Abstract
Hyaluronidases are enzymes controlling many crucial physiological processes. Imbalanced enzymatic activity is connected with severe diseases. Because there is limited availability of drugs modulating hyaluronidase activity, the search for hyaluronidase interacting compounds is getting more and more important. A series of fifteen indole carboxamides and acetamides were synthesized and tested on inhibition of bovine testes hyaluronidase. In vitro assays were performed using stains-all at pH 7 and the Morgan-Elson reaction at pH 3.5. At neutral pH, the most active inhibitory compound was N-(Pyridin-4yl)-[5-bromo-1-(4-fluorobenzyl)indole-3-yl]carboxamide (20) with an IC50 value of 46 μM. Surprisingly, inhibition of all compounds was completely abolished by a decrease in pH. At pH 3.5 the activity of the enzyme was increased up to 134% by compound N-(4,6-Dimethylpyridin-2yl)-(1-ethylindole-3-yl)acetamide (24) at a concentration of 100 μM. The known activating effect of bovine serum albumine (BSA) on hyaluronidase activity was verified in the assay and compared to the effect of compound 24. Structure-activity relationships are discussed and a model is proposed, which explains the increase in activity at pH 3.5 by bonding of the protonated form of N-(4,6-Dimethylpyridin-2yl)-(1-ethylindole-3-yl)acetamide (24) to hyaluronic acid. The bonding results in an elongated form of the substrate with easier enzymatic access.
Introduction
Hyaluronic acid (HA), a non-sulfated glycosaminoglycan also known as Hyaluronan, is consisting of repeated disaccharide units of D-glucuronic acid and N-acetyl-D-glucosamine [Citation1]. It is the main component of the extracellular matrix and its turnover is undoubtedly connected with important physiological processes such as ovum fertilization [Citation2], angiogenesis [Citation3], cell adhesion and proliferation, growth or repair of tissues Citation4Citation5Citation6.
In human, HA concentration depends on the balance between synthesis via hyaluronate synthases [Citation7] and enzymatic degradation via hyaluronidases Hyal-1, Hyal-2 and PH20 [Citation8]. Elevated activity of hyaluronidases is connected with pathologic processes like inflammation or arthrosis [Citation9]. It has also been found in many human cancer cells [Citation10,Citation11]. Small HA fragments generated by degradation induce angiogenesis [Citation12] and show inflammatory effects [Citation13]. Furthermore, degradation of the extracellular matrix can support cancer cells leaving the primary tumor and forming metastasis. It has been found that hyaluronidase negative tumor cells showed significant reduced angiogenesis [Citation12] and Hyal-1-siRNA showed potency in cancer inhibition [Citation14]. Furthermore, Hyal-1 and PH20 can be used as tumor markers Citation15Citation16Citation17. On the other hand, decreased hyaluronidase activity can lead to severe diseases like mucopolysaccharidosis [Citation18]. Some type of cancer cells were reported to show increased HA concentrations and low activity of hyaluronidases Citation19Citation20Citation21, probably enabling these cells to hide from being identified by immunocompetent cells. In these cases, adding of hyaluronidases to the cytostatics made the cancer cells more sensitive against the cytostatic therapy [Citation22,Citation23].
Inhibitors of hyaluronidases could represent a completely new group of cytostatic drugs, contraceptives and anti-arthrosis therapy. Activators of hyaluronidases have also been identified over the last decades [Citation24,Citation25], but a potential use as drugs for treatment of low hyaluronidase activity has never been discussed. Benzimidazole and indole derivatives have been tested as hyaluronidase inhibitors before Citation26Citation27Citation28Citation29. Buschauer and co-workers identified several inhibitors of hyaluronan lyase derived from Streptococcus agalactiae [Citation26,Citation27]. 1-Decyl-2-(4-sulfamoyloxyphenyl)-1H-indol-6-yl sulfamate showed an IC50 value of 11 μM at pH5 [Citation27] and 1,3-diacetylbenzimidazole-2-thione of 160 μM at pH 5 and 5 μM at pH 7.4 [Citation26]. N-substituted benzoxazole-2-thione derivatives were also tested and IC50 values between 15 and 260 μM [Citation27] were obtained. Our group examined indole-2- and 3-carboxamide derivatives for their BTH inhibitory activities and found an IC50 value of 26 μM for N-(4-fluorobenzyl)-[1-(4-fluorobenzyl)indole-3-yl]carboxamid at pH 7 [Citation28].
In the present study, we tested a new series of carboxamide and acetamide derivatives for their hyaluronidase modulating capabilities at neutral and acidic pH values using our recently developed stains-all and Morgan-Elson assay [Citation29]. All mammalian hyaluronidases belong to an identical class of enzymes catalyzing the degradation of HA by cleavage of the β(1 → 4)-bonds between the disaccharide units. The most important human hyaluronidases are Hyal-1, Hyal-2 and PH20. In the present investigation all assays were performed using bovine testes hyaluronidase (BTH) which exhibits a homology of about 65% to PH20 and 40% to Hyal-1.
Hyal-2, PH20 and BTH show high activities over a wide range starting at neutral pH down to pH 3 Citation30Citation31Citation32, whereas Hyal-1 is much more active at acidic pH than at neutral pH [Citation31]. Because in contrast to blood and most healthy tissues, where a neutral pH has to be expected, the pH-value of inflamed areas is remarkably lower, e.g. in the state of arthrosis. Therefore we decided to test the compounds at pH 7 and pH 3.5 as described earlier [Citation29].
Materials and methods
Chemistry
Instrumentation
All chemicals and solvents were commercially available and of analytical or HPLC grade and supplied by Acros Organics (Geel, Belgium), Fisher Scientific (Nidderau, Germany), Merck (Darmstadt, Germany) or Sigma Aldrich (Steinheim, Germany). They were used without further purification. Hyaluronidase from bovine testes (BTH) was purchased from Serva (Heidelberg, Germany), hyaluronic acid was purchased from Sigma Aldrich (Steinheim, Germany). Water was purified using a Milli-Q Biocel system.
Melting points were determined using an Electrothermal IA9300 digital melting point apparatus and reported uncorrected. IR spectra were recorded on a Perkin-Elmer Paragon FTIR 1000 PC spectrometer in KBr. Only the most significant absorption bands have been reported. 1H NMR spectra were recorded on a Bruker AC250 (250 MHz) or on Bruker Avance 400 spectrometer (400 MHz). Chemical shifts are expressed as δ values (ppm) relative to tetramethylsilane as internal standard (in NMR description, s = singlet, d = doublet, and m = multiplet). Coupling constants J are given in Hz. All reactions were monitored by TLC analytic, using 0.2 mm-thick silica gel plates 60F–254 (5735 Merck). Column chromatography was carried out using silica gel 60 (70–230 Mesh, ASTM, Merck). Compounds 16-24 and 27 have been previously described Citation33Citation34Citation35Citation36.
Synthesis
Synthesis of N-(4,6-Dimethylpyridin-2-yl)-(1-benzylindole-3-yl) acetamide (25) (Method h)
A mixture of triphenylphosphine (6.94 g, 26.4 mmol), bromotrichloromethane (10.5 g, 52.8 mmol), (1-benzylindol-3-yl)acetic acid (7.00 g, 26.4 mmol) and 2-amino-4,6-dimethylpyridine (6.59 g, 52.8 mmol) in 150 mL of anhydrous THF was refluxed for 3 h. After filtration, the filtrate was concentrated in vacuum to dryness and chromatographed on silica gel eluting with dichloromethane/ethanol (98/2) to afford pure amide 25 in 60% yield. White powder; mp = 40–50°C; IR (KBr) ν cm− 1: 3240 (NH), 1650 (C = O); NMR 1H (DMSO-d6): δ ppm 2.26 (s, 3H, 4′-CH3), 2.38 (s, 3H, 6′-CH3), 3.84 (s, 2H, CH2), 5.42 (s, 2H, NCH2), 6.81 (s, 1H, H5′), 7.05 (dd, 1H, J = J′ = 7.60 Hz, H5), 7.13 (dd, 1H, J = J′ = 7.60 Hz, H6), 7.23-7.35 (m, 5H, Bn), 7.44-7.46 (m, 2H, H2, H7), 7.68 (d, 1H, J = 7.60 Hz, H4), 7.79 (s, 1H, H3′), 10.49 (s, 1H, NH).
Synthesis of N-(pyridin-2-yl)-(1-benzylindol-3-yl) acetamide (26) (Method g)
To a solution of (1-benzylindol-3-yl)acetic acid (2.28 g, 8.6 mmol) in 100 mL of dry dichloromethane were added 2-chloro-1-methylpyridinium iodide (2.2 g, 8.6 mmol), triethylamine (6 mL, 21.6 mmol) and 2-aminopyridine (0.85 g, 8.6 mmol). The mixture was refluxed for 2 h and after cooling washed with H2O, dried (Na2SO4), concentrated and chromatographed on silica gel eluting with dichloromethane/ethanol (97/3) to provide the amide 26 in 78% yield. White powder; mp = 120-121°C; IR(KBr) ν cm− 1: 3260 (NH), 1690 (C = O); NMR 1H (DMSO-d6): δ ppm 3.88 (s, 2H, CH2), 5.43 (s, 2H, NCH2), 7.03-7.16 (m, 3H, H5, H6, H4′), 7.23-7.35 (m, 5H, Bn), 7.45-7.47 (m, 2H, H2, H7), 7.68 (d, 1H, J = 7.60 Hz, H4), 7.78 (dd, 1H, J = J′ = 7.60 Hz, H5′), 8.11 (d, 1H, J = 8.40 Hz, H6′), 8.34 (d, 1H, J = 4.80 Hz, H3′), 10.64 (s, 1H, NH).
N-(4,6-Dimethylpyridin-2-yl)-[1-(4-fluorobenzyl)indol-3-yl] acetamide (28)
was prepared as described for compound 26. Yield: 73%; White powder; mp = 130–132°C; IR (KBr) ν cm− 1: 3260 (NH), 1675 (C = O); NMR 1H (DMSO-d6): δ ppm 2.26 (s, 3H, 4′-CH3), 2.38 (s, 3H, 6′-CH3), 3.84 (s, 2H, CH2), 5.41 (s, 2H, NCH2), 6.81 (s, 1H, H5'), 7.05 (dd, 1H, J = J′ = 7.60 Hz, H5), 7.14 (dd, 1H, J = 7.60 Hz, H6), 7.17 (dd, 2H, J = J′ = 8.80 Hz, Bn-H3, H5), 7.30 (dd, 2H, J = 5.60 Hz, J = 8.40 Hz, Bn-H2, H6), 7.45-7.47 (m, 2H, H2, H7), 7.67 (d, 1H, J = 7.60 Hz, H4), 7.78 (s, 1H, H3′), 10.50 (s, 1H, NH).
N-(Pyridin-2-yl)-[1-(4-fluorobenzyl)indol-3-yl] acetamide (29)
was prepared as described for compound 26. Yield: 73%; White powder; mp = 110–111°C; IR (KBr) ν cm− 1: 3280 (NH), 1715 (C = O); NMR 1H (DMSO-d6): δ ppm 3.87 (s, 2H, CH2), 5.42 (s, 2H, NCH2), 7.05 (dd, 1H, J = J′ = 7.60 Hz, H5), 7.10-7.19 (m, 4 H, H6, Bn-H3, H5, H4′), 7.30 (dd, 2H, J = 5.60 Hz, J = 7.60 Hz, Bn-H2, H6), 7.47-7.48 (m, 2H, H2, H7), 7.67 (d, 1H, J = 7.60 Hz, H4), 7.77 (dd, 1H, J = J′ = 7.60 Hz, H5′), 8.10 (d, 1H, J = 8.40 Hz, H6′), 8.34 (d, 1H, J = 4.00 Hz, H3′), 10.64 (s, 1H, NH).
N-(Pyridin-3-yl)-[1-(4-fluorobenzyl)indol-3-yl] acetamide (30)
was prepared as described for compound 26. Yield: 68%; White powder; mp = 144–145°C; IR (KBr) ν cm− 1: 3240 (NH), 1710 (C = O); NMR 1H (DMSO-d6): δ ppm 3.83 (s, 2H, CH2), 5.42 (s, 2H, NCH2), 7.06 (dd, 1H, J = J′ = 7.60 Hz, H5), 7.15 (dd, 1H, J = J′ = 7.60 Hz, H6), 7.17 (dd, 2H, J = J′ = 8.80 Hz, Bn-H3, H5), 7.31 (dd, 2H, J = 5.60 Hz, J = 8.80 Hz, Bn-H2, H6), 7.37 (dd, 1H, J = 4.40 Hz, J = 8.00 Hz, H5′), 7.47-7.49 (m, 2H, H2, H7), 7.65 (d, 1H, J = 7.60 Hz, H4), 8.09 (d, 1H, J = 8.00 Hz, H4′), 8.29 (d, 1H, J = 4.40 Hz, H6′), 8.80 (d, 1H, J = 2 Hz, H2′), 10.42 (s, 1H, NH).
Measurement of hyaluronidase activity
Stains-all assay
An enzyme solution of 100 U/mL hyaluronidase was prepared from 3110 U/mg hyaluronidase powder with 50 mM sodium phosphate buffer at pH 7.0. As substrate solution, 2 mg/mL hyaluronic acid was dissolved in water. All compounds (16-30) were prepared with DMSO to give stock solutions of 10 mM.
25 μM, 50 μM and 100 μM inhibitor concentration were obtained by adding inhibitor stock solution to the enzyme solution. The inhibitor/enzyme-solution was incubated for 1 h at 37°C. After incubation substrate solution, 0.2 M phosphate buffer and water were mixed and spotted onto a microplate. The assay was started by adding the same amount of enzyme/inhibitor-solution to the wells. While running, the plate was incubated at 37°C. To measure HA concentration, stains-all solution and water were added to the wells and the absorption was monitored at 650 nm by a microplate reader (Mithras LB940, Berthold, Germany). The activity of a positive control with pure DMSO instead of inhibitor solution was measured and set to 100%. The activity was calculated depending on the change in absorption using this equation:
A more detailed description of the assay procedure and used solutions has been published by us recently [Citation29].
For investigating the influence of NaCl and BSA concentrations on BTH activity, enzyme solutions of 100 U/ml hyaluronidase containing different amounts of NaCl and BSA were prepared and used in the same way as the pure hyaluronidase solution. Negative controls without enzyme were used to eliminate possible interactions of stains-all with BSA or NaCl.
Morgan-Elson assay
An enzyme solution of 800 U/mL hyaluronidase was prepared from 3110 U/mg hyaluronidase powder with formate buffer containing 0.1 M sodium formate and 0.1 M NaCl at pH 3.5. The compounds (16-30) were dissolved to 10 mM in DMSO as above. 5 mg/mL HA were dissolved in water to give the substrate solution. 25 μM, 50 μM and 100 μM inhibitor concentration were obtained by adding inhibitor stock solution to the enzyme solution. The inhibitor/enzyme-solution was incubated for one hour at 37°C.
After incubation 0.2 mg/mL BSA solution, incubation buffer, water and inhibitor/enzyme-solution were mixed and the assay was started by adding substrate solution. While running, the mixture was incubated at 37°C. For investigating the influence of different BSA concentrations on BTH activity at pH 3.5, solutions with different protein concentration were used in the same way as the original 0.2 mg/mL BSA solution.
To measure HA fragments concentration, several quota of the incubated solution were heated for 4.5 min at 100°C after addition of a KOH-solution. Subsequently, the solutions were transferred onto a microplate and stained using DMAB (dimethylaminobenzaldehyde).
The absorption was measured at 590 nm using a microplate reader (Mithras LB940, Berthold, Germany). The activity of a positive control with pure DMSO instead of inhibitor solution was set to 100%. The activity was also calculated using Equation (1). A more detailed description of the assay procedure and used solutions has been published by us recently [Citation29].
For both assays a negative control lacking hyaluronidase enzyme was measured. 6-Palmitoyl-L-ascorbic acid was used as a control compound in the same way as the other inhibitors.
For compound activity measurement at pH 7 with the Morgan-Elson assay a phosphate-citrate buffer containing 0.1M sodium citrate, 0.2 M sodium dihydrogenphosphate and 0.1 M NaCl was used in the same way as the formate buffer at pH 3.5.
Results and discussion
Chemistry
As previously described Citation33Citation34Citation35Citation36, (indol-3-yl)carboxamides and acetamides were synthesized from the corresponding activated carboxylic acids using 2-chloro-1-methylpyridinium iodide, the couple PPh3/BrCCl3, or phenyl dichlorophosphate, dicyclohexylcarbodiimide. N-substituted (indo-3-yl)carboxylic acids precursors were obtained from the commercially available (indo-3-yl)carboxylic or (indo-3-yl)acetic acids, after esterification, N-alkylation followed by an alkaline hydrolysis. The 5-bromo-(indol-3-yl)carboxylic acids 14-15 were prepared by oxidation of the corresponding carbaldehydes (Scheme ).
Scheme 1 Synthesis of indo-3-ylcarboxamides and acetamides 16-30. Reagents and conditions: (a) 1M HCl, EtOH, reflux; (b) NaH, DMF, CH3I or C2H5I, rt; (c) Cs2CO3, CH3CN, BnCl or 4F-BnCl, reflux; (d) 1) 2M NaOH, EtOH, reflux, 2) 2M HCl; (e) POCl3, DMF, 10 °C; (f) NaClO2, NaH2PO4, 2-methyl-2-butene, tBuOH, THF, rt; (g) 2-chloro-1-methylpyridinium iodide, THF, reflux; (h) PPh3, CBrCl3, THF, reflux; (i) phenyldichlorophosphate, CH2Cl2, rt; (j) DCC, THF, rt.
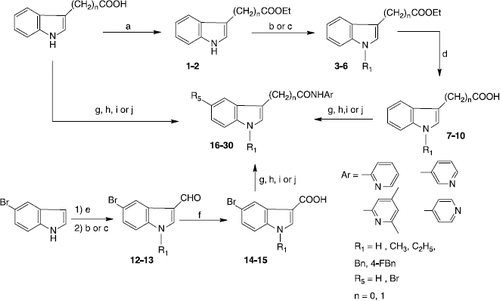
Hyaluronidase modulating effects at pH 7
Compounds 16-30 () were first tested at a concentration of 50 μM at pH 7. The assay at neutral pH is based on the cationic carbocyanine dye (l-ethyl-2-[3-(l-ethyl-naphtho[l,2-d]thiazolin-2-ylidene)-2-methylpropenyl]naphtha[1,2-d]thiazolium bromide, also known as “stains-all”. This compound forms blue colored complexes with long chains of HA. Reduced chain length of HA as obtained by hyaluronidase activity results in a reduced absorbance of the complex at the absorption maximum of 650 nm. A detailed description of this inhibitor assay has been published before [Citation29]. Compound 20 and 17 were by far the most active inhibitors of bovine testes hyaluronidase (BTH) with IC50 values of 46 μM and 78 μM respectively. These results are summarized in . Ascorbic acid palmitate (Vcpal), which is a well known inhibitor of hyaluronidases [Citation37], was used as a control substance. All compounds containing an acetamide chain were less active than compounds with a carboxamide chain. Among the carboxamide series N-methyl substituted compounds (16-18) showed very low activity unless the pyridine was additionally substituted with two methyl groups in meta positions (17) which made this part of the molecule more lipophilic. The addition of Br in position R3 seemed to have no effect as compounds 16 and 18 showed nearly the same amount of inhibition. In contrast, the introduction of a p-fluoro benzyl ring enhanced the inhibitory activity from 3% (18) up to 50% (20) at 50 μM. Interestingly, introducing both the p-fluroro benzyl ring and the additional substitution of the pyridine with two methyl groups decreased the activity from 50% (18) down to 17% (19). Exactly the same effect could be observed within the acetamide series but in less amount: Inhibitory activity decreased from 10% (29) to 5% (28) and from 9% (26) down to a complete loss of activity (25) when both substituents were combined. Probably the compounds are not allowed to get too much lipophilic in order to interact with the enzyme. Another explanation could be, that the free electron pair of the nitrogen and the electron rich p-fluoro-benzyl ring are interfering and pushing each other into a less advantageous position.
Table I. Compounds tested on hyaluronidase modulating activity at pH 7 and pH 3.5.
Table II. Hyaluronidase activity in the presence of compounds 16 – 30 in a concentration of 50 μM at pH 7 (stains-all assay) and pH 3.5 (Morgan-Elson assay).
Within the acetamide series the additional substitution of the pyridine with methyl groups in meta position did not influence the inhibitory activity (22, 24, 25, 28). As expected, the introduction of a benzyl ring or a p-fluoro benzyl ring elevated the inhibitory activity (26, 27, 29, 30) up to a maximum of 15%. In contrast to the carboxamide series the amount of inhibitory activity still was low, so we could assume the molecule did not fit the active site as good as before, probably due to the longer backbone and the shifted position of both the amide and the pyridine. If compound 30 was compared to compound 21, which also had a longer backbone and therefore a shifted position of the pyridine but not the amide, they showed the same inhibitory activity. This could be a hint, that the pyridine plays the more important role in fitting the active site than the position of the amide. These findings support the view of previous investigations Citation26Citation27Citation28Citation29 that benzimidazoles are promising structures for the development of hyaluronidase inhibitors and their full potential of inhibitory capabilities has not been tapped yet.
Hyaluronidase modulating effect at pH 3.5
Because the absorption of the stains-all complex with HA is reduced at pH values below 5 [Citation38], which definitely interferes with enzyme activity measurement, a Morgan-Elson assay [Citation39] based on staining of N-acetyl-D-glucosamine with dimethyl amino benzaldehyd (DMAB) was used for measuring at pH 3.5. Incubation of HA with mammalian hyaluronidases produces short chains with N-acetyl-D-glucosamine at the reducing end. If these sugars converts from the pyranoside into the furanoside form and are boiled under alkaline conditions, a Morgan-Elson reaction can take place. One or more of the resulting chromogens reacts with DMAB under acidic conditions [Citation40] and gives a red-colored product with a maximum in absorbance at 590 nm.
Pyridines in general show good water solubility, because the free electron pair of the nitrogen is able to interact with polar water molecules at neutral pH. At pH 3.5 the pyridine structure is protonated and therefore we expected a reduced solubility of the compounds in aqueous buffers for this pH. Actually nearly all benzyl ring containing compounds were found to be not completely soluble (19-21, 25-30). Although the effect on BTH activity was measured for all compounds, only the data of those compounds, which were completely soluble give a reliable picture on structure-activity relationship. All data obtained with the Morgan-Elson assay at pH 3.5 are summarized in . In contrast to our expectations, none of the compounds tested (except the control inhibitor Vcpal) showed any inhibitory activity on BTH. However, the indole acetamide derivatives in particular obviously had an activating effect on the degradation of HA. To verify that the activation was not an artifact caused by a reaction between the potential inhibitors and the solute DMSO, all compounds were measured in a control assay without enzyme. As expected, no influence on absorbance at 590 nm could be observed for any compound. Furthermore, in a cross control, the three most potent compounds in activating BTH at pH 3.5, compounds 22, 23 and 24, were also tested in a Morgan-Elson assay at pH 7 () in order to exclude that the activation was not an pH dependent phenomenon, but an assay depending phenomenon, because in the stains-all assay no activation of HA degradation could be observed at neutral pH. It turned out, that all also in the Morgan-Elson assay, the three compounds were weak inhibitors of HA degradation and their inhibitory potential was in the same order of magnitude as measured before. This indicates, that activation and inhibition is pH-depended and probably an effect of protonation. The nitrogen of the pyridine is the most basic one within the molecule and will be protonated and positively charged at pH 3.5. By comparison to pyridine derivatives with determined pKS values, a value of about 5 for our compounds can be assumed.
Table III. Inhibitory effect of compounds 22, 23 and 24 (50 μM) on hyaluronidase activity at pH 7 (Morgan-Elson assay).
This indicates, that the activating effect of compounds 22-24 on BTH is pH-depended and could probably be an effect of protonation. The nitrogen of the pyridine is the most basic one within the molecule and will most probably be protonated at pH 3.5, resulting in a positive charge. By structure comparison to pyridine derivatives with known pKa values, a pKa value of about 5 appeared to be expectable for our compounds.Interestingly, activation was much higher in the acetamide series than in the carboxamide series. One possible explanation could be a possible additional protonation of the indole structure, since the indole of the acetamides is expected to be much more basic than that of the carboxamides. In the acetamide group, the indole nitrogen is not part of a vinylogous amide and its free electron pair is less delocalized than the free electron pair in the correspondent carboxamides, leading to a higher pKa value. Therefore, these compounds could carry two positive charges at pH 3.5, as proposed in . An additional charge would then enhance the activating effect on HA degradation. Besides the acetamide backbone, a substitution with bromine at R3 (17, 18) seemed to increase activating effect, as compound 16 showed no activity in comparison to 17 and 18, which could be the result of a more basic indole. Additional methyl groups at the pyridine, identified as important for hyaluronidase inhibitory activity, had no effect. On the contrary, ethyl substitution at R1 had great effect on activation of HA degradation, since compound 24 showed the highest rate of activation and BTH activity raised up to 134% at 100 μM concentration of the compound. The concentration dependent increase in BTH activity was evaluated for compounds 22-24 and appeared to show logarithmic relationship ().
Figure 1 Change of the protonation state of compound 24 (N-(4,6-Dimethylpyridin-2yl)-(1-ethylindole-3-yl)acetamide) from pH 7 to pH 3.5.
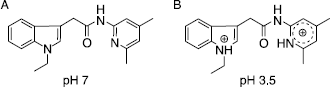
Figure 2 Concentration dependent activation of BTH by compounds 22 (▴), 23 (▪) and 24 (♦) in the Morgan-Elson assay at pH 3.5.
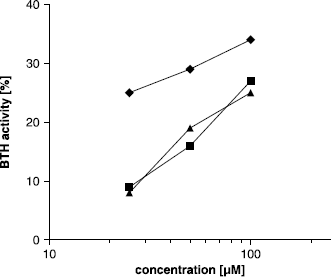
Inhibitors of BTH at pH 7, like compound 17, were not at the same time activators of the enzyme at pH 3.5. We assumed that the protonated form of the compounds could no longer be able to bind to the active site of BTH. Proteins like BSA, hemoglobin or transferrin [Citation24,Citation25], as well as smaller compounds like platinum complexes [Citation41], tranilast or monovalent salts [Citation42] have been reported to be activators of HA degradation as well in previous studies. To get a deeper insight on the activating effect observed with our compounds, the influence of BSA on activity of BTH was measured at pH 7 and pH 3.5 and compared to our most active compound 24. It is well known, that BSA forms complexes with HA [Citation25], resulting in an elevated HA degradation. Mainly two explanations have been suggested in the literature so far for this observation [Citation25,Citation43]. The first suggestion is, that hyaluronidases can bind hyaluronic acid nonspecifically too, leading to the formation of catalytically inactive complexes [Citation43]. This could explain the decrease in activity observed at high hyaluronic acid concentrations, which has been described [Citation44]. If BSA is added to a solution of hyaluronidase, it competes the binding of HAhis which results in reduced amount off bound and hence inactive hyaluronidase. The second explanation suggests a modifying of the complex structure of HA caused by binding of BSA. In previous studies, structure analysis revealed that HA chains are randomly coiled and contain entanglements [Citation45]. An opening of the random coil structure would make HA better accessible for the enzyme [Citation25]. Furthermore, HA is likely to include alternating folded and stretched parts, as can be derived from recently done molecular-dynamic simulations of theoretical polyelectrolytes in solution [Citation46]. It is to assume, that the stretched and unfolded form of HA is easier accessible to the enzyme and can be digested. If BSA binding increases the amount of unfolded HA, this could result in an increased activity of hyaluronidase. In our experiments, we observed high activation of BTH activity by BSA at pH 7 (), as expected by the preceding considerations. The concentration dependence of effect was almost linear. The highest activation measured in our test was 163% in comparison to the enzyme activity with no modulator added, obtained by a concentration of 1 g/l BSA. Higher concentrations of BSA did not yield another increase in BTH activity.
Figure 3 Activating effect of BSA on BTH activity measured by the stains-all assay at pH 7. Activity without BSA was set to 100%.
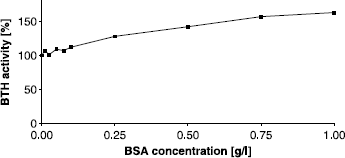
In the protocol of the Morgan-Elson assay at pH 3.5, as developed by our group [Citation29], BSA is added in a concentration of 0.44 g/l just before the digestion is started. In these experiments the influence of BSA was found to be critical, for enzyme activity and inhibitor effect, as well [Citation29]: if BSA was added together with potential inhibitors in one step, the inhibitory effect was completely abolished. On the other hand, omitting BSA completely from the assay resulted in very poor BTH activities. In the present experiments we tried to elucidate the effect of different BSA concentrations at pH 3.5 and performed the assay with concentrations ranging from 0 to 1 g/l (). As reported before, a complete lack of albumin resulted in a severe reduction of activity. In contrast to the stains-all assay at pH 7, addition of BSA had a much stronger effect in the Morgan Elson assay at pH3.5. The optimal concentration of BSA appeared to be 0.1 g/l with an activation of up more than 400% in comparison to the activity without albumin. These results are consistent with those reported earlier by Maingonnat et al. [Citation25], who found a four-fold activation of hyaluronidase activity after addition of 0.1 g/l BSA. To summarize, we observed an increase of BTH activity after addition of BSA in the stains-all assay at pH 7 of about 63% and in the Morgan-Elson assay at pH 3.5 of more than 400%. Due to its IEP of 4.8, BSA is expected to be positively charged at pH 3.5 and can interfere with the negatively charged HA much stronger than at pH 7. Furthermore HA is likely to be more twisted and folded at lower pH [Citation45,Citation46]. If we assume the effect of BSA is based on elevating the quota of stretched and unfolded HA, this could explain the higher amount of HA degradation activation we found at pH 3.5.
Figure 4 Activating effect of BSA on BTH activity at pH 3.5 (Morgan-Elson assay) without additional activator (▴) and after addition of compound 24 in a concentration of 100 μM (▪). BTH activity with 44 mg/L BSA, as used in our standard protocol of the Morgan-Elson assay, was set to 100%.
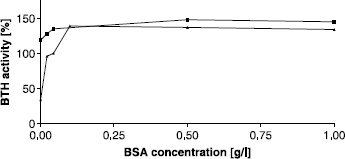
If the most active compound 24 was present in the assay at a concentration of 100 μM, BTH was active even without any BSA added (). It appeared that an indole compound carrying a basic pyridine, is able to replace BSA in its role to activate BTH. If the BSA concentration was increased in the presence of compound, the final maximum level of BTH activation was identical with that at higher concentrations of BSA, but without compound (). Almond et al. [Citation46] recently proposed a new three dimensional structure of an HA octamer (HA8) in solution. We used this structure as a model for a HA chain in order to analyze possible interactions between our most activating compound 24 and HA. Interestingly electrostatic interactions were found to be presumable between the negatively charged carboxylate groups of the HA chain and both positively charged nitrogen atoms in our compound (). The compound obviously is able to bridge every disaccharide unit by binding to glucuronic acid, resulting in one bonded activator molecule per tetrasaccharide. It is conceivable that these interactions could be able to open randomly coiled structures of HA and also to stabilize and elevate the amount of unfolded parts and finally making HA better accessible to BTH for degradation.
Figure 5 Interactions between the protonated form of compound 24 (ball-and-stick model) and HA (stick model) mediated by saltbridges. Only hydrogens in hydroxyl groups or connected to nitrogen are shown. Building of the compound and visualization of both molecules were performed using the program SYBYL from Tripos (www.tripos.com). HA8 molecule was taken from the RCSB protein data bank (PDB accession code: 2BVK) [Citation46].
![Figure 5 Interactions between the protonated form of compound 24 (ball-and-stick model) and HA (stick model) mediated by saltbridges. Only hydrogens in hydroxyl groups or connected to nitrogen are shown. Building of the compound and visualization of both molecules were performed using the program SYBYL from Tripos (www.tripos.com). HA8 molecule was taken from the RCSB protein data bank (PDB accession code: 2BVK) [Citation46].](/cms/asset/cf3eb757-62f2-41e4-94e9-decfd315715b/ienz_a_320982_f0006_b.gif)
Therefore we conclude that soluble (indol-3-yl)acetamides carrying a pyridine structure as N-(4,6-Dimethylpyridin-2yl)-(1-ethylindole-3-yl)acetamide, our compound 24, N-(4,6-Dimethylpyridin-2-yl)-(1-benzylindole-3-yl) acetamide (25) and N-(pyridin-2-yl)-(1-benzylindol-3-yl) acetamide (26) are able to bind HA in the protonated form at pH 3.5 via electrostatic interaction between the two positively charged nitrogens and the negatively charged carboxylate groups of HA. This binding leads to a more stretched and less folded HA polymer which is better accessible and cleavable by hyaluronidases. Because the indole nitrogen of the (indol-3-yl)carboxamide derivatives is not as basic as of the acetamide derivatives it could be not protonated and as a consequence indole carboxamides would be much weaker activators of HA degradation.
A lack of hyaluronidase activity has been identified for different sort of mucopolysaccharidosis [Citation18] and some cancer cells Citation19Citation20Citation21 in combination with an increased level of HA. In these cases, drugs which elevate HA digestion might be very useful. NaCl or other salts cannot be used as treatment agents without disturbing salt homeostasis in the human body. Hyaluronidase activating proteins like BSA or even hyaluronidases themselves are also difficult drugs, because they might cause allergic reactions and show a poor bioavailability. The compounds we presented here are non-proteinogenic and could lead to drugs which can be used to elevate hyaluronidase activity. Tranilast, an antiallergenic substance, has been described as a hyaluronidase activating drug in the literature before [Citation42]. It is an acidic compound and therefore carries no positive chargings at lower pH. In contrast to our compound N-(4,6-Dimethylpyridin-2yl)-(1-ethylindole-3-yl)acetamide (24), which elevated BTH activity up to 125% at a concentration of 26 μM, a very high concentration of about 500 μM tranilast was needed to reach the same amount of activation at pH 3.5. In summary, it can be assumed, that (indol-3-yl)acetamide derivatives carrying a pyridine structure can be used as a starting point for further development of both, hyaluronidase inhibiting as well as activating agents, at different pH.
Acknowledgements
We would like to thank Birte Schmitz for excellent technical assistance in the visualization of the interaction of the molecules.
Declaration of interest: The authors report no conflicts of interest. The authors alone are responsible for the content and writing of the paper.
References
- JY Lee, and AP Spicer. (2000). Curr Opin Cell Biol 12:581–586.
- PA Martin-DeLeon. (2006). Mol Cell Endocrinol 250:114–121.
- DC West, and S Kumar. (1989). Exp Cell Res 183:179–196.
- BP Toole. (1997). J Intern Med 242:35–40.
- WYJ Chen, and G Abatangelo. (1999). Wound Repair Regen 7:79–89.
- O Oksala, T Salo, R Tammi, L Hakkinen, M Jalkanen, P Inki, and HJ Larjava. (1995). Histochem Cytochem 43:125–135.
- PH Weigel, VC Hascall, and M Tammi. (1997). J Biol Chem 272:13997–14000.
- G Kreil. (1995). Protein Sci 4:1666–1669.
- A Roth, J Mollenhauer, A Wagner, R Fuhrmann, A Straub, RA Venbrocks, P Petrow, R Bräuer, H Schubert, J Ozegowski, G Peschel, PJ Müller, and RW Kinne. (2005). Arthritis Res Ther 7:R677–R686.
- P Bertrand, MN Courel, C Maingonnat, F Jardin, H Tilly, and C Bastard. (2005). Int J Cancer 113:207–212.
- JL Kovar, MA Johnson, WM Volcheck, J Chen, and MA Simpson. (2006). Am J Pathol 169:1415–1426.
- D Liu, E Pearlman, E Diaconu, K Guo, H Mori, T Haqqi, S Markowitz, J Wilson, and MS Sy. (1996). Proc Natl Acad Sci USA 93:7832–7837.
- J Lesley, R Hyman, N English, JB Catterall, and GA Turner. (1997). Glycoconj J 14:611–622.
- JX Tan, GS Ren, G Tu, XT Li, XY Wang, and L Ran. (2006). Ai Zheng 25:844–848.
- JT Posey, MS Soloway, S Ekici, M Sofer, F Civantos, RC Duncan, and VB Lokeshwar. (2003). Cancer Res 63:2638–2644.
- VB Lokeshwar, WH Cerwinka, and BL Lokeshwar. (2005). Cancer Res 65:2243–2250.
- DA Godin, PC Fitzpatrick, AB Scandurro, PC Belafsky, BA Woodworth, RG Amedee, DJ Beech, and BS Beckman. (2000). Arch Otolaryngol Head Neck Surg 126:402–404.
- B Triggs-Raine, TJ Salo, H Zhang, BA Wicklow, and MR Natowicz. (1999). Proc Natl Acad Sci USA 96:6296–6300.
- W Knudson. (1996). Am J Pathol 148:1721–1726.
- S Shuster, GI Frost, AB Csoka, B Formby, and R Stern. (2002). Int J Cancer 102:192–197.
- RK Boregowda, HN Appaiah, M Siddaiah, SB Kumarswamy, S Sunila, KN Thimmaiah, KK Mortha, BP Toole, and SD Banerjee. (2006). J Carcinogen 5:36–44.
- I Muckenschnabel, G Bernhardt, T Spruß, and A Buschauer. (1996). Cancer Chemother Pharmacol 38:88–94.
- G Baumgartner, C Gomar-Hoss, L Sakr, E Ulsperger, and C Wogritsch. (1998). Cancer Lett 131:85–99.
- A Mandal, and AK Bhattacharyya. (1995). Hum Reprod 10:1745–1750.
- C Maingonnat, R Victor, P Bertrand, MN Courel, R Maunoury, and B Delpech. (1999). Anal Biochem 268:30–34.
- A Botzki, S Salmen, G Bernhardt, A Buschauer, and S Dove. (2005). QSAR Comb Sci 24:458–469.
- DJ Rigden, A Botzki, M Nukui, RB Mewbourne, E Lamani, S Braun, E von Angerer, G Bernhardt, S Dove, A Buschauer, and MJ Jedrzejas. (2006). Glycobiology 16:757–765.
- S Olgen, A Kaeßler, D Nebioglu, and J Jose. (2007). Chem Biol Drug Des 70:547–551.
- A Kaessler, O Algul, and J Jose. (2007). Lett Drug Des Discov 4:562–569.
- CH Yang, and PN Srivastava. (1975). J Biol Chem 250:79–83.
- G Frost, and R Stern. (1997). Anal Biochem 251:263–269.
- R Stern, and MJ Jedrzejas. (2006). Chem Rev 106:818–839.
- F Fouchard, C Menciu, M Duflos, and G Le Baut. (1999). Arzneim-Forsch/Drug Res 49:96–105.
- M Duflos, MR Nourrisson, J Brelet, J Courant, G Le Baut, N Grimaud, and JY Petit. (2001). Eur J Med Chem 36:545–553.
- F Fouchard, P Marchand, G Le Baut, P Emig, and B Nickel. (2001). Arzneim-Forsch/Drug Res 51:814–824.
- A Breteche, M Duflos, A Dassonville, MR Nourrisson, J Brelet, G Le Baut, N Grimaud, and JY Petit. (2002). J Enz Inhib Med Chem 17:415–424.
- A Botzki, DJ Rigden, S Braun, M Nukui, S Salmen, J Hoechstetter, G Bernhardt, S Dove, MJ Jedrzejas, and A Buschauer. (2004). J Biol Chem 279:45990–45997.
- RE Kay, ER Walwick, and CK Gifford. (1964). J Phys Chem 68:1896–1906.
- JL Reissig, JL Storminger, and LF Leloir. (1955). J Biol Chem 217:959–966.
- I Muckenschnabel, G Bernhardt, T Spruss, B Dietl, and A Buschauer. (1998). Cancer Lett 131:13–20.
- H Kakegawa, H Matsumoto, and T Satoh. (1987). J Pharmacobio-Dyn 10:153.
- H Kakegawa, H Matsumoto, and T Satoh. (1985). Chem Pharm Bull 33:642–646.
- B Deschrevel, H Lenormand, F Tranchepain, N Levasseur, T Astériou, and JC Vincent. (2008). Matrix Biol 27:242–253.
- T Astériou, JC Vincent, F Tranchepain, and B Deschrevel. (2006). Matrix Biol 25:166–174.
- ER Morris, DA Rees, and EJ Welsh. (1980). J Mol Biol 138:383–400.
- HJ Limbach, and C Holm. (2003). J Phys Chem B. 107:8041–8055.
- A Almond, PL Deangelis, and CD Blundell. (2006). J Mol Biol 358:1256–1269.