Abstract
Complexes of the type [M(bssdh)]Cl and [M(dspdh)]Cl, where M = Co(II), Ni(II), Cu(II), Zn(II) and Cd(II); Hbssdh = benzil salicylaldehyde succinic acid dihydrazone, Hdspdh = diacetyl salicylaldehyde phthalic acid dihydrazone have been synthesized and characterized with the help of elemental analyses, electrical conductance, magnetic susceptibility measurements, electronic, ESR and IR spectra and X–ray diffraction studies. Magnetic moment values and electronic spectral transitions indicate a spin free octahedral structure for Co(II), Ni(II) and Cu(II) complexes. IR spectral studies suggest that both the ligands behave as monobasic hexadentate ligands coordinating through three > C = O, two > C = N– and a phenolate group to the metal. ESR spectra of Cu(II) complexes are axial type and suggest as the ground state. X–ray powder diffraction parameters for [Co(bssdh)]Cl and [Co(dspdh)]Cl complexes correspond to an orthorhombic crystal lattice. The ligands as well as their metal complexes show a significant antifungal and antibacterial activity against various fungi and bacteria. The metal complexes are more active than the parent ligands.
Introduction
A considerable interest is being shown in the phenomenon of metal chelation in biological systems. The role of several drugs in relation to their metal binding has been established. The discovery of antitumour activity of certain platinum coordination compounds [Citation1] opened up a new class of antitumour agents, namely inorganic coordination complexes. It is accepted that the antitumour activity is due to inhibition of DNA synthesis in the cancer cells [Citation2]. The antitumour activity of platinum(II) complexes with nucleosides and their bases have been investigated extensively [Citation3]. Several bivalent [Citation4–11] transition metal complexes of uracil, substituted uracils and its mixed ligand complexes have been reported to show appreciable antitumour activity.
Since acylhydrazones are strong biologically active compounds [Citation12–14], their complexes may have potential use as fungicides and bactericides. Expecting some new compounds of biological interest, we have synthesized and characterized a number of bivalent transition metal complexes with benzil salicylaldehyde succinic acid dihydrazone (Hbssdh) and diacetyl salicylaldehyde phthalic acid dihydrazone (Hdspdh) and studied their antifungal and antibacterial properties.
Experimental
Materials
All the chemicals were of BDH (AnalaR) or equivalent grade. Succinic acid dihydrazide (sdh) and phthalic acid dihydrazide (pdh) were prepared by reacting hydrazine hydrate with the corresponding diethyl esters in 2:1 molar ratio [Citation15].
Synthesis of the ligands
For the synthesis of the ligand, benzil salicylaldehyde succinic acid dihydrazone (Hbssdh), C6H5COC(C6H5) = NNHCO(CH2)2CONHN = CHC6H4(OH), the solutions of benzil (10 mmol, 2.10 g) and salicylaldehyde (10 mmol, 1.22 mL) in 50 mL ethanol were mixed in a RB flask by stirring. To the above solution mixture, 50 mL of aqueous solution of succinic acid dihydrazide (10 mmol, 1.46 g) was added and stirred continuously for 2–3 h on a magnetic stirrer at room temperature. The product was filtered by suction and washed thoroughly with water and then with ethanol to ensure purity of the ligand. The pure ligand was dried in a desicator over anhydrous CaCl2.
Diacetyl salicylaldehyde phthalic acid dihydrazone (Hdspdh),CH3COC(CH3) = NNHCOC6H4CONHN = CHC6H4(OH) was prepared by reacting 50 mL aqueous ethanol solution (v/v, 1:1) of phthalic acid dihydrazide (10 mmol, 1.94 g) with a mixed solution of diacetyl (10 mmol, 0.86 mL) and salicylaldehyde (10 mmol, 1.22 mL) in 50 mL ethanol. The reacting solution mixture was stirred for ∼3 h at room temperature. The product was filtered, washed several times with water and aqueous ethanol (v/v, 1:1) and dried at room temperature in a desicator.
The ligands were characterized by their melting points, elemental analyses (), IR spectra (), 1H and 13C NMR spectra. Hbssdh, 1H NMR (ppm): Two >CH2 (2.5), two >NH (7.0 and 8.3), aromatic ring protons (7.4–8.1), = CH (9.0), –OH (11.3). Hbssdh, 13C NMR (ppm): Two >CH2 (28.316), aromatic ring carbons (116.131–146.476), two >C = N (156.375, 157.306), two >C = O (167.723 and 172.973). Hdspdh, 1H NMR (ppm): Two –CH3 (1.2 and 2.3), two >NH (6.8 and 7.1), aromatic ring protons (7.3–8.1), = CH (9.0), –OH (11.5). Hdspdh, 13C NMR (ppm): Two –CH3 (9.146 and 39.78), aromatic ring carbons (116.576–133.282), = CH (154.718), two >C = N (158.682), two >C = O (162.861 and 185.016).
Table I. Analytical data of the ligands and their complexes.
Synthesis of the metal complexes
The metal complexes were synthesized by reacting 50 mL aqueous solution of each metal(II) chloride (10 mmol) with 50 mL suspended ethanol solution of each ligands, Hbssdh (10 mmol, 4.42 g) and Hdspdh (10 mmol, 3.66 g) in 1:1 (M:L) molar ratio. Cu(II), Zn(II) and Cd(II) complexes were formed by stirring the reaction mixture for 2–4 h on a magnetic stirrer at room temperature. However, Co(II) and Ni(II) complexes were obtained by refluxing the solution mixture for 4 h and 6 h, respectively. The resulting complexes were filtered in a glass crucible and washed several times with water and ethanol to remove unreacted metal chloride and ligand. The pure complexes were dried in a desicator over anhydrous calcium chloride at room temperature.
Analyses and instrumentation
Metal contents were determined gravimetrically by a literature procedure [Citation16] after digesting the organic matter with aquaregia and evaporating the residue to dryness with conc. sulfuric acid. The chloride content was analysed gravimetrically as silver chloride. C, H, N data were determined on an Elementar Vario EL model elemental analyzer.
The molar conductance of 10− 3 M solutions of the complexes in DMF were measured at room temperature on a Systronic Conductivity meter model-306. Room temperature magnetic susceptibilities of the complexes were determined on a Faraday balance using Hg[Co(SCN)4] as calibrant and corrected for diamagnetism [Citation17]. IR spectra of the ligands and their metal complexes were recorded in KBr medium in the 4000–500 cm− 1 range on a Vertex 70 (Bruker) Spectrophotometer and electronic spectra were recorded in DMF on a Cintra 10 Spectrophotometer. 1H and 13C NMR spectra of the ligands were recorded on a JEOL AL 300 FT NMR Spectrophotometer in DMSO-d6 at 25°C. The X–band ESR spectra of Cu(II) complexes were recorded on a EMX 1444 EPR Spectrometer at liquid nitrogen temperature (LNT) in DMSO solution and at room temperature (300 K) in solid state. Powder X–ray diffraction patterns were recorded on Iso Debye Flex 2002 apparatus using CuKα radiations.
The analytical and physico-chemical data are given in Tables .
Biocidal activity
Antifungal activity
The ligands as well as their complexes were screened for antifungal activity against several fungi viz. Curvularia, Fusarium and Colletotrichum species. These species were isolated from the infected organs of the host plants on potato dextrose agar (potato 250 g + dextrose 20 g + agar 20 g) medium. The cultures of the fungi were purified by single spore isolation technique.
The solutions in different concentrations 0.5, 1.0 and 1.5 mg/mL of each compound in DMSO were prepared for testing against spore germination. A drop of the solution of each concentration was kept separately on glass slides. The conidia, fungal reproducing spores (approx. 200) lifted with the help of an inoculating needle, were mixed in every drop of each compound separately. Each treatment was replicated thrice and a parallel DMSO solvent control set was run concurrently on separate glass slides. All the slides were incubated in humid chambers at 25 ± 2°C for 24 h. Each slide was observed under the microscope for spore germination and percent germination was finally calculated. The results were also compared with a standard antifungal drug miconazole at the same concentrations.
Antibacterial activity
The antibacterial activity of the ligands and their complexes was studied against Pseudomonas (gram –ve) and Bacillus sp. (gram +ve) bacteria. Each of the compounds was dissolved in DMSO and solutions of the concentration 1.0 and 2.0 mg/mL were prepared separately. Paper discs of whatman filter paper (No. 42) of uniform diameter were cut and sterilized on an autoclave. The paper disc soaked in the desired concentration of the complex solution was placed aseptically in the petridishes containing nutrient agar media (agar 15 g+ beef extract 3 g+ peptone 5 g) seeded with Bacillus and Pseudomonas sp. bacteria separately. The Petridishes were incubated at 32° C and the inhibition zones were recorded after 24 h of incubation. Each treatment was replicated 9 times.
A common standard antibiotic Ampicillin was also screened for the antibacterial activity in the same solvent and at the same concentration. The percent Activity Index data for the metal complexes were calculated as follows:
Determination of minimum inhibitory concentration (MIC) value
The compounds showing antibacterial activity over 50 % were selected for the determination of minimum inhibition concentration (MIC). The MIC of the selected compounds against selected bacteria was estimated using the disc diffusion technique by preparing discs containing 0.1, 0.25, 0.5 and 1.0 mg/mL of the compounds and applying the protocol. The results of MIC values (mg/mL) are summarized below:
Results and discussion
It appears from the analytical data () that both the ligands undergo deprotonation of their phenolic proton during the complex formation with metal(II) chloride. The ligands Hbssdh and Hdspdh react with metal salts in 1:1 (M:L) molar ratio to give complexes of the general compositions [M(bssdh)]Cl and [M(dspdh)]Cl. The reactions may have proceeded as follows:
The complexes are insoluble in water, methanol,a ethanol, benzene, chloroform, carbon tetrachloride, diethyl ether and ethyl acetate but are fairly soluble in polar organic solvents such as DMF and DMSO. They are orange, green, green-yellow to light yellow in colour. Some of the complexes either melt or decompose above 300 °C while others between 250–300° C. The molar conductance values of 10− 3 M solution of the complexes measured at room temperature fall in the range 65.8–79.2 Ω− 1mol− 1cm2 indicating that they are 1:1 electrolytes [Citation18].
Magnetic moments
Cobalt(II) tetrahedral complexes generally show magnetic moments between 4.0–4.6 B.M. while the octahedral complexes show between 4.7–5.2 B.M. because of the orbital contribution [Citation19]. The μeff values 4.82 B.M. and 4.96 B.M. observed for Co(II) complexes with Hbssdh and Hdspdh, respectively, are fairly close to those reported for three unpaired electrons in an octahedral environment. The effective magnetic moments reported for high spin octahedral Ni(II) complexes are in the range 2.9–3.4 B.M., while for the tetrahedral nickel(II) complexes, the value ranges from 3.5–4.0 B.M. [Citation17]. Both Ni(II) complexes in this study, show μeff values 3.20 B.M. and 3.16 B.M. corresponding to two unpaired electrons in octahedral environment. The magnetic moments of two Cu(II) complexes (1.93 and 1.87 B.M.) correspond to μeff values for one unpaired electron.
Electronic spectra
Cobalt(II) complexes generally show three absorption bands in the visible range under the influence of the octahedral field by the excitation of the electron from ground state 4T1g (F) to the excited states 4T2g (F), 4A2g (F) and 4 T1g (P). In case of [Co(H2O)6]2 + , three transitions are observed at 8130, 17540 and 21980 cm− 1 [Citation20]. [Co(bssdh)]Cl and [Co(dspdh)]Cl complexes show only two bands 9025 and 9060 cm− 1 (ν1) and 18250 and 18350 cm− 1 (ν3), respectively suggesting octahedral geometry for the complexes. The ν2 transition was not observed due to very weak intensity [Citation20]. Various ligand–field parameters (10 Dq, B, β, β0 and LFSE) were also calculated for both Co(II) complexes () which indicate significant covalent bonding in metal–ligand bonds.
Table II. Magnetic moments, electronic spectral data and ligand field parameters of the complexes.
Nickel(II) complexes give rise to three bands in octahedral environment corresponding to transitions 3A2g (F) → 3T2g (F) (ν1), → 3T1g (F) (ν2) and → 3T1g (P) (ν3) [Citation21]. In the spectra of [Ni(NH3)6]2 + , these bands have been reported at 10700, 17540 and 28170 cm− 1, respectively [Citation17]. Both the Ni(II) complexes also show above three transitions at 10255 and 10460 cm− 1 (ν1), 18025 and 16015 cm− 1 (ν2), 25305 and 26250 cm− 1 (ν3) for [Ni(bssdh)]Cl and [Ni(dspdh)]Cl, respectively suggesting an octahedral geometry for the complexes. The ligand–field parameters have also been calculated by the procedure given by Lever [Citation20].
Both Cu(II) complexes show an intense broad band centered at 15310 cm− 1 and 14880 cm− 1 which may be due to envelope of the transitions 2B1 → 2B2g and → 2Eg suggesting a distorted octahedral geometry for the complexes [Citation22].
ESR spectra
ESR spectra of [Cu(bssdh)]Cl complex in solid state at 300 K exhibit isotropic spectra with a broad signal having no hyperfine structure. This may be due to dipolar exchange and unresolved hyperfine interactions [Citation23]. The giso = 2.1357 suggests a geometry involving grossly misaligned tetragonal axes. [Cu(dspdh)]Cl shows an axial signal with two g values at 300 K (). The g‖ and g⊥ values are >2.04 () indicating an elongated tetragonally distorted octahedral stereochemistry for the complex with all the principal axes aligned parallel.
Figure 2. ESR spectra of copper (II) complexes (a) in solid state at 300 K (b) In DMSO solution at 120 K.
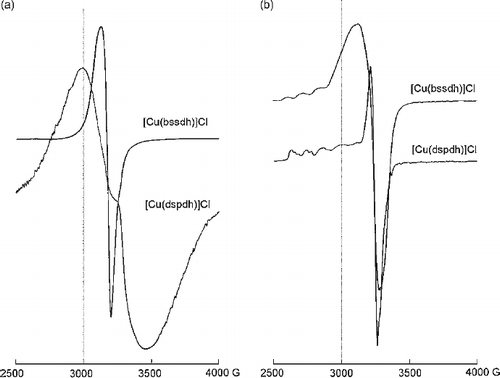
Table III. ESR and bonding parameters of the Cu(II) complexes.
DMSO solution spectra at LNT give axial spectra with two anisotropic g values for both Cu(II) complexes. The g‖, g⊥ , A‖ and A⊥ values were accurately measured from the spectra. The trend g‖ > g⊥ > ge (2.0023) shows the presence of unpaired electron in orbital of the copper(II) ion. Both the complexes show four well defined copper hyperfine lines due to coupling of the electron with nuclear spin (I = 3/2) of the copper atom. The gav and Aav values were calculated using the equations;
.
A significant degree of covalent character in the metal–ligand bonds is indicated by the values of the in-plane σ–bonding parameter α2 (0.89, 0.87), out of plane σ–bonding parameter α+2 (0.13, 0.15) and in-plane π–bonding parameter β12 (0.86, 0.71), respectively [Citation24].
IR spectra
The ligands Hbssdh and Hdspdh show ν(NH) at 3207 cm− 1 and 3162 cm− 1 respectively due to presence of two >NH groups in each ligand. In all the metal complexes ν(NH) occurs nearly at the same or slightly altered positions as in the ligands () indicating non-involvement of >NH groups in bonding. Both the ligands also show a broad band due to phenolic OH group at 3294 cm− 1 and 3392 cm− 1 respectively in Hbssdh and Hdspdh. ν(OH) is not observed in metal complexes indicating deprotonation of phenolic proton during complexation. Appearance of a new band assigned as ν(C–O) in all complexes in the region 1263–1296 cm− 1 confirms deprotonation of the ligands.
Table IV. IR spectral data (cm−1) and assignment of important bands.
ν(C = O) in the ligands Hbssdh and Hdspdh appears as a broad band at 1672 cm− 1 and 1686 cm− 1, respectively due to presence of three >C = O groups in each ligand. ν(C = O) shifts to lower frequency by 21–30 cm− 1 in the complexes as compare to the parent ligands suggesting coordination of all the three >C = O groups to the metal [Citation25]. The amide II bands appear to have shifted to lower frequency by 10–23 cm− 1 upon complex formation. Compared to the ligand band, a shift to higher frequency (9–19 cm− 1) is observed in amide III bands in all the metal complexes supporting coordination through the >C = O groups [Citation26].
The ν(C = N) bands observed at 1619 cm− 1 in Hbssdh and at 1635 cm− 1 in Hdspdh ligands shifted considerably to lower frequency by 10–20 cm− 1 in the metal complexes suggesting coordination through two azomethine groups [Citation27]. The shift to higher wave numbers in ν(N–N) from the free ligands to their complexes by 20–40 cm− 1, suggests involvement of only one nitrogen of the hydrazone (–NHN = C < ) group [Citation28].
On the basis of above discussion, general structures for the metal complexes have been proposed in .
X–ray diffraction studies
X–ray powder method provides a way of investigating within limits, the crystallography of a crystal in powder form. All the complexes in present study are stable microcrystalline powders. The diffraction patterns for [Co(bssdh)]Cl and [Co(dspdh)]Cl were recorded and successfully indexed by using Ito's method [Citation29] and the lattice constants calculated () as follows:
These constants indicate an orthorhombic crystal lattice for both complexes.
Table V. Observed and calculated Q and hkl values.
Antifungal activity
The antifungal experimental results of the compounds were compared against DMSO as the control and are expressed as percentage inhibition versus control (). The experimental antifungal activity data show the following observations:
Table VIA. Antifungal activity of the ligands and their complexes.
The complexes show a fair degree of activity against Curvularia sp., Fusarium sp. and Colletotrichum sp. at 0.5, 1.0 and 1.5 mg/mL concentration [Citation30].
The metal complexes are appreciably more active than the ligands. Their activity is enhanced at higher concentration.
DMSO control showed negligible activity as compare to the metal complexes and ligands.
Hbssdh and its complexes are more effective than Hdspdh and its complexes.
All the metal complexes are more active against Fusarium sp. in comparison to other fungal species.
Cd(II) complexes with both ligands show 100% activity against all three fungal species at the concentration of 1.5 mg/mL. [Cu(bssdh)]Cl complex also shows 100% activity against Fusarium sp. and Colletotrichum sp. at the dose of 1.5 mg/mL.
The antifungal activity of Hbssdh complexes varies in the following order of the fungal species:
For Hdspdh complexes antifungal activity varies as:
The activity varies in the following order of metal ions:
Cu(II), Zn(II) and Cd(II) complexes exhibited slightly better activity as compare to the standard drug miconazole against Curvularia and Colletotrichum sp.
Antibacterial activity
The metal complexes, ligands, standard drug Ampicillin and DMSO solvent control were screened separately for their antibacterial activity against Psuedomonas and Bacillus sp… The activity of the complexes has been compared with the activity of a common standard antibiotic ampicillin and % Activity Index has been calculated for the complexes. The antibacterial activity data () indicate the following observations:
Table VIB. Antibacterial activity of the ligands and their complexes.
All the complexes show a significant antifungal activity against both the bacteria [Citation31,32] at the concentration of 1.0 and 2.0 mg/mL as compared to the standard drug (ampicillin).
The activity generally increases with increasing the concentration of the complexes.
The metal complexes are more effective than the ligands.
The DMSO control showed no activity against any bacterial strain.
The % Activity Index data indicate that [Cu(bssdh)]Cl shows the highest activity (77%) against Bacillus sp. at the dose of 2.0 mg/mL.
Copper(II) complexes are highly effective against both the bacteria.
Acknowledgements
The authors thank the Director, Institute of Engineering & Technology, C.S.J.M. University, Kanpur for providing laboratory facilities, Head, Department of Chemistry, Indian Institute of Technology, Kanpur for recording UV–Vis, IR, and ESR spectra, Dr. Nand Lal, Department of Life Sciences, C.S.J.M. University, Kanpur for the help in biological screening.
References
- RJ Rutman, A Cantarow, and KE Paschkis. (1954). Studies in 2-acetylamino fluorine carcinogenesis. . Liver's Cancer Research 14:111–114.
- W Jiazhu, H Jingshuo, H Liyiao, S Dashuang, and H Shangzhi. (1988). Antitumor activity of organotin compounds. Reaction, synthesis and structure of Et2SnCl2(phen) with 5-fluorouracil. Inorg Chim Acta 152:67.
- W Saenger. Principles of nucleic acid structure. Berlin: Springer-Verlag; (1983).
- M Gupta, and MN Srivastava. (1985). Synthesis and characterization of mixed ligand complexes of copper(II), nickel(II), cobalt(II) and zinc(II) with glycine and uracil or 2-thiouracil. Polyhedron 4:475.
- UP Singh, R Ghose, and AK Ghose. (1988). Transition metal complexes of 5-chlorouracil. Transition Met Chem 13:50.
- R Ghose. (1989). Complex formation of adenine-uracil base pair with some transition metal ions. Inorg Chim Acta 156:303.
- P Ghose, T Mukhopadhya, and A Sarkar. (1984). Interaction of divalent metal ions with uracil III. Complexes of MnII, FeII, CoII, NiII and CuII with uracil acting as bidentate ligand. Transition Met Chem 9:46.
- KK Narang, VP Singh, and D Bhattacharya. (1997). 5-Fluorouracil and 5-fluorouracil-histidine complexes with AlIII, CrIII and FeIII ions and their antitumour activity. Polyhedron 16:2491.
- KK Narang, VP Singh, and D Bhattacharya. (1997). Synthesis, characterization and antitumour activity of uracil and uracil-histidine complexes with metal(III) ions. Transition Met Chem 22:333.
- VP Singh, A Singh, KK Narang, and D Bhattacharya. (2004). 5-Bromouracil and 5-bromouracil-histidine complexes with metal(III) ions and their antitumour activity. Transition Met Chem 29:107.
- KK Narang, VP Singh, and D Bhattacharya. (1998). Synthesis, characterization and antitumour activity of 5-chlorouracil and 5- chlorouracil-histidine complexes with some metal(III) ions. Synth React Inorg Met-Org Chem 28:37.
- L Pickart, WH Goodwin, TB Murphy, and DK Johnson. (1982). Growth-inhibitory analog of the GLY-HIS-LYS-Cu(II) complex. J Cell Biochem Suppl 6:172.
- M Mohan, NS Gupta, MP Gupta, A Kumar, and NK Jha. (1988). Synthesis, characterization and antitumor activity of some metal complexes of 3- and 5-substituted salicylaldehyde o-hydroxybenzoylhydrazones. J Inorg Chim Acta 152:25.
- A Maiti, and S Ghosh. (1989). Synthesis and reactivity of the oxovanadium(IV) complexes of two N–O donors and potentiation of the antituberculosis activity of one of them on chelation to metal ions. J Inorg Biochem 36:131.
- KS Patel, and AA Adeniyi. (1991). Dicarboxylic acid hydrazide complexes of nickel(II) tetrafluoroborate. Synth React Inorg Met-Org Chem 21:1331.
- AI Vogel. Vogel's text book of quantitative chemical analysis. 5th ed. Amsterdam: Longman; (1989).
- RL Dutta, and A Syamal. Elements of magnetochemistry2nd ed. New Delhi: Affiliated East-West Press Pvt. Ltd.; (1993).
- WJ Geary. (1971). The use of conductivity measurements in organic solvents for the characterisation of coordination compounds. Coord Chem Rev 7:81.
- A Earnshaw. Introduction to magnetochemistry. 1st ed. London: Academic press; (1968).
- ABP Lever. Inorganic electronic spectroscopy. 2nd ed. Amsterdam: Elsevier; (1984).
- FA Cotton, G Wilkinson, CA Murillo, and M Bochmann. Advanced inorganic chemistry. 6th ed. New York: John Wiley & Sons Inc; (2003).
- KK Narang, JP Pandey, and VP Singh. (1994). Synthesis, characterization and physicochemical studies of some Copper(II) tetrathiocyanato dithallate(I) complexes with hydrazides and hydrazones. Polyhedron 13:529.
- KK Narang, and VP Singh. (1996). ESR studies on acylhydrazine and hydrazone copper(II) sulfate complexes. Transition Met Chem 21:507.
- D Kivelson, and R Neiman. (1961). ESR studies on the bonding in copper complexes. J Chem Phys 35:149.
- SA Shama, and H Omara. (2001). Synthesis and characterization of some transition metal complexes with Shiff bases derived from 2-hydroxy acetylacetophenone. Spectroscopy Letters 34:49.
- MC Styka, RC Smierciak, EL Blinn, RE Desimone, and RV Passariella. (1978). Copper complexes containing a 12-membered macrocyclic ligand. Inorg Chem 17:82.
- KK Narang, and VP Singh. (1993). Synthesis and characterization of cobalt(II), nickel(II), copper(II) and zinc(II) complexes with acetylacetone bis-benzoyl hydrazone and acetylacetone bis-isonicotinoyl hydrazone. Transition Met Chem 18:287.
- ZH Chohan, MA Farooq, A Scozzafava, and CT Supuran. (2002). Antibacterial Shiff bases of oxalyl-hydrazine/diamide incorporating pyrolyl and salicylyl moieties and of their zinc(II) complexes. J Enz Med Chem 17:1.
- LV Azaroff, and MJ Buerger. The powder methods in X-ray crystallography. New York: Mc-Graw Hill; (1958) p 119.
- KK Narang, VP Singh, SK Singh, and GD Mishra. (1996). Synthesis, characterization, X-ray diffraction studies and antifungal activity of cobalt(II) complexes with some aroylhydrazines. Synth React Inorg Met-Org Chem 26:191.
- PK Panchal, HM Parekh, and MN Patel. (2005). Preparation, characterization and toxic activity of oxovanadium(IV) mixed-ligand complexes. Toxicol Environ Chem 87:(2005).
- K Deepa, and KK Aravindakshan. (2005). Synthesis, characterization and antifungal studies of metal complexes of benzoyl and salicylylhydrazones of ω-bromoacetoacetanilide. Synth React Inorg Met-Org Nano-Met Chem 35:409.