect, high doses of 5-FU have been reported to cause toxicity in in vivo studies. As expected, THS did not exhibit angiostatic activity at any of the concentrations tested in this assay. However, the physical mixtures of THS and 5FU in a 1:1 molar ratio and in a 1:2 molar ratio both demonstrated synergistic effects in the CAM assay, with the 1:2 molar ratio showing the highest percent inhibition of angiogenesis (45.5%) (). When comparing the angiostatic response of the THS:5FU physical mixture in a 1:2 molar ratio with the THS-BIS-5FU codrug at the same dose equivalent (i.e. 50 μg THS and 36 μg 5FU) the codrug showed superior angiostatic activity of around 70.6%, compared to 45.5% for the physical mixture. This might be related to the nature of the hydrolysis kinetics of the codrug that might facilitate longer exposure of the cells to THS and 5FU.
Table I. The angiostatic response between 5FU, THS, physical mixtures of THS and 5FU versus the THS-5FU codrug at varying dosages. Angiostatic effect is determined by the presence of an avascular zone. An avascular zone of ≥4 mm at the site of implantation indicates a positive response, otherwise a negative response is indicated.
Based upon the above data from the CAM assay it was deemed appropriate to design and synthesize O3α-,O21-di-(N1-methyloxycarbonyl-2,4-dioxo-5-fluoropyrimidinyl)17α-hydroxy-5β-pregnan-20-one codrug THS-BIS-5FU (11) in which the molar ratio was (1:2) THS: 5FU.
Chemical synthesis of THS was achieved utilizing Reichstein's Substance S (RSS) as starting material (). RSS was treated with 37% aqueous formaldehyde in the presence of concentrated hydrochloric acid to yield 17α, 20:20, 21-bis-(methylenedioxy)pregn-4-ene-3-one (2). This reaction serves to protect the 17α-side chain in preparation for subsequent double bond reduction in the A-ring [Citation25]. The A-ring 4, 5 double bond of the bis-methylenedioxy derivative of RSS was reduced using H2 gas over palladium-on-calcium carbonate as a catalyst, in ethanolic potassium hydroxide [Citation25,Citation26]. Reduction of the resulting 3-keto derivative (3) was achieved with NaBH4, using a modified procedure of the method described by Vandenheuvel [Citation27], after which the product (4) was deprotected using hydrofluoric acid, to yield THS (5). THS was treated with phosgene in a mixture of benzene and tetrahydrofuran, with triethylamine to quench the formation of HCl [Citation23]. Chloroformylation of THS proceeded to completion, with the formation of only one product, O3α, O21-di-(chloroformyl)-17α-hydroxy-5β-pregnan-20-one (6). Synthesis of bis-hydroxymethyl-5FU from 5FU was accomplished using a procedure described by Ahmad et al. [Citation23]. Treatment of 5FU (7) with 37% aqueous formalin gave a clear, viscous liquid identified as N1, N3-di-(hydroxymethyl)-5-fluorouracil (8). The reactions of dichloroformylated-THS (6) with N1, N3-di-(hydroxymethyl)-5-fluorouracil (8) proceeded rapidly yielding 3 products: i.e. the desired codrug; O3α-,O21-di-(N1-methyloxycarbonyl-2,4-dioxo-5-fluoropyrimidinyl)17α-hydroxy-5β-pregnan-20-one (11), O3α-(N1-methyloxycarbonyl-2,4-dioxo-5-fluoropyrimidinyl)17α-hydroxy-5β-pregnan-20-one (9), and O21-(N1-methyloxycarbonyl-2,4-dioxo-5-fluoropyrimidinyl)17α-hydroxy-5β-pregnan-20-one (10) ().
Figure 1 Synthesis of 3α-O, 21-O-(dichloroformyl)-5β-pregnane-17-ol-20-one (5) from Reichstein's Substance S (1).
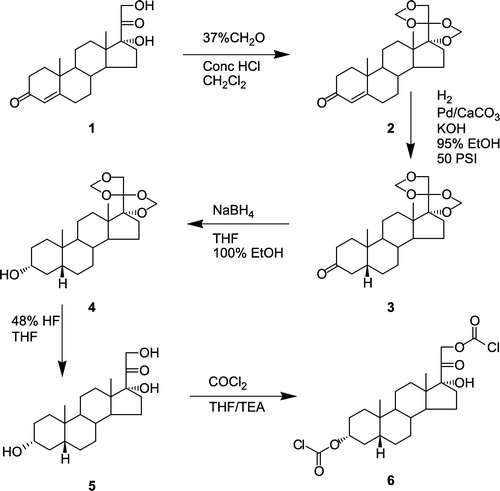
Figure 2 Coupling of 3α-O, 21-O-(dichloroformyl)-5β-pregnane-17-ol-20-one (6) and N1,N3-Bis-dihydroxymethyl 5-fluorouracil (8) to produce O3-(N1-methyloxycarbonyl-5-fluorouracil)5-β-pregnane-17-ol-20-one (9),O21-(N1-methyloxycarbonyl-5-fluorouracil)5-β-pregnane-17-ol-20-one (10), and O3-, O21-Di(N1-methyloxycarbonyl-5-fluorouracil)5-β-pregnane-17-ol-20-one; (THS-BIS-5FU) (11).
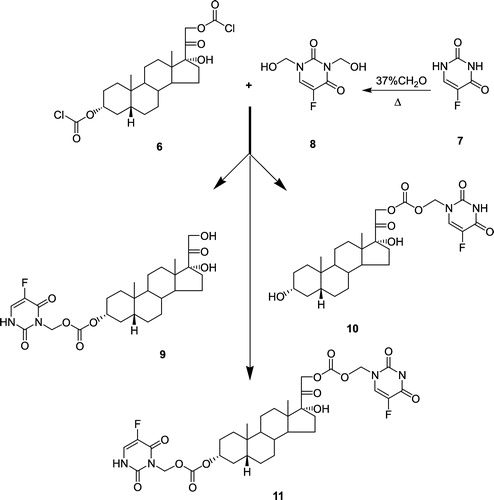
Hydrolysis studies for the O3α-, O21-di-(N1-methyloxycarbonyl-2, 4-dioxo-5-fluoropyrimidinyL)17α-hydroxy-5β-pregnan-20-one codrug THS-BIS-5FU were conducted in 0.1 M phosphate buffer, human plasma, and bovine vitreous humor. Samples of THS in buffer extracted with acetonitrile could be injected onto the HPLC system without compromising the efficiency of the analytical column. However, inorganic salts present in the sample caused significant tailing of the solute front at the low wavelength (208 nm) employed for detection. Likewise, samples of THS and 5FU in biological media deproteinated with acetonitrile could also be injected directly onto the HPLC system without compromising the efficiency of the analytical column. However, the chromatograms were complicated by the presence of endogenous peaks in the biological matrix. In order to remove the salt front from the buffer samples and the endogenous peaks from the biological samples, an additional step, involving extraction into ethyl acetate was necessary. Following extraction, the samples were evaporated to dryness to completely remove all traces of ethyl acetate, and the resulting residue was reconstituted in HPLC mobile phase. Injection of the resulting sample solutions produced HPLC chromatograms devoid of interfering peaks.
Hydrolysis of O3α-, O21-di-(N1-Methyloxycarbonyl-2, 4-dioxo-5-fluoropyrimidinyL)17α-hydroxy-5β-pregnan-20-one codrug THS-BIS-5FU will afford 1 molar equivalent of THS and 2 molar equivalents of 5FU ().
Figure 3 Proposed hydrolysis of the model THS-BIS-5FU codrug (11). One molar equivalent of codrug should generate 1 molar equivalent of THS, 2 molar equivalents of 5FU, and 2 molar equivalents of both formaldehyde and carbon dioxide.
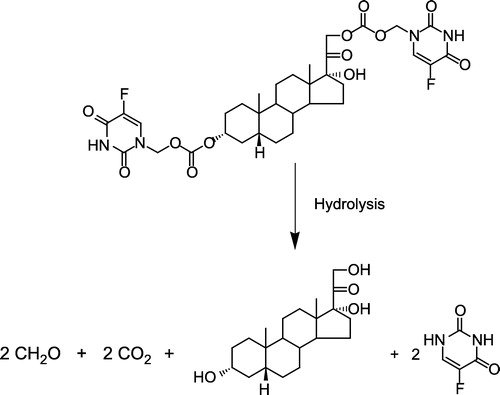
The T1/2 of hydrolysis for O3α-, O21-di-(N1-Methyloxycarbonyl-2, 4-dioxo-5-fluoropyrimidinyL)17α-hydroxy-5β-pregnan-20-one codrug THS-BIS-5FU was 3.2 hours in 0.1 M phosphate buffer (pH 7.4), 42.5 minutes in human serum, and 45.0 minutes in bovine vitreous humor.
The rate of disappearance of the THS-BIS-5FU codrug (11) should correlate with the rate of appearance of both THS and 5FU. Analysis of the hydrolysis products of the THS-BIS-5FU codrug demonstrated the release of both THS and 5FU in all media (, and ) indicating that THS-BIS-5FU is truly a codrug. However, the hydrolysis data indicates that THS and 5FU do not appear to be simultaneously produced in the expected ratio of 1:2, respectively. For THS-BIS-5FU codrug, the rate of disappearance is similar in both serum and vitreous, and is much faster in the biological media than in 0.1 M phosphate buffer (pH 7.4). Consequently, the rate of appearance of THS and 5FU is faster in both serum and vitreous humor compared to 0.1 M phosphate buffer (pH 7.4). The rate of appearance of 5FU correlates with the rate of disappearance of the THS-BIS-5FU codrug; the release of 5FU from the codrug is immediate and complete, eventually reaching a plateau. In comparison, the first appearance of THS is initially delayed, and the rate of appearance is significantly slower than that of 5FU. THS then continues to be released even after 5FU concentrations have plateaud. Thus, the THS:5FU molar ratio increases with time. The expected ratio of 1:2 THS:5FU is not reached during the experimental time period examined. Since the regeneration of 5FU and THS in the expected molar ratios, is dependent on complete hydrolysis of THS-BIS-5FU codrug at the linker moieties, it is possible that partial or incomplete hydrolysis is occurring, or the THS-BIS-5FU codrug is being hydrolyzed into 5FU and some intermediate product, which is then subsequently further hydrolyzed, at a slower rate, into THS. The data suggest the latter is occurring due to the formation of two equivalents of 5FU and the disappearance of the codrug during the hydrolysis studies.
Figure 4 The appearance of 5FU (•) and THS (▴) in molar concentration from the degradation of THS-BIS-5FU codrug (▪) in phosphate buffer (0.1 M, pH 7.4).
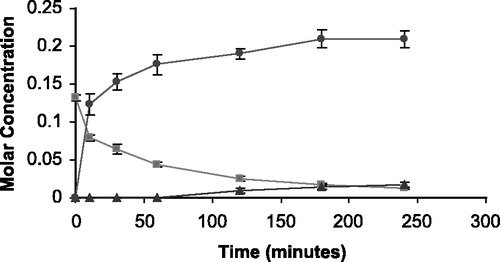
Figure 5 The appearance of 5FU (•) and THS (▴) in molar concentration from the degradation of THS-BIS-5FU codrug (▪) in human serum.
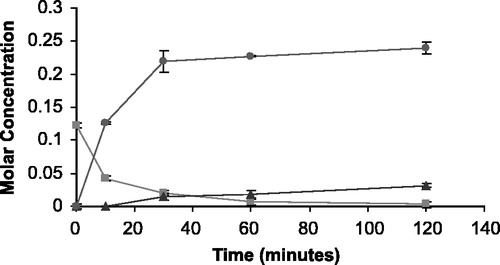
Figure 6 The appearance of 5FU (•) and THS (▴) in molar concentration from the degradation of THS-BIS-5FU codrug (▪) in bovine vitreous humor.
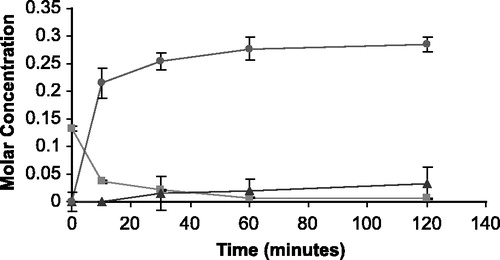
Further analysis of the HPLC chromatograms from the hydrolysis of THS-BIS-5FU codrug demonstrated the generation and subsequent disappearance of an uncharacterized, UV-detectable peak, with a retention time of 9.1 minutes. The retention time of this product suggests the generation of an unknown intermediate that is less polar than THS. THS has a retention time of 7.4 minutes. The rate of appearance of this unknown peak correlates with the disappearance of the THS-BIS-5FU codrug and the subsequent disappearance of the unknown peak appears to correlate with the appearance of THS. To determine the exact mechanism of hydrolysis of the THS-BIS-5FU codrug, it was deemed necessary to isolate and characterize the above unknown intermediate.
After isolation by preparative HPLC, a sample of the unknown intermediate was re-injected onto the HPLC column, to test for purity. Standard stock solutions of THS and both the mono-5FU-THS codrugs were injected onto the HPLC column. All three standards had different retention times than the retention time of the isolated hydrolysis intermediate. O21-(N1-methyloxycarbonyl-2,4-dioxo-5-fluoropyrimidinyl)17α-hydroxy-5β-pregnan-20-one codrug (10) had a retention time of 24 minutes, while the O3α-(N1-methyloxycarbonyl-2,4-dioxo-5-fluoropyrimidinyl)17α-hydroxy-5β-pregnan-20-one codrug (9) had a retention time of 35 minutes, and THS had a retention time of 7.4 minutes. Each of these data taken together demonstrates that the hydrolysis intermediate is neither THS, nor any of the mono-substituted THS-5FU codrugs (9) and (10). NMR spectrophotometric analysis, as expected, indicated that no 5FU moiety was present in the structure of the intermediate, demonstrating that 5FU had been cleaved from the molecule at both the 3α, and the 21 sites of the THS-BIS-5FU (11) codrug. NMR spectrophotometric analysis also suggested that the intermediate was a THS analogue, probably containing one or two carbonic acid moieties.
Discussion
Chemical synthesis of THS was achieved utilizing Reichstein's Substance S (RSS) as starting material. THS then was treated with phosgene in a mixture of benzene and tetrahydrofuran, with triethylamine added to quench the formation of HCl. Chloroformylation of THS proceeded to completion, with the formation of only one product, O3α, O21-di-(chloroformyl)-17α-hydroxy-5β-pregnan-20-one (6). Treatment of 5FU (7) with 37% aqueous formalin gave a clear, viscous liquid identified as N1, N3-di-(hydroxymethyl)-5-fluorouracil (8). The reactions of dichloroformylated-THS (6) with N1, N3-di-(hydroxymethyl)-5-fluorouracil (8) proceeded rapidly yielding 3 products: i.e. the desired bis-codrug; O3α-,O21-di-(N1-methyloxycarbonyl-2,4-dioxo-5-fluoropyrimidinyl)17α-hydroxy-5β-pregnan-20-one (11), and the mono codrugs: O3α-(N1-methyloxycarbonyl-2,4-dioxo-5-fluoropyrimidinyl)17α-hydroxy-5β-pregnan-20-one (9), and O21-(N1-methyloxycarbonyl-2,4-dioxo-5-fluoropyrimidinyl)17α-hydroxy-5β-pregnan-20-one (10).
In preliminary studies, 5FU exhibited low angiostatic activity in the chick chorioallantoic membrane (CAM) assay over the doses ranges studied (25-100 μg), whereas THS demonstrated no angiostatic activity when administered alone in the CAM assay, over the same dose range. Physical mixtures of THS and 5FU were prepared in both a 1:1 (50 μg THS:18 μg 5FU) and a 1:2 (50 μg THS:36 μg 5FU) molar ratio, respectively. In the CAM assay the results from these physical mixtures of THS and 5FU indicated that the 1:2 molar ratio was more effective than the 1:1 molar ratio. In the physical mixtures it is likely that 5FU will have a higher bioavailability than THS when released from the implanted methylcellulose pellets, resulting in the more soluble 5FU rapidly leached out of the pellet, leaving behind the less water-soluble THS. For this reason the synthesis of a THS-BIS-5FU codrug (incorporating 1 molar equivalent of THS and 2 molar equivalents of 5FU) was pursued in order to develop a sustained release delivery system for THS and 5FU that would be suitable for long-term ocular implantation. This differential diffusion of 5FU and THS should not be a problem in THS-BIS-5FU codrug formulation, since upon dissolution, the codrug should rapidly hydrolyze to produce a sustained and concomitant release of THS and 5FU in quantitative ratios.
Examining the results from the kinetic studies, the half-life of disappearance (T1/2d) for O3α-, O21-di-(N1-methyloxycarbonyl-2, 4-dioxo-5-fluoropyrimidinyL)17α-hydroxy-5β-pregnan-20-one codrug THS-BIS-5FU follows first order kinetic principles and is substantially shorter in biological media than in phosphate buffer. This can be attributed to the presence of esterase activity in the biological matrices. The conversion of the codrug to 5FU and THS was not obtained in the expected molar ratios. It is possible that partial or incomplete hydrolysis of the codrug is occurring or that the THS-BIS-5FU codrug is being hydrolyzed into 5FU and some intermediate product, which is then subsequently further hydrolyzed, at a slower rate, into THS. However, the data clearly show that the codrug rapidly disappears with a concomitant appearance of 5FU, and the 5FU release is complete (i.e. two molar equivalents).
A number of possible products can be generated from the hydrolysis of the THS-BIS-5FU codrug as a result of cleavage of the 5FU moiety (11). First, cleavage of one of the 5FU components along with its corresponding linker, could generate either O3α-(N1-methyloxycarbonyl-2,4-dioxo-5-fluoropyrimidinyl)17α-hydroxy-5β-pregnan-20-one (9), O21-(N1-methyloxycarbonyl-2,4-dioxo-5-fluoropyrimidinyl)17α-hydroxy-5β-pregnan-20-one (10), or a mixture of the two. If this were to occur, the rate of appearance of 5FU might display biphasic kinetic characteristics, as the rate of hydrolysis at the 3α-position is believed to be much faster than the rate of hydrolysis at the 21-position. It also seems more likely that mixed hydrolysis kinetics would make it impossible to differentiate between the hydrolysis rates of the two different 5FU moieties in the codrug molecule. It is also possible in this case that evidence of formation of the 3α-codrug (9) or the 21-codrug (10) would be observed, however, this was not evident from the HPLC analyses. In addition to this, NMR spectrophotometric analyses suggested that the intermediate was a THS analogue, probably containing one or two carbonic acid moieties. A Second possibility is that an intra-molecular, cyclic intermediate could be formed in the steroid side chain ( and ). For the cyclization reactions to occur in these side chains, the presence of a good leaving group is essential. Given the known side-arm chemistry of THS, it is possible that a cyclic intermediate could be formed via the mechanism illustrated in [Citation28]. This reaction could also be initiated externally via buffer or enzymatic attack at the ester carbonyl carbon. Third, it is possible that 5FU is hydrolyzed from THS-BIS-5FU codrug at both the 3α- and the 21-positions, leaving a non-cyclic THS carbonate ester as the hydrolysis intermediate (). The THS carbonate ester may then directly lose CO2 to form THS, or may undergo intramolecular cyclization, and upon further degradation, lose CO2 to form THS.
Figure 8 Chemical structure of a proposed THS carbonate ester, the cyclic intermediate formed from the hydrolysis of the O3α-, O21-di-(N1-methyloxycarbonyl-2, 4-dioxo-5-fluoropyrimidinyl)17α- hydroxy-5β-pregnan-20-one codrug [THS-BIS-5FU] (11), and the degradation of the cyclic intermediate to form THS.
![Figure 8 Chemical structure of a proposed THS carbonate ester, the cyclic intermediate formed from the hydrolysis of the O3α-, O21-di-(N1-methyloxycarbonyl-2, 4-dioxo-5-fluoropyrimidinyl)17α- hydroxy-5β-pregnan-20-one codrug [THS-BIS-5FU] (11), and the degradation of the cyclic intermediate to form THS.](/cms/asset/78f7df48-a670-4e4c-92ab-65f9e745224f/ienz_a_122014_f0008_b.jpg)
Analysis of the 1H-NMR spectrum of the unknown intermediate demonstrates the presence of two compounds. One of these compounds is THS, which presumably results from the facile hydrolysis of the unknown intermediate during isolation, together with the unknown intermediate, which apparently is an analogue of THS, in that it is susceptible to hydrolytic degradation to THS. The NMR characteristics of the unknown compound are not similar to those in either of the mono codrug molecules. However, some similarity in splitting pattern is observed when a comparison of the 1H-NMR spectrum of the unknown compound with the 1H-NMR spectrum of the THS-BIS-5FU codrug is made. These data provide supporting evidence that the hydrolysis intermediate is probably a carbonate ester or carbonate ester analogue of THS ().
Figure 9 Proposed hydrolysis products, based upon 1H-NMR spectrum of the isolated hydrolysis intermediate O3α-, O21-di-(N1-methyloxycarbonyl-2, 4-dioxo-5-fluoropyrimidinyl)17α- hydroxy-5β-pregnan-20-one codrug [THS-BIS-5FU] (11).
![Figure 9 Proposed hydrolysis products, based upon 1H-NMR spectrum of the isolated hydrolysis intermediate O3α-, O21-di-(N1-methyloxycarbonyl-2, 4-dioxo-5-fluoropyrimidinyl)17α- hydroxy-5β-pregnan-20-one codrug [THS-BIS-5FU] (11).](/cms/asset/32c10d1d-6d2f-45d0-bc93-522e705af7c7/ienz_a_122014_f0009_b.jpg)
In conclusion, a novel THS-BIS-5FU codrug has been synthesized which exhibited good angiogenesis activity in the preliminary studies utilizing the CAM assay. This codrug was also shown to convert to the parent drug products THS and 5FU when evaluated in vitro in different media. These data suggest that such novel codrugs are worthy of further evaluation, and may be amenable to formulation in a sustained release dosage form.
Acknowledgements
The authors gratefully acknowledge support for this work by Control Delivery Systems, Inc. Watertown, Boston, MA. Thanks are also due to Drs. Paul Ashton, Grazyna Cynkowska, and Tadeusz Cynkowski, Control Delivery Systems, Inc., for helpful discussions and technical advice.
References
- Varner JA, Brooks PC, Cheresh DA. Cell Adh Comm 1995; 3(4)367–374
- Casey R, Li W. Am J Opth 1997; 124(4)521–529
- Pepper MS, Montesano R, Mandriota SJ, Orci L, Vassalli JD. Enz Prot 1996; 49: 138–162
- Shultz GS, Grant MB. Eye 1991; 5: 170–180
- Liotta LA, Steeg PS, Stetler-Stevenson WG. Cell 1991; 64(2)327–336
- Mullican JB, Young AE. Vascular Birthmarks: Hemangiomas and Malformation. P.A. Saunders;1988.
- White CW, Sondheimer HM, Crouch EC, Wilson H, Fan LL. N Eng J Med 1989; 320: 1197–1200
- Palweletz N, Knierim M. Crit Rev Oncol Hematol 1989; 9: 197–242
- Folkman J. Can Res 1986; 46: 467–473
- Morse P. Vitreoretinal Disease: Diseases Associated with the Complications of Subretinal and Choroidal Neovascularization. 1988; 10: 335–340
- Barinaga M. Res News 1995; 267: 452–453
- Crum R, Szabo S, Folkman J. Science 1985; 230: 1375–1378
- Blei F, Wilson EL, Mignatti P, Rifkin DB. J Cell Physiol 1993; 155: 568–578
- Zhou T, Lewis H, Foster RE, Schwendeman SP. J Con Rel 1998; 55: 281–295
- Del Nozal MJ, Bernal JL, Pampliega A, Pastor JC, Lopez MI. J Chrom 1992; 607: 183–189
- Swintosky JV, Caldwell HC, Chong CW, Irwin GM, Dittert LW. J Pharm Sci 1968; 57(5)752–756
- Dittert LW, Caldwell HC, Adams HJ, Irwin GM, Swintosky JV. J Pharm Sci 1968; 57(5)774–780
- Thorpe PE, Derbyshire EJ, Andrade SP, Press N, Knowles PP, King S, Watson GJ, Yang YC, Rao-Bette M. Can Res 1993; 53(13)3000–3007
- Danis RP, Bingaman DP, Yang Y, Ladd B. Ophth 1996; 103(12)2099–2104
- Smit A, Bakker J. Rec Trav Chim Pays-Bas 1966; 85(7)731–743
- Bouchard R, Engel ChR. Can J Chem 1968; 46(13)2201–2206
- Harnik M. Steroids 1964; 3: 359–379
- Ahmad S, Ozaki S, Nagase T, Iigo M, Tokuzen R, Hoshi A. Chem Pharm Bull 1987; 35(10)4137–4143
- Danenberg PV. Colorec Can 2002; 621–633
- Wettstein A, Hunzinker F. Helv Chim Acta 1940; 23: 764–768
- Vandenheuvel FA. J Chromatogr 1975; 103: 113–134
- El Masry AH, Braun VC, Neilson CJ, Pratt WB. J Med Chem 1977; 20(9)1134–1139
- Lewbart M. J Org Chem 1972; 37(24)3892–3898