Abstract
The inhibition of jack bean urease by 2,3-dichloro-1,4-naphthoquinone (DCNQ) was studied at ambient temperature in 20 mM phosphate buffer, pH 7.8. The process was investigated by incubation procedure in the absence of substrate. It was found that DCNQ acted as a time- and concentration-dependent inactivator of urease. The time course of the reaction displayed a biphasic mode. Each phase followed a pseudo-first-order kinetics, however the inactivation rate at the first phase was significantly faster than at the next one. The biphasity indicated the complex mechanism of DCNQ action on urease. Quinones action on proteins has been elucidated as at least two processes: direct arylation of essential protein thiols and/or indirect oxidation of essential thiols by reactive oxygen species (ROS) realising during quinone reduction to semiquinones. The next evidence of the studied mechanism was provided by the reactivation experiment that showed the participation of reversible and irreversible processes in the inactivation. The application of dithiothreitol (DTT) into DCNQ blocked-urease solution resulted in an effective enzyme activity regain which quickly returned to 70 ± 10%. The irreversible inactivation of urease was attributed to DCNQ arylation of thiol residues in the protein. On the other hand, it was assumed that the reversible inactivation was a result of the action of ROS such as H2O2. Presence of H2O2 in the incubation system was proved by an experiment with the use of catalase. The enzyme by the elimination of H2O2 decreased DCNQ inactivating influence on urease. The comparison of participation of the fast and slow phase in the inactivation with the percentage of the process reversibility was assumed that the fast period was a result of the arylation mechanism while the slow phase was related to the oxidative influence of H2O2.
Introduction
Urease (urea amidohydrolase, EC 3.5.1.5) catalyzes the hydrolysis of urea: CO(NH2)2 + H2O → 2NH3 + CO2. The enzyme is widely distributed in nature and is found in plants, algae, fungi and bacteria [Citation1–3]. Urease active site contains two nickel ions directly involved in binding of substrates and inhibitors [Citation4]. The plant urease from jack bean is the homohexameric molecule (α6). The subunit contains 840 amino acid residues and its molecular weight is equal to 90.77 ± 5 kD. Urease is thiol rich enzyme. The total thiol content in jack bean urease has been determined by DTNB titration in the presence of 6M guanidinum chloride. It was proved that urease contains 15 thiol groups per subunit. However, only 6 of 15 cysteines are accessible to the thiol reagent (without denaturation of the enzyme). One of them, cysteine-592, is located on the mobile flap closing the active site of urease plays a critical role in the catalytic activity. The chemical modification of cysteine-592 results in the inactivity of urease [Citation5–7].
The study of urease inhibition is of medical, agricultural and environmental significance. Common occurrence of urease in the environment enables decomposition of urea as a product of catabolism of nitrogen-containing compounds. On the other hand, the negative influence of products of urea hydrolysis on human and animal health as well as on the environment causes that the urease activity has to be controlled [Citation1]. Lots of bacteria containing urease (e.g. Proteus, Klebsiella, Staphylococcus, Helicobacter pylori) are responsible for infections of urinary and gastrointestinal tracts [Citation8–9]. The urease inhibitors such as hydroxamic acids and phosphoroamides have been applied in therapy for blocking the enzyme action [Citation10]. The inhibitory ability also shows the garlic extract applied as a natural antibiotic against urease [Citation11].
High activity of urease in soil decreases economic use of urea as a fertilizer. One of the approaches meant to counteract this negative effect is the use of urease inhibitors in combination with urea fertilizers. Among a variety of substances studied as urease inhibitors are quinones and their derivatives [Citation12–16]. This group of chemicals has been shown to be cytotoxic, therefore it has been utilised as anticancer, antibacterial or antimalarial drugs and fungicides [Citation17,Citation18]. The inhibitory power of substituted quinones largely depends upon their substituent group. It was found that halogenation increased inhibitory effectiveness hence chloranil (tetrachloro-1,4-benzoquinone) and dichlone (2,3-dichloro-1,4-naphthoquinone) are fungicides most frequently used [Citation19].
Two general mechanisms of cytotoxic quinones action have been elucidated. The first one is sulfhydryl arylation, which resulted in irreversible, covalent modification of protein residues. The next mechanism is redox cycling leading to generation of reactive oxygen species (ROS) able to oxidize of cellular macromolecules [Citation20–22]. In our previous studies we showed that 1,4-benzoquinone, tetrachloro-1,4-benzoquinone and 2,5-dimethyl-1,4-benzoquinone are slow binding inhibitors of jack bean urease, and their overall inhibition constants Ki* were found to be 45 nM, 0.45 nM and 12 μM, respectively [Citation23–25]. In contrast to mentioned derivatives, 1,4-naphthoquinone is a time and concentration dependent inactivator of urease. The inactivation followed two mechanisms: arylation and oxidation of urease thiol residues provoked by quinone-catalyzed redox cycling [Citation15,Citation16].
This work is aimed at elucidation of the kinetics and mechanism of jack bean urease inhibition by 2,3-dichloro-1,4-naphthoquinone (DCNQ). The process was studied at pH 7.8. The kinetics of inactivation was examined. The protective effects of thiols and catalase as well as the reversibility of the urease-DCNQ complex were investigated. The participation of arylation and redox cycling was elucidated.
Materials and methods
Materials
Jack bean urease, Sigma type III, specific activity 16 U mg−1 solid, the total content of reducing agents 0.5 μg/unit, urea (Molecular Biology Reagent), dithiothreitol (DTT), glutathione (Glu), L-cysteine (L-Cys), catalase (from bovine liver), activity 1340 U mg−1 solid were purchased from Sigma and the inhibitor 2,3-dichloro-1,4-naphthoquinone (DCNQ) from Aldrich. Other chemicals were obtained from POCh, Gliwice, Poland. All reagents used were of analytical grade. Phosphate buffer 20 mM, pH 7.8, was prepared by adjusting pH of phosphoric(V) acid with NaOH. 2 mM EDTA was added to all enzyme-containing solutions.
Standard urease activity assay
The standard assay mixture (25 cm3) consisted of 50 mM urea in 20 mM phosphate buffer, pH 7.8 and 2 mM EDTA. The reactions were initiated by the addition of small aliquots of the enzyme-containing solution (0.5 cm3), and the urease activity was determined by measuring ammonia concentration after a 5 min reaction. Ammonia was determined by the spectrophotometric, phenol-hypochlorite method. The absorbance was registered at 630 nm [Citation26]. The measurements were performed at ambient temperature. The activity of uninhibited urease was accounted as the control activity of 100%.
Inactivation of urease by 2,3-dichloro-1,4-naphthoquinone
The solution of urease was incubated with the solution of the inhibitor in the absence of urea. The incubation mixture contained 1.0 mg cm−3 of urease, 20 mM phosphate buffer, pH 7.8, 2 mM EDTA and DCNQ in the range: 3-12 μM. The time when the enzyme and the inhibitor were mixed was marked as zero time of incubation. After appropriate periods of time, aliquots were withdrawn from the incubation mixture and transferred into the standard assay mixtures for urease residual activity determination.
Protective influence of thiols on urease inactivation by DCNQ
The protection influence of reducing agents: L-cysteine, glutathione and dithiothreitol on urease inactivation by DCNQ was tested. The incubation mixture contained 1.0 mg cm−3 urease, 8 μM DCNQ, 20 mM phosphate buffer, pH 7.8 and 0.5 mM reducing agent. The incubations were carried out for 10 and 30 min. The control sample instead of the reducing agent contained a proper volume of the buffer. After a 10 and 30 min incubation, a sample of the incubation mixture was withdrawn and transferred into the standard assay mixture for urease residual activity determination.
Protective influence of catalase on urease inactivation by DCNQ
Catalase was incubated with DCNQ for 30 min. Next urease was added to the mixture and incubation was continued for another 30 min. The final mixtures contained 1 mg cm−3 urease, 200 U cm−3 catalase, 20 mM phosphate buffer, pH 7.8 and DCNQ: 0, 5.5, 11 μM, respectively. After incubations, aliquots were withdrawn from the incubation mixture and transferred into the standard assay mixtures for urease residual activity determination.
Reactivation of DCNQ inactivated urease
The reactivation of DCNQ inactivated urease was studied by using DTT. The incubation mixture contained 1.0 mg cm−3 urease, 12 μM DCNQ, 20 mM phosphate buffer, pH 7.8. After a 45 min incubation DTT was added. DTT concentration in the incubation mixture was equal to 125 μM. The activity of urease was determined before and after DTT addition. After appropriate periods of time samples of the incubation mixture were withdrawn and transferred into the standard assay mixture and urease residual activity was determined. The experiment was triple repeated.
Quantitative measurements of hydrogen peroxide
Concentration of hydrogen peroxide in DCNQ containing mixtures was measured by titration with 0.002 M KMnO4 in the presence of H2SO4. 14 μM DCNQ was mixed with 2.0 mg cm−3 of urease, 0.2 or 0.4 mM DTT, respectively, in the volume ratio 1:1. The mixtures were incubated for 60 min. Urease without DCNQ was titrated and accounted as a blank assay. The result obtained for the blank assay was subtracted from all titrations.
Results and discussion
Urease inactivation by DCNQ: kinetics elucidation
The inactivation progress curves as a dependence of the urease residual activity (RA) vs incubation time of urease with DCNQ are presented in . The data obtained showed that DCNQ inhibited urease in a time and concentration dependant manner. Total inactivation was observed for 12 μM DCNQ after a one hour incubation. Transfer of the inactivation data into semilogarithmic scale () revealed the biphasic mode of the inactivation kinetics. Each phase exhibited a first-order-kinetics. The time course of the inhibition (shown in ) can be represented by the equation containing two first-order terms, corresponding to the fast and slow phase of the reaction (Equation 1) [Citation27]:
where: RA is the residual activity in %; kf, ks are the rate constants of the fast and slow period of the inhibition; a denotes the slow phase contribution parameter that indicates the percentage of the phase participation in the process.
Figure 1. (a) Inactivation progress curves as a dependence of urease residual activity vs incubation time at pH 7.8, for different DCNQ concentrations. (b) Dependence of urease residual activity vs incubation time in semilogarithmic system. DCNQ concentration is given numerically.
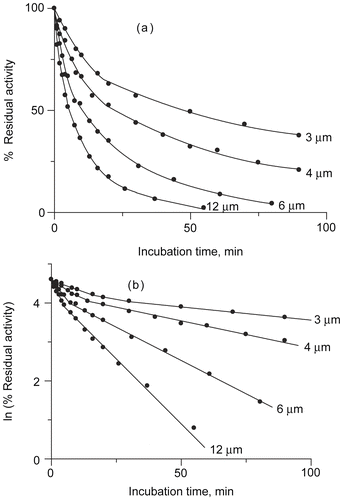
The rate constants and parameter a were obtained by linear regression analysis of semilogarithmic plots of residual activity vs incubation time and are listed in . The results indicated that the increase of DCNQ concentration caused the increase of the rate constants, however the influence on the parameter a was insignificant. In the studied DCNQ concentration range, ~30% of the enzymatic activity was inhibited in the fast phase, the remaining ~70% was blocked in the slow phase.
Table 1. Kinetic constants of urease inactivation by DCNQ: the rate constants of the fast (kf) and slow phase (ks) and the slow phase contribution parameter a.
The decrease of the enzymatic activity as a function of DCNQ concentration for two incubation times equal to 20 minutes and 60 minutes is presented in . Obtained relationships were used for determination of the parameter IC50. The determined values were equal to 4.2 μM and 2.9 μM for a 20 and 60 min incubation, respectively.
1,4-naphthoquinone (at the same experimental conditions) inhibited urease also by biphasic mode [Citation16] but was found to be a weaker urease inhibitor. Its IC50 was equal to 20 μM for a 20 minute incubation, which means 4 times lower inhibitory strength of 1,4-naphthoquinone than DCNQ.
Protective experiments
The protective influence of thiols was tested at two time points of the incubation. The results were compared with the data of unprotected system. It was shown that the coexistence of monothiols: L-cysteine, glutathione or dithiol: dithiothreitol (DTT) in the incubation mixture prevented urease against DCNQ inhibition (). It was observed that urease lost more than 80% of activity after a 30 minutes incubation with DCNQ while the coexistence of above mentioned thiols resulted in less than 20% decrease of urease activity. The fact that thiols affected the DCNQ blocking process indicated the urease sulfhydryl group(s) as the residue(s) responsible for the inactivation. It is assumed that the thiol protection is an effect of formation of the reversible protein mixed disulfide by urease with the thiol protector. Urease in this reversible complex is accessible for substrate in contrast to complex urease-DCNQ.
Figure 3. Thiol influence on urease inactivation by DCNQ relative to the control activity. The percent of the enzyme activity in the presence of DCNQ without the thiol is given for comparison. Concentration of the thiols: L-cysteine (L-cys), glutathione (GSH), dithiothreitol (DTT) and DCNQ in the incubation mixture was equal to 0.5 mM and 8 μM, respectively. Enzyme activity was determined after a 10 and 30 minutes incubation.
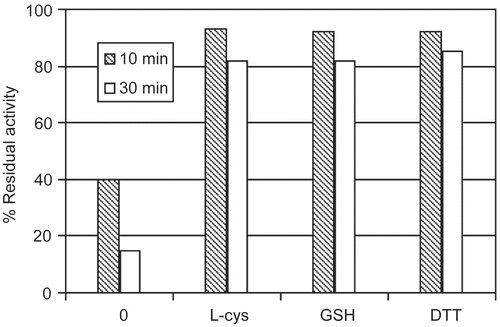
The protection effect of catalase in the incubation system is showed in . Catalase decreased the inactivation of approximately 75 ± 10% relatively to the unprotected system. Catalase similarly to thiols prevented urease against DCNQ inhibition. However, the way of the prevention is completely different. Catalase by the decomposition of H2O2 prevented urease thiols from oxidation by H2O2. The experiment directly proved the presence of H2O2 in the incubation mixture and indirectly showed the possible mechanism of the inactivation. Hydrogen peroxide is generated in the redox cycling with the use of quinone in the presence of reducing agent [Citation16,Citation28].
Figure 4. Catalase influence on urease inactivation by DCNQ. DCNQ concentration was equal to 5.5 and 11 μM.
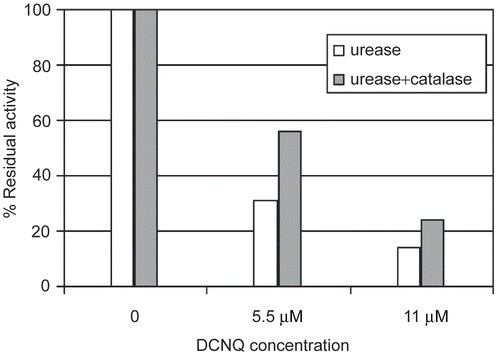
The quantity of H2O2 produced in the DCNQ-urease system was measured by titration with the use of KMnO4. The supplementary measurement with the presence of DTT in DCNQ solution estimated the activity of the reducing agents present in urease ().Our previous studies showed that H2O2 is an inactivator of urease with kinact= 0.114 min−1 [Citation16]. Hydrogen peroxide was identified as a mediator of protein-tyrosine phosphatase α (PTPα) inhibition by ortho-quinone inhibitors. The active site cysteine of PTPα was shown to be the target of oxidation by H2O2. The lower concentration of H2O2 (below 1 mM) produced reversible modification of the active site cysteine while the concentration above 1 mM irreversibly oxidized the catalytic cysteine to sulfinic and sulfonic acids [Citation28,Citation29]. In DCNQ-urease system H2O2 reached 0.7 mM concentration respective to reversible modification of catalytic cysteine to sulfenic acids (Equation 2).
Table 2. Estimation of hydrogen peroxide production by 7 μM DCNQ in 20 mM phosphate buffer pH 7.8, after a 60 min incubation.
The comparison of H2O2 produced after a 60 min incubation in 1) DCNQ-urease system and 2) DCNQ with 0.2 mM DTT, indicated that the activity of reducing agents presented in commercial urease (Sigma) corresponded to 0.2 mM DTT ().
Reactivation of DCNQ modified urease
The reactivation of DCNQ inactivated urease was studied by DTT application. Urease was incubated with 8 μM DCNQ until the reactivity of the enzyme decreased to 2.6% (after a 45 minutes incubation), then DTT was added into the incubation mixture. The enzyme quickly (1-2 minutes) regained activity to 70 ± 10%. Obtained results are presented in . The experiment indicated that DCNQ modification of urease followed two mechanisms that lead to reversible and irreversible modification of urease.
Conclusion
This study is a continuation of our previous research on inhibitory influence of quinones on urease [Citation15,Citation16,Citation23,Citation24]. The examined quinones represented two types of inhibition kinetics. The slow-binding kinetics was displayed by the benzoquinones: 1,4-benzoquinone (BQ), tetrachloro-1,4-benzoquione (TCpBQ), tetrachloro-1,2-benzoquione (TCoBQ), 2,5-dimethyl-1,4-benzoquinone (DMBQ). The inhibitory strength, based on the value of the overall inhibition constant, arranged the mentioned benzoquinones in the order:
TCpBQ > TCoBQ > BQ > DMBQ
The sequence confirmed the positive influence of a chloro-substituent on benzoquinone inhibitory effectiveness. The time-dependent inactivation kinetics showed 1,4-naphthoquinone (NQ) and presented in this paper 2,3-dichloro-1,4-naphthoquinone (DCNQ). Taking into account the parameters IC50, DCNQ was found to be four times stronger urease inactivator than NQ. The chloro-substituent in naphthoquinone also increased inhibitory power. Both naphthoquinones followed biphasic mode of the inactivation.
The inhibitory influence of quinones on enzymes has been suggested to be the result of at least two mechanisms. Direct interaction between enzyme and quinone leads to covalent modification of protein thiols and generation of thioethers. The result is irreversible inactivation of enzyme that cannot be restored by DTT application. The indirect mechanism is attributed to quinone ability to act as catalysts in the generation of reactive oxygen species (ROS) e.g. H2O2, O2·- [Citation20]. Created ROS can oxidize the catalytic thiols to sulfenic, sulfinic or sulfonic acids. Unstable sulfenic acid is susceptible for further reaction. It can react with externally implicated DTT or undergo further oxidation to sulfinic and sulfonic acids. DTT reduces sulfenic acid back to thiol group that finally results in restoration of enzyme activity. In contrast, sulfinic and sulfonic acids are irreversibly formed and cannot return to the initial thiol residue (Equation 2) [Citation20]:
Thiol protection experiment proved the significant role of thiols in DCNQ inactivation of urease. Moreover, the protection influence of catalase and measured the amount of hydrogen peroxide in the incubation mixture (< 1 mM) indicated on hydrogen peroxide as an inhibitory factor. This double mechanism was confirmed by the reactivation test. The application of DTT caused only partial (70 ± 10%) urease activity restoration. The irreversible inactivation was attributed to the arylation reaction between DCNQ and urease thiols as well as further oxidation of sulfenic acid. The arylation process between urease thiols (R-SH) and DCNQ is represented in Equation (3):
The thiols are able to substitute the subsequent chlorine and form di-conjugate. The multisubstitution was observed for the urease inhibition by TCpBQ. The investigations showed that one TCpBQ molecule reacts with 2.3 ± 0.2 thiol groups [Citation15]. This interaction causes strong conformational changes in enzyme molecule enclosing subunits. The effect is an intensive decrease of enzymatic activity. The similar stoichiometry for reaction DCNQ with urease cannot be excluded. Multisubstitution chlorine in TCBQ by thiol group of cysteine and hydroxyl groups of tyrosines was observed by Ploemen at al. in the system with glutathione S-transferase and the quinone [Citation30]. Comparison of participation of the fast and slow phases in the inactivation with the percentage of DTT reactivation leads to the conclusion that the arylation mechanism was related to the fast phase, and the slow phase was caused by the redox cycling.
Acknowledgements
Declaration of interest: The authors report no conflicts of interest. The authors alone are responsible for the content and writing of the paper.
References
- Mobley HLT, Hausinger RP. Microbiol Rev 1989; 53:85.
- Sirko A, Brodzik R. Acta Biochim 2000; 47:1189.
- Follmer C. Phytochemistry 2008; 69:18.
- Benini S, Rypniewski WR, Wilson KS, Miletti S, Ciurli S, Mangani S. Structure 1999; 7:205.
- Norris R, and Brocklehurst K. Biochem J 1976; 159:245.
- Mamiya G, Takishima K, Masakuni M, Kayumi T, Ogawa K, Sekita T. Proc Jap Acad 1985; 61:395, Ser B.
- Blakeley RL, Zerner B. J Mol Cat 1984; 23:263.
- Mobley HLT, Island MD, Hausinger RP. Microbiol Rev 1995; 59:451.
- Burne RA, Chen Y-YM. Microbe and Infect 2000; 2:533.
- Ha NCh, Oh ST, Sung JY, Cha KA, Lee MH, Oh BH. Nature-Structural Biology 2001; 8:505.
- Juszkiewicz A, Zaborska A, łaptas´ A, Olech Z. Food Chem 2004; (85):553.
- Mulvaney RL, Bremner JM. Soil Biochem 1981; 5:153.
- Bundy LG, Bremner JM. Soil Biol Biochem 1973; 5:847.
- Ashiralieva A, Kleiner D. FEBS Letters 2003; 555:367.
- Zaborska W, Krajewska B, Kot M, Karcz W. Bioorg Chem 2007; 35:233.
- Krajewska B, Zaborska W. Bioorg Med Chem 2007; 15:4144.
- Clark AM, Tannis MJ, Hufford CD. Phytotherapy Res 1990; 4:11.
- Inbaraj JJ, Chingnel CF. Chem Res Toxicol 2004; 17:55.
- Lukens RJ. Chemistry of fungicidal action, 10 in Molecular, Biochemistry, Biophysics Series. Verlag: Springer; 1971.
- Bolton JL, Trush MA, Penning TM, Dryhurst G, Monks TJ. Chem Res Toxicol 2000; 13:135.
- Rodriguez CE, Fukuto JM, Taguchi K, Froines J, Cho AK. Chem Biol Inter 2005; 155:97.
- O’Brien PJ. Chem Biol Interact 1991; 80:1
- Zaborska W, Kot M, Superata K. J Enz Med Chem 2002; 17:247.
- Kot M, Zaborska W. J Enz Med Chem 2006; 21 (5):537.
- Kot M. J Enz Med Chem 2006; 21 (6):697.
- Weatherburn MW. Anal Chem 1967; 39:971.
- Srivastava PK, Kayastha AM. Plant Sci 2000; 159:149.
- Bova MP, Mattson MN, Vasile S, Tam D, Holsinger L, Bremer M, Hui T, McMahon G, Rice A, Fukuto JM. Arch Biochem Biophys 2004; 429:30.
- Salmeen A, Andersen JN, Myers MP, Tzu-Ching Meng, Hinks JA, Tonks NK, Barford D. Nature 2003; 423:769.
- Ploemen JHTM, Johnson WW, Jespersen S, Vanderwall D, Ommen B, Greef J, Bladeren PJ, Armstrong RN. J Biol Chem 1994; 269:26890.