Abstract
The selective inhibition of PTP1B has been widely recognized as a potential drug target for the treatment of type 2 diabetes and obesity. In the course of screening for PTP1B inhibitory natural products, the MeOH extract of the dried sample of the Antarctic lichen Umbilicaria antarctica was found to exhibit significant inhibitory effect, and the bioassay-guided fractionation and purification afforded three related lichen metabolites 1-3. Compounds 1-3 were identified as gyrophoric acid (1), lecanoric acid (2), and methyl orsellinate (3) mainly by analysis of NMR and MS data. These compounds inhibited PTP1B activity with 50% inhibitory concentration values of 3.6 ± 0.04 μM, 31 ± 2.7 μM, and 277 ± 8.6 μM, respectively. Furthermore, the kinetic analysis of PTP1B inhibition by compound 1 suggested that the compound inhibited PTP1B activity in a non-competitive manner.
Introduction
Protein tyrosine phosphatase 1B (PTP1B) is an intracellular phosphatase shown to be implicated in the negative regulation of insulin signaling by dephosphorylating the insulin receptor (IR) as well as its substrate, insulin receptor substrate-1 (IRS-1) and insulin receptor substrate-2 (IRS-2) [Citation1–3]. The protein levels are increased in insulin-resistant diabetes patients and the deletion of PTP1B in mice has been shown to increase insulin sensitivity [Citation4–Citation6]. In supporting this observation, small molecule inhibitors of PTP1B work synergistically with insulin to increase signaling and augment insulin-stimulated glucose uptake [Citation7, Citation8]. In addition to the role of PTP1B in insulin signaling, several genetic and biochemical studies indicate that PTP1B is also implicated in the inhibition of leptin signaling, which inhibits food intake and promotes energy expenditure [Citation9–11]. In agreement, recent study showed that body weight, adiposity and leptin action can be regulated by neuronal PTP1B [Citation12]. Taken together, PTP1B has emerged as one of well-validated drug targets for the treatment of both diabetes and obesity. Recognition of PTP1B as a novel drug target has led to a number of studies on the designing and development of synthetic PTP1B inhibitors [Citation13, Citation14]. Several classes of plant-derived secondary metabolites have been also described as PTP1B inhibitors [Citation14].
Lichens, comprising about 17,000 species, are symbiotic associations formed through symbiosis between fungi and algae and/or cyanobacteria [Citation15]. They produce secondary metabolites that are sometimes suggested to serve as antimicrobial or antiherbivore agents [Citation16–Citation18]. Several lichen extracts have been used for various remedies in folk medicine, and a variety of biological activities of lichen metabolites, including antibiotic, antimycobacterial, antiviral, analgesic, and antipyretic properties, have been indicated by screening processes [Citation15, Citation18]. Thus, there is considerable interest in lichen metabolites as potential sources of pharmacological agents [Citation19].
In the course of continuing search for PTP1B inhibitory metabolites from Antarctic lichens and mosses, [Citation20–22] the MeOH extract of the dried sample of Umbilicaria antarctica was selected for further bio-assay guided investigation based on the observation of significant PTP1B inhibitory (> 90%) effect at the concentration of 30 μg/mL, and this report describes the isolation, structure elucidation, and biological activities of compounds 1–3 ().
Materials and methods
General experimental procedures
ESIMS data were obtained using a API-2000 ESI-MS instrument (Applied Bio system, USA) at Pukyung Nat’l University, Korea. NMR spectra (1D and 2D) were recorded in DMSO-d6, CD3OD, and acetone-d6 using a JEOL JNM ECP-400 spectrometer (400 MHz for 1H and 100 MHz for 13C), and chemical shifts were referenced relative to the respective residual solvents (δH/δC = 2.49/39.5 for DMSO-d6; δH/δC = 3.30/49.0 for CD3OD; δH/δC = 2.04/29.8 for acetone-d6). HMQC and HMBC experiments were optimized for 1JCH = 140 Hz and nJCH = 8 Hz, respectively. Solvents for extractions and flash column chromatography were reagent grade and used without further purification. Solvents used for HPLC were analytical grade. Flash column chromatography was carried out using YMC octadecyl-functionalized silica gel (ODS-A, 75-μm particle size). HPLC separations were performed on a Shiseido Capcell Pak® C18 column (10 × 250 mm; 5-μm particle size) with a flow rate of 2 mL/min. Compounds were detected by UV absorption at 254 nm.
Specimen collection and identification
Umbilicaria antarctica was collected and identified by one of us (J. H. Yim) from Barton Peninsular around King Sejong Station (62°13′97″S/58°46′93″W) on King George Island, Antarctica in January, 2003. Voucher specimens (reference L19) have been deposited in the Korea Polar Research Institute.
Isolation and characterization of compounds 1-3
A dried sample of U. antarctica (10 g) was extracted with MeOH (300 mL × 2) for 24 h. The resulting crude MeOH extract (1.1 g) was subjected to C18-functionalized silica gel flash column chromatography (3 × 15 cm), eluting with a stepwise gradient of 20%, 40%, 60%, 70%, 80%, 90%, and 100 % (v/v) MeOH in H2O (400 mL each). The fraction eluted at 80 % MeOH (49.5 mg) was then subjected to semi-preparative reversed-phase HPLC using a gradient from 40 to 75% CH3CN in H2O (0.1% formic acid) over 35 min to yield 3 (5.9 mg; tR = 21 min), 2 (3.1 mg; tR = 23 min), and 1 (13.1 mg; tR = 34 min).
Gyrophoric acid (1).
White amorphous powder; ESIMS m/z 467 [M – H]−; 1H-NMR (400 MHz, DMSO-d6) δ: 6.24 (1H, br s, H-3), 6.23 (1H, br s, H-5), 6.67 (1H, br s, H-3’), 6.66 (1H, br s, H-5’), 6.57 (1H, br s, H-3’’), 6.55 (1H, br s, H-5’’), 2.35 (3H, s, H-8), 2.36 (3H, s, H-8’), 2.40 (3H, s, H-8’’), 10.35 (2-OH), 10.49 (2’-OH), 10.02 (2’’-OH); 13C-NMR (100 MHz, DMSO-d6) δ 109.1 (C-1), 160.5 (C-2), 101.1 (C-3), 161.6 (C-4), 110.3 (C-5), 140.7 (C-6), 167.7 (C-7), 21.8 (C-8), 118.7 (C-1’), 156.8 (C-2’), 107.8 (C-3’), 152.7 (C-4’), 114.6 (C-5’), 138.5 (C-6’), 166.2 (C-7’), 19.9 (C-8’), 177.8 (C-1’’), 160.5 (C-2’’), 107.7 (C-3’’), 152.5 (C-4’’), 114.8 (C-5’’), 140.5 (C-6’’), 171.2 (C-7’’), 21.8 (C-8’’).
Lecanoric acid (2).
White amorphous powder; ESIMS m/z 317 [M – H]−; 1H-NMR (400 MHz, DMSO-d6) δ: 6.28 (1H, br s, H-3), 6.21 (1H, br s, H-5), 6.60 (1H, br s, H-3’), 6.57 (1H, br s, H-5’), 2.35 (3H, s, H-8), 2.44 (3H, s, H-8’), 10.39 (2-OH), 10.03 (2’-OH); 13C-NMR (100 MHz, DMSO-d6) δ 108.8 (C-1), 160.8 (C-2), 101.0 (C-3), 161.5 (C-4), 110.4 (C-5), 140.9 (C-6), 167.9 (C-7), 22.0 (C-8), 117.2 (C-1’), 160.8 (C-2’), 107.9 (C-3’), 152.0 (C-4’), 114.0 (C-5’), 140.9 (C-6’), 171.6 (C-7’), 22.6 (C-8’).
Methyl orsellinate (3).
White amorphous powder; ESIMS m/z 181 [M – H]−; 1H-NMR (400 MHz, DMSO-d6) δ: 6.19 (1H, br s, H-3), 6.14 (1H, br s, H-5), 2.43 (3H, s, H-8), 3.88 (3H, s, -OCH3); 13C-NMR (100 MHz, DMSO-d6) δ 108.0 (C-1), 161.6 (C-2), 101.0 (C-3), 161.8 (C-4), 110.8 (C-5), 141.2 (C-6), 170.8 (C-7), 22.6 (C-8), 52.3 (-OCH3).
Methylation of gyrophoric acid (1)
To a solution of gyrophoric acid (1) (2.6 mg) in 1 mL of MeOH was added 10 μL of a 2 M solution of trimethylsilyldiazomethane (TMSCHN2) in hexane. After confirming the disappearance of the starting material by TLC analysis (i.e., after stirring for 10 min), the reaction mixture was concentrated, and subjected to semi-preparative reversed-phase HPLC using a using a gradient from 50 to 81% CH3CN in H2O (0.1% formic acid) over 37 min to yield 4 (1.0 mg; tR = 37 min). The structure of compound 4 was confirmed by analysis of 1H NMR and MS data.
Methyl gyrophoriate (4).
White amorphous powder; ESIMS m/z 481 [M – H]− 1H-NMR (400 MHz, acetone-d6) δ 6.89 (1H, d, J = 2.1 Hz, Ar-H), 6.86 (2H, br s, Ar-H), 6.82 (1H, d, J = 2.1 Hz, Ar-H), 6.40 (1H, d, J = 2.1 Hz, Ar-H), 6.31 (1H, d, J = 2.1 Hz, Ar-H), 4.00 (3H, s, OCH3), 2.66 (3H, s, Ar-CH3), 2.61 (3H, s, Ar-CH3), 2.57 (3H, s, Ar-CH3).
Assay procedures
PTP1B (human, recombinant) was purchased from BIOMOL Research Laboratories, Inc. The enzyme activity was measured in a reaction mixture containing 2 mM p-nitrophenyl phosphate (pNPP) in 50 mM citrate, pH 6.0, 0.1 M NaCl, 1 mM EDTA and 1mM dithiothreitol (DTT). The reaction mixture was placed in a 37 °C incubator for 30 min, and the reaction was terminated by addition of 10 N NaOH. The amount of produced p-nitrophenol was estimated by measuring the increase in absorbance at 405 nm. The non-enzymatic hydrolysis of 2 mM pNPP was corrected by measuring the increase in absorbance at 405 nm obtained in the absence of PTP1B enzyme [Citation24].
Kinetic analysis
The reaction mixture consisted of different concentrations of pNPP as a PTP1B substrate in the absence or presence of compound 1, and assayed as described above. Data were fitted by nonlinear regression analysis according to a Michaelis-Menten kinetic model (Graphpad Prism version 4.02).
Results and discussion
To isolate and identify the PTP1B inhibitory active prin-ciple(s) from the MeOH extract of the sample of U. antarctica, we performed bioassay and 1H-NMR guided fractionation and purification by using C18-functionalized silica gel column chromatography and HPLC, which led to the isolation of three related lichen metabolites (compounds 1-3). The isolated compounds were identified as gyrophoric acid (1), lecanoric acid (2), and methyl orsellinate (3) by analysis of NMR and MS data, together with comparison of their spectral data with those in the literature [Citation23]. Compounds 1-3 inhibited the hydrolysis of the pNPP catalyzed by PTP1B in a dose-dependent manner () with IC50 values of 3.6 ± 0.04 μM, 31 ± 2.7 μM, and 277 ± 8.6 μM, respectively. A known PTP1B inhibitor, ursolic acid (IC50 = 2.5 ± 0.5 μM) was used as a positive control in the assay [Citation24, Citation25]. Even though compounds 1-3 share the similar structural pattern with the monomeric hydroxylbenzoic acid, only compound 1 showed potent inhibitory effect comparable to that of the positive control. In addition, carboxylic acid moiety in compound 1 was converted to the corresponding methyl ester (compound 4) to evaluate the significance of the carboxylic acid functionality in the compound for exerting the inhibitory effect on PTP1B activity. In the assay for the determination of PTP1B inhibitory potency, compound 4 appeared to sustain the inhibitory activity (IC50 = 14.1 ± 0.04 μM), but the potency was decreased about 4-fold compared to that of compound 1. Therefore, it was suggested that the acid functionality in 1 was crucial to exhibiting the inhibitory activity. However, based on the results showing compound 1 as the only potent PTP1B inhibitor despite of the fact that both compounds 1 and 2 commonly have the carboxylic acid functionality, it could be suggested that the molecular size could be an additional important factor for the inhibition of PTP1B activity among these compounds. To investigate the inhibition mode of the most potent inhibitor (i.e., compound 1) on the activity of PTP1B, substrate titration studies using the small substrate pNPP were conducted. As shown in , compound 1 decreased the Vmax value, but didn’t alter the Km value of PTP1B, suggesting that the inhibition mode was non-competitive toward pNPP. Several non-competitive inhibitors of PTP1B have been shown to interact with an allosteric site of PTP1B [Citation18], and the allosteric modulation of PTP1B activity has been suggested to be a promising approach for developing selective inhibitors [Citation7, Citation26].
Figure 2. Dose-dependent inhibition of compound 1 on PTP1B enzyme activity. The means with S.D. of three independent determinations are shown.
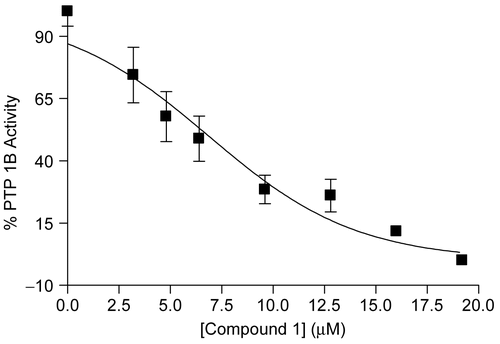
Figure 3. Non-competitive inhibitor of PTP1B. (a) Substrate titration reveals that compound 1 is a classical non-competitive inhibitor that inhibits substrate catalysis (Vmax), but not substrate binding (constant Km). (b) Plots of Vmax and Km as a function of concentration of compound 1. Values were derived from nonlinear regression analysis in (a).
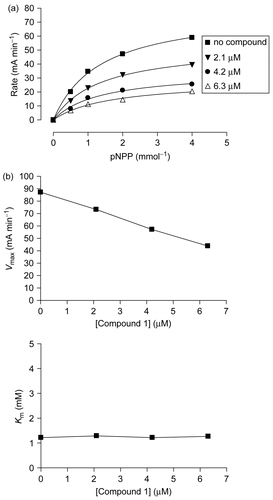
Depsides (e.g., compound 2) and tridepsides (e.g., compound 1) are one of distinctive types of lichen metabolites formed by condensation of two or more hydroxybenzoic acids (e.g., compound 3) through the esterification steps between the carboxylic acid and a phenolic hydroxyl group [Citation18]. Lichen depsides have been described to show various biological effects such as inhibitions of 5-lipoxygenase and prostaglandin biosynthesis [Citation18, Citation27]. On the other hand, tridepside gyrophoric acid has been shown to have antiproliferative activity against human keratinocyte growth [Citation28]. However, the PTP1B inhibitory effects by the member of this class have not been previously reported. Further studies with this class of compounds with the aim of providing selectivity against other PTP panels as well as in vivo efficiency are necessary to evaluate their potential as lead compounds in the treatment of diabetes and obesity.
Acknowledgements
This work was supported by a grant from the KOPRI Project (PE08050).
Declaration of interest: The authors report no conflicts of interest.
References
- Kenner KA, Anyanwu E, Olefsky JM, Kusari J. Protein-tyrosine phosphatase 1B is a negative regulator of insulin- and insulin-like growth factor-I-stimulated signaling. J Biol Chem 1996; 271: 19810–19816.
- Seely BL, Staubs PA, Reichart DR, Berhanu P, Milarski KL, Saltiel AR, Kusari J, Olefsky JM. Protein tyrosine phosphatase 1B interacts with the activated insulin receptor. Diabetes 1996; 45: 1379–1385.
- Calera MR, Vallega G, Pilch PF. Dynamics of protein-tyrosine phosphatases in rat adipocytes. J Biol Chem 2000; 275: 6308–6312.
- Ahmad F, Azevedo JL, Cortright R, Dohm GL, Goldstein BJ. Alterations in skeletal muscle protein-tyrosine phosphatase activity and expression in insulin-resistant human obesity and diabetes. J Clin Invest 1997; 100: 449–458.
- Elchebly M, Payette P, Michaliszyn E, Cromlish W, Collins S, Loy A L, Normandin D, Cheng A, Himms-Hagen J, Chan CC, Ramachandran C, Gresser MJ, Tremblay ML, Kennedy BP. Increased insulin sensitivity and obesity resistance in mice lacking the protein tyrosine phosphatase-1B gene. Science 1999; 283: 1544–1548.
- Klaman LD, Boss O, Peroni OD, Kim JK, Martino JL, Zabolotny JM, Moghal N, Lubkin M, Kim YB, Sharpe AH, Stricker-Krongrad A, Shulman GI, Neel BG, Kahn BB. Increased energy expenditure, decreased adiposity, and tissue-specific insulin sensitivity in protein-tyrosine phosphatase 1B-deficient mice. Mol Cell Biol 2000; 20: 5479–5489.
- Zhang S, Zhang Z-Y. PTP1B as a drug target: recent developments in PTP1B inhibitor discovery. Drug Discov Today 2007; 12: 373–381.
- Xie L, Lee S-Y, Anderson JN, Waters S, Shen K, Guo XL, Moller NPH, Olefsky JM, Lawrence DS, Zhang Z-Y. Cellular effects of small molecule PTP1B inhibitors on insulin signaling. Biochemistry 2003; 42: 12792–12804.
- Zabolotny JM, Bence-Hanulec KK, Stricker-Krongrad A, Haj F, Wang Y, Minokoshi Y, Kim YB, Elmquist JK, Tartaglia LA, Kahn BB, Neel BG. PTP1B regulates leptin signal transduction in vivo. Dev Cell 2002; 2: 489–495.
- Kaszubska W, Falls HD, Schaefer VG, Haasch D, Frost L, Hessler P, Kroeger P E, White DW, Jirousek MR, Trevillyan JM. Protein tyrosine phosphatase 1B negatively regulates leptin signaling in a hypothalamic cell line. Mol Cell Endocrinol 2002; 195: 109–118.
- Myers MG Jr. Leptin receptor signaling and the regulation of mammalian physiology. Recent Prog Horm Res 2004; 59: 287–304.
- Morrison CD, White CL, Wang Z, Lee SY, Lawrence DS, Cefalu WT, Zhang ZY, Gettys TW. Increased hypothalamic protein tyrosine phosphatase 1B contributes to leptin resistance with age. Endocrinology 2007; 148: 433–440.
- Johnson TO., Ermolieff J, Jirousek MR. Protein tyrosine phosphatase 1B inhibitors for diabetes. Nat Rev Drug Discov 2002; 1: 696–709.
- Lee S, Wang Q. Recent development of small molecular specific inhibitor of protein tyrosine phosphatase 1B. Med Res Rev 2007; 27: 553–573.
- Huneck S. The significance of lichens and their metabolites. Naturwissenschaften 1999; 86: 559–570.
- Ingólfsdóttir K. Usnic acid. Phytochemistry 2002; 61: 729–736.
- Lohezic-Le Devehat F, Tomasi S, Elix JA, Bernard A, Rouaud I, Uriac P, Boustie J. Stictic acid derivatives from the lichen Usnea articulata and their antioxidant activities. J Nat Prod 2007; 70: 1218–1220.
- Kumar KCS., Műller K. Lichen metabolites. 1. Inhibitory action against leukotriene B4 biosynthesis by a non-redox mechanism. J Nat Prod 1999; 62: 817–820.
- Ernst-Russell MA, Chai CLL, Wardlaw JH, Elix JA. Euplectin and coneuplectin, new naphthopyrones from the lichen Flavoparmelia euplecta. J Nat Prod 2000; 63: 129–131.
- Seo C, Choi YH, Sohn JH, Ahn JS., Yim JH, Lee HK, Oh H. Ohioensins F and G: protein tyrosine phosphatase 1B inhibitory benzonaphthoxanthenones from the Antarctic moss Polytrichastrum alpinum. Bioorg Med Chem Lett 2008; 18: 772–775.
- Seo C., Yim JH, Lee HK, Park SM, Sohn JH, Oh H. Stereocalpin A, a bioactive cyclic depsipeptide from the Antarctic lichen Stereocaulon alpinum. Tetrahedron Lett 2008; 49: 29–31.
- Seo C., Sohn JH., Park SM, Yim JH, Lee HK, Oh H. Usimines A-C, bioactive usnic acid derivatives from the Antarctic lichen Stereocaulon alpinum. J Nat Prod 2008; 71: 710–712.
- Narui T, Sawada K, Takatsuki S, Okuyama T, Culberson CF, Culberson WL, Shibata S. NMR assignments of depsides and tridepsides of the lichen family Umbilicariaceae. Phytochemistry 1998; 48: 815–822.
- Na M, Tang S, He L, Oh H, Kim BS, Oh WK, Kim BY, Ahn JS. Inhibition of protein tyrosine phosphatase 1B by ursane-type triterpenes isolated from Symplocos paniculata. Planta Med 2006; 72: 261–263.
- Zhang W, Hong D, Zhou Y, Zhang Y, Shen Q, Li J, Hu L, Li J. Ursolic acid and its derivative inhibit protein tyrosine phosphatase 1B, enhancing insulin receptor phosphorylation and stimulating glucose uptake. Biocheim Biophy Acta 2006; 1760: 1505–1512.
- Wiesmann C, Barr KJ, Kung J, Zhu J, Erlanson DA, Shen W, Fahr BJ, Zhong M, Taylor L, Randal M, McDowell RS, Hansen SK. Allosteric inhibition of protein tyrosine phosphatase 1B. Nat Struct Mol Biol 2004; 11: 730–737.
- ögmundsdóttir HM, Zoega GM, Gissurarson SR, Ingólfsdóttir K. Anti-proliferative effects of lichen-derived inhibitors of 5-lipoxygenase on malignant cell-lines and mitogen-stimulated lymphocytes. J Pharm Pharmacol 1998; 50: 107–115.
- Kumar KC S, Műller K. Lichen metabolites. 2. Antiproliferative and cytotoxic activity of gyrophoric, usnic, and diffractaic acid on human keratinocyte growth. J Nat Prod 1999; 62: 821–823.