Abstract
The inhibitory effects of diesel exhaust components and flavonoids on 20α-hydroxysteroid dehydrogenase (20α-HSD) activity were examined in cytosolic fractions from the liver, kidney and lung of male mice. 9,10-Phenanthrenequinone (9,10-PQ) and 1,2-naphthoquinone (1,2-NQ), which are contained in diesel exhaust particles (DEPs), potently inhibited 20α-HSD activity in liver cytosol. 9,10-PQ also inhibited the enzyme activity in lung cytosol. However, 20α-HSD activity in kidney cytosol was little inhibited by 9,10-PQ or 1,2-NQ. Flavonoids such as quercetin, fisetin and kaempferol exhibited high inhibitory potencies for 20α-HSD activity in liver cytosol, whereas these flavonoids were poor inhibitors for the enzyme activity in kidney cytosol. It is likely that several diesel exhaust components and flavonoids augment the signaling of progesterone in the liver cells, by potently inhibiting 20α-HSD activity in mouse liver cytosol. The possibility that there are distinct enzymes catalyzing 20α-HSD activity in the non-reproductive tissues of male mice is also discussed.
Introduction
20α-Hydroxysteroid dehydrogenase (20α-HSD) was originally purified from rat ovary and characterized Citation1-3. This enzyme belongs to the aldo-keto reductase (AKR) superfamily [Citation4] and catalyzes the reduction of progesterone to its inactive metabolite 20α-hydroxy-4-pregnen-3-one, as shown in . Progesterone is essential for maintaining pregnancy in mammals, and its metabolism to 20α-hydroxy-4-pregnen-3-one is associated with the termination of pregnancy. However, progesterone at high levels has adverse effects on the development of fetuses [Citation5]. 20α-HSD probably plays a role in regulating the amount of progesterone and in protecting against its toxic effect. Recently, the ratio of 5α-pregnanes produced by 5α-reductase to 4-pregnenes produced by 20α-HSD or 3α-HSD from progesterone has been demonstrated to provide a hormonal basis for breast cancer [Citation6,Citation7]. Thus, the inhibition of 20α-HSD by environmental pollutants and xenobiotic compounds can increase this ratio and may promote breast cancer by stimulating cell proliferation and detachment.
Exposure of diesel exhaust particles (DEPs) to experimental animals and humans has been reported to cause lung cancer, allergic inflammation, asthma, and cardiopulmonary diseases Citation8-10. DEPs contain a variety of quinones that are capable of catalyzing the generation of reactive oxygen species in biological systems [Citation11,Citation12]. Among quinones, 9,10-phenanthrenequinone (9,10-PQ) is known as a relatively abundant quinone in DEPs [Citation13,Citation14]. We have recently shown that 20α-HSD activity is observed in cytosolic fractions from the lung in addition to the liver and kidney of male mice [Citation15]. Since 9,10-PQ is a good substrate of 20α-HSD [Citation3], it may inhibit 20α-HSD activity as a substrate inhibitor.
Flavonoids are naturally occurring compounds that are widely found in plants. Much attention has been paid to their antioxidant activities that affect the formation of reactive oxygen species and lipid peroxidation [Citation16,Citation17]. However, there is also evidence that flavonoids exhibit adverse health effects Citation18-20. Our previous report [Citation15] has found that flavonoids such as fisetin and quercetin potently inhibit 20α-HSD activity in cytosolic fraction from the liver of male mice, suggesting that the inhibition by flavonoids may result in disordered progesterone signaling. Furthermore, in our preliminary experiments, the inhibitory potency of a typical flavonoid quercetin for 20α-HSD activity was found to be distinguished clearly between liver and kidney cytosols.
The purpose of the present study was to elucidate whether diesel exhaust components including 9,10-PQ inhibit 20α-HSD activity in cytosolic fractions from the liver, kidney and lung of male mice. Furthermore, the inhibitory potencies of flavonoids for 20α-HSD activity in kidney cytosol were compared with those for the enzyme activity in liver cytosol.
Materials and methods
Materials
Progesterone (4-pregnene-3,20-dione) and 20α-hydroxy-4-pregnen-3-one were purchased from Sigma (St. Louis, MO, USA). Diesel exhaust components were obtained from the following sources: 9,10-PQ (Sigma); 1,2-naphthoquinone (1,2-NQ), 1,4-naphthoquinone (1,4-NQ), 9,10-anthraquinone (9,10-AQ), phenanthrene, and pyrene (Aldrich, Milwaukee, WI); anthracene and β-naphthoflavone (β-NF) (Wako Pure Chemicals, Tokyo, Japan). Flavonoids were obtained from the following sources: morin, myricetin, genistein, taxifolin (racemate), kaempferol and daidzein (Sigma); naringenin, apigenin and ( − )-epicatechin (Aldrich); quercetin and luteolin (Wako Pure Chemicals); fisetin and quercitrin (Tokyo Kasei, Tokyo, Japan). Genistin, (+)-catechin and rutin were donated by Dr J. Kinjo (Faculty of Pharmaceutical Sciences, Fukuoka University, Fukuoka, Japan). Sulfobromophthalein (SBP) and succinic semialdehyde (SSA) were obtained from Sigma, and 2-carboxybenzaldehyde (2-CBA) was obtained from Wako Pure Chemicals. NADP, glucose-6-phosphate and glucose-6-phosphate dehydrogenase were from Oriental Yeast (Tokyo, Japan). All other chemicals were of reagent grade.
Animals
Male ddY mice at 8 weeks of age were purchased from Japan SLC (Shizuoka, Japan). The animals had free access to a diet of standard laboratory chow and water. All animal experiments were undertaken in compliance with the guideline principles and procedures of Kumamoto University for the care and use of laboratory animals.
Preparation of cytosolic fraction
The animals were slightly anesthetized and killed by decapitation. The liver, kidney and lung were quickly excised, and homogenized in a Potter-Elvehjem homogenizer with three volumes of 10 mM sodium-potassium phosphate buffer containing 1.15% KCl (pH 7.4). All subsequent procedures were performed at 3–5°C. The homogenates were centrifuged at 10,000 × g for 20 min and the resulting supernatants were centrifuged at 105,000 × g for 60 min to obtain the cytosolic fraction. The cytosolic fractions from the liver, kidney and lung of male mice were used as enzyme preparations.
Assay of 20α-HSD activity
The enzyme activity was conducted in an NADPH-generating system consisting of progesterone (0.1 mM), NADP (0.25 mM), glucose-6-phosphate (6.25 mM), glucose-6-phosphate dehydrogenase (0.25 units), MgCl2 (6.25 mM), enzyme preparation (cytosolic fraction: 2 mg as protein) and 100 mM sodium-potassium phosphate buffer (pH 7.4) in a final volume of 2.0 mL. In the case of determination of the optimal pH, 100 mM sodium-potassium phosphate buffers at 5.0–9.0 were used. The mixture was incubated at 37°C for 30 min under aerobic condition. The reaction was stopped by addition of 0.5 mL of 1.0 N HCl to the mixture. The reduction product (20α-hydroxy-4-pregnen-3-one) of progesterone was determined by HPLC according to a slightly modified method of Swinney et al [Citation21]. HPLC was carried out using a Shimadzu LC-10AD HPLC apparatus (Shimadzu, Kyoto, Japan) equipped with a Tosoh ODS-80Ts column (Tosoh, Tokyo, Japan) and a JASCO 875-UV monitor (240 nm) (JASCO, Tokyo, Japan). A mixture of water-acetonitrile-methanol-tetrahydrofuran (44:28:17:11, v/v) was used as a mobile phase at a flow rate of 0.6 mL/min. Protein concentration was estimated by the method of Lowry et al. [Citation22] with bovine serum albumin as standard.
Inhibition experiments
Inhibitors were dissolved in dimethyl sulfoxide (DMSO) or methanol, and then added to the reaction mixture. The final concentration of DMSO or methanol did not exceed 2% (v/v), and this concentration did not affect the enzyme reaction. The final concentration of inhibitors was 10 μM for diesel exhaust components, 20 or 50 μM for flavonoids and 100 μM for SBP, 2-CBA and SSA.
Results
Effects of diesel exhaust components on 20α-HSD activity
The inhibitory effects of diesel exhaust components at a concentration of 10 μM on 20α-HSD activity were examined in the cytosolic fractions from the liver, kidney and lung of male mice (). Among the diesel exhaust components tested, 9,10-PQ and 1,2-NQ were potent inhibitors for 20α-HSD activity in liver cytosol, although diesel exhaust components other than these two o-quinones had little ability to inhibit 20α-HSD activity in liver cytosol. Interestingly, 9,10-PQ also inhibited 20α-HSD activity in lung cytosol. On the other hand, the enzyme activity in kidney cytosol was little inhibited by diesel exhaust components including 9,10-PQ or 1,2-NQ.
Figure 2 Inhibitory effects of diesel exhaust components on 20α-HSD activity in cytosolic fractions from the liver, kidney and lung of male mice. Progesterone at a concentration of 100 μM was used as the substrate. The concentration of diesel exhaust components was 10 μM. Each bar represents the mean ± SD of three to seven experiments.
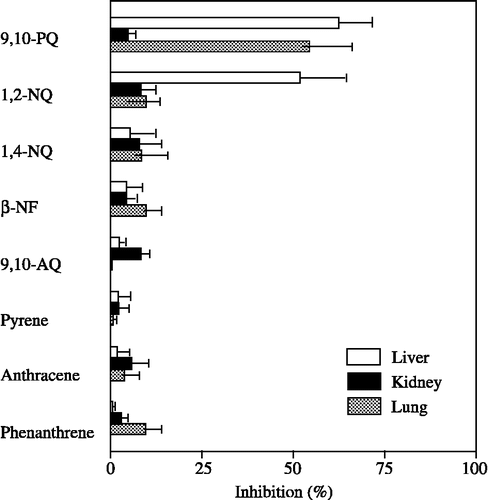
Effects of flavonoids on 20α-HSD activity
The inhibitory effects of flavonoids on 20α-HSD activity in the cytosolic fraction from the kidney of male mice were compared with those from the liver [Citation15]. As shown in , the inhibitory potencies of several flavonoids were much lower for 20α-HSD activity in kidney cytosol than that in liver cytosol. For example, although quercetin, fisetin and kaempferol at a concentration of 20 μM exhibited high inhibitory potencies for 20α-HSD activity in liver cytosol, these flavonoids, even at a concentration of 50 μM, were poor inhibitors of the enzyme activity in kidney cytosol. Furthermore, naringenin potently inhibited 20α-HSD activity in liver cytosol, whereas it was unable to inhibit 20α-HSD activity in kidney cytosol.
Figure 3 Inhibitory effects of flavonoids on 20α-HSD activity in cytosolic fractions from the liver and kidney of male mice. Progesterone at a concentration of 100 μM was used as the substrate. The concentrations of flavonoids were 20 and 50 μM. Each bar represents the mean ± SD of three experiments. Data in cytosolic fraction from the liver were cited from reference [Citation15].
![Figure 3 Inhibitory effects of flavonoids on 20α-HSD activity in cytosolic fractions from the liver and kidney of male mice. Progesterone at a concentration of 100 μM was used as the substrate. The concentrations of flavonoids were 20 and 50 μM. Each bar represents the mean ± SD of three experiments. Data in cytosolic fraction from the liver were cited from reference [Citation15].](/cms/asset/caa35fdb-3160-447e-bcca-411a871110a1/ienz_a_216140_f0003_b.gif)
Optimal pH of 20α-HSD activity
shows the pH-dependent profiles of 20α-HSD activity in mouse liver and kidney cytosols. The optimal pH of 20α-HSD activity in liver cytosol was pH 6.5–7.0. On the other hand, the optimal enzyme activity in kidney cytosol was observed at around pH 6.0.
Effects of specific inhibitors on 20α-HSD activity
The inhibitory effects of SBP, 2-CBA and SSA at a concentration of 100 μM on 20α-HSD activity were examined in the cytosolic fractions from the liver and kidney of male mice. SBP is a potent inhibitor of a mouse aldo-keto reductase (AKR) AKR1C20 [Citation23]. 2-CBA and SSA were used as substrate inhibitors since they are good substrates for AKR7A1 and AKR7A5 [Citation24,Citation25]. As shown in , SBP strongly inhibited 20α-HSD activity in kidney cytosol, whereas 2-CBA and SSA had little ability to inhibit the enzyme activity in kidney cytosol. These three inhibitors had little effect on 20α-HSD activity in liver cytosol.
Figure 5 Inhibitory effects of specific inhibitors on 20α-HSD activity in cytosolic fractions from the liver and kidney of male mice. Progesterone at a concentration of 100 μM was used as the substrate. The concentration of specific inhibitors (SBP, 2-CBA and SSA) was 100 μM. SBP is a potent inhibitor of AKR1C20. 2-CBA and SSA are substrate inhibitors for AKR7A1 and AKR7A5. Each bar represents the mean ± SD of three experiments.
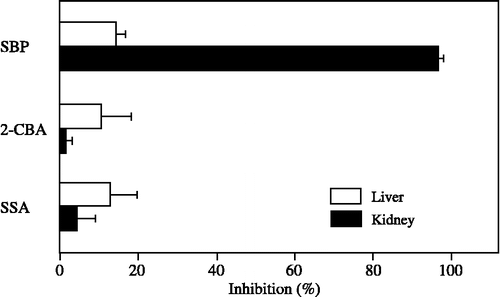
Discussion
It has been reported that 9,10-PQ and 1,2-NQ serve as inhibitors of enzymes such as nitric oxide synthase [Citation26], carbonyl reductase [Citation27,Citation28] and glyceraldehyde-3-phosphate dehydrogenase [Citation29]. In the present study, 9,10-PQ was demonstrated to be a potent inhibitor for 20α-HSD activity in the cytosolic fractions from the liver and lung of male mice. It should be noted that 9,10-PQ inhibits 20α-HSD activity in lung cytosol. However, 20α-HSD activity in kidney cytosol, unlike those in liver and lung cytosols, was little inhibited by 9,10-PQ. Although 1,2-NQ, one of the o-quinones, also inhibited 20α-HSD activity in liver cytosol, it had little ability to inhibit 20α-HSD activity in kidney and lung cytosols. 20α-HSD catalyzes the reduction of progesterone to its inactive metabolite 20α-hydroxy-4-pregnen-3-one and is involved in regulating the amount of progesterone that binds to its nuclear receptor. Therefore, 9,10-PQ and 1,2-NQ contained in DEPs probably augment the signaling of progesterone in liver cells, by potently inhibiting 20α-HSD activity. 9,10-PQ is produced from phenanthrene, which comprises 6% of the total organic extract of DEPs, by photooxidation in the atmosphere [Citation30]. However, phenanthrene was found to have little ability to inhibit 20α-HSD activity in cytosolic fractions of mouse liver, kidney and lung.
Very recently, evidence has been provided that flavonoids such as fisetin, quercetin and naringenin potently inhibit 20α-HSD activity in the cytosolic fraction from the liver of male mice [Citation15]. Thus, it is reasonable to assume that these flavonoids, like 9,10-PQ and 1,2-NQ, augment progesterone signaling by potently inhibiting hepatic 20α-HSD activity. In this study, we further attempted to evaluate the inhibitory effects of flavonoids on 20α-HSD activity in the cytosolic fraction of mouse kidney. However, the inhibitory potencies of flavonoids for the enzyme activity were much lower in kidney cytosol than in liver cytosol.
On the basis of the tissue-dependent inhibitory potencies of o-quinones and flavonoids for 20α-HSD activity as described above, we propose the possibility that there are several distinct enzymes catalyzing 20α-HSD activity in the tissues of mice. In addition, the optimal pH of 20α-HSD activity in mouse liver cytosol was found to be distinguished from that in mouse kidney cytosol. This finding also supports the idea that in cytosolic fractions of mouse liver and kidney, different enzymes catalyze 20α-HSD activity.
In mice, 17β-HSD type5 (AKR1C6) has been demonstrated to exhibit low 20α-HSD activity [Citation31,Citation32]. Thus, it is possible that enzymes belonging to the AKR subfamily other than mouse 20α-HSD (AKR1C18) play a role in the reduction of progesterone to 20α-hydroxy-4-pregnen-3-one (20α-HSD activity) in cytosolic fractions of mouse liver and kidney. AKR1C20 exhibits the highest sequence identity with AKR1C6, and its mRNA is specifically expressed in the liver [Citation23]. This enzyme also reduces S-camphorquinone, showing a broad pH optimum from 6.5 to 7.5, similarly to the pH-dependent profile of 20α-HSD activity in mouse liver cytosol. Thus, AKR1C20 may be an enzyme responsible for 20α-HSD activity in mouse liver cytosol. However, SBP, a potent inhibitor of AKR1C20 [Citation23], had little effect on 20α-HSD activity in mouse liver cytosol. Unexpectedly, SBP strongly inhibited 20α-HSD activity in mouse kidney cytosol, even though AKR1C20 mRNA is not expressed in the kidney [Citation33]. Furthermore, SSA and 2-CBA, substrate inhibitors of AKR7A5 [Citation25], had little effect on enzyme activity in mouse liver or kidney cytosol. Additional studies are in progress to identify the AKR enzymes that catalyze 20α-HSD activity in cytosolic fractions from the non-reproductive tissues of male mice.
References
- Wiest WG, Wilcox RB. Purification and properties of rat ovarian 20α-hydroxysteroid dehydrogenase. J Biol Chem 1961; 236: 2425–2428
- Wilcox RB, Wiest WG. Further studies of rat ovarian 20α-hydroxysteroid dehydrogenase. Steroids 1966; 7: 395–413
- Ma H, Penning TM. Characterization of homogeneous recombinant rat ovarian 20α-hydroxysteroid dehydrogenase: fluorescent properties and inhibition profile. Biochem J 1999; 341: 853–859
- Penning TM. Molecular endocrinology of hydroxysteroid dehydrogenases. Endocr Rev 1997; 18: 281–305
- Petrelli EA, Forbes TR. Toxicity of progesterone to mouse fetuses. Endocrinol 1964; 75: 145–146
- Ji Q, Aoyama C, Nien Y-D, Liu PI, Chen PK, Chang L, Stanczyk FZ, Stolz A. Selective loss of AKR1C1 and AKR1C2 in breast cancer and their potential effect on progesterone signaling. Cancer Res 2004; 64: 7610–7617
- Wiebe JP, Lewis MJ, Cialacu V, Pawlak KJ, Zhang G. The role of progesterone metabolites in breast cancer: Potential for new diagnostics and therapeutics. J Steroid Biochem Mol Biol 2005; 93: 201–208
- McClellan RO. Health effects of exposure to diesel exhaust particles. Annu Rev Pharmacol Toxicol 1987; 27: 279–300
- Dockery DW, Pope ACIII, Xu X, Spengler JD, Ware JH, Fay ME, Ferris BG, Jr, Speizer FE. An association between air pollution and mortality in six U.S. cities. New Engl J Med 1993; 329: 1753–1759
- Nel AE, Diaz-Sanchez D, Li N. The role of particulate pollutants in pulmonary inflammation and asthma: evidence for the involvement of organic chemicals and oxidative stress. Curr Opin Pulm Med 2001; 7: 20–26
- Monks TJ, Hanzlik RP, Cohen GM, Ross D, Graham DG. Quinone chemistry and toxicology. Toxicol Appl Pharmacol 1992; 112: 2–16
- Bolton JL, Trush MA, Penning TM. Role of quinones in toxicology. Chem Res Toxicol 2000; 13: 135–160
- Schuetzle D. Sampling of vehicle emmissions for chemical analysis and biological testing. Environ Health Perspect 1983; 47: 65–80
- Cho AK, Di Stefano E, You Y, Rodriguez CE, Schmitz D, Kumagai Y, Miguel AH, Eiguren-Fernandez AA, Kobayashi T, Vol A, Froines JR. Determination of four quinones in diesel exhaust particles, SRM 1649a, and atmospheric PM2.5. Aerosol Sci Technol 2004; 38: 68–81
- Shimada H, Miura K, Imamura Y. Characteristics and inhibition by flavonoids of 20α-hydroxysteroid dehydrogenase activity in mouse tissues. Life Sci 2006; 78: 2931–2936
- Rice-Evans CA, Miller NJ, Paganga G. Structure-antioxidant activity relationships of flavonoids and phenolic acids. Free Radic Biol Med 1996; 20: 933–956
- Di Carlo G, Mascolo N, Izzo AA, Capasso F. Flavonoids: old and new aspects of a class of natural therapeutic drug. Life Sci 1999; 65: 337–353
- Stopper H, Schmitt E, Kobras K. Genotoxicity of phytoestrogens. Mutat Res 2005; 574: 139–155
- van der Woude H, Alink GM, van Rossum BEJ, Walle K, van Steeg H, Walle T, Rietjens IMCM. Formation of transient covalent protein and DNA adducts by quercetin in cells with and wothout oxidative enzyme activity. Chem Res Toxicol 2005; 18: 1907–1916
- Morris ME, Zhang S. Flavonoid-drug interactions: Effects of flavonoids on ABC transporters. Life Sci 2006; 78: 2116–2130
- Swinney DC, Ryan DE, Thomas PE, Levin W. Regioselective progesterone hydroxylation catalyzed by elever rat hepatic cytochrome P-450 isozymes. Biochemistry 1987; 26: 7073–7083
- Lowry OH, Rosebrough NJ, Farr AL, Randall RJ. Protein measurement with the folin phenol reagent. J Biol Chem 1951; 193: 265–275
- Matsumoto K, Endo S, Ishikura S, Matsunaga T, Tajima K, El-Kabbani O, Hara A. Enzymatic properties of a member (AKR1C20) of the aldo-keto reductase family. Biol Pharm Bull 2006; 29: 539–542
- Ellis EM, Hayes JD. Substrate specificity of an aflatoxin-metabolizing aldehyde reductase. Biochem J 1995; 312: 535–541
- Hinshelwood A, McGarvie G, Ellis EM. Substrate specificity of mouse aldo-keto reductase AKR7A5. Chem Biol Interact 2003; 143–144: 263–269
- Kumagai Y, Nakajima H, Midorikawa K, Homma-Takeda S, Shimojo N. Inhibition of nitric oxide formation by nitric oxide synthase by quinones: Nitric oxide synthase as a quinone reductase. Chem Res Toxicol 1998; 11: 608–613
- Shimada H, Oginuma M, Hara A, Imamura Y. 9, 10-Phenanthrenequinone, a component of diesel exhaust particles, inhibits the reduction of 4-benzoylpyridine and all-trans-retinal and mediates superoxide formation through its redox cycling in pig heart. Chem Res Toxicol 2004; 17: 1145–1150
- Oginuma M, Shimada H, Imamura Y. Involvement of carbonyl reductase in superoxide formation through redox cycling of adrenochrome and 9,10-phenanthrenequinone in pig heart. Chem Biol Interact 2005; 155: 148–154
- Rodriguez CE, Fukuto JM, Taguchi K, Froines J, Cho AK. The interactions of 9,10-phenanthrenequinone with glyceraldehyde-3-phosphate dehydrogenase (GAPDH), a potential site for toxic actions. Chem Biol Interact 2005; 155: 97–110
- Moza PN, Hustert K, Kettrup A. Photooxidation of naphthalene and phenanthrene in hexane as an oil film on water. Chemosphere 1999; 39: 569–574
- Deyashiki Y, Ohshima K, Nakanishi M, Sato K, Matsuura K, Hara A. Molecular cloning and characterization of mouse estradiol 17β-dehydrogenase (A-specific), a member of the aldoketoreductase family. J Biol Chem 1995; 270: 10461–10467
- Matsunaga T, Shintani S, Hara A. Multiplicity of mammalian reductases for xenobiotic carbonyl compounds. Drug Metab Pharmacokinet 2006; 21: 1–18
- Vergnes L, Phan J, Stolz A, Reue K. A cluster of eight hydroxysteroid dehydrogenase genes belonging to the aldo-keto reductase supergene family on mouse chromosome 13. J Lipid Res 2003; 44: 503–511