Abstract
D-Amino acid oxidase (DAO), a potential risk factor for schizophrenia, has been proposed to be involved in the decreased glutamatergic neurotransmission in schizophrenia. Here we show the inhibitory effect of an antipsychotic drug, chlorpromazine, on human DAO, which is consistent with previous reports using porcine DAO, although human DAO was inhibited to a lesser degree (Ki = 0.7 mM) than porcine DAO. Since chlorpromazine is known to induce phototoxic or photoallergic reactions and also to be transformed into various metabolites, we examined the effects of white light-irradiated chlorpromazine on the enzymatic activity. Analytical methods including high-resolution mass spectrometry revealed that irradiation triggered the oligomerization of chlorpromazine molecules. The oligomerized chlorpromazine showed a mixed type inhibition with inhibition constants of low micromolar range, indicative of enhanced inhibition. Taken together, these results suggest that oligomerized chlorpromazine could act as an active substance that might contribute to the therapeutic effects of this drug.
Introduction
D-Amino acid oxidase (EC 1.4.3.3, DAO) is a flavoenzyme that contains noncovalently bound FAD as a prosthetic group and catalyzes the oxidation of D-amino acids to give the corresponding imino acids while reducing molecular oxygen to hydrogen peroxide. The imino acids are spontaneously hydrolyzed to α-keto acid and ammonia. In mammals, DAO is found at highest concentrations in the kidney, and also in the liver and brain. We have previously determined the primary structures of DAO mRNAs isolated from the porcine [Citation1] and human [Citation2] kidney, and also detected a single mRNA species in the brain [Citation3]. In addition, we reported the structural organization of the human DAO gene and its expression, and localized the gene for DAO to human chromosome 12 [Citation4]. Recently, we demonstrated the gene expression of DAO in type-1 astrocytes from rat cerebellum and cerebral cortex [Citation5], and confirmed that extracellular D-serine is metabolized by DAO expressed in astrocytes [Citation6]. In the brain, activation of DAO, which leads to enhanced degradation of D-serine, an endogenous co-agonist of the NMDA receptors, was implicated in the altered NMDA receptor-mediated neurotransmission in schizophrenia.
Schizophrenia is a severe but common mental disease that affects 1% of the world's population. In the late 1950s, the prognosis of schizophrenia was dramatically improved with the introduction of a dopamine D2 receptor antagonist, chlorpromazine (CPZ). Since then, CPZ has been widely used as an antipsychotic drug in the treatment of this disease [Citation7]. Recently, DAO itself and in combination with a novel gene, G72, whose gene product was reported to physically interact with DAO and enhance the activity of the enzyme in vitro, were shown to be associated with schizophrenia [Citation8]. In this context, it is notable that CPZ inhibited porcine DAO by competition with FAD [Citation9]. However, the effects of antipsychotic drugs on human DAO, which is expected to actually play a role in pathophysiology in schizophrenia, have not been investigated. Therefore, the first aim of the present study was to examine whether one of the most widely used antipsychotic drugs, CPZ, influences the activity of the human enzyme.
Since it is well recognized that CPZ metabolism plays an important role in the overall antipsychotic activity of CPZ [Citation10], we further investigated whether metabolites of CPZ affect the activity of human DAO in the next part of the study. CPZ is metabolized into a number of related compounds. Examination of urine showed that less than 1% of over 20 metabolites of the drug consisted of free CPZ [Citation11], indicating that CPZ is extensively metabolized into other related compounds. CPZ causes both phototoxic and photoallergic reactions in patients receiving this drug [Citation12,13], and free radicals from CPZ have a crucial role in the damage of tissues induced through phototoxic reactions [Citation14]. In early reports, CPZ was shown to acquire inhibitory activity against D-glyceraldehyde-3-phosphate dehydrogenase during incubation in daylight [Citation15], and the inhibitory effects of CPZ free radicals on enzymes were demonstrated [Citation16,17]. Radicals derived from CPZ can be generated by several mechanisms, including photochemical [Citation16] and enzymatic reactions [Citation18]. CPZ sulfoxide, one of the known major physiological metabolites, can also be generated in vitro by photochemical [Citation19] and enzymatic reactions [Citation20]. Substances isolated from UV-irradiated CPZ solution were also found in the liver and urine of patients who had received long-term medication with CPZ [Citation21,22]. Reactions occurring under light exposure might thus partly duplicate reactions in vivo [Citation23]. Therefore, examining the effect of light in in vitro systems might be helpful in finding active compounds.
In this study, we show the inhibition of the activity of human DAO by CPZ and its related compound, oligomerized CPZ, in vitro, indicating that oligomerized CPZ is a pharmacologically active substance with a possible involvement in the glutamatergic system through the inhibition of DAO.
Materials and methods
Drugs and reagents
D-Alanine (Wako, Osaka, Japan) was dissolved in water and stored as a 1 M stock solution at − 30°C. FAD (Wako) was dissolved in water and stored as a 50 mM stock solution at − 30°C. Chlorpromazine hydrochloride (Wako) solution was freshly prepared prior to use under minimum light to give a concentration of 100 mM or 125 mM (w/v). (+)-Sodium L-ascorbate (Sigma-Aldrich, MO, USA) and L(+)-ascorbic acid (Wako) solutions were prepared just prior to use. Other chemicals are of analytical grade.
Enzyme purification and preparation of apoenzyme
Human DAO was expressed and purified as described [Citation24]. The preparation of the apoenzyme of human DAO was prepared by extensive dialysis of the holoenzyme against 1 M potassium bromide according to the method of Massey and Curti [Citation25]. The absence of an absorption band around 450 nm demonstrated the complete removal of FAD during the preparation. Then the apoenzyme solution was divided into aliquots and frozen in liquid nitrogen, and then stored at − 80°C. A frozen aliquot was thawed immediately before use. The apoenzyme was stable at − 80°C for at least several months. The concentration of apoprotein was determined using the extinction coefficient of 73,000 M− 1 cm− 1 at 280 nm [Citation26]. Its catalytic activity (5.1 × 102 min− 1) was almost identical to that of the holoenzyme and agreed well with the previous reports on human and porcine enzymes [Citation24,27,28] when measured by means of an oxygraphic method at pH 8.3 with saturating concentrations of FAD (0.03 mM) and D-alanine (0.05 M) at 25°C using the oxygen concentration of 250 μM in an air-equilibrated aqueous solution.
Formation of photoproducts and gel filtration chromatography
Typically, a 125 mM transparent solution of CPZ in a glass tube sealed with parafilm was irradiated with white light for approximately 2.5 h until the colour of the solution turned brown. During the irradiation, sample tubes were put in an ice-water bath to keep the temperature below 25°C. The light intensity in the 290-340 nm region was approximately 43 mW/cm2 at the position of the sample when measured with a UV radiometer UV-340 (Custom, Tokyo, Japan). After the irradiation, the solution was gel fractionated. Typically, a 3% column-volume of sample was size-fractionated on a Sephadex G-25 fine column (GE Healthcare, St. Giles, UK) (40 × 1 cm, size exclusion limit of 5 kDa) equilibrated and run at room temperature in degassed water at a flow rate of 0.5 mL/min. Fractions (1 mL) were examined by measuring UV absorption. Absorption spectra were obtained with a spectrophotometer (Beckman, model DU® 640).
Electron paramagnetic resonance (EPR) spectroscopy
EPR spectra were recorded at 9 GHz on a JEOL JES-TE 300 spectrometer equipped with a cavity and an aqueous quartz flat cell. The lyophilized Fraction i (fraction containing inhibitory substance(s) with high molecular weight(s)) and powder of CPZ were dissolved in D2O (Thermo Fisher Scientific Inc., MA, USA). For UV irradiation, samples were irradiated with UV through the quartz cell attached to the EPR cavity. The irradiation source was a 300 W xenon arc light (Ushio, Tokyo, Japan). EPR data were analysed with the software ESPRIT 432 (JEOL Co., Ltd., Tokyo, Japan). Measurements were carried out in air-saturated solution at room temperature.
NMR spectroscopy
1H-NMR spectra were recorded at 400 MHz on a JEOL-JNM-AL 400 spectrometer with the temperature of the NMR samples maintained at 300 K, using a 5-mm-diameter sample tube. The lyophilized Fraction i and powder of CPZ were dissolved in CD3OD (Thermo Fisher Scientific Inc.). 1H-NMR spectra were obtained by acquisition of 32 scans.
Electrospray ionization-time of flight-mass spectrometry (ESI-TOF-MS) analysis
The eluates of the Sephadex G-25 column were evaporated to dryness with methanol and analysed using a liquid chromatography/mass spectrometry system, Agilent Time-of-Flight LC/MS, which was operated in positive ion mode. The separation of the collected fraction (#33–36) was carried out using a reversed-phase C3 analytical column of 50 mm × 2.1 mm and 5 × 10− 6 m particle size (ZORBAX Eclipse® SB-C3). Mobile phases A and B were 0.1% acetic acid aqueous solution and methanol, respectively. Gradient elution was performed using a linear gradient from 50 to 100% solvent B for 5 min. The flow-rate was 0.5 mL/min. Measurements of CPZ and Fraction i were conducted without liquid chromatography. These samples were introduced into the mass spectrometer with the carrier solvents as follows: for CPZ, 0.1% acetic acid-methanol (50:50, v/v) at a flow rate of 0.3 mL/min, and for Fraction i, the same condition as that for CPZ or 0.1% acetic acid-acetonitrile (5:95, v/v) at a flow rate of 0.5 mL/min. Eluates were monitored by measuring the absorbance at 255 nm.
Determination of the concentration of Fraction i
The fraction that showed the strongest inhibition of DAO (Fraction i) was frozen at − 80°C and lyophilized overnight to remove moisture completely. Then the weight of the powder was measured, and the mean value was determined from 4 experiments. In a typical experiment, 0.30 mg of Fraction i was obtained after lyophilization, from 44 mg of CPZ. The molecular weight of the trimer was used for the determination of the concentration of Fraction i, as trimer was expected to be the major component of Fraction i from the ESI-TOF-MS experiment.
Measurement of the enzymatic activity
The activity of DAO was measured by monitoring the O2 consumption rate at air saturation using a D-amino acid as substrate with a Clarke oxygen electrode (Gilson, model 5/6 Oxygraph). The constituents of standard reaction mixture were 5 μg DAO (apoprotein), FAD, D-alanine and 0.05 M sodium pyrophosphate (pH 8.3), in a total volume of 1.8 mL. In typical experiments, DAO and FAD were preincubated for 3 min, then D-alanine was added as a substrate to start the reaction. Each reaction time was less than approximately 10 min. Kinetic parameters of the enzyme reactions were calculated from the conventional Michaelis equations [Citation29,30]. The following values were obtained at pH 8.3 and 25°C from double-reciprocal plots: Michaelis constant Km (for D-alanine) = 1 mM, the apparent dissociation constant for FAD Kf = 0.2 μM. These values are in good agreement with those reported [Citation24,29]. The apoprotein used was found to have no activity without the addition of exogenous FAD. In the assays to characterize the mechanisms of inhibition, kinetic parameters (Km, Kf and inhibition constants) were obtained using replots from double-reciprocal plots. In the case of a mixed type inhibition, two inhibition constants can be obtained. One is Ki, and the other is defined as K′i. The enzyme rate (v) is expressed as micromoles of oxygen consumption per minute per micromole of DAO. In cases when either FAD or substrate was not being varied in concentration, it was held at saturating levels. In assays using non-irradiated CPZ, the apoprotein was added to a mixture of FAD and CPZ (). The reactions were carried out at 37°C and pH 7.3, conditions that were employed in earlier studies [Citation31]. After a 5-min incubation, the reaction was initiated by the addition of substrate. In other assays ( and ), reactions were carried out using a similar procedure except for the incubation time (3 min) and temperature (25°C). Considering the stability of the compounds from the irradiated CPZ solution, we examined the effects of compounds from the irradiated CPZ solution on the enzyme at 25°C instead of 37°C.
Figure 1. Kinetic analysis of the effect of CPZ on DAO. The reaction mixtures contained 5 μg of DAO (apoprotein), 50 mM D-alanine, FAD and CPZ as indicated, and 0.1 M sodium pyrophosphate (pH 7.3), in a total volume of 1.8 mL. The final CPZ concentrations were: none (open triangles), 200 μM (filled diamonds), 300 μM (open squares), and 400 μM (filled circles). Assays were carried out at 37°C. The data are representative of three experiments.
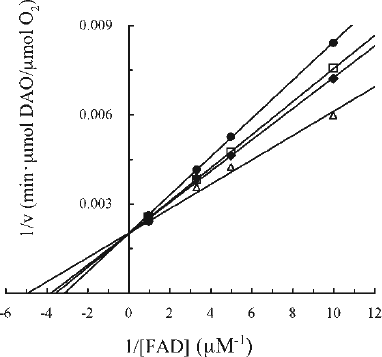
Figure 2. Elution profile of irradiated CPZ. The absorbances at 254 and 263 nm correspond to the λmax of CPZ and fraction #12, respectively. The effect of the fractions on DAO activity was examined at 25°C. The reaction mixtures contained 4 μg of DAO (apoprotein), 50 mM D-alanine, 0.3 μM FAD, 100 μL effluent and 0.1 M sodium pyrophosphate (pH 7.3), in a total volume of 1.8 mL. The ratio of DAO activity in the presence of each fraction was determined relative to the activity measured in the absence of effluent.
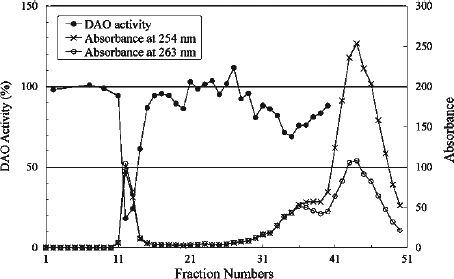
Statistics
Data were analysed by one-way ANOVA with an independent post-hoc Turkey's multiple comparison test. Data are expressed as mean ± SD. A difference was considered to be significant at p < 0.01.
Results
Inhibition of human DAO activity by CPZ and irradiated CPZ
CPZ was reported to inhibit the activity of porcine DAO by competition with FAD (Ki = 2.3 × 10− 5 M at pH 8.3 and Ki = 5 × 10− 5 M at pH 7.3, 37°C) [Citation30,31]. In order to evaluate the effect of CPZ on human DAO, we examined the characteristics of human DAO in the presence of CPZ by kinetic analysis as described by Yagi et al. [Citation30]. As shown in , we found that CPZ had an inhibitory effect on human DAO activity as well, but to a lesser degree than on porcine DAO. The inhibition could be demonstrated only over a narrow range of CPZ concentration (up to 400 μM) due to the limited solubility of CPZ at pH 7.3 [Citation32]. Under the conditions employed, CPZ inhibited the DAO activity in a concentration-dependent manner, and the double-reciprocal plots of the velocity in the presence or absence of CPZ against the concentration of FAD showed a series of lines that converged at a point on the y axis. This result indicated that CPZ inhibits DAO in a competitive manner with respect to FAD with an inhibition constant (Ki) of 0.7 mM and an apparent dissociation constant for FAD (Kf) of 0.2 μM. The Ki and Kf values were obtained based on replots from the double-reciprocal plots. The Kf value is in good agreement with previous findings obtained at the same pH [Citation31]. Considering the possibility that CPZ will undergo transformation in vivo and thereby gain further activity against the enzyme, we next tested the effects of CPZ-derived substances on DAO. Exposure to light could enhance the inhibitory capacity of CPZ as reported previously for its activity against D-glyceraldehyde-3-phosphate dehydrogenase [Citation15].
To prepare CPZ derivatives, CPZ solution was irradiated with white light. The solution that was colourless at the beginning turned deep brown at the end of the irradiation, and the pH was shifted from 6.8 to 3.8. We then tested DAO activity with the addition of the solution of irradiated CPZ and found that the inhibitory capacity of CPZ against the enzyme was increased roughly 10-fold in terms of a Ki value after light irradiation compared with that of non-irradiated CPZ, and the inhibition pattern was no longer competitive type with respect to FAD (data not shown).
Separation and identification of inhibitory CPZ photoproducts
To identify the active compounds that possess a stronger inhibitory effect than the original CPZ, we first conducted gel filtration to fractionate the compounds in the irradiated solution. The eluates were examined for the ability to inhibit the enzymatic activity. As shown in , the absorption data revealed that there were three peaks on the chromatogram based on the absorbance at 254 nm: fractions #12, 36 and 44. Among them, the 12th fraction, which appeared very near the void volume and corresponded to the first absorption peak, showed the strongest inhibition (82% inhibition of DAO activity). In contrast, the last-eluting absorbance peak (strongest absorption at fraction #44) showed weak inhibitory effects (maximal 20% inhibition of DAO activity) (data not shown).
As shown in , there was a shift in the λmax in these spectra with increasing fraction number. The λmax of fraction #12 (263 nm) was red-shifted by 9 nm compared with CPZ (254 nm). Absorbance at either 245 nm, which represents CPZ sulfoxide [Citation19] or 530 nm, which is characteristic of a cation radical of CPZ [Citation33], was not observed in the spectra shown in , indicating that these compounds are not candidates as inhibitory compound(s). The elution profile constructed using the absorbance at 263 nm was similar to that obtained using the absorbance at 254 nm ().
Figure 3. Absorption spectra of CPZ (20 μM), gel filtration fractions #12, 36 and 44. Fractions were diluted with H2O. The dilution factors for fractions #12, 36 and 44 were 1/100, 1/50 and 1/200, and the λmax for the fractions were 263, 257 and 254, respectively. Authentic CPZ has λmax at 254 nm.
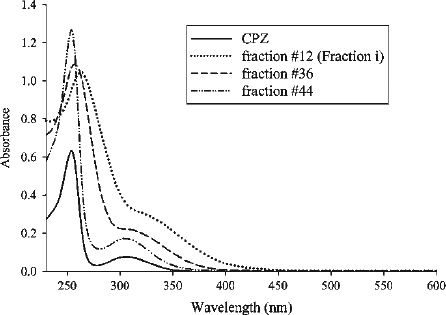
In order to identify the substance(s) in fraction #12 (named Fraction i), we next analysed this fraction using EPR, NMR and mass spectrometry.
EPR measurement
To detect any radicals in Fraction i, we measured the EPR spectrum of the fraction and detected only a fairly weak signal (, top). However, when the same sample was irradiated with UV while measuring the EPR spectrum, it gave rise to a distinct big signal, indicating the production of radical(s) (, centre). The line shape of the spectrum was similar to that observed without UV irradiation (, top) and different from that generated for UV-irradiated CPZ (, bottom). The peak-to-trough width of spectra (indicated by arrows) of Fraction i and CPZ obtained under conditions of UV irradiation was 10 gauss and 16 gauss, respectively (, centre and bottom), indicating the difference between the two EPR spectra. These results suggest that the inhibitory substance(s) in this fraction still have the ability to generate radicals on UV irradiation, although the radical(s) derived from Fraction i are not identical to the radical(s) derived from CPZ. The inhibitory substance might be apt to release an electron when irradiated, or possess a chloride function that is probably cleaved upon irradiation to generate radicals, as reported for the chloride function at position 2 of the CPZ molecule [Citation14].
Figure 4. Examination for radicals. (a) EPR spectra of Fraction i in D2O (estimated to be 2 mM based on the measurement of dry weight) obtained without (top) and under (centre) UV irradiation, and EPR spectrum for 56 mM CPZ in D2O measured under UV irradiation (bottom). EPR conditions were: power, 20 mW; sweep width, 3358.4 ± 25 Gauss; modulation amplitude, 0.2 Gauss; time constant, 0.1 s; sweep time, 4 min; gain, 2 000 (top), 1 000 (centre), 500 (bottom). (b) Effect of radicals on DAO activity. The reaction mixtures contained 5 μg of DAO (apoprotein), 50 mM D-alanine, 0.3 μM FAD, 20 μL of Fraction i (estimated to be 2.9 μM based on the measurement of dry weight) and 1 mM ascorbate as indicated and 0.1 M sodium pyrophosphate (pH 7.3), in a total volume of 1.8 mL. Fraction i and ascorbate were preincubated for 3 min, then FAD and the apoprotein were added. After another 3-min incubation, D-alanine was added as substrate. Assays were carried out at 25°C. Data are the mean ± SD of three experiments. *p < 0.01, compared with control measured in the absence of Fraction i and ascorbate (one-way ANOVA with post-hoc Tukey's multiple comparison test).
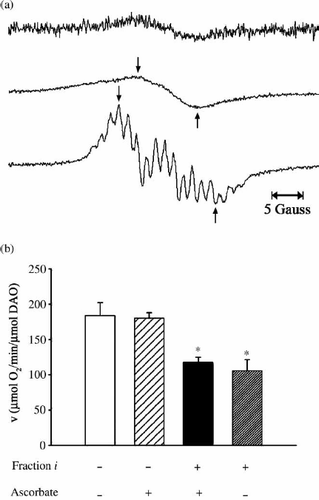
Since a trace amount of radical was observed in the EPR spectrum of Fraction i, we investigated whether radical(s) were directly involved in the inhibition by examining the effect of a radical scavenger, ascorbate, on the inhibition of the enzymatic activity. We confirmed that CPZ-derived radical(s) were not detected by EPR in the presence of ascorbic acid when CPZ solution was analysed under UV irradiation or immediately after 1 h of white light irradiation (data not shown), whereas the omission of ascorbic acid resulted in the detection of distinct CPZ-derived radicals by EPR. As shown in , the radical-scavenging activity of ascorbate did not alter the inhibitory effect of Fraction i, which caused significant inhibition in both the presence and absence of ascorbate. Therefore, we concluded that radical(s) are not responsible for the enzyme inhibition.
NMR measurement
We carried out NMR experiments to determine the structure of Fraction i. A comparison of the 1H-NMR spectra for Fraction i and authentic CPZ is presented in . Line broadening was observed in the spectrum of Fraction i (, top), which suggests the existence of a polymer or a radical in the solution, and therefore further NMR analysis for structural assignments was considered to be inconclusive. The chemical shifts of Fraction i were similar to those of CPZ (, bottom), suggesting that Fraction i has structural similarity to CPZ. The collected fraction (#42–45), which corresponded to the final absorbance peak on the gel filtration chromatogram (), was also analysed by NMR. The constituent in these fractions was identified as CPZ by comparing its 1H-NMR and UV spectra with those of authentic CPZ. Considering the elution profile of Fraction i (#12), these results led us to expect that the molecular weight of Fraction i was larger than that of CPZ. Therefore, we assume that Fraction i likely contains a polymer, which is expected to have structural similarity to CPZ.
Figure 5. Line-broadening effect in 1H-NMR spectrum of Fraction i. The inset shows the structure of CPZ with the phenothiazine nucleus designated as A, the side chain carbon and methyl groups designated as α-γ and B, respectively. (Top) 1H spectrum (CD3OD at 400 MHz) of Fraction i. (Bottom) 1H spectrum (CD3OD at 400 MHz) of CPZ. The strong peaks at δ3.35 and δ4.9 in both spectra are due to CD2HOD and CD3OH, respectively.
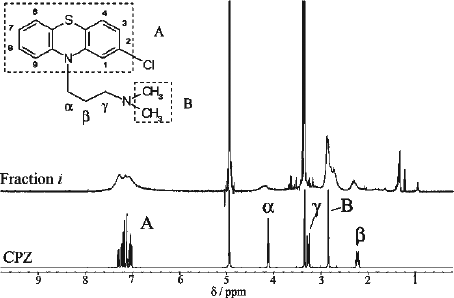
We then used high-resolution mass spectrometry measurement to identify the constituents of Fraction i and their elemental compositions.
Identification of the inhibitory constituents by high-resolution mass spectrometry
Electrospray ionization-time of flight-mass spectrometry (ESI-TOF-MS) analysis of Fraction i revealed protonated molecular ions [M + H]+ at m/z 883.3417 and 1 165.4597. Because of the high affinity to the reversed phase (C18, C8 and C3) columns, the samples from Fraction i were introduced into the mass spectrometer through a capillary without liquid chromatography. The total-ion chromatogram (TIC), which was constructed by plotting the total ion current of the introduced sample against time, showed a single but broad and tailed peak from about 1 min with maximal intensity at 1.31 min (data not shown). As shown in , the protonated molecular ion [M + H]+ at m/z 883.3417 was detected by summation of the area under the peak including the top of the peak on the TIC between 1.1 and 1.4 min. No prominent peak was observed above m/z 1 200 in . Since Fraction i is expected to contain molecule(s) with higher molecular weight(s) than that of CPZ (molecular weight 318), the large peak below m/z 100 was considered to be a fragment ion. The peak at m/z 883.3417 was found to correspond to the formula weight for a trimer ion of CPZ [(3 CPZ − 2HCl) + H]+ based on comparison between the experimental and calculated isotope peak intensities of the molecular ion [M + H]+. High intensity of the signal for the trimer, together with lower intensity of the signal for tetramer (4 CPZ − 3HCl), was also found in the mass spectrum obtained from summing the area under the tailed part of the peak on the TIC between 1.3 and 1.6 min (data not shown). These results suggest that trimer is the major component in Fraction i.
Figure 6. ESI (positive ionization mode) mass spectrum of Fraction i obtained by summing the area beneath the peak on TIC (see text).
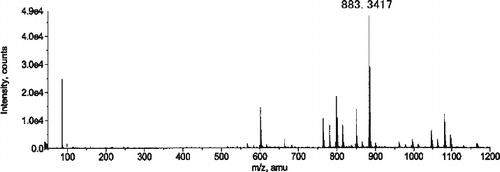
CPZ and the collected fraction (#33–36), which corresponded to the middle absorbance peak in , were also analysed using ESI-TOF-MS. Protonated molecular ions at m/z 319.1032 and m/z 601.2214, identified as the monomer and dimer (2 CPZ − HCl) of CPZ, were detected in the authentic CPZ sample and the collected fraction, respectively.
The results of ESI-TOF-MS analysis suggest that the first peak (Fraction i) and the middle peak on the gel filtration chromatogram () contained oligomers (mainly trimer) and dimer, respectively, which was consistent with the elution order in the gel filtration experiment. The detailed results of ESI-TOF-MS analysis are shown in . The difference between these m/z values of 282 m/z unit, which is the mass of the repeat unit of the oligomer, was found to correspond to the formula weight for CPZ devoid of H and Cl. On the basis of these results, we concluded that the major constituent of Fraction i, which contributed to inhibition of the DAO activity, was trimer of CPZ.
Table I. Summary Of Esi-tof-ms Analysis Of Cpz And Oligomers.
Kinetic analysis of the inhibitory activity of Fraction i
The mechanism of inhibition of DAO by Fraction i was addressed by investigating the effect of Fraction i on the kinetic parameters (Kf and apparent Vmax) as a function of FAD concentration in the presence of varying concentrations of Fraction i () using the same kinetic analysis procedures as described for CPZ (). The concentration of the oligomers in Fraction i was calculated using the molecular weight for the major compound, trimer (see “Materials and methods”). Fraction i inhibited the activity of DAO in a concentration-dependent manner under the conditions employed. The double-reciprocal plots showed that the inhibition was associated with a decrease in apparent Vmax and increase in Kf (). A series of lines intersected at a point to the left of the y axis, which is consistent with a mixed type inhibition with respect to FAD with a Ki of 2 μM, a K′i of 8 μM and a Kf of 0.2 μM. The Ki, K′i and Kf values were obtained based on the replots from the double-reciprocal plots. Since the apparent Vmax decreased with the increasing concentration of Fraction i, the inhibitory effect of Fraction i on the apoenzyme could be observed even in the presence of excess FAD, indicating that the manner of inhibition is not simple competitive type with FAD. In fact, when the activity of DAO was measured using the same kinetic analysis procedures with varying concentrations of a substrate (D-alanine) but in the presence of excess FAD (50 μM) (see “Materials and methods”), Fraction i exhibited a noncompetitive inhibition with a Ki of 7 μM and a Km of 2 mM (data not shown), which suggests that Fraction i does not interfere with the binding of D-alanine to the enzyme. Thus, Fraction i as well as CPZ inhibited the activity of DAO; moreover, the Ki value of Fraction i was decreased compared with that of CPZ, indicating that Fraction i inhibited DAO more efficiently than CPZ.
Figure 7. Kinetic analysis of the effect of Fraction i on DAO. The reaction mixtures were essentially the same as described in the legend to . Final Fraction i concentrations were: none (filled triangles), 0.92 μM (open diamonds), 1.5 μM (filled squares), 2.8 μM (open circles), and 3.7 μM (filled diamonds). Assays were carried out at 25°C. The data are representative of three experiments. Data points obtained at 1.5 μM and 2.8 μM of Fraction i in the presence of the lowest concentration of FAD were ignored.
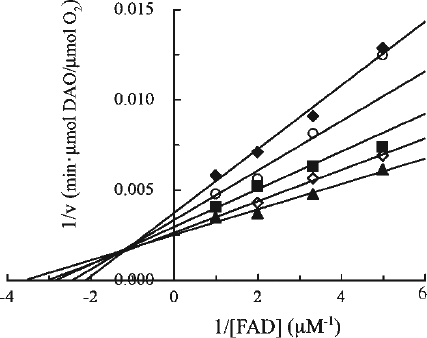
Discussion
In the present study, the inhibitory effects of a clinically used antipsychotic drug and its related compounds on human DAO, which can be a target of therapy for schizophrenia, were explored. The recent model of the pathophysiology of schizophrenia emphasizes an imbalance between an increased dopaminergic and decreased glutamatergic neurotransmission [Citation34,35]. This hypothesis shed light on the regulation of the NMDA receptor-mediated neurotransmission. In this context, it is anticipated that drugs activating the NMDA receptors might be therapeutically beneficial. In fact, administration of the NMDA receptor co-agonist D-serine greatly improved symptoms of schizophrenia patients [Citation36]. It should be noted that beneficial actions of antipsychotic drugs are probably accompanied by an improvement of both the dopaminergic and glutamatergic neurotransmission [Citation37]. On the basis of the genetic and physiological data implicating the new human gene G72 and DAO in schizophrenia [Citation8], the activation of DAO by G72 may lead to enhanced degradation of D-serine followed by the hypofunction of the NMDA receptors in schizophrenia. Thus, inhibiting the activity of DAO can be therapeutically beneficial in the treatment of schizophrenia. Indeed, novel selective inhibitors of DAO have been intensively explored for clinical purposes [Citation38].
In the present study, using human DAO, CPZ showed a competitive type inhibition with respect to FAD. In addition to CPZ, other phenothiazine derivatives have been shown to inhibit porcine DAO by competing with FAD [Citation31]. The inhibitory capacities of these compounds were reported to be generally in good agreement with their relative clinical efficacy and potency in antipsychotic therapy. The inhibition of riboflavin metabolism in the rat tissues by CPZ was also reported [Citation39]. These observations suggest that CPZ might act by inhibiting flavoenzymes due to the structural similarity between the phenothiazine nucleus of CPZ and the isoalloxazine moiety of flavins. We previously reported the inhibition of D-serine metabolism, catalyzed by the overexpressed intracellular DAO, in glial cells treated with 1 μM CPZ [Citation6]. Taken together, these data suggest that inhibition of the catalytic activity of DAO has some connection with the pharmacological action of the drug.
Considering the importance of the metabolism of CPZ, its metabolites are also expected to contribute to the therapeutic effects. Therefore, we investigated whether there is/are CPZ-related compound(s) that is/are more effective as an inhibitor of this enzyme. The results of the activity assay demonstrated the inhibition of DAO by CPZ-derived substances from a white light-irradiated solution of CPZ. In this study, no relationship was suggested between the observed inhibition of DAO and radicals from CPZ (), although inhibitory effects of the radicals on other enzymes have been reported [Citation16,17]. An ESI-TOF-MS study of the most potent fraction (Fraction i) that was eluted from the gel filtration column revealed that this fraction contained oligomerized CPZ (). Kinetic analysis showed that the inhibition of DAO by Fraction i was mixed type with respect to FAD () and noncompetitive type with respect to D-alanine (data not shown). The expected molecular weights of the oligomers were larger than that of a common D-amino acid or FAD molecule. Considering the architecture of the active site of human DAO [Citation24] and the manner of inhibition, CPZ oligomers are unlikely to enter the active site. Therefore, we assume that Fraction i impairs the catalytic activity of DAO likely by inhibiting the formation of the active holoenzyme, through preventing FAD from binding to the apoprotein and possibly through inhibiting the protein conformation change necessary for the formation of the active holoenzyme. Our preliminary results at present actually showed that Fraction i had little effect on the activity of DAO preincubated with excess FAD to form the holoenzyme.
The data obtained here suggest that Fraction i contains CPZ oligomers and these oligomers are responsible for the observed inhibition. These oligomers seem to be similar to a polymer of CPZ that was reported by Huang and Sands [Citation40]. They isolated a polymer, which showed a negative reaction for chlorine, from the UV-irradiated solution of CPZ and postulated the structure of 2-7 linked polymer. Our study provides evidence for this structure as follows. (1) As reported for CPZ [Citation14], the ability of Fraction i to generate radicals () would be partly due to the residual chloride function. In this study, we observed oligomers with one chlorine atom. Considering the possibility that the chloride function can be cleaved during the course of radical generation, the reported polymer devoid of the chloride function may be produced from that with the chloride function. (2) Structural analogy between CPZ and Fraction i is expected based on the similarity in their chemical shifts (). (3) High-resolution mass spectra revealed a series of molecular species with a repeat unit that is expected to be CPZ devoid of H and Cl. An acidic shift of pH (from 6.8 to 3.8) was observed during the course of white light irradiation. This could have resulted from liberation of hydrogen chloride, followed by subsequent formation of an oligomer. In fact, a dechlorinated compound of CPZ, promazine, was reported to be a major plasma metabolite in patients, indicating that dechlorination of CPZ represents an important metabolic pathway [Citation41]. Therefore, the observed oligomers and the reported polymer of CPZ would be generated through similar mechanisms. The deduced structure of an oligomer (trimer) is depicted in . The peak in the mass spectrum () at m/z below 100, which was revealed to be m/z 86, was considered to be a fragment ion that corresponds to the side chain of the CPZ molecule ( − CH2CH2CH2N(CH3)2, formula weight 86) [Citation42].
Figure 8. The deduced structure of trimer of CPZ. CPZ devoid of H and Cl (designated with dashed line) is thought to be a repeat unit (formula weight 282) for the observed oligomers.
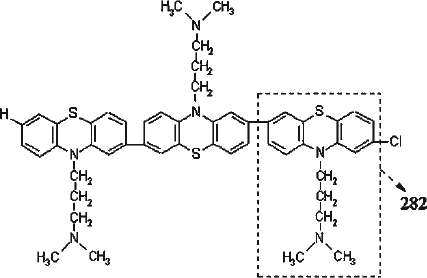
The addition of a radical scavenger to CPZ solution suppressed EPR radical signals both under UV irradiation and after white light irradiation. The dark colour in the solution increased with irradiation time, while ascorbic acid suppressed the development of the colour. The inhibitory effect of the irradiated solution on DAO also seemed to correlate with irradiation time. These facts suggest that the amount of oligomer increases according to the irradiation. Taken together, these observations indicate that the first step of polymerization is probably the formation of radicals.
Van Woert isolated brown pigments from the liver of patients who had received prolonged, high-dose CPZ treatment [Citation21]. He showed that the chemical characteristics of the pigments were similar to those of the polymer produced by UV irradiation of CPZ in vitro, as referred to above [Citation40]. Tests of the biological activity of the polymer produced by UV irradiation of CPZ on human volunteers suggested that formation of a polymer is the cause of the skin discolouration observed in patients [Citation40]. Evidence for the presence of a polymer of the same nature in the urine of psychotic patients receiving long-term medication with CPZ was also presented [Citation22].
In the rat liver, kidney and brain, the tissue-to-plasma partition ratios of CPZ concentration after oral administration were calculated based on the report by Sgaragli et al. to be 98, 34 and 21, respectively [Citation43]. The accumulation in the liver showed the highest ratio. The time course of CPZ concentration in rat serum was reported to be approximately 1 μg/mL immediately after intravenous administration (4 mg/kg) and then decline biexponentially with time [Citation44]. The decreased concentration in the serum probably means that CPZ is taken up by the tissues. Since CPZ exhibits good cell permeability and crosses the blood-brain barrier due to its hydrophobicity, a high local concentration of the drug might be achieved in these tissues. At physiological pH (7.3), CPZ molecules form micelles, with the critical micelle concentration of 0.2 mM [Citation45]. Considering the presence of the polymers of CPZ in the liver and urine of patients, local concentrations of CPZ might be high enough to cause polymerization, although these concentrations are not yet known exactly. Concentrated CPZ might polymerize as a result of photoirradiation or radical generation in cells.
In conclusion, we have shown the functional inhibition of DAO by an antipsychotic drug, CPZ, and oligomers of this drug in vitro. Detailed studies on the effects of oligomerized CPZ on physiological, especially neural, systems will be necessary to better understand the actions of these substances in vivo. Furthermore, examination of effects of antipsychotic drugs and their metabolites on DAO might pave the way for the development of novel therapeutic approaches for schizophrenia.
Acknowledgements
We are grateful to Dr. H. Uchida (Agilent Technologies Japan, Ltd., Tokyo, Japan) for the courtesy of his conducting detailed analyses in ESI-TOF-MS. We also wish to thank Mr. K. Kodama and Mr. A. Nishigami for their help with the measurement of the NMR spectra and helpful discussions. We extend our deepest thanks to Dr. H. Takiwaki and Dr. E. J. Jung for helpful comments and information. This work was supported by a grant for the 21st Century COE Program from the Ministry of Education, Culture, Sports, Science and Technology of Japan, by a Grant-in-Aid for Scientific Research from the Japan Society for the Promotion of Science, and by a Health and Labour Sciences Research Grant from the Ministry of Health, Labour and Welfare of Japan.
References
- K Fukui, F Watanabe, T Shibata, and Y Miyake. (1987). Molecular cloning and sequence analysis of cDNAs encoding porcine kidney D-amino acid oxidase. Biochemistry 26:3612–3618.
- K Momoi, K Fukui, F Watanabe, and Y Miyake. (1988). Molecular cloning and sequence analysis of cDNA encoding human kidney D-amino acid oxidase. FEBS Lett 238:180–184.
- K Fukui, K Momoi, F Watanabe, and Y Miyake. (1988). In vivo and in vitro expression of porcine D-amino acid oxidase: In vitro system for the synthesis of a functional enzyme. Biochemistry 27:6693–6697.
- K Fukui, and Y Miyake. (1992). Molecular cloning and chromosomal localization of a human gene encoding D-amino-acid oxidase. J Biol Chem 267:18631–18638.
- Y Urai, O Jinnouchi, KT Kwak, A Suzue, S Nagahiro, and K Fukui. (2002). Gene expression of D-amino acid oxidase in cultured rat astrocytes: Regional and cell type specific expression. Neurosci Lett 324:101–104.
- HK Park, Y Shishido, S Ichise-Shishido, T Kawazoe, K Ono, S Iwana, Y Tomita, K Yorita, T Sakai, and K Fukui. (2006). Potential role for astroglial D-amino acid oxidase in extracellular D-serine metabolism and cytotoxicity. J Biochem (Tokyo) 139:295–304.
- A Sawa, and SH Snyder. (2002). Schizophrenia: Diverse approaches to a complex disease. Science 296:692–695.
- I Chumakov, M Blumenfeld, O Guerassimenko, L Cavarec, M Palicio, H Abderrahim, L Bougueleret, C Barry, H Tanaka, P La Rosa, and et al (2002). Genetic and physiological data implicating the new human gene G72 and the gene for D-amino acid oxidase in schizophrenia. Proc Natl Acad Sci USA 99:13675–13680.
- K Yagi, T Nagatsu, and T Ozawa. (1956). Inhibitory action of chlorpromazine on the oxidation of D-amino-acid in the diencephalon part of the brain. Nature 177:891–892.
- PKF Yeung, JW Hubbard, JK Cooper, and KK Midha. (1983). A study of the kinetics of chlorpromazine sulfoxide by a specific radioimmunoassay after a single oral dose of chlorpromazine in healthy volunteers. J Pharmacol Exp Ther 226:833–838.
- EGC Clarke, editor. An extra pharmacopoeia companion volume: Isolation and identification of drugs in pharmaceuticals, body fluids and post-mortem materialLondon: Pharmaceutical Press; (1969). p 256–257.
- B Ljunggren. (1977). Phenothiazine phototoxicity: Toxic chlorpromazine photoproducts. J Invest Dermatol 69:383–386.
- J Menz, SA Muller, and SM Connolly. (1988). Photopatch testing: A six-year experience. J Am Acad Dermatol 18:1044–1047.
- CF Chignell, AG Motten, and GR Buettner. (1985). Photoinduced free radicals from chlorpromazine and related phenothiazines: Relationship to phenothiazine-induced photosensitization. Environ Health Perspect 64:103–110.
- M Wollemann, and P Elödi. (1961). Die Hemmung der kristallisierten D-glyceraldehyd-3-phosphat dehydrogenase aktivität durch phenothiazine und aminoketone. [Inhibition of crystallized D-glyceraldehyde-3-phosphate dehydrogenase activity by phenothiazine and aminoketone.]. Biochem Pharmacol 6:228–232.
- T Akera, and TM Brody. (1972). Effects of chlorpromazine free radical on brain microsomal enzymes. Biochem Pharmacol 21:1403–1411.
- S Muraoka, and T Miura. (2003). Inactivation of cholinesterase induced by chlorpromazine cation radicals. Pharmacol Toxicol 92:100–104.
- LH Piette, G Bulow, and I Yamazaki. (1964). Electron-paramagnetic-resonance studies of the chlorpromazine free radical formed during enzymic oxidation by peroxidase-hydrogen peroxide. Biochim Biophys Acta 88:120–129.
- B Ljunggren, and H Möller. (1977). Phenothiazine phototoxicity: An experimental study on chlorpromazine and its metabolites. J Invest Dermatol 68:313–317.
- DJ Cavanaugh. (1957). Oxidation of chlorpromazine by peroxidase and catalase. Science 125:1040–1041.
- MH Van Woert. (1968). Isolation of chlorpromazine pigments in man. Nature 219:1054–1056.
- CL Huang. (1967). Isolation and identification of urinary chlorpromazine metabolites in man. Int J Neuropharmacol 6:1–13.
- IS Forrest, FM Forrest, and M Berger. (1958). Free radicals as metabolites of drugs derived from phenothiazine. Biochim Biophys Acta 29:441–442.
- T Kawazoe, H Tsuge, MS Pilone, and K Fukui. (2006). Crystal structure of human D-amino acid oxidase: Context-dependent variability of the backbone conformation of the VAAGL hydrophobic stretch located at the si-face of the flavin ring. Protein Sci 15:2708–2717.
- V Massey, and B Curti. (1966). A new method of preparation of D-amino acid oxidase apoprotein and a conformational change after its combination with flavin adenine dinucleotide. J Biol Chem 241:3417–3423.
- AA Raibekas, K Fukui, and V Massey. (2000). Design and properties of human D-amino acid oxidase with covalently attached flavin. Proc Natl Acad Sci USA 97:3089–3093.
- G Molla, S Sacchi, M Bernasconi, MS Pilone, K Fukui, and L Pollegioni. (2006). Characterization of human D-amino acid oxidase. FEBS Lett 580:2358–2364.
- C Setoyama, Y Nishina, H Tamaoki, H Mizutani, I Miyahara, K Hirotsu, K Shiga, and R Miura. (2002). Effects of hydrogen bonds in association with flavin and substrate in flavoenzyme D-amino acid oxidase. The catalytic and structural roles of Gly313 and Thr317. J Biochem (Tokyo) 131:59–69.
- M Miyano, K Fukui, F Watanabe, S Takahashi, M Tada, M Kanashiro, and Y Miyake. (1991). Studies on Phe-228 and Leu-307 recombinant mutants of porcine kidney D-amino acid oxidase: Expression, purification, and characterization. J Biochem (Tokyo) 109:171–177.
- K Yagi, T Ozawa, and T Nagatsu. (1960). Mechanism of inhibition of D-amino acid oxidase. IV. Inhibitory action of chlorpromazine. Biochim Biophys Acta 43:310–317.
- S Gabay, and SR Harris. (1967). Studies of flavin-adenine dinucleotide-requiring enzymes and phenothiazines. 3. Inhibition kinetics with highly purified D-amino acid oxidase. Biochem Pharmacol 16:803–812.
- S Gabay, and SR Harris. (1965). Studies of flavin adenine dinucleotide-requiring enzymes and phenothiazines-I. Interactions of chlorpromazine and D-amino acid oxidase. Biochem Pharmacol 14:17–26.
- A Vázquez, J Tudela, R Varón, and F García-Cánovas. (1992). Determination of the molar absorptivities of phenothiazine cation radicals generated by oxidation with hydrogen peroxide/peroxidase. Anal Biochem 202:245–248.
- M Carlsson, and A Carlsson. (1990). Interactions between glutamatergic and monoaminergic systems within the basal ganglia–implications for schizophrenia and Parkinson's disease. Trends Neurosci 13:272–276.
- JT Coyle. (1996). The glutamatergic dysfunction hypothesis for schizophrenia. Harv Rev Psychiatry 3:241–253.
- G Tsai, P Yang, LC Chung, N Lange, and JT Coyle. (1998). D-Serine added to antipsychotics for the treatment of schizophrenia. Biol Psychiatry 44:1081–1089.
- BK Yamamoto, and MA Cooperman. (1994). Differential effects of chronic antipsychotic drug treatment on extracellular glutamate and dopamine concentrations. J Neurosci 14:4159–4166.
- PE Brandish, CS Chiu, J Schneeweis, NJ Brandon, CL Leech, O Kornienko, EM Scolnick, B Strulovici, and W Zheng. (2006). A cell-based ultra-high-throughput screening assay for identifying inhibitors of D-amino acid oxidase. J Biomol Screen 11:481–487.
- J Pinto, YP Huang, and RS Rivlin. (1981). Inhibition of riboflavin metabolism in rat tissues by chlorpromazine, imipramine, and amitriptyline. J Clin Invest 67:1500–1506.
- CL Huang, and FL Sands. (1967). Effect of ultraviolet irradiation on chlorpromazine. II. Anaerobic condition. J Pharm Sci 56:259–264.
- GP Sgaragli, R Ninci, L Della Corte, M Valoti, M Nardini, V Andreoli, and G Moneti. (1986). Promazine: A major plasma metabolite of chlorpromazine in a population of chronic schizophrenics. Drug Metab Dispos 14:263–266.
- Y Mizuno, K Sato, T Sano, R Kurihara, T Kojima, Y Yamakawa, A Ishii, and Y Katsumata. (2002). Identification and characterization of 17 phenothiazine compounds by capillary high-performance liquid chromatography/fast atom bombardment mass spectrometry. Leg Med (Tokyo) 4:207–216.
- GP Sgaragli, M Valoti, M Palmi, M Frosini, MG Giovannini, L Bianchi, and L Della Corte. (1995). Rat tissue concentrations of chlorimipramine, chlorpromazine and their N-demethylated metabolites after a single oral dose of the parent compounds. J Pharm Pharmacol 47:782–790.
- S Sato, and A Koshiro. (1995). Pharmacokinetic analysis of chlorpromazine in rat serum, cerebrospinal fluid and striatum. Biol Pharm Bull 18:593–599.
- E Wajnberg, M Tabak, PA Nussenzveig, CMB Lopes, and SRW Louro. (1988). pH-dependent phase transition of chlorpromazine micellar solutions in the physiological range. Biochim Biophys Acta 944:185–190.