Abstract
A series of novel β-lactam containing compounds are described as antiproliferative agents and potential selective modulators of the oestrogen receptor. The purpose of the study is to evaluate the antiproliferative effects of these compounds on human MCF-7 and MDA MB-231 breast cancer cells. The compounds are designed to contain three aryl ring substituents arranged on the heterocyclic azetidin-2-one (β-lactam), thus providing conformationally restrained analogues of the triarylethylene arrangement exemplified in the tamoxifen type structure. The compounds demonstrated potency in antiproliferative assays against MCF-7 human breast cancer cell line at low micromolar to nanomolar concentrations with low cytotoxicity and moderate binding affinity to the oestrogen receptor. The effect of a number of aryl and amine functional group substitutions on the antiproliferative activity of the β-lactam products was explored and a brief computational structure–activity relationship investigation with molecular simulation was investigated.
Introduction
The oestrogen receptor has proven to be an important target in breast cancer over the last 30 years [Citation1]. The use of the Selective Estrogen Receptor Modulator (SERM) tamoxifen 1 for the treatment and prevention of breast cancer has changed therapeutics Citation2Citation3Citation4. The SERM raloxifene, approved for the treatment of osteoporosis, lacks the increased risk for endometrial cancer associated with the use of tamoxifen 1 and has been evaluated for the prevention of breast cancer [Citation5,Citation6]. Aromatase inhibitors such as the non-steroidal agents letrozole and anastrole [Citation7], and the steroidal agent exemestane are reported to be more efficacious than tamoxifen as first-line therapy and are useful for second-line therapy and against tamoxifen-resistant disease. The aromatase inhibitors and the pure antioestrogen fulvestrant [Citation8] is also effective as second line therapy against advanced breast cancer in patients who develop resistance to tamoxifen treatment Citation9Citation10Citation11Citation12.
The oestrogen receptor (comprising of two isoforms ERα and ERβ) is a ligand activated transcript factor which mediates the physiological effects of the oestrogen hormones. The ER isoforms have been characterised and found to have different tissue distribution patterns. ERα is predominantly found in the uterus, bone, cardiovascular tissue and liver and is the predominant ER expressed in breast cancer [Citation13]. ERβ is expressed in many tissues including prostate, breast, vascular endothelium and ovary. However, ERα and ERβ demonstrate distinct activation properties and may play different roles in gene expression [Citation14,Citation15].
The oestrogen receptors are widely distributed in the body tissues and are regarded as attractive therapeutic agents for diseases such as osteoporosis and breast cancer. Design of ligands which can modulate the activity of the ER in a tissue selective manner continues to attract interest due to the regulating effects of the ER on many diseased states. Tamoxifen 1 is an established oestrogen receptor antagonist and is a useful endocrine drug in the treatment of ER positive breast cancer.
The binding modes of several ligands (e.g. 4-hydroxytamoxifen 2 and raloxifene 3) in the ligand binding domain (LBD) of the ERα and ERβ has been determined by X-ray crystallographic studies and have shown that the interaction of SERMs with the ER-LBD results in induction of key conformational changes to Helix 12 Citation16Citation17Citation18Citation19Citation20. Many different modifications of the tamoxifen molecular design have been investigated in an effort to improve its effectiveness as an antioestrogen and to reduce the associated side effects Citation21Citation22Citation23Citation24. Due to the increased risk of endometrial cancer with the use of tamoxifen, a rigid structure in place of the ethylene double bond to prevent E/Z isomerisation is found to be effective. Many alternative novel non-isomerisable scaffolds for oestrogen receptor modulators have been discovered [Citation25,Citation26]. Examples of SERMs which contain rigid cyclic scaffolds are illustrated in , e.g. tetrahydronaphthalene (lasofoxifene) 4 [Citation27], pyrazole 5 [Citation28], tetrahydroisoquinoline 6 [Citation29], benzopyran EM-652 7 [Citation30] and dihydrobenzoxathiin 8 [Citation26]
We now report the design and synthesis of non-isomerisable SERMs based on the four membered β-lactam ring. The β-lactam ring was chosen for this purpose mainly because it can be easily modified to incorporate the triaryl structural features necessary for ER ligand activity. One disadvantage of the β-lactam ring as a drug is its chemical instability; however most of its degradation products are non toxic and appropriate chemical modifications to improve stability can be considered. Although the primary biological targets of β-lactam antibiotics are the transpeptidase penicillin binding proteins, the cytotoxic potential of some β-lactam containing compounds have been reported Citation31Citation32Citation33Citation34Citation35 together with activity of some β-lactams as inhibitors of cholesterol absorption [Citation36], prostate specific antigen [Citation37] and tryptase enzyme [Citation38].
In this work, a number of different β-lactam compounds were synthesised having the common core structure substituted with aromatic rings at the N-1 and C-4 positions to replace the rings B and C of tamoxifen and raloxifene. Directly attached to the C-3 position is a carbon bearing a secondary alcohol group which can also be oxidized to the carbonyl function. A variety of different aryl groups are introduced at this carbon site and will mimic Ring A of the tamoxifen and raloxifene structures. The basic side chain required for antagonist interaction with Asp351 of the ER LBD is accommodated on this aryl ring. The general features of the β-lactam target compounds selected for synthesis are illustrated in , structural types I and II.
Materials and methods
Chemistry
IR spectra were recorded as thin films on NaCl plates on a Perkin-Elmer Paragon 100 FT-IR spectrometer. 1H and 13C NMR spectra were obtained on a Bruker Avance DPX 400 instrument at 20°C, 400.13 MHz for 1H spectra, 100.61 MHz for 13C spectra, in either CDCl3 (internal standard tetramethylsilane) or CD3OD. Low resolution mass spectra were run on a Hewlett-Packard 5973 MSD GC–MS system in an electron impact mode, while high resolution accurate mass determinations for all final target compounds were obtained on a Micromass Time of Flight mass spectrometer (TOF) equipped with electrospray ionization (ES) interface operated in the positive ion mode at the High Resolution Mass Spectrometry Laboratory in the Department of Chemistry, Trinity College Dublin. Flash chromatography was carried out using standard silica gel 60 (230–400 mesh) obtained from Merck. All products isolated were homogenous on TLC. HLPC analysis was carried out using a Waters reverse-phase instrument with Phenomonex 250 × 4.6 mm, 4 μm column operating with the following conditions: flow rate: 1 ml/min; solvent system: methanol/water; 4:1; UV detector, 254 nM. Compounds 9a [Citation39], 9b [Citation40], 10a [Citation41], 10b [Citation41], 11a [Citation42,Citation43] were prepared as previously reported.
General preparation of azetidin-2-ones 11a–l
A solution of the appropriate azetidin-2-one 10a–b (2.5 mmol) in dry THF (25 ml) was stirred at − 78°C under a N2 atmosphere. Lithium diisopropylamide (2 M, 5 mmol, 2.5 ml) was added quickly and the solution was stirred at − 78°C for 5 min. A solution of the appropriate aldehyde or ketone (3.75 mmol) in dry THF (12.5 ml) was added to the reaction mixture at the same temperature. The mixture was stirred at − 78°C for 30 min and then poured into a saturated sodium chloride solution (50 ml). EtOAc was added and the organic layer separated and dried (Na2SO4) and the solvent removed in vacuo to afford the crude product which was purified by silica gel flash column chromatography (CH2Cl2/AcOEt, 4:1).
3-[Hydroxy-(2-hydroxyphenyl)-methyl]-1,4-bis-(4-methoxyphenyl)-azetidin-2-one 11b
Preparation as above from 10a (0.9 mmol, 0.250 g) and salicylaldehyde (1.33 mmol, 0.14 ml). Yield 41%, Orange gel, IR νmax (film) cm− 1: 1729.9 cm− 1 (C = O), 3362.1 cm− 1 (OH). 1H NMR (400 MHz, CDCl3) δ 3.56 (dd, 1H, J = 2.52 Hz, J = 5 Hz), 3.72 (s, 3H), 3.74 (s, 3H), 4.79 (d, 1H, J = 2 Hz), 5.31 (d, 1H, J = 7.52 Hz), 6.71–6.76 (m, 4H), 6.81–6.96 (m, 4H), 7.08–7.24 (m, 4H); HRMS: Found 428.1494; C24H23NO5 requires 428.1474.
3-[Hydroxy-(3-hydroxyphenyl)-methyl]-1,4-bis-(4-methoxyphenyl)-azetidin-2-one 11c
Preparation as above from 10a, (0.9 mmol, 0.250 g) and 3-hydroxybenzaldehyde (1.33 mmol, 0.162 g). Yield 42%, Orange gel, IR νmax (film) cm− 1: 1725.5 cm− 1 (C = O), 3383.9 (OH). 1H NMR (400 MHz, CDCl3) δ 3.34 (dd, 1H, J = 3.51 Hz), 3.69 (3H, s), 3.72 (s, 3H), 4.7 (d, 0.175H, J = 2 Hz), 4.96, (d, 0.75H, J = 7.04 Hz), 5.10 (d, 0.25 H, J = 2 Hz), 5.18–5.20 (m, 0.25 H), 6.68–6.77 (m, 5H), 6.81–7.19 (m, 7H). HRMS: Found 428.1465; C24H23NO5 requires 428.1474.
3-[Hydroxy-(4-hydroxyphenyl)-methyl]-1,4-bis-(4-methoxyphenyl)-azetidin-2-one 11d
Preparation as above from 10a (1.5 mmol, 0.426 g) and 4-hydroxybenzaldehyde (2.25. mmol, 0.27 g). Yield 20%, Orange oil, IR νmax (film) cm− 1: 1731.7 cm− 1 (C = O), 3384.0 cm− 1 (OH). 1H NMR (400 MHz, CDCl3) δ 3.35 (dd, 0.38H, J = 2.04 Hz), 3.41 (dd, 0.62H, J = 3.4 Hz), 3.69 (s, 3H), 3.72 (s, 3H), 4.72 (d, 0.62H, J = 2.04 Hz), 4.98 (d, 0.62H, J = 6.16 Hz), 5.11 (s, 0.38H), 5.15 (d, 0.38H, J = 4.08 Hz), 6.72–6.79 (m, 6H), 6.96–7.00 (m, 2H), 7.11–7.23 (m, 4H). HRMS: Found 428.1494; C24H23NO5 requires 428.1474.
3-[1-Hydroxy-1-(3-hydroxyphenyl)-ethyl]-1,4-bis-(4-methoxyphenyl)-azetidin-2-one 11e
Preparation as above from 10a (0.9 mmol, 0.250 g) and 3-hydroxyacetophenone (1.33 mmol, 0.18 g). Yield 41%, Yellow oil, IR νmax (film) cm− 1: 1727.2 cm− 1 (C = O), 3379.9 cm− 1 (OH). 1H NMR (400 MHz, CDCl3) δ 1.64 (s, 2.55H), 1.73 (s, 0.45H), 3.33 (d, 0.15H, J = 2.52 Hz), 3.48 (d, 0.85H, J = 2 Hz), 3.69 (s, 3H), 3.76 (s, 3H), 4.76 (d, 0.15H, J = 2.48 Hz), 4.87 (d, 0.85H, J = 2 Hz), 6.67–6.72, (m, 4H), 6.83 (d, 2H, J = 8.52 Hz), 6.94 (d, 1H, J = 8 Hz), 7.11–7.18 (m, 5H). HRMS: Found 442.1630; C25H25NO5 requires 442.1631.
3-[1-Hydroxy-1-(4-hydroxyphenyl)-ethyl]-1,4-bis-(4-methoxyphenyl)-azetidin-2-one 11f
Preparation as above from 10a (0.636 mmol, 0.180 g) and 4-hydroxyacetophenone (0.95 mmol, 0.130 g). Yield 30%, Yellow gel, IR νmax (film) cm− 1: 1724.6 cm− 1 (C = O), 3412.5 cm− 1 (OH). 1H NMR (400 MHz, CDCl3) δ 1.70 (s, 2.4H), 1.79 (s, 0.6H), 3.39 (d, 0.2H, J = 2.48 Hz), 3.54 (d, 0.8H, J = 2.52 Hz), 3.74 (s, 3H), 3.80 (s, 3H), 4.75 (d, 0.2H, J = 2.52 Hz), 4.90 (d, 0.8H, J = 2.48 Hz), 6.72–6.77, (m, 4H), 6.86 (d, 2H, J = 8.2 Hz), 7.17–7.23 (m, 4H), 7.32 (d, 2H, J = 8.88 Hz). HRMS: Found 442.1637; C25H25NO5 requires 442.1630.
3-[Hydroxy-(3-hydroxyphenyl)-phenylmethyl]-1,4-bis-(4-methoxyphenyl)-azetidin-2-one 11g
Preparation as above from 10a (0.9 mmol, 0.250 g) and 3-hydroxybenzophenone (1.33 mmol, 0.263 g). Yield 41%, Yellow oil, IR νmax (film) cm− 1: 1726.0 (C = O), 3418.7 (OH). 1H NMR (400 MHz, CDCl3) δ 3.69 (s, 3H), 3.72 (s, 3H), 4.07 (d, 1H, J = 2.0 Hz), 4.85 (d, 1H, J = 2.52 Hz), 6.58–6.69 (m, 4H), 6.71–6.74 (m, 3H), 6.92–7.07 (m, 2H), 7.17–7.20 (m, 3H), 7.26–7.32 (m, 5H). HRMS: Found 504.1497; C30H27NO5 requires 504.1787.
3-[Hydroxy-(4-hydroxyphenyl)-phenylmethyl]-1,4-bis-(4-methoxyphenyl)-azetidin-2-one 11h
Preparation as above from 10a, (0.62 mmol, 0.175 g) and 4-hydroxybenzophenone (0.924 mmol, 0.183 g). Yield 18%, Yellow oil, IR νmax (film) cm− 1: 1720.5 (C = O), 3427.2 (OH). 1H NMR (400 MHz, CDCl3) δ 3.71 (s, 3H), 3.76 (s, 3H), 4.14 (s, 0.96H), 4.18 (s, 0.04H), 4.92 (s, 0.96H), 4.97, (s, 0.04H), 6.75–6.80 (m, 8H), 7.19–7.29 (m, 4H), 7.45–7.48 (m, 4H). HRMS: Found 504.1799; C30H27NO5 requires 504.1787.
3-[1-Hydroxy-3-(4-hydroxyphenyl)-1-methylallyl]-1,4-bis-(4-methoxyphenyl)-azetidin-2-one 11i
Preparation as above from 10a, (0.9 mmol, 0.250 g) and 4-hydroxybenzylideneacetone (1.33 mmol, 0.215 g). Yield 57%, Orange oil, IR νmax (film) cm− 1: 1720.5 (C = O), 3317.1(OH). 1H NMR (400 MHz, CDCl3) δ 1.47 (s, 2H), 1.55 (s, 1H), 1.99 (s, 0.65H), 2.12 (s, 0.35H), 3.17 (d, 0.35H, J = 2.48 Hz), 3.24 (d, 0.65H, J = 2.52 Hz), 3.63 (s, 3H), 3.68 (s, 1H), 3.71 (s, 2H), 4.85 (d, 0.35H, J = 2.0 Hz), 4.93 (d, 0.65H, J = 2.0 Hz), 5.96 (d, 0.35H, J = 16.04 Hz), 6.23 (d, 0.65H, J = 16.08 Hz), 6.48–6.57 (m, 1H), 6.61–6.69 (m, 4H), 6.77–6.82 (m, 2H), 7.07–7.09 (m, 2H), 7.10–7.15 (m, 2H), 7.30 (d, 2H, J = 8.56 Hz). HRMS: Found 468.1791; C27H27NO5 requires 468.1787.
3-[Hydroxy-(2-hydroxynaphthalen-1-yl)-methyl]-1,4-bis-(4-methoxyphenyl)-azetidin-2-one 11j
Preparation as above from 10a (0.838 mmol, 0.250 g) and 2-hydroxy-1-naphthaldehyde (1.325 mmol, 0.228 g). Eluant CH2Cl2:MeOH, 19:1; Yield 28%, Yellow gel, IR νmax (KBr) cm− 1: 1739.0 (C = O), 3308.9 (OH). 1H NMR (400 MHz, CDCl3) δ 3.58 (d, 1H, J = 2.4 Hz,), 3.65 (s, 3H), 3.68 (s, 3H), 4.04 (d, 1 H, J = 2 Hz), 4.10 (d, 1H, J = 2.08 Hz), 6.64–6.68 (m, 6H), 6.89 (q, 1H, J = 2 Hz), 7.02 (d, 2H, J = 9.2 Hz), 7.10–7.11 (m, 2H), δ 7.48 (q, 1H, J = 6.3 Hz (av.)), δ 7. 46 (d, 1H, J = 7.6 Hz).
3-Hydroxy-(4-hydroxynaphthalen-1-yl)-methyl]-1,4-bis-(4-methoxyphenyl)-azetidin-2-one 11k
Preparation as above from 10a (0.838 mmol, 0.250 g) and 4-hydroxy-1-naphthaldehyde (1.325 mmol, 0.228 g). Eluant CH2Cl2:MeOH, 19:1; Yield 30%, Red oil, IR νmax (film) cm− 1: 1743.3 (C = O), 3365.9 (OH). 1H NMR (400 MHz, CDCl3) δ 3.52 (dd, 1H, J = 4.11 Hz av.), 3.74 (s, 3H), 3.80 (s, 3H), 4.72 (d, 0.5H, J = 2.04 Hz), 4.80 (d, 0.5H, J = 6.8 Hz), 4.92 (q, 0.5H, J = 2.74 Hz (av.)), 5.04 (d, 0.5H, J = 6.16 Hz), 6.35 (d, 1H, J = 8.84 Hz), 6.56 (d, 1H, J = 9.56 Hz), 6.69–6.92 (m, 6H), 7.15–7.24 (m, 6H). HRMS: Found 478.1635: C28H25NO5 requires: 478.1630 (M+ + Na).
General procedure for alkylation of phenolic β-lactams
To a solution of the appropriate β-lactam 11a–k (10 mmol) in dry acetone (100 ml) was added anhydrous potassium carbonate (0.16 mol, 22 g) and the mixture stirred gently for 10 min under a N2 atmosphere. The corresponding aminoalkyl halide (40 mmol, 5.78 g) was then added and the reaction was heated under reflux until reaction was complete by TLC. The solution was filtered and the solvent was removed under reduced pressure and the residue purified using silica gel flash column chromatography, eluant: CH2Cl2:EtOAc, 4:1.
3-([4-(2-Dimethylaminoethoxy)-phenyl]-hydroxymethyl)-1,4-diphenylazetidin-2-one 12a
Preparation as above from 11a (0.23 mmol, 80 mg) and 2-(dimethylamino)-ethylchloride hydrochloride (0.92 mmol, 0.133 g). Yield 44%, Orange crystals, m.p. 168°C. IR νmax (film) cm− 1: 1749.2 (C = O), 3450.9 (OH). 1H NMR (400 MHz, CDCl3) δ 2.32 (s, 6H), 2.73–2.76 (m, 2H), 3.41–3.47 (m, 1H), 4.02–4.06 (m, 2H), 4.81 (d, 0.5H, J = 2.52 Hz), 5.09 (d, 0.5H, J = 7.00 Hz), δ 5.19 (d, 0.5H, J = 2.48 Hz), 5.31 (d, 0.5H, J = 4.04 Hz), 6.85–6.94 (m, 2H), 7.03–7.06 (m, 3H), 7.21–7.32 (m, 8H), 7.37 (d, 1H, J = 8.8 Hz). 13C NMR (100 MHz, CDCl3): δ 45.43, 55.48, 57.26, 57.75, 65.68, 65.68, 66.51, 68.93, 72.00, 114.16, 114.25, 116.63, 116.71, 123.38, 123.51, 125.26, 128.59, 132.63, 133.29, 136.76, 137.32, 157.94, 165.25. HRMS: Found 417.2198; C26H28N2O3 requires 417.2178.
3-([4-(2-Dimethylaminoethoxy)-phenyl]-hydroxymethyl)-1,4-bis-(4-methoxyphenyl)-azetidin-2-one 12b
Preparation as above from 11d (0.247 mmol, 100 mg) and 2-(dimethylamino)ethylchloride hydrochloride (0.988mmol, 0.142 g). Yield 48%, Orange gel, IR νmax (film) cm− 1: 1729.2 (C = O), 3338.4 (OH). 1H NMR (400 MHz, CDCl3) δ 2.36 (s, 6H), 2.77 (s, 2H), 3.37–3.41 (m, 1H), 3.73 (s, 3H), 3.76 (s, 3H), 4.06 (s, 2H), 5.05–5.10 (m, 1H), 5.27 (d, 1H, J = 11.6 Hz), δ 6.76–6.97 (m, 8H), 7.20–7.50 (m, 4H). 13C NMR (100 MHz, CDCl3): δ 45.22, 54.75, 54.97, 55.19, 57.01, 57.57, 65.22, 65.71, 66.47, 68.75, 71.86, 113.76, 114.15, 117.98–118.05, 126.37, 129.19, 130.59, 132.91, 133.55, 155.46, 155.53, 158.17, 159.04, 165.13. HRMS: Found 477.2413; C28H32N2O5 requires 477.2389.
3-(Hydroxy-[4-((2-pyrrolidin-1-yl)-ethoxy)-phenyl]-methyl)-1,4-diphenylazetidin-2-one 12c
Preparation as above from 11a (0.26 mmol, 90 mg) and 1-(2-chloroethyl)-pyrrolidine hydrochloride (1.04 mmol, 0.180 g). Yield 50%, Orange gel, IR νmax (film) cm− 1: 1750 (C = O, β-lactam); 1H NMR (400 MHz, CDCl3) δ 1.81 (s, 4H), 2.62 (s, 4H), 2.89 (m, 2H), 3.39–3.47 (m, 1H), 4.05–4.19 (m, 2H), 4.80 (d, 0.5H, J = 2.16 Hz), 5.07 (d, 0.5H, J = 6.6 Hz), 5.19 (d, 0.5H, J = 2.2 Hz), 5.28–5.29 (d, 0.5H, J = 3.68 Hz), 6.84–6.89 (m, 2H), 7.03–7.39 (m, 12H). 13C NMR (100 MHz, CDCl3): δ 23.00, 54.29, 54.54, 55.54, 65.52, 66.06, 72.10, 114.20, 114.29, 116.70, 116.63, 125.26, 128.57, 133.17, 137.04, 155.48, 165.41. HRMS: Found 443.2341; C28H30N2O3 requires 443.2335.
3-(Hydroxy-[4-((2-pyrrolidin-1-yl)-ethoxy)-phenyl]-methyl)-1,4-bis-(4-methoxyphenyl)-azetidin-2-one 12d
Preparation as above from 11d (0.247 mmol, 100 mg) and 1-(2-chloroethyl)-pyrrolidine hydrochloride (0.988 mmol, 0.168 g); Yield 45%, Orange gel, IR νmax(film) cm− 1 1736.0 (C = O), 3371.3 (OH). 1H NMR (400 MHz, CDCl3) δ 1.82 (s, 4H), 2.62 (s, 4H), 2.88–2.90 (m, 2H), 3.36–3.40 (m, 1H), 3.73, 3.74, 3.76 (3s, 6H), 4.05–4.10 (m, 2H), 4.69 (d, 0.5H, J = 2.92 Hz), 5.04 (d, 0.5H, J = 7.32 Hz), 5.08 (d, 0.5H, J = 2.16 Hz), 5.27 (d, 0.5H, J = 3.68 Hz), 6.74–6.80 (m, 4H), 6.85–6.89 (m, 2H), 6.96 (d, 2H, J = 8.8 Hz), 7.19–7.37 (m, 4H). 13C NMR (100 MHz, CDCl3): δ 22.99, 54.32, 54.59, 54.75, 54.79, 54.97, 57.02, 66.58, 72.05, 113.76, 114.39, 117.95, 118.14, 126.31, 129.27, 130.67, 130.76, 132.70, 155.52, 157.89, 158.37, 165.01. HRMS: Found 503.2532; C30H34N2O5 requires 503.2546.
3-([4-(2-Diethylaminoethoxy)-phenyl]-hydroxymethyl)-1,4-diphenylazetidin-2-one 12e
Preparation as above from 11a (0.29 mmol, 100 mg) and 2-diethylaminoethylchloride hydrochloride (1.16 mmol, 0.2 g). Yield 40%, Yellow gel, IR νmax (KBr) cm− 1: 1750.1 (C = O), 3414.3 (OH). 1H NMR (400 MHz, CDCl3) δ 1.06 (t, 6H, J = 7.16 Hz), 2.62 (q, 4H, J = 7.16 Hz), 2.86 (t, 2H, J = 6.5 Hz), 3.40 (q, 0.4H, J = 2.17 Hz), 3.44 (q, 0.6H, J = 3.19 Hz), 4.03–4.06 (m, 2H), 4.80 (d, 0.6H, J = 2.04 Hz), 5.09 (d, 0.6H, J = 6.84 Hz), 5.18 (d, 0.4H, J = 2.72 Hz), 5.31 (d, 0.4H, J = 3.44 Hz), 6.67–6.91 (m, 2H), 7.04–7.08 (m, 3H), 7.22–7.40 (m, 9H). 13C NMR (100 MHz, CDCl3): δ 11.16, 11.18, 47.27, 47.29, 51.15, 55.48, 57.25, 66.05, 65.68, 66.52, 68.83, 71.99, 114.09, 116.72, 123.38, 123.52, 125.26, 125.37, 125.26, 126.30, 127.48, 128.59, 133.17, 136.75, 137.33, 157.95, 158.46, 165.32. HRMS: Found 445.2494; C28H32N2O3 requires 445.2491.
3-([4-(2-Diethylaminoethoxy)-phenyl]-hydroxymethyl)-1,4-bis-(4-methoxyphenyl)-azetidin-2-one 12f
Preparation as above from 11d (0.247 mmol, 100 mg) and 2-diethylaminoethylchloride hydrochloride (0.988 mmol, 0.170 g). Purified by flash column chromatography, eluant: CH2Cl2:MeOH (3:1) afforded the product as an orange oil, Yield 47%. IR νmax (film) cm− 1: 1738.4 cm− 1 (C = O), 3389.3 (OH). 1H NMR (400 MHz, CDCl3) δ 1.16 (t, 6H, J = 7.2 Hz), 2.82 (d, 4H, J = 7.2 Hz), 3.05–3.06 (m, 2H), 3.34–3.44 (m, 1H), 3.72 (s, 3H), 3.76 (s, 3H), 4.14–4.17 (m, 2H), 4.77 (d, 0.5H, J = 2.04 Hz), 5.07 (d, 0.5H, J = 6.8 Hz), 5.11 (d, 0.5H, J = 2.08 Hz), 5.26 (d, 0.5H, J = 4.08 Hz), 6.74–6.86 (m, 6H), 6.97–7.04 (m, 2H), 7.19–7.23 (m, 2H), 7.28 (d, 1H, J = 9.56 Hz), 7.37 (d, 1H, J = 8.2 Hz). 13C NMR (100 MHz, CDCl3): δ 9.99, 47.06, 50.78, 54.78, 55.32, 57.02, 57.91, 64.54, 65.62, 66.37, 68.95, 71.71, 113.77, 114.09, 117.98, 118.04, 126.55, 127.76, 128.60, 130.64, 133.24, 133.80, 155.48, 155.54, 157.26, 159.09, 164.83, 165.00. HRMS: Found 505.2480; C30H36N2O5 requires 505.2624.
3-(Hydroxy-[4-((2-piperidin-1-yl)-ethoxy)-phenylmethyl)-1,4-diphenylazetidin-2-one 12g
Preparation as above from 11a (0.29 mmol, 100 mg) and 2-chloroethylpiperidine hydrochloride (1.16 mmol, 0.210 g). Purified by flash column chromatography, (eluant: MeOH); Yield 47%, Orange gel, IR νmax (film) cm− 1: 1747.4 cm− 1 (C = O), 3417.6 (OH). 1H NMR (400 MHz, CDCl3) δ 1.47 (s, 2H), 1.62 (d, 4H), 2.52 (s, 4H), 2.76–2.79 (m, 2H), 3.41–3.47, (m, 1H), 4.08–4.11 (m, 2H), 4.83 (d, 0.5H, J = 2.52 Hz), 5.10 (d, 0.5H, J = 7.04 Hz), 5.20 (d, 0.5H, J = 2.48 Hz), 5.31 (d, J = 4.52 Hz, 0.5H), 6.86–7.61 (m, 14H). 13C NMR (100 MHz, CDCl3): δ 23.72, 25.43, 29.26, 54.66, 55.52, 57.26, 57.44, δ 65.55, 68.90, 71.96, 114.19, 114.40, 116.30, 116.70, 123.50, 125.28, 128.66, 131.33, 137.05, 158.43, 160.70. HRMS: Found 457.2494; C29H32N2O3, requires 457.2491.
3-(Hydroxy-[4-((2-piperidin-1-yl)-ethoxy)-phenyl]-methyl)-1,4-bis-(4-methoxyphenyl)-azetidin-2-one 12h
Preparation as above from 11d (0.247 mmol, 100 mg) and 1-(2-chloroethyl)-piperidine hydrochloride (0.988 mmol, 0.182 g). Purified by flash column chromatography, (eluant: CH2Cl2:MeOH, 3:1); Yield 50%, Orange gel, IR νmax (film) cm− 1: 1736.6 cm− 1 (C = O), 3436.0 (OH). 1H NMR (400 MHz, CDCl3) δ 1.48 (s, 2H), 1.67–1.70 (m, 4H), 2.62 (s, 4H), 2.84–2.87 (m, 2H), 3.36–3.42 (m, 1H), 3.74–3.78 (m, 6H), 4.12 (t, 2H, J = 5.8 Hz), 4.77 (d, 0.5H, J = 2.04 Hz), 5.08 (d, 0.5H, J = 6.16 Hz), 5.11 (d, 0.5H, J = 2.04 Hz), 5.28 (d, 0.5H, J = 4.12 Hz), 6.76–6.87 (m, 6H), 6.98–7.04 (m, 2H), 7.21–7.25 (m, 2H), 7.28 (t, 1H, J = 6.48 Hz), 7.37 (d, 1H, J = 8.2 Hz). 13C NMR (100 MHz, CDCl3): δ 23.27, 24.80, 25.15, 54.41, 54.53, 54.77, 54.98, 55.32, 57.04, 57.10, 64.92, 65.61, 66.38, 69.09, 71.95, 113.77, 114.22, 117.97, 118.03, 126.45, 127.69, 128.59, 133.42, 155.48, 159.09, 164.70, 164.99. HRMS: Found 517.2708; C31H36N2O5 requires 517.2702.
3-(Hydroxy-[4-((2-morpholin-4-yl)-ethoxy)-phenyl]-methyl)-1,4-diphenylazetidin-2-one 12i
Preparation as above from 11a (0.29 mmol, 100 mg) and 2-chloroethylpiperidine hydrochloride (1.16 mmol, 0.216 g). Purified by flash column chromatography, eluant: CH2Cl2:EtOAc (1:1); Yield 47%, Yellow crystals; m.p. 120°C. IR νmax (KBr) cm− 1 1739.0 cm− 1 (C = O), 2956.0 (OH). 1H NMR (400 MHz, CDCl3) δ 2.57 (s, 4H), 2.79 (m, 2H), 3.38–3.48 (m, 1H), 3.71–3.73 (m, 4H), 4.07–4.13, (m, 2H), 4.81 (d, 0.65H, J = 2.52 Hz), 5.07 (d, 0.65H, J = 6.52 Hz), 5.18 (d, 0.35H, J = 2.52 Hz), 5.26 (s, 0.35H), 6.86 (q, 2H, J = 4.02 Hz), 7.03–7.09 (m, 3H), 7.21–7.30 (m, 9H). 13C NMR (100 MHz, CDCl3): δ 53.04, 53.59, 57.11, 57.20, 65.25, 65.75, 66.53, 68.71, 71.66, 114.14, 114.22, 116.62, 116.69, 123.40, 123.49, 125.31, 128.56, 132.89, 133.58, 136.83, 137.33, 157.74, 158.19, 165.32, 165.35. HRMS: Found 459.2242; C28H30N2O4, requires 459.2284.
3-(Hydroxy-[4-((2-morpholin-4-yl)-ethoxy)-pheny]-methyl)-1,4-bis-(4-methoxyphenyl)-azetidin-2-one 12j
Preparation as above from 11d (0.247 mmol, 100 mg) and 4-(2-chloroethyl)-morpholine hydrochloride (0.988 mmol, 0.184 g) purified using flash column chromatography, (eluant: CH2Cl2:MeOH, 19:1); Yield 50%, Yellow oil, IR νmax (film) cm− 1: 1741.3 cm− 1 (C = O), 3371.3 (OH). 1H NMR (400 MHz, CDCl3) δ 2.58–2.59 (m, 4H), 2.75–2.81 (m, 2H), 3.37–3.42 (m, 1H), 3.75–3.77 (m, 10H), 4.09 (t, 2H, J = 5.76 Hz), 4.73 (d, 0.5H, J = 2.52 Hz), 5.07 (d, 0.5H, J = 7.04 Hz), 5.10 (d, 0.5H, J = 2 Hz), 5.28 (d, 0.5H, J = 4 Hz), 6.75–6.81 (m, 4H), 6.86–6.89 (m, 2H), 6.98–7.02 (m, 2H), 7.22–7.26 (m, 2H), 7.29–7.32 (m, 1H), 7.38–7.41 (m, 1H). 13C NMR (100 MHz, CDCl3): δ 40.19, 53.06, 54.76, 55.23, 56.98, 57.14, 59.68, 65.32, 65.71, 66.34, 66.48, 68.80, 71.81, 113.78, 114.21, 117.94, 117.96, 126.41, 127.67, 128.68, 130.70, 132.94, 133.63, 155.44, 151.51, 157.73, 159.07, 164.80, 164.87. HRMS: Found 519.2488; C30H34N2O6, requires 519.2417.
3-(Hydroxy-(4-[2-(1-methylpyrrolidin-2-yl)-ethoxy]-phenyl)-methyl)-1,4-diphenyl azetidin-2-one 12k
Preparation as above from 11a (0.290 mmol, 100 mg) and 2-(2-chloroethyl)-1-methylpyrroldine hydrochloride (1.16 mmol, 0.214 g) followed by purification with column chromatography, (eluant: CH2Cl2:MeOH, 3:1); Yield 39%, Green gel, IR νmax (film) cm− 1: 1743.6 (C = O), 3394.0 (OH). 1H NMR (400 MHz, CDCl3) δ 1.98–2.20 (m, 2H), 2.22–2.27 (m, 1H), 2.31–2.52 (m, 4H), 2.81 (s, 3H), 2.89 (m, 2H), 3.43 (dd, 1H, J = 2.53 Hz), 4.94 (d, 0.75H, J = 2.8 Hz), 5.14 (d, 0.75H, J = 5.6 Hz), 5.21 (d, 0.25H, J = 2.4 Hz), 5.29 (s, 0.25H), 6.84–6.86 (m, 2H), 7.03–7.10 (m, 3H), 7.15–7.33 (m, 8H), 7.41–7.43 (m, 1H). 13C NMR (100 MHz, CDCl3): δ 18.54, 18.68, 24.68, 28.74, 32.04, 38.86, 49.95, 50.05, 55.54, 55.71, 63.28, 63.86, 65.86, 66.02, 66.43, 68.88, 70.63, 113.85, 116.61, 116.65, 123.41, 125.45, 128.57, 133.88, 134.32, 137.06, 137.27, 155.50, 155.77, 157.18, 157.41, 165.23, 165.36. HRMS Found 457.2468; C29H32N2O3, requires 457.2491.
3-(Hydroxy-[4-[2-(1-methylpyrrolidin-2-yl)-ethoxy]-phenyl]-methyl)-1,4-bis-(4-methoxyphenyl)-azetidin-2-one 12l
Preparation as above from 11d (0.247 mmol, 100 mg) and 2-(2-chloroethyl)-1-methylpyrrolidine hydrochloride (0.988 mmol, 0.182 g) followed by purification using column chromatography, (eluant: CH2Cl2:MeOH, 3:1); Yield 50%, Orange oil, IR νmax (film) cm− 1 1735.6 cm− 1 (C = O), 3426.4 (OH). 1H NMR (400 MHz, CDCl3) δ 1.97–2.26 (m, 6H), 2.42–2.45 (m, 1H), 2.73 (s, 3H), 3.05–3.29 (m, 2H), 3.37 (dd, 1H, J = 5.45 Hz), 3.73–3.77 (m, 6H), 3.94–4.14 (m, 2H), 4.66 (s, 0.63H), 5.27 (d, 0.37H, J = 4.08 Hz), 5.09–5.12 (m, 1H), 6.76–6.87 (m, 6H), 7.00–7.07 (m, 2H), 7.20–7.24 (m, 2H), 7.28–7.36 (m, 1H), 7.40 (d, 1H, J = 8.2 Hz). 13C NMR (100 MHz, CDCl3): δ 19.22, 21.19, 28.59, 31.38, 44.58, 53.02, 54.79, 54.98, 55.39, 55.46, 55.76, 56.80, 64.04, 65.59, 65.70, 66.35, 68.95, 71.28, 71.31, 113.78, 113.93, 115.58, 118.02, 126.75, 127.83, 130.58, 133.54, 134.28, 155.47–159.11, 164.78, 164.84. HRMS: Found 517.2709; C31H36N2O5 requires 517.2702
3-(Hydroxy-[2-(2-(pyrrolidin-1-yl)-ethoxy)-phenyl]-methyl)-1,4-bis-(4-methoxyphenyl)-azetidin-2-one 12m
Preparation as above from 11b (0.247 mmol, 100 mg) and 1-(2-chloroethyl)-pyrrolidine hydrochloride (0.988 mmol, 0.168 g) followed by purification using column chromatography, (eluant: CH2Cl2:MeOH, 9:1); Yield 16%, Pale orange oil, IR νmax (film) cm− 1: 1742.3 cm− 1 (C = O), 3247.9 (OH). 1H NMR (400 MHz, CDCl3) δ 1.76–1.82 (m, 4H), 2.56–2.58 (m, 4H), 2.82–2.85 (m, 2H), 3.59–3.61 (m, 1H), 3.71–3.73 (m, 6H), 4.12–4.15 (m, 2H), 4.81 (d, 0.85 H, J = 2.0 Hz), 5.10 (q, 0.15 H, J = 5.26 Hz), 5.34 (d, 0.85 H, J = 6.52 Hz), 5.48 (d, 0.15 H, J = 4.52 Hz), 6.75–6.82 (m, 4H), 6.87–7.04 (m, 4H), 7.21–7.28 (m, 4H). 13C NMR (100 MHz, CDCl3): δ 22.86, 53.64, 54.99, 55.18, 57.57, 64.92, 65.03, 67.87, 114.18, 114.33, 118.29, 118.40, 121.57, 126.73, 127.12, 127.47, 128.56, 129.12, 155.48, 159.09, 165.05, 165.12. HRMS: Found 503.2560; C30H34N2O5 requires 503.2546.
3-(Hydroxy-[3-(2-(pyrrolidin-1-yl)-ethoxy)-phenyl]-methyl)-1,4-bis-(4-methoxyphenyl)-azetidin-2-one 12n
Preparation as above from 11c (0.161 mmol, 65 mg) and 1-(2-chloroethyl)-pyrrolidine hydrochloride (0.988 mmol, 0.168 g) followed by purification using column chromatography, (eluant: CH2Cl2:MeOH, 9:1); Yield 63%, Pale orange oil, IR νmax (film) cm− 1: 1736.4 (C = O), 3430.0 (OH). 1H NMR (400 MHz, CDCl3) δ 1.87 (s, 4H), 2.78 (s, 4H), 2.99–3.02 (m, 2H), 3.39 (dd, 1H, J = 3.17 Hz), 3.74 (s, 3H), 3.74 (s, 3H), 4.16 (d, 2H, J = 4.8 Hz), 4.75 (d, 1H, J = 2.04 Hz), 5.07 (d, 1H, J = 7.48 Hz), 6.72–6.68 (m, 5H), 6.98–7.28 (m, 7H). 13C NMR (100 MHz, CDCl3): δ 22.87, 50.19, 52.99, 54.15, 54.80, 56.96, 65.55, 65.69, 71.93, 105.73, 112.20, 114.31, 117.78, 118.88, 126.66, 128.55, 129.14, 129.22, 142.26, 155.57, 158.23, 159.06, 165.01. HRMS: Found 503.2542; C30H34N2O5 requires 503.2546.
3-(1-Hydroxy-[3-(2-(pyrrolidin-1-yl)-ethoxy)-phenyl]-ethyl)-1,4-bis-(4-methoxyphenyl)-azetidin-2-one 12o
Preparation as above from 11e (0.179 mmol, 75 mg) and 1-(2-chloroethyl)-pyrrolidine hydrochloride (0.716 mmol, 0.122 g) as described in the general preparation followed by purification using column chromatography, (eluant: CH2Cl2: MeOH, 9:1); Yield 22%, Orange gel, IR νmax (film) cm− 1: 1736.4 (C = O), 3356.0 (OH). 1H NMR (400 MHz, CDCl3) δ 1.73 (s, 3H), 1.94 (s, 4H), 2.96 (s, 4H), 3.13–3.17 (m, 2H), 3.40 (d, 0.2H, J = 2.72 Hz), 3.49 (d, 0.8H, J = 2.72 Hz), 3.71 (s, 3H), 3.79 (s, 3H), 4.25–4.29 (m, 2H), 4.74 (d, 0.2H, J = 2.72 Hz), 4.91 (d, 0.8H, J = 2.72 Hz), 6.69–6.89 (m, 6H), 7.09 (d, 1H, J = 8.16 Hz), 7.15–7.30 (m, 5H). 13C NMR (100 MHz, CDCl3): δ 22.77, 28.25, 53.19, 54.82, 55.36, 57.22, 64.69, 70.32, 71.55, 111.79, 113.60, 114.42, 118.10, 118.45, 127.38, 128.91, 129.10, 129.42, 140.70, 158.91, 165.15. HRMS: Found 517.2723; C31H36N2O5 requires 517.2702.
3-(1-Hydroxy-[4-(2-(pyrrolidin-1-yl)-ethoxy)-phenyl]-ethyl)-1,4-bis-(4-methoxyphenyl)-azetidin-2-one 12p
Preparation as above from 11f (0.18 mmol, 75 mg) and 1-(2-chloroethyl)-pyrrolidine hydrochloride (0.716 mmol, 0.122 g) followed by purification using column chromatography, (eluant: CH2Cl2: MeOH, 9:1); Yield 21%, Orange oil, IR νmax (film) cm− 1: 1734.6 (C = O), 3420.5 (OH). 1H NMR (400 MHz, CDCl3) δ 1.71 (s, 2.4H), 1.79 (s, 0.60H), 1.90 (s, 4H), 2.73 (s, 4H), 2.92–2.96 (m, 2H), 3.39 (d, 0.2H, J = 2.52 Hz), 3.54 (d, 0.8H, J = 2.48 Hz), 3.74 (s, 3H), 3.80 (s, 3H), 4.76 (d, 0.2H, J = 2.52 Hz), 4.90 (d, 0.8H, J = 2.48 Hz), 6.74–6.79, (m, 4H), 6.84 (d, 2H, J = 8 Hz), 7.13–7.20 (m, 4H), 7.32 (d, 2H, J = 8.88 Hz). 13C NMR (100 MHz, CDCl3): δ 23.12, 27.25, 54.55, 55.12, 54.86, 56.36, 57.22, 65.56, 69.32, 72.16, 113.80, 114.07, 115.56, 115.86, 118.12, 118.24, 125.73, 126.12, 126.56, 126.93, 128.96, 130.20, 136.70, 154.94, 155.65, 160.13, 164.78. HRMS: Found 517.2719; C31H36N2O5 requires 517.2702.
3-(4-Hydroxyphenyl-[3-(2-(pyrrolidin-1-yl)-ethoxy)phenyl]-methyl)-1,4-bis-(4-methoxyphenyl)-azetidin-2-one 12q
Preparation as above from 11g (0.135 mmol, 65 mg) and 1-(2-chloroethyl)-pyrrolidine hydrochloride (0.54 mmol, 0.09 g) followed by purification using column chromatography, (eluant: CH2Cl2: MeOH, 9:1); Yield 49%, Orange gel, IR νmax (film) cm− 1: 1738.3 (C = O), 3421.6 (OH). 1H NMR (400 MHz, CDCl3) δ 1.86 (s, H), 2.72–2.76 (m, 4H), 2.94–2.99 (m, 2H), 3.74 (s, 3H), 3.76 (s, 3H), 4.07 (d, 1H, J = 2.08 Hz), 4.10–4.15 (m, 2H), 4.83 (d, 1H, J = 2.04 Hz), 6.62–6.85 (m, 7H), 7.17–7.22 (m, 4H), 7.28–7.36 (m, 6H). 13C NMR (100 MHz, CDCl3): δ 22.86, 22.94, 54.06, 54.17, 54.27, 54.71, 54.96, 56.31, 65.05, 68.67, 113.46, 113.73, 118.05, 119.63, 125.55, 126.58, 128.90, 128.58, 144.27, 155.44, 157.74, 158.76, 163.89. HRMS: Found 579.2845; C36H38N2O5 requires 579.2859.
3-(Hydroxyphenyl-[4-(2-(pyrrolidin-1-yl)-ethoxy)-phenyl]-methyl)-1,4-bis-(4-methoxyphenyl)-azetidin-2-one 12r
Preparation as above from 11h (0.105 mmol, 51 mg) and 1-(2-chloroethyl)-pyrrolidine hydrochloride (0.42 mmol, 0.071 g) followed by purification using column chromatography, (eluant: CH2Cl2: EtOAc,1:1); Yield 30%, Orange oil, IR νmax (film) cm− 1: 1738.0 (C = O), 3437.7 (OH). 1H NMR (400 MHz, CDCl3) δ 1.81 (s, 4H), 2.66 (s, 4H), 2.92 (m, 2H), 3.74 (s, 6H), 4.21 (m, 2H), 4.22 (q, 1H, J = 6 Hz), 4.83 (d, 1H, J = 2.4 Hz), 6.57 (d, 2H, J = 8.4 Hz), 6.66 (d, 2H, J = 8.8 Hz), 6.75 (d, 2H, J = 8.8 Hz), 6.83 (d, 2H, J = 8.8 Hz), 7.20 (d, 2H, J = 8.8 Hz), 7.26–7.35 (m, 4H), 7.48 (d, 2H, J = 8.8 Hz), 7.78 (dd, 1H, J = 10.9 Hz). 13C NMR (100 MHz, CDCl3): δ 22.92, 54.23, 54.42, 54.72, 54.97, 56.28, 66.10, 68.89, 113.52, 113.65, 113.75, 118.04, 125.59, 126.51, 126.59, 127.72, 128.32, 129.30, 129.70, 136.86, 155.44, 157.75, 163.99. HRMS: Found 579.2872; C36H38N2O5 requires 579.2859.
3-(1-Hydroxy-1-methyl-3-[4-(2-(pyrrolidin-1-yl)-ethoxy)-phenyl]-allyl)-1,4-bis-(4-methoxyphenyl)-azetidin-2-one 12s
Preparation as above from 11i (0.225 mmol, 100 mg) and 1-(2-chloroethyl)-pyrrolidine hydrochloride (0.90 mmol, 0.153 g) followed by purification using column chromatography, (eluant: CH2Cl2: EtOAc, 4:1); Yield 41%, Orange oil, IR νmax (film) cm− 1: 1738.1 cm− 1 (C = O), 3428.4 (OH). 1H NMR (400 MHz, CDCl3) δ 1.56 (s, 1.5H), 1.64 (s, 1.5H), 1.85 (s, 4H), 2.18 (bs, 0.5H), 2.21 (bs, 0.5H), 2.73 (s, 4H), 2.98–3.02 (m, 2H), 3.21 (s, 0.5H), 3.29 (s, 0.5H), 3.73 (s, 3H), 3.80 (s, 3H), 4.14–4.19 (m, 2H), 4.92 (s, 0.5H), 4.94 (s, 0.5H), 6.06 (d, 0.5H, J = 15.72 Hz), 6.34 (d, 0.5H, J = 15.68 Hz), 6.59–6.63 (m, 1H), 6.75–6.95 (m, 6H), 7.16–7.50 (m, 6H). 13C NMR (100 MHz, CDCl3): δ 22.91, 26.96, 54.08, 54.85, 54.96, 56.22, 56.47, 65.82, 69.21, 71.68, 113.75, 114.56, 117.99, 124.67, 126.83, 127.01, 127.33, 127.46, 128.18, 129.55, 142.81, 155.40, 160.04. HRMS: Found 543.2845; C33H38N2O5 requires 543.2859.
3-(Hydroxy-[2-((2-pyrrolidin-1-yl)-ethoxy)-naphthalen-1-yl]-methyl)-1,4-bis-(4-methoxyphenyl)-azetidin-2-one 12t
Preparation as above from 11k (0.178 mmol, 83 mg) and 1-(2-chloroethyl)-pyrrolidine (0.711 mmol, 0.121 g) followed by purification using column chromatography, (eluant: CH2Cl2: MeOH 3:1); Yield 41%, Yellow crystals, m.p. 208–212°C. IR νmax (KBr) cm− 1: 1739.1 (C = O), 3437.7 (OH). 1H NMR (400 MHz, CDCl3) δ 1.73 (s, 4H), 2.45 (s, 4H), 2.75 (s, 2H), 3.64 (d, 1H, J = 2.04 Hz), 3.87–3.89 (m, 6H), 3.90–4.01 (m, 2H), 4.03 (d, 1H, J = 3.4 Hz), 4.10 (d, 1H, J = 2.08 Hz), 4.11 (s, 2H), 6.64–6.92 (m, 6H), 7.08–7.12 (m, 3H), 7.16–7.20 (m, 1H), 7.42–7.47 (m, 1H), 7.56–7.79 (m, 3H). 13C NMR (100 MHz, CDCl3): δ 22.73, 53.73, 53.99, 54.71, 54.91, 56.23, 65.55, 66.43, 79.88, 113.61, 113.65, 117.98, 120.47, 124.40, 126.49, 128.54, 130.41, 131.38, 139.62, 155.44, 158.58, 159.84, 164.28. HRMS: Found 553.2699; C34H36N2O5 requires 553.2702.
3-(Hydroxy-[4-((2-pyrrolidin-1-yl)-ethoxy)-napthalen-1-yl]-methyl)-1,4-bis-(4-methoxyphenyl)-azetidin-2-one 12u
Preparation as above from 11l (0.25 mmol, 114 mg) and 1-(2-chloroethyl)-pyrrolidine (1 mmol, 0.171 g) followed by purification using column chromatography, (eluant: CH2Cl2:MeOH 3:1); Yield 35%, Yellow oil, IR νmax (film) cm− 1: 1740.3 cm− 1 (C = O), 3306.7 (OH). 1H NMR (400 MHz, CDCl3) δ 1.81 (s, 4H), 2.74 (s, 4H), 3.04 (s, 2H), 3.54 (dd, 1H, J = 4.11 Hz av), 3.74–3.80 (m, 6H), 4.22 (s, 2H), 4.72 (d, 0.5H, J = 2.04 Hz), 4.80 (d, 0.5H, J = 6.8 Hz), 4.93 (q, 0.5H, J = 2.74 Hz), 5.04 (d, 0.5H, J = 6.16 Hz), 6.36 (d, 1H, J = 8.84 Hz), 6.57 (d, 1H, J = 9.56 Hz), 6.69–6.92 (m, 6H), 7.15–7.24 (m, 6H). 13C NMR (100 MHz, CDCl3): δ 22.99, 54.29, 54.44, 54.69, 54.97, 57.52, 64.60, 66.60, 68.20, 103.40, 113.74, 113.97, 118.01, 121.84, 122.25, 122.34, 124.54, 124.89, 125.15, 125.36, 128.78, 129.20, 132.81, 141.17, 155.89, 158.56, 159.24, 163.84, 164.38. HRMS: Found 553.2714; C34H36N2O5 requires 553.2702.
General preparation for oxidation of secondary alcohols 13a–e
A solution of the appropriate alcohol (15 mmol, 1.5 equiv.) in CH2Cl2 (20 ml) was added to a suspension of pyridinium chlorochromate (PCC, 10 mmol, 2.114 g) in CH2Cl2 (15 ml) and the mixture was stirred for 2 h. The reaction mixture was then diluted anhydrous ether (100 ml). The solvent was decanted and the black residue was further washed with ether until the entire product was extracted. The solvent was then removed in vacuo.
1,4-Bis-(4-methoxyphenyl)-3-[4-((2-morpholin-4-yl)-ethoxy)-benzoyl]-azetidin-2-one 13a
Preparation as above from 12j (0.251 mmol, 130 mg) followed by purification with flash column chromatography (eluant: CH2Cl2); Yield 35%, Yellow oil, IR νmax (film) cm− 1: 1745.9 cm− 1 (C = O). 1H NMR (400 MHz, CDCl3) δ 2.60 (s, 4H), 2.83–2.86 (m, 2H), 3.76 (s, 3H), 3.77 (s, 3H), 3.82 (s, 4H), 4.19–4.22 (m, 2H), 4.75 (d, 1H, J = 2 Hz), 5.67 (d, 1H, J = 2 Hz), 6.80 (d, 2H, J = 9.04 Hz), 6.92 (d, 2H, J = 8.52 Hz), 6.99 (d, 2H, J = 8.52 Hz), 7.25–7.28 (m, 2H), δ 7.38 (d, 2H, J = 8.52 Hz), 8.10 (d, 2H, J = 5.52 Hz). 13C NMR (100 MHz, CDCl3): δ 53.46, 54.88, 54.97, 55.19, 56.83, 66.04, 68.14, 113.84, 114.16, 118.05, 127.15, 130.42, 131.32, 155.75, 159.44, 159.55, 162.61, 188.85. HRMS: Found 517.2344; C30H32N2O6 requires 517.2339
3-[4-(2-Dimethylaminoethoxy)-benzoyl]-1,4-bis-(4-methoxyphenyl)-azetidin-2-one 13b
Preparation as above from 12b (0.267 mmol, 127 mg) followed by purification with flash column chromatography (eluant: CH2Cl2); Yield 20%, Orange oil, IR νmax (film) cm− 1: 1748.2 cm− 1 (C = O). 1H NMR (400 MHz, CDCl3) δ 2.47 (s, 6H), 2.91 (s, 2H), 3.76 (s, 3H), 3.82 (s, 3H), 4.24 (s, 2H), 4.76 (bs, 1H), 5.68 (bs, 1H), 6.80–7.02 (m, 6H), 7.21–7.38 (m, 4H), 8.11 (s, 2H).
4-Bis-(4-methoxyphenyl)-3-[4-(2-pyrrolidin-1-yl-ethoxy)benzoyl]-azetidin-2-one 13c
Preparation as above from 12d (0.177 mmol, 89 mg) followed by purification with flash column chromatography (eluant CH2Cl2); Yield 35%, Orange oil, IR νmax (film) cm− 1: 1743.6 (C = O), 1740.0 (C = O). 1H NMR (400 MHz, CDCl3) δ 1.85 (s, 4H), 2.69 (s, 4H), 2.97 (s, 2H), 3.75 (s, 3H), 3.81 (s, 3H), 4.22 (s, 2H), 4.76 (bs, 1H), 5.67 (bs, 1H), 6.80 (d, 2H, J = 8.16 Hz), 6.92 (d, 2H, J = 8.2 Hz), 6.70 (d, 2H, J = 6.84 Hz), 7.26 (d, 2H, J = 8.2 Hz), 7.28–7.38 (m, 2H), 8.09 (d, 2H, J = 7.52 Hz). 13C NMR (100 MHz, CDCl3): δ 23.00, 54.26, 54.89, 54.97, 55.23, 66.69, 68.11, 113.84, 114.15, 118.05, 127.15, 131.29, 155.72, 159.42, 162.92, 188.89. HRMS: Found 501.2376; C30H32N2O5 requires 501.2389.
3-[4-(2-Diethylaminoethoxy)-benzoyl]-1,4-bis-(4-methoxyphenyl)-azetidin-2-one 13d
Preparation as above from 12f (0.196 mmol, 99 mg) followed by purification with flash column chromatography (eluant: CH2Cl2); Yield 20%, Yellow oil, IR νmax (film) cm− 1: 1733.6 cm− 1 (C = O). 1H NMR (400 MHz, CDCl3) δ 1.14 (s, 6H), 2.75 (s, 4H), 2.99 (s, 2H), 3.75 (s, 3H), 3.81 (s, 3H), 4.20 (s, 2H), 4.76 (bs, 1H), 5.67 (bs, 1H), 6.80–6.98 (m, 6H), 7.27–7.38 (m, 4H), 8.09 (s, 2H). 13C NMR (100 MHz, CDCl3): δ 10.89, 30.51, 47.27, 50.89, 54.89, 55.22, 65.88, 68.53, 113.84, 114.16, 118.05, 127.15, 128.34, 130.45, 131.30, 155.32, 159.59, 162.81, 185.60. HRMS: Found 503.2539; C30H34N2O5 requires 503.2546.
3-[4-(2-Morpholin-4-yl-ethoxy)-benzoyl]-1,4-diphenylazetidin-2-one 13e
Preparation as above from 12i (0.109 mmol, 50 mg) followed by purification with flash column chromatography (eluant: CH2Cl2: MeOH, 9:1); Yield 61%, Yellow crystals, IR νmax (KBr) cm− 1: 1750.0 (C = O), 1714.1 (C = O). 1H NMR (400 MHz, CDCl3) δ 2.59–2.63 (m, 4H), δ 2.84 (d, 2H, J = 5.52 Hz), 3.75 (d, 4H, J = 4.04 Hz), 4.10–4.21 (m, 2H), 4.80 (bs, 1H), 5.78 (bs,1H), 6.98–7.07 (m, 2H, Ar-H), 7.24–7.32 (m, 5H, Ar-H), 7.35–7.42 (m, 3H), 7.46 (d, 2H, J = 7.04 Hz), 8.10 (d, 2H, J = 8.52 Hz). 13C NMR, (100 MHz, CDCl3): δ 50.46, 52.99, 53.60, 55.35, 59.92, 67.72, 68.16, 114.06, 116.69, 123.81, 125.76, 128.45, 128.65, 128.81, 130.46, 131.37, 136.52, 136.86, 159.98, 162.89, 188.38. HRMS: Found 457.2115; C28H31N2O4 requires 457.2127, (M++1).
Biochemical evaluation of activity
Antiproliferation studies
All assays were performed in triplicate for the determination of mean values reported. Compounds were assayed as the free bases isolated from reaction. The human breast tumour cell line MCF-7 was cultured in Eagles minimum essential medium in a 95%O2/5% CO2 atmosphere with 10% foetal calf serum. The medium was supplemented with 1% non-essential amino acids. Cells were trypsinised and seeded at a density of 2.5 × 104 into a 96-well plate and incubated at 37°C, 95%O2/5% CO2 atmosphere for 24 h. After this time they were treated with 2 μl volumes of test compound which had been pre-prepared as stock solutions in ethanol to furnish the final concentration range of study, 1 nM–100 μM, and re-incubated for a further 72 h. Control wells contained the equivalent volume of the vehicle ethanol (1% v/v). The culture medium was then removed and the cells washed with 100 μl phosphate buffered saline (PBS) and 50 μl MTT added, to reach a final concentration of 1 mg/ml MTT added. Cells were incubated for 2 h in darkness at 37°C. At this point solubilization was begun through the addition of 200 μl DMSO and the cells maintained at room temperature in darkness for 20 min to ensure thorough colour diffusion before reading the absorbance. The absorbance value of control cells (vehicle treated) was set to 100% cell viability and from this graphs of absorbance versus cell density per well were prepared to assess cell viability and from these, graphs of percentage cell viability versus concentration of subject compound added were drawn.
Cytotoxicity studies
Human MCF-7 breast cancer cells were plated at a density of 2.5 × 104 per well in a 96-well plate, then incubated at 37°C, 95%O2/5% CO2 atmosphere for 24 h. Cells were treated with the compound of choice at varying concentrations (1 nM–100 μM), then incubated for a further 72 h. Following incubation 50 μl aliquots of medium were removed to a fresh 96-well plate. Cytotoxicity was determined using and LDH assay kit obtained from Promega, following the manufacturer's instructions for use. A 50 μl per well LDH substrate mixture was added and the plate left in darkness at room temperature for equilibration. Stop solution (50 μl) was added to all wells before reading the absorbance at 490 nm. A control of 100% lysis was determined for a set of untreated cells which were lysed through the addition of 20 μl lysis solution to the media 45 min prior to harvesting. Data was presented following calculation, as percentage cell lysis versus concentration of subject compound.
Oestrogen receptor binding assay
ERα and ERβ fluorescence polarization based competitor assay kits were obtained from Panvera at Invitrogen Life Technologies. The recombinant ER (insect expressed, full length, untagged human ER obtained from recombinant baculovirus-infected insect cells) and the fluorescent oestrogen ligand were removed from the − 80°C freezer and thawed on ice for one hour prior to use. The fluorescent oestrogen ligand (2 nM) was added to the ER (30 nM for ERα and 20 nM for ERβ) and screening buffer (100 mM potassium phosphate (pH 7.4), 100 μg /ml BGG, 0.02%NaN3 was added to make up to a final volume that was dependant on the number of tubes used (number of tubes (e.g. 50) × volume of complex in each tube(50 μl) = total volume (e.g. 2500 μl). Test compound (1 μl, concentration range 1 nM) to 100 μM) was added to 49 μl screening buffer in each borosilicate tube (6 mm diameter). To this 50 μl of the fluorescent oestrogen/ER complex was added to make up a final volume of 100 μl and final concentration range for the test compound of 0.01 nM to 1 μM. A vehicle control contained 1% (v/v) of ethanol and a negative control contained 50 μl screening buffer and 50 μl fluorescent oestrogen/ER complex. The negative control was used to determine the polarisation value when no competitor was present (theoretical maximum polarization). The tubes were incubated in the dark at room temperature for 2 h and were mixed by shaking on a plate shaker. The polarization values were read on a Beacon single-tube fluorescent polarization instrument with 485 nm excitation and 530 nm emission interference filters. For ERα and ERβ, graphs of anisotropy (mA) versus competitor concentration were obtained for determination of IC50 values.
Computational procedure
Ligand preparation
Compound 12d was drawn using ACD/Chemsketch v10 (Advanced Chemistry Labs. http://www.acdlabs.com/download/chemsk.html) and SMILES strings generated. A single conformer was generated ensuring a final MMFF optimisation step for refinement of the compound, using OMEGA v2.1 (developed and distributed by Openeye Scientific Software. http://www.eyesopen.com).
Receptor preparation
Protein Data Bank (PDB) entries 3ERT and 1QKN were downloaded from the PDB. A single bridging water molecule held between Glu353 (Glu305) and Arg394 (Arg346) was retained in both isoforms. Addition and optimisation of hydrogen positions was carried out using MOE 2005.06 (OMEGA v2.1, developed and distributed by Openeye Scientific Software. http://www.eyesopen.com) ensuring all other atom positions remained fixed.
Docking
FRED v2.11 (developed and distributed by Openeye Scientific Software. http://www.eyesopen.com) was utilized in this study to dock the conformer in both oestrogen receptor isoforms. All default values were applied with rigid-body optimisation of each ligand pose with Chemgauss2. The docked complexes for both isoforms were imported to Sybyl v6.91 (distributed by Tripos Inc. http://www.tripos.com) and processed by a flexible ligand and active site docking run. The active site residues assessed previously using MOE 2005.06 were allowed to be flexible during the docking calculation. All other values were kept as default except the number of iterations, which was increased to 10,000. Finally the docked complex was optimised under the MMFF force field using Szybki v1.1 (developed and distributed by Openeye Scientific Software. http://www.eyesopen.com), with optimisation of free rotor torsions and of polar hydrogen positions close to the ligand.
Results and discussion
Chemistry
The general procedure for the synthesis of the required β-lactams is shown in Scheme . In the present work the Reformatsky reaction was used exclusively for the β-lactam ring synthesis, because it affords 3-unsubstitued β-lactam products relatively easily and facilitates the subsequent chemical modification of the β-lactam at C-3. Surprisingly the Reformatsky type reaction has received very little attention within the context for β-lactam antibiotic synthesis but this is probably due to the low yields often produced in the ring formation [Citation44]. The products 10a–b were obtained in moderated yield by reaction of imines 9a–b (obtained by condensation of the appropriate arylamine and aryl aldehyde) with ethylbromoacetate in the presence of zinc and trimethylchlorosilane, (Scheme ) using the conditions reported by Palomo et al. [Citation41]. The choice of aryl substitutents was based on the requirement for hydrogen bonding substituents on the N-1 and C-4 phenyl rings to facilitate ER binding. The introduction of the required substituent at the C-3 position of the β-lactam ring is achieved by means of an aldol type reaction with a suitable phenolic aldehyde or ketone in order to introduce an α-(hydroxyaryl)methyl group at C-3 position of the β-lactam [Citation43]. The reactivity of protons in position 3 of the β-lactam is rather low, but by using a strong base (lithium diisopropylamide) under aprotic conditions it is possible to abstract one proton for the aldol reaction to proceed.
Scheme 1 Synthesis of β-lactams 12a–u. Scheme Reagents: (a) CH3CH2OCOCH2Br, Zn, TMCS, Benzene, reflux, 6h. (b) aldehyde/ketone, LDA, THF, 78°C, 30min. (c) R3R4N-CH2CH2Cl, Acetone, reflux, 2 h.
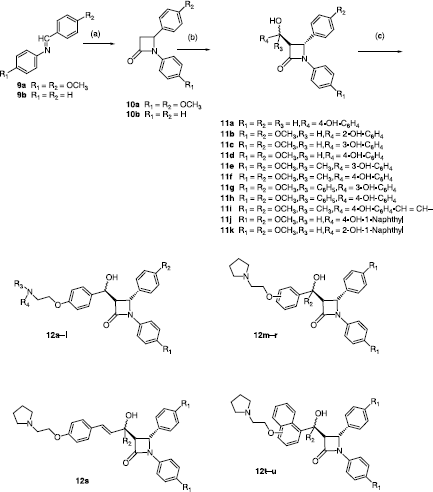
1,4-Diarylazetidin-2-ones 10a–b were subsequently used as the precursor for the synthesis of a variety of different 3-substituted β-lactams 11a–k. 4-Hydroxybenzophenone together with 3- and 4-hydroxyacetophenones were initially employed as the aryl carbonyl components. 2, 3- and 4-Hydroxybenzaldehydes, 2- and 4-hydroxynaphthaldehydes and 4-hydroxybenzylideneacetone were also utilized as carbonyl substrates in this reaction. Attempts to react the ortho-hydroxybenzophenone and ortho-hydroxyacetophenones were not successful, possibly for steric reasons.
The products 11a–k obtained in the aldol reactions contain three asymmetric centres (at C-3, C-4 and C-5). Reaction with the aryl aldehydes and ketones affordsed the trans β-lactam products in all cases which is clearly identified from the coupling constant displayed by the H-3, H-4 protons in the 1H NMR spectrum(∼2 Hz). Each product is obtained as a diastereomeric mixture which is evident from examination of the 1H NMR spectra. For example, in the case of compound 11d, H-3 is observed as two multiplets δ 3.35 (0.38H,) and δ 3.41 (0.62H), while H-4 appears as a two doublets δ 4.72 (0.62H) J = 2.04 Hz), and δ 5.15 (d, 0.38H, J = 4.08 Hz). The two doublets at 4.98 (0.62H, J = 6.16 Hz) and 5.11 (0.38H) are assigned to H-5. The diasteomeric components could be separated by HPLC, e.g. in the case of compound 11a, the two components are observed with retention times of 9.6 min (46%) and 11 min (54%). For each product 11a–k, the diastereomeric mixture was taken forward to the next reaction.
The nucleophilic addition of the basic side chain, required for ER antagonist activity onto the product 11a–k is illustrated in Scheme . Anhydrous potassium carbonate (K2CO3) is the most effective base used for the reaction. A variety of basic alkyl halide side-chains containing N,N-dimethylamino, N,N-diethylamino, pyrrolidine, methylpyrrolidine, piperidine and morpholine groupings were initially reacted with compounds 11a and 11d to afford the products 12a–l. Following initial SAR studies on the antiproliferative activity of products 12a–l with MCF-7 cells, the pyrrolidine type basic side-chain was shown to have the optimum effects for cytotoxic activity therefore the subsequent 3-substituted β-lactams were designed to contain this heterocyclic substituent. shows the structures of the series of β-lactams containing pyrrolidine side chain products obtained (12m–u). These products were isolated as diastereomeric mixtures in most cases, and the daistereomeric ratios could be determined on examination of the 1H NMR spectrum. For example, in the case of compound 12a, H-3 is observed as a multiplet at δ3.41-3.47, H-4 occurs as two doublets at δ 4.81 and δ 5.31 (each 0.5H, J = 2.52, 4.04 Hz,), while H-5 was identified as two doublets at δ 5.09 and δ 5.19 (each 0.5H, J = 7.00, 2.48 Hz). In the case of compounds 12r and 12t, only a single diastereomer was observed in the 1H NMR spectrum, possibly because of the hindered nature of the alcohol product. Compound 12o, which was obtained in a diastereomeric ratio of 4:1 from 1H NMR, was resolved into its four component stereoisomers by chiral capillary electrophoresis. Retention times of 11.8 and 12.2 min (corresponding to each enantiomer of the smaller component) and 13.0 and 14.0 min for each enantiomer of the larger component.
To study the effect on antiproliferative activity of the introduction of a carbonyl group at C-3, the secondary alcohol in compounds 12b, 12d, 12f, 12i and 12j was examined (Scheme ). This carbonyl hinge type structural feature is present in raloxifene and contributes to the binding and ER activity of the drug in orientating the basic side group to bind to Asp351. The oxidation of the alcohols 12b, 12d, 12f, 12i and 12j was achieved by treatment of the appropriate secondary alcohol with PCC to afford carbonyl products 13a–e with moderate yield. The trans β-lactam stereochemistry is retained in the products 13a–e as evident from the 1H NMR spectrum, (e.g. for compound 13a H-3 appears as a doublet at δ4.75 which is coupled to H-4 at δ5.67 (J = 2 Hz).
Biochemistry
The β-lactam compounds prepared above were evaluated in a series of in vitro assays which determined their antiproliferative activity in MCF-7 and MDA-MB 231 breast cancer cell lines and also their affinity for the oestrogen receptor.
Antiproliferative activity in MCF-7 and MDA-MB 231 breast cancer cells
Compounds 12a–l were initially screened for their antiproliferative activity using the ER expresing (ER dependent) MCF-7 human breast cancer cell line. shows the structures of the β-lactam compounds 12a–l that were initially screened to identify different antagonist properties depending on the substiutents on the basic side-chain. The IC50 values of the initial β-lactam products evaluated are reported in below and compared to that of tamoxifen1a (IC50 = 2.48 μM). Most of the compounds have values much higher than tamoxifen however compound 12d (containing the pyrrolidine type side chain and methoxy groups at the para position on the phenyl rings at the C-4 and N-1 positions) was found to be the most potent compound in the series with IC50 value of 4.63 μM. This was the template chosen for the remaining products synthesised.
Table I. Antiproliferative and cytotoxic effects of compounds 12a–l in MCF-7 cells..
Oxidation of the hydroxy group at the C-5 position resulted in the synthesis of the ketones 13a–e, which were screened to assess whether the ketone had any positive or negative effect on the antiproliferative efficacy of these compounds. The SERM raloxifene 3, , contains a carbonyl linking hinge group; replacement of this carbonyl group with an ether linkage as in arzoxifene afforded products with similar efficacy profiles to tamoxifen and also induced a 10-fold increase in antioestrogen potency both in vivo and in vitro [Citation45] Methylene groups are also reported as Ring A linking hinge groups in benzopyranone SERMs [Citation46]. The antiproliferative and cytotoxic results for the carbonyl type β-lactam compounds 13a–d are shown in . Oxidation from secondary alcohol to ketone in 13a–e does not appear to increase the biochemical activity of the products in MCF-7 cells although it does alter the molecular structure of the compounds and alignment of the basic substituent of Ring A. The most potent compound in this series 13b (IC50 = 3.95 μM) is considerably more active than the corresponding alcohol 12b (IC50 = 25.2 μM). Comparing 12d (IC50 = 4.63 μM) to its corresponding carbonyl analogue 13c (IC50 = 37.25 μM), there is a significant loss in activity. Subsequent β-lactam SERMs were developed as the 5-hydroxy compounds.
Table II. Antiproliferative and cytotoxic effects of compounds 13a–e containing carbonyl at C-5 in MCF-7 cells.
Further SAR studies of these 5-hydroxy-β-lactam compounds were carried out with a variety of structural modifications, e.g. H-5 was replaced by a methyl group or a phenyl ring, (compounds 12o–s). The position of the basic side chain was also examined at ortho and meta positions of ring A, while fused cyclic systems were also used in place of the phenyl ring (compounds 12t–u). These structural variations are illustrated in . The biochemical results are displayed in and reveal improved activity over the previous compounds with IC50 values of 10 μM or less for most of the products and no significant cytotoxicity effect. The presence of the basic side chain at the para position of Ring A appears to be the optimum structure with a slight decrease in activity noted if it is placed in the meta position; a greater loss in activity is observed if the basic substituent is located at the ortho position, e.g. for compound 12d (para), IC50 = 4.63 μM, 12m (ortho), IC50 = 10.70 μM) and 12n (meta), IC50 = 5.74 μM. The replacement of the C-5 hydrogen (R2) in compounds 12o–p with the methyl group does not improve activity; however replacement with the phenyl ring dramatically improves activity for the para substituted compound 12r resulting in IC50 values in the nanomolar region (IC50 = 130 nM) for the most potent compound. The presence of the additional phenyl ring at C-5 might result in greater displacement of H12 by the ligand and therefore greater antagonist properties. Inclusion of phenylethylene and naphthyl groups as C-5 substituents also resulted in products with good antiproliferative activity (compounds 12s–u). shows the antiproliferative and cytotoxic results for 12u, a representative example of these modified β-lactam type compounds.
Table III. Antiproliferative and cytotoxic effects of compounds 12m–u with modifications at the C-5 position in MCF-7 cells.
Figure 2 Compound 12u inhibited proliferation and induced cytotoxicity of MCF-7 cells. Antiproliferative and cytotoxic activity of compounds 12u on oestrogen sensitive MCF-7 breast cancer cells, IC50 = 5.51 μM. The optical density values are given as a ratio of the treated cells and control cells × 100% and are means of at least 9 replicates. The absence of error bars indicates that the error was smaller than the size of the symbol.
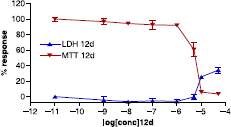
Compounds (12m, 12n, 12t) were tested for their antiproliferative effects in MDA-MB-231 cells. If these compounds are acting as SERMs they may not show significant antiproliferative effects in this ER negative cell line. Tamoxifen has been shown to have some effects in ER negative cell lines but always at much higher concentrations (IC50 value approx. 20 μM) than in MCF-7 cells suggesting that some of its effects are mediated through an alternative mechanism. In the case of the β-lactam compounds 12m, 12n and 12t similar results were observed for MDA-MB-231 cell line (IC50 = 12.40, 12.50 and 3.74 μM respectively) as were observed for the MCF-7 inhibition.
The cytotoxicity of the compounds was determined in the standard LDH assay to establish that the antiproliferative effects observed were due to cytostasis rather than cellular necrosis. The results obtained are displayed in . The majority of the compounds demonstrated low cytotoxicity indicating that their action is cytostatic rather than cytotoxic. Cytotoxicity values considerably below that obtained for tamoxifen, (13.4%, 10 μM) were observed, with the most potent compound 12r, having low cytotoxicity, (3%, 10 μM in MCF-7 cell line). The products also showed low cytotoxicity in MDA-MB-231 cell line (e.g. compound 12n 2%, 10 mM).
Oestrogen receptor binding studies
Receptor binding studies were carried out with ERα and ERβ to determine if the observed antiproliferative effects were mediated through the oestrogen receptor. A fluorescence polarisation procedure was employed. [Citation22]. This is a competitive binding assay which measures the displacement of fluorescein labelled estradiol (fluoromone) from the human recombinant full length ERα and ERβ expressed from baculovirus-infected insect cells. As an example of the series, compound 12b exhibited moderate binding to both ERα and ERβ with IC50 values = 75.20 μM for ERα and IC50 values = 71.95 μM for ERβ. The compounds did not display oestrogenic alkaline phosphatase activity in the Ishikawa cell line [Citation47].
Molecular modelling studies of novel β-lactam compounds
To gain a greater understanding of the mode of binding of these β-lactam products, a brief computational docking study was carried out with ERα and ERβ using compound 12d as an example. The crystal structures used in the docking studies were obtained from the cocrystallisation of human ERα with 4-hydroxytamoxifen 2 [Citation16] (3ERT)and rat ERβ with raloxifene 3 (1QKN) [Citation18] which shows raloxifene bound in a position similar to that observed in the human ERα complex. The major difference is observed in the phenolic ring B, where the distal hydroxy moieties in the two isotypes are 1.4 Å apart. The result of this orientation of raloxifene 3 in the β-isotype is that the piperidine ring is directed outward from the cavity and prevents H12 from adopting its agonist position. This shift is most probably responsible for the pure antagonist character of raloxifene 3 on ERβ [Citation17].
To quantify the relative interactions predicted for the ligand in comparison to the experimental results observed for 4-hydroxytamoxifen and raloxifene, a simple ligand-protein contact (LPC) [Citation48] analysis was carried out using Chem Gauss II (LPC), referring to the following specific residues: Glu353 and Arg394 (which are known to be crucial in the binding of Ring B of the ligands to the active site), His524 (known to be important oestrogenic residue in the binding of diethylstilboestrol and oestradiol) and Asp351 which is well recognised as an important antioestrogenic residue associated with the binding of the basic side chain nitrogen.
As a representative example, compound 12d is docked in the active site of Erα superposed with 4-hydroxytamoxifen (), and also in ERβ superposed with raloxifene, . Compound 12d appears to show some interaction with the relevant amino acid residues of the LBDs of both ERα and Erβ as illustrated in . The basic side chain of Ring A, which interacts with the Asp 351, extends further from the binding pocket in ERα than the corresponding chain in 4-hydroxytamoxifen, (). However ring B appears in close proximity to Glu353 and Arg394 while ring C is in the region of His524 as required. In ERβ there also appears to be good fit for 12d in the ligand binding pocket when compared with raloxifene, (). When compared with raloxifene 3 which also contains an extended basic side-chain substituent on Ring A, the pyrrolidine substituent of the β-lactam 12d would also seem to be positioned well for binding to Asp303 in ERβ.
Conclusion
We have synthesised a number of novel β-lactam compounds designed as potential oestrogen receptor ligands, which demonstrate antiproliferative activity against the MCF-7 human breast cancer cell line. The effect of a number of aryl and amine functional group substitutions on the antiproliferative activity of the β-lactam products was explored. Initial molecular modelling studies indicate that the compounds may dock in the expected antioestrogenic mode in the ERα and ERβ ligand binding sites. Because of moderate affinity shown by these compounds for the ER, these results appear to confirm that the primary antiproliferative activity may not be exclusively via an ER pathway. In further work, the optimisation of the aryl substituent requirements for ER binding will be investigated. These compounds which are ring fused analogues of (Z)-tamoxifen expand the structural diversity of ligands which act as antagonists for the oestrogen receptor without the possibility of the metabolic isomerisation of the tamoxifen type antioestrogen structures.
Acknowledgements
We are very grateful to Professor Richard Hochberg at Yale University Medical School, for kindly facilitating the alkaline phosphatase experiments and for the generous gift of the Ishikawa cells. We thank Dr. Ana Luisa Simplicio, School of Pharmacy and Pharmaceutical Sciences, Trinity College Dublin for the capillary electrophoresis experiments. This work was supported through funding from the Trinity College IITAC research initiative (HEA PRTLI, Cycle 3), with additional support for computational facilities from the Wellcome Trust.
Declaration of interest: The authors report no conflicts of interest. The authors alone are responsible for the content and writing of the paper.
References
- JI MacGregor, and VC Jordan. (1998). Basic guide to the mechanisms of antiestrogen action. Pharmacol Rev 50 (2):151–196.
- VC Jordan. (2003). Antiestrogens and selective estrogen receptor modulators as multifunctional medicines. 1. Receptor interactions. J Med Chem 46 (6):883–908.
- VC Jordan. (2003). Antiestrogens and selective estrogen receptor modulators as multifunctional medicines. 2. Clinical considerations and new agents. J Med Chem 46 (7):1081–1111.
- EA Ariazi, JL Ariazi, F Cordera, and VC Jordan. (2006). Estrogen receptors as therapeutic targets in breast cancer. Curr Top Med Chem 6 (3):181–202.
- TA Grese, S Cho, DR Finley, AG Godfrey, CD Jones, CW Lugar3rd, MJ Martin, K Matsumoto, LD Pennington, MA Winter, MD Adrian, HW Cole, DE Magee, DL Phillips, ER Rowley, LL Short, AL Glasebrook, and HU Bryant. (1997). Structure–activity relationships of selective estrogen receptor modulators: Modifications to the 2-arylbenzothiophene core of raloxifene. J Med Chem 40 (2):146–167.
- B Fisher, JP Costantino, DL Wickerham, CK Redmond, M Kavanah, WM Cronin, V Vogel, A Robidoux, N Dimitrov, J Atkins, M Daly, S Wieand, E Tan-Chiu, L Ford, and N Wolmark. (1998). Tamoxifen for prevention of breast cancer: Report of the National Surgical Adjuvant Breast and Bowel Project P-1 study. J Natl Cancer Inst 90 (18):1371–1388.
- S Ahmed. (1998). Review of the molecular modelling studies of the cytochrome P-450 estrogen synthetase enzyme, aromatase. Drug Des Discov 15 (4):239–252.
- JF Robertson. (2001). Faslodex (ICI 182, 780), a novel estrogen receptor downregulator–future possibilities in breast cancer. J Steroid Biochem Mol Biol 79 (1–5):209–212.
- V Stearns, NE Davidson, and DA Flockhart. (2004). Pharmacogenetics in the treatment of breast cancer. Pharmacogenomics J 4 (3):143–153.
- J Jensen, JW Kitlen, P Briand, F Labrie, and AE Lykkesfeldt. (2003). Effect of antiestrogens and aromatase inhibitor on basal growth of the human breast cancer cell line MCF-7 in serum-free medium. J Steroid Biochem Mol Biol 84 (4):469–478.
- I Kijima, T Itoh, and S Chen. (2005). Growth inhibition of estrogen receptor-positive and aromatase-positive human breast cancer cells in monolayer and spheroid cultures by letrozole, anastrozole, and tamoxifen. J Steroid Biochem Mol Biol 97 (4):360–368.
- AM Brodie, Q Lu, BJ Long, A Fulton, T Chen, N Macpherson, PC DeJong, MA Blankenstein, JW Nortier, PH Slee, J van de Ven, JM van Gorp, JR Elbers, ME Schipper, GH Blijham, and JH Thijssen. (2001). Aromatase and COX-2 expression in human breast cancers. J Steroid Biochem Mol Biol 79 (1-5):41–47.
- JA Gustafsson. (1999). Estrogen receptor beta–a new dimension in estrogen mechanism of action. J Endocrinol 163 (3): 379–383.
- ST Pearce, and VC Jordan. (2004). The biological role of estrogen receptors alpha and beta in cancer. Crit Rev Oncol Hematol 50 (1):3–22.
- A Bardin, N Boulle, G Lazennec, F Vignon, and P Pujol. (2004). Loss of ERbeta expression as a common step in estrogen-dependent tumor progression. Endocr Relat Cancer 11 (3): 537–551.
- AK Shiau, D Barstad, PM Loria, L Cheng, PJ Kushner, DA Agard, and GL Greene. (1998). The structural basis of estrogen receptor/coactivator recognition and the antagonism of this interaction by tamoxifen. Cell 95 (7):927–937.
- AM Brzozowski, AC Pike, Z Dauter, RE Hubbard, T Bonn, O Engstrom, L Ohman, GL Greene, JA Gustafsson, and M Carlquist. (1997). Molecular basis of agonism and antagonism in the oestrogen receptor. Nature 389 (6652):753–758.
- AC Pike, AM Brzozowski, RE Hubbard, T Bonn, AG Thorsell, O Engstrom, J Ljunggren, JA Gustafsson, and M Carlquist. (1999). Structure of the ligand-binding domain of oestrogen receptor beta in the presence of a partial agonist and a full antagonist. EMBO J 18 (17):4608–4618.
- YL Wu, X Yang, Z Ren, DP McDonnell, JD Norris, TM Willson, and GL Greene. (2005). Structural basis for an unexpected mode of SERM-mediated ER antagonism. Mol Cell 18 (4): 413–424.
- AC Pike, AM Brzozowski, J Walton, RE Hubbard, AG Thorsell, YL Li, JA Gustafsson, and M Carlquist. (2001). Structural insights into the mode of action of a pure antiestrogen. Structure 9 (2):145–153.
- DG Lloyd, RB Hughes, DM Zisterer, DC Williams, C Fattorusso, B Catalanotti, G Campiani, and MJ Meegan. (2004). Benzoxepin-derived estrogen receptor modulators: A novel molecular scaffold for the estrogen receptor. J Med Chem 47 (23):5612–5615.
- DG Lloyd, HM Smith, T O' Sullivan, DM Zisterer, and MJ Meegan. (2005). Synthesis, structure–activity realtionships and antagonistic effects in human MCF-7 breast cancer cells of flexible estrogen receptor modulators. Med Chem 1 (4): 335–353.
- MJ Meegan, I Barrett, J Zimmermann, AJ Knox, DM Zisterer, and DG Lloyd. (2007). Benzothiepin-derived molecular scaffolds for estrogen receptor modulators: Synthesis and antagonistic effects in breast cancer cells. J Enzyme Inhib Med Chem 22 (5):655–666.
- DG Lloyd, HM Smith, T O'Sullivan, AS Knox, DM Zisterer, and MJ Meegan. (2006). Antiestrogenically active 2-benzyl-1,1-diarylbut-2-enes: Synthesis, structure–activity relationships and molecular modeling study for flexible estrogen receptor antagonists. Med Chem 2 (2):147–168.
- AJ Knox, MJ Meegan, and DG Lloyd. (2006). Estrogen receptors: Molecular interactions, virtual screening and future prospects. Curr Top Med Chem 6 (3):217–243.
- S Kim, JY Wu, ET Birzin, K Frisch, W Chan, LY Pai, YT Yang, RT Mosley, PM Fitzgerald, N Sharma, J Dahllund, AG Thorsell, F DiNinno, SP Rohrer, JM Schaeffer, and ML Hammond. (2004). Estrogen receptor ligands. II. Discovery of benzoxathiins as potent, selective estrogen receptor alpha modulators. J Med Chem 47 (9):2171–2175.
- L Gennari. (2006). Lasofoxifene: A new type of selective estrogen receptor modulator for the treatment of osteoporosis. Drugs Today (Barc) 42 (6):355–367.
- SR Stauffer, YR Huang, ZD Aron, CJ Coletta, J Sun, BS Katzenellenbogen, and JA Katzenellenbogen. (2001). Triarylpyrazoles with basic side chains: Development of pyrazole-based estrogen receptor antagonists. Bioorg Med Chem 9 (1): 151–161.
- J Renaud, SF Bischoff, T Buhl, P Floersheim, B Fournier, M Geiser, C Halleux, J Kallen, H Keller, and P Ramage. (2005). Selective estrogen receptor modulators with conformationally restricted side chains. Synthesis and structure–activity relationship of ERalpha-selective tetrahydroisoquinoline ligands. J Med Chem 48 (2):364–379.
- F Labrie, C Labrie, A Belanger, J Simard, S Gauthier, V Luu-The, Y Merand, V Giguere, B Candas, S Luo, C Martel, SM Singh, M Fournier, A Coquet, V Richard, R Charbonneau, G Charpenet, A Tremblay, G Tremblay, L Cusan, and R Veilleux. (1999). EM-652 (SCH 57068), a third generation SERM acting as pure antiestrogen in the mammary gland and endometrium. J Steroid Biochem Mol Biol 69 (1–6):51–84.
- BK Banik, and FF Becker. (2001). Synthesis, electrophilic substitution and structure–activity relationship studies of polycyclic aromatic compounds towards the development of anticancer agents. Curr Med Chem 8 (12):1513–1533.
- BK Banik, FF Becker, and I Banik. (2004). Synthesis of anticancer beta-lactams: Mechanism of action. Bioorg Med Chem 12 (10):2523–2528.
- EL Setti, D Davis, JW Janc, DA Jeffery, H Cheung, and W Yu. (2005). 3,4-disubstituted azetidinones as selective inhibitors of the cysteine protease cathepsin K. Exploring P3 elements for potency and selectivity. Bioorg Med Chem Lett 15 (5):1529–1534.
- G Veinberg, M Vorona, I Shestakova, I Kanepe, O Zharkova, R Mezapuke, I Turovskis, I Kalvinsh, and E Lukevics. (2000). Synthesis and antitumor activity of selected 7-alkylidene substituted cephems. Bioorg Med Chem 8 (5):1033–1040.
- G Veinberg, I Shestakova, M Vorona, I Kanepe, and E Lukevics. (2004). Synthesis of antitumor 6-alkylidenepenicillanate sulfones and related 3-alkylidene-2-azetidinones. Bioorg Med Chem Lett 14 (1):147–150.
- DA Burnett. (2004). Beta-lactam cholesterol absorption inhibitors. Curr Med Chem 11 (14):1873–1887.
- RM Adlington, JE Baldwin, GW Becker, B Chen, L Cheng, SL Cooper, RB Hermann, TJ Howe, W McCoull, AM McNulty, BL Neubauer, and GJ Pritchard. (2001). Design, synthesis, and proposed active site binding analysis of monocyclic 2-azetidinone inhibitors of prostate specific antigen. J Med Chem 44 (10):1491–1508.
- JC Sutton, SA Bolton, ME Davis, KS Hartl, B Jacobson, A Mathur, ML Ogletree, WA Slusarchyk, R Zahler, SM Seiler, and GS Bisacchi. (2004). Solid-phase synthesis and SAR of 4-carboxy-2-azetidinone mechanism-based tryptase inhibitors. Bioorg Med Chem Lett 14 (9):2233–2239.
- M Masui, and H Ohmuri. (1972). Aniodic oxidation of Schiff bases. Part I. Oxidation of N-benzylidene-p-anisidines in acetonitrile. J Chem Soc Perkin Trans 2 1882–1886.
- KS Balachandran, I Bhatnagar, and MV George. (1968). Oxidation by metal oxides. IV. Oxidation of organic compounds using nickle peroxide. J Org Chem 33:3891–3895.
- C Palomo, FP Cossio, A Arrieta, JM Odriozola, M Oiarbide, and JM Ontoria. (1989). The Reformatsky type reaction of Gilman and Speeter in the preparation of valuable beta-lactams in carbapenem synthesis–scope and synthetic utility. J Org Chem 54 (24):5736–5745.
- HH Otto, and R Mayrhofer. (1983). Stereochemistry of dehydration and halogenation of alpha-R-star and alpha-S-star isomeric 3-(alpha-hydroxybenzyl)-1,4-diphenyl-2-azetidinones. Liebigs Ann Chem (7):1162–1168.
- HH Otto, R Mayrhofer, and HJ Bergmann. (1983). Synthesis and stereochemistry of 3-(a-hydroxybenzyl)-1,4-diphenyl-2-azetidinones. Liebigs Ann Chem (7):1152–1161.
- C Palomo, JM Aizpurua, I Ganboa, and M Oiarbide. (2004). Asymmetric synthesis of beta-lactams through the Staudinger reaction and their use as building blocks of natural and nonnatural products. Curr Med Chem 11 (14):1837–1872.
- CR Overk, KW Peng, RT Asghodom, I Kastrati, DD Lantvit, Z Qin, J Frasor, JL Bolton, and GR Thatcher. (2007). Structure–activity relationships for a family of benzothiophene selective estrogen receptor modulators including raloxifene and arzoxifene. Chem Med Chem 2 (10):1520–1526.
- JA McKie, SS Bhagwat, H Brady, M Doubleday, L Gayo, M Hickman, RK Jalluri, S Khammungkhune, A Kois, D Mortensen, N Richard, J Sapienza, G Shevlin, B Stein, and M Sutherland. (2004). Lead identification of a potent benzopyranone selective estrogen receptor modulator. Bioorg Med Chem Lett 14 (13):3407–3410.
- BA Littlefield, E Gurpide, L Markiewicz, B McKinley, and RB Hochberg. (1990). A simple and sensitive microtiter plate estrogen bioassay based on stimulation of alkaline phosphatase in Ishikawa cells: Estrogenic action of delta 5 adrenal steroids. Endocrinology 127 (6):2757–2762.
- V Sobolev, A Sorokine, J Prilusky, EE Abola, and M Edelman. (1999). Automated analysis of interatomic contacts in proteins. Bioinformatics 15 (4):327–332.