Abstract
Tiliroside was found to inhibit both monophenolase and diphenolase activity of mushroom tyrosinase. The lag time of tyrosine oxidation catalyzed by mushroom tyrosinase was obviously lengthened; 0.337 mM of tiliroside resulted in the lag time extension from 46.7 s to 435.1 s. A kinetic analysis shown that tiliroside was a competitive inhibitor for monophenolase and diphenolase with Ki values of 0.052 mM and 0.26 mM, respectively. Furthermore, tiliroside showed 34.5% (p < 0.05) inhibition of intracellular tyrosinase activity and 54.1% (p < 0.05) inhibition of melanin production with low cytotoxicity on B16 mouse melanoma cells at 0.168 mM. In contrast, arbutin displayed 9.1% inhibition of cellular tyrosinase activity and 29.5% inhibition of melanin production at the same concentration. These results suggested that tiliroside was a potent tyrosinase inhibitor and might be used as a skin-whitening agent and pigmentation medicine.
Introduction
Driven by rising people interest and demand for skin whitening, increasing researches have focused on screening skin whitening agents to suppress accumulation of melanin. Furthermore, the over production and accumulation of melanin pigment in the skin can result in a large number of skin problems including chloasoma dermatitis, freckles, and geriatric pigment spots [Citation1]. And they are also the common aesthetic problems. Melanin biogenesis, stimulated by ultraviolet radiation, widely occurs in insects, animals and plants through free-radical mechanism [Citation2]. During the melanogenesis, tyrosinase (EC 1.14.18.1) containing a binuclear copper cluster is the key enzyme to catalyse the rate-limited steps of pigmentation [Citation3]: the hydroxylation of tyrosine to 3, 4- dihydroxyphenylalanine (DOPA) and the oxidation of DOPA to dopaquinone. Many chemicals from artificial or natural sources were reported as tyrosinase inihibitors to block melanogenic pathways. Apart from avoiding UV exposure, application of tyrosinase inhibitors may be the least invasive procedure for maintaining skin whiteness [Citation4]. Recently, tyrosinase inhibitors are becoming increasingly important in cosmetic products that promote skin-whitening [Citation5]. Many of products are being developed to control melanogenesis with the similar principles.
Natural tyrosinase inhibitors, such as the flavonoids, are generally considered to be free of harmful side effects [Citation6, Citation7] and show significant inhibition of tyrosinase activity [Citation8]. Raspberry, the fruit of Rubus chingii Hu (Rosaceae, Rubus), has been used traditionally to treat impotence, prospermia, enuresis with renal asthenia and gonobolia [Citation9]. Tiliroside, as the acylated flavonoid of Rubus species [Citation10], possessed anti-oxidative [Citation11], anti-inflammation [Citation12], anti-microbial activity [Citation13], UV-protectant [Citation14] and cytotoxic activity against human leukaemic cell lines [Citation15].
In addition, tiliroside was reported to show inhibitory effect on diphenolase activity of tyrosinase [Citation16]. However, the inhibition mechanism on tyrosinase, and the medicinal effect on B16 mouse melanoma cells (B16 cells) remained unclear. Further works are necessary to provide more biological evidence before the application of tiliroside. In our works, tiliroside was first purified and characterized from raspberry. The inhibitory effect on monophenolase activity by tiliroside was investigated. And the kinetic study of the inhibition of the monophenolase and diphenolase activity of tyrosinase by tiliroside was carried out. Furthermore, its inhibitory effect on tyrosinase- and melanin-reducing activities in B16 cells was also investigated as intracellular evidence.
Materials and methods
Chemicals
Tyrosinase from mushroom (EC 1.14.18.1, T3824, tyrosinase activity 5460 U/mg solid) was purchased from Sigma-Aldrich (St. Louis, USA). DMEM Medium (high glucose) was from GIBCOBRL, Grand Island (NewYork, USA), tyrosine, L-3,4-dihydroxyphenylalanine (L-DOPA) from Qiude biotech Ltd. (Shanghai, China), Fetal bovine serum (FBS) from Hangzhou Sijiqing Co., Ltd. (Hangzhou, China), 3-(4, 5-dimethylthiazol-2-yl)-2, 5-diphenyl tetrazolium bromide (MTT) from Bioseen Technology, Inc. (Shanghai, China), Triton X-100 from Xitang biotech Ltd. (Shanghai, China) and dimethyl sulfoxide (DMSO, analytical grade) from Shanghai Chemical Co. (Shanghai, China). Tiliroside was isolated and purified in our lab with individual purity no less than 98% (HPLC assay, UV detection). The water used was re- distillated and ion-free.
Extraction and isolation of tiliroside
Tiliroside was isolated from the ethanol extract of raspberry. Briefly, dried raspberry (10 kg) was extracted with 95% ethanol three times at room temperature. The ethanol extract was concentrated under reduced pressure. Then, the suspension of the extract in deionized water was liquid-liquid extracted by petroleum ether and acetic ether in sequence. The acetic ether phase (170 g) was fractionated by silica gel column chromatography and eluted with CHCl3-MeOH using gradient elution (100:0; 50:1; 20:1; 12:1; 5:1; 3:1; 2:1; 0:1). A total of 106 Frs. (500 mL each) were collected. The combined fraction (Frs. 52-61) was further purified by using Sephadex LH-20 eluted with methanol twice, and then recrystalized in methanol to finally obtain tiliroside (232 mg). Spectral dates were identical to the previously report [Citation17]. Its individual purity was no less than 98% (HPLC assay, UV detection).
Assay of extracellular tyrosinase activity
The monophenolase and diphenolase activity assay was performed with modification as reported by Chen et al. [Citation18]. Tyrosine was used as substrate in the monophenolase activity assay, and L-DOPA in the diphenolase activity assay, respectively. Tiliroside was first dissolved in DMSO at 33.65 mM and then diluted to different concentrations with DMSO. The reaction media (200 μL) for activity assay adapted to a 96-well plate: each sample (40 μL ) was first added to the 96-well plate, and then 130 μL 0.5 mM tyrosine or L-DOPA in 50 mM Na2HPO4–NaH2PO4 buffer (pH 6.6) was added to the reaction system. At last, 30 μL mushroom tyrosinase was added to start the enzymatic reaction. The final concentration of mushroom tyrosinase was 1.334 μg/mL for the monophenolase activity and 3.000 μg/mL for the diphenolase activity. The test mixture was incubated for 20 min at 30 °C and absorbance at 490 nm was measured by a Sunrise absorbance microplate reader (Tecan Trading AG, Switzerland). The absorbance of the same mixture without tiliroside was used as blank control. The inhibition type was assayed by the Lineweaver-Burk plot, and the inhibition constant (Ki) was determined by the slope versus the concentration of the inhibitor [Citation19]. Activity of mushroom tyrosinase was calculated as % relative activity = B/A×100, where A = ΔOD490nm in 20 min without tiliroside and B = ΔOD490nm in 20 min with tiliroside.
Cell culture
The B16 cells were purchased from Chinese Type Culture Collection (Shanghai Institute of Cell Biology, Chinese Academy of Science, Shanghai, China). The cells were cultured in complete DMEM medium (high glucose) containing 10% dialyzed heat inactivated FBS, penicillin (100 U/mL) and streptomycin (100 U/mL) in a humidified atmosphere with 5% CO2 at 37 °C.
Assay of cell viability
The cell viability of B16 cells was determined using the MTT method [Citation20]. The cells were plated at a density of 1 × 103 cells/well (96-well plates) and cultured for 12 h. Tiliroside was added and incubated for 72 h. The plates were read with an automated Bio-Rad 550 microtiter plate reader (Bio-Rad Laboratories, CA, USA) at 570 nm for the reading and at 655 nm for the reference wavelength. 0.5% DMSO medium was used as negative control and arbutin was used as positive reference.
Assay of intracellular tyrosinase activity
Tyrosinase activity was measured as reported previously [Citation21, Citation22], with slight modification. Briefly, the cells were plated at a density of 1 × 103 cells/well (96-well plates), and then treated with tiliroside for 72 h. The cells were washed with potassium phosphate buffered saline (PBS, pH 6.8) twice and lysed with PBS containing 1% Triton X-100. The cells were shaken for 5 min, then frozen at −20 °C for 2 h, and disrupted by thawing at room temperature. At last, 100 μL of 0.5 mM L-Dopa was added to a 96-well microplate. After incubation at 37 °C for 2 h, the absorbance of samples was measured at 490 nm with automated Bio-Rad 550 microtiter plate reader. Arbutin was used as reference. The percentage of inhibition was calculated as follows: % inhibition = (A−B) / A × 100. A: OD at 490 nm with 0.5% DMSO medium; B: OD at 490 nm with test sample.
Assay of melanin content of melanocytes
The assay was followed as reported previously [Citation23, Citation24, Citation25], with slight modification. The B16 cells were plated at a density of 1 × 103 cells/well (96-well plates) and treated with tiliroside for 72 h. After 3-day culturing, the cells were washed with PBS for twice. 100 μL of ethanol-ethyl ether (1:1) were added to 96-well microplate in order to dissolve the insolubilities. Then, the microplate was kept at room temperature for 15 min. After the organic solvents were removed, cell pellets were dissolved in 150 μL 1 M NaOH−10% DMSO solution (v/v) at 80 °C for 3 h, and then kept at 37 °C overnight. The absorbance was measured at 405 nm using a Bio-Rad 550 microtiter plate reader. 0.5% DMSO medium was used as negative control and arbutin was used as positive reference.
Statistical analysis
All the experiments were run at least three times. SPSS 13.0 (SPSS Inc., Chichago, USA) was used for all the statistical analysis: all experiment data were presented by their calculated means and standard deviation in descriptive statistical analysis. The comparison between groups was complemented by method of pairwise t-test. Values of p <0.05 were considered to be significant.
Results and discussion
The effect of tiliroside on monophenolase activity of mushroom tyrosinase
The different concentration of tiliroside was examined for its effect on the monophenolase activity of mushroom tyrosinase on substrate, tyrosine. The kinetic course of the oxidation of tyrosine in the presence of different concentrations of tiliroside was shown in . As a characteristic of monophenolase activity, there was a lag period occurred simultaneously to the appearance of the stable product, dopachrome. The reaction system reached a steady-state rate after the lag period, which was estimated by extrapolation of the linear portion of the product accumulation curve to the abscissa [Citation26]. The lag period increased with increasing of concentration of tiliroside. When the concentrations of tiliroside were 0, 0.021, 0.042, 0.084, 0.168, 0.337 mM, the lag times were 46.7, 129.3, 174.4, 248.8, 339.5, 435.1 s, respectively. When the concentration of tiliroside increased from 0 to 0.337 mM, the lag time was prolonged from 46.7 to 435.1 s, which lengthened about ten times. On contrary, the steady-state rates which were the slopes of the line parts of the process courses, decreased with increasing of concentration of tiliroside. The result showed that tiliroside inhibited the monophenolase activity and lengthened the lag phase. In the catalysis of tyrosinase, there were three existing forms: Emet (met-tyrosinase), Eoxy (oxy-tyrosinase) and Edeoxy (deoxy-tyrosinase). Eoxy can catalyze both diphenol substrates and monophenol substrates while Emet can only catalyze diphenol substrates [Citation27, Citation28]. When Emet form binds with monophenol, the system will produce the dead-end complex EmetM [Citation29]. Tiliroside might combine with Emet to form dead-end complex, which resulted in the prolongation of lag time. In addition, the inhibitory effect could be explained by the structural similarity between inhibitor and tyrosinase [Citation30]. Tiliroside and the amino acid tyrosine () have a similar skeleton, which possibly explained the inhibitory effect of tiliroside on monophenolase.
Figure 1. Process curves for the inhibition of monophenolase of mushroom tyrosinase by tiliroside. Tyrosine was a substrate. The reaction was done in 50 mM Na2HPO4-NaH2PO4 buffer, pH 6.6, at 30 °C in the presence of different concentrations of tiliroside for curves 1–6 were 0, 0.021, 0.042, 0.084, 0.168 and 0.337 mM, respectively. The final concentration of mushroom tyrosinase was 14.934 μg/mL.
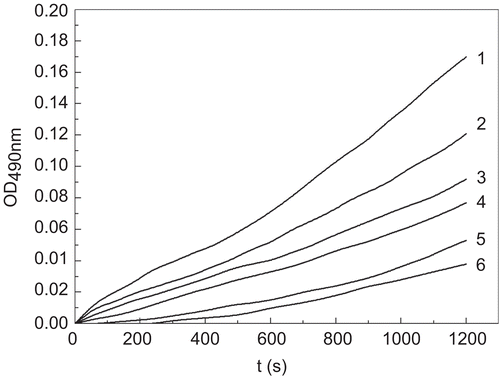
Inhibition type and inhibition constant of tiliroside on the monohenolase activity of mushroom tyrosinase
The steady-state rates of oxidation reaction of tyrosine by the enzyme followed Michaelis-Menten kinetic. The kinetic studies of the enzyme by the plot of Lineweaver-Burk were shown in . Double-reciprocal Lineweaver-Burk plots for tiliroside yielded a family of straight lines intersecting nearly on the vertical axis. The values of Km increased with increasing inhibitor concentration. Tiliroside seemed to show competitive inhibitory activity. The plot obtained from different slopes versus inhibitor concentrations was shown in the inset of . It was used to estimate inhibition constant for free enzyme. The inhibition constant (Ki) was 0.052 mM. The Michaelis-Menten (Km) was determined to be 3.93 mM. The inhibition mechanism of tiliroside on the monophenolase activity of tyrosinase may also be explained by the structural similarity between tiliroside and the amino acid tyrosine (). They have a similar skeleton and thus possibly acting as a competitive substrate to tyrosine.
Figure 3. Lineweaver-Burk plots for inhibition of tiliroside on the oxidation of tyrosine by mushroom tyrosinase. Tyrosine was a substrate. The reaction was done in 50 mM Na2HPO4-NaH2PO4 buffer, pH 6.6 and at 30 °C in the presence of different concentrations of tiliroside for curves 1–5 was 0, 0.042, 0.084, 0.168 and 0.337 mM, respectively. The final concentration of mushroom tyrosinase was 14.934 μg/mL. The inset was the secondary plot of the slope versus concentration of inhibitor (tiliroside).
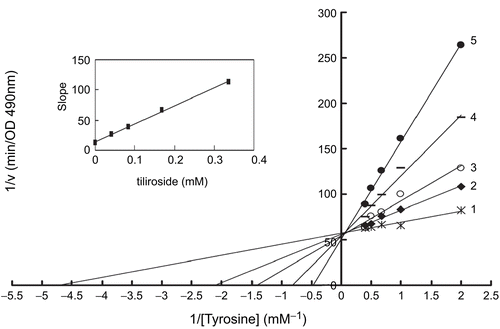
Inhibition type and inhibition constant of tiliroside on the diphenolase activity of mushroom tyrosinase
The kinetic behavior of mushroom tyrosinase during the oxidation of L-DOPA has been studied. In the presence of tiliroside, the kinetic studies of the enzyme by the plot of Lineweaver-Burk were shown in . The results showed that tiliroside was a competitive inhibitor because increasing the concentration resulted in a family of lines with a common intercept on the 1/v axis but with different slopes. According to the competitive inhibition, tiliroside inhibited the diphenolase activity of tyrosinase by combining with the active site of the enzyme. The oxidation reaction of L-DOPA conformed to Michaelis-Menten kinetics formula and the Michaelis-Menten (Km) was calculated as 1.28 mM. The plot obtained from different slopes versus inhibitor concentrations was shown in the inset of . It was used to estimate inhibition constant for free enzyme. The inhibition constant (Ki) was 0.26 mM. The Ki of arbutin for the diphenolase activity was 2.98 mM [Citation31]. It denoted that tiliroside was more effective inhibitor of the diphenolase activity than arbutin, had been reported to have inhibitory activity and widely used in cosmetic industry as whitening composition [Citation32, Citation33].
Figure 4. Lineweaver-Burk plots for inhibition of tiliroside on the oxidation of L-DOPA by mushroom tyrosinase. L-DOPA was a substrate. The reaction was done in 50 mM Na2HPO4-NaH2PO4 buffer, pH 6.6, at 30 °C in the presence of different concentrations of tiliroside for curves 1–5 was 0, 0.042, 0.084, 0.168 and 0.337 mM, respectively. The final concentration of mushroom tyrosinase was 3.000 μg/mL. The inset was the secondary plot of the slope versus concentration of inhibitor (tiliroside).
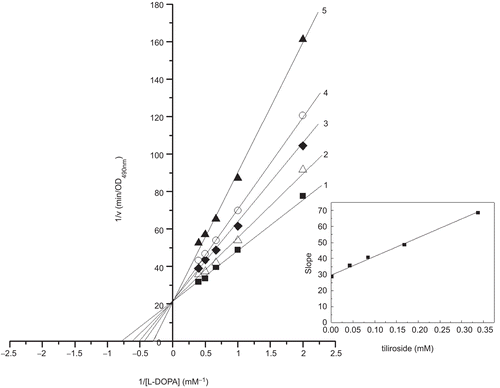
Noticeably, tiliroside (kaempferol-3-O-β-D-(6″-E-p-coumaroyl)-glucopyranoside) derives from kaempferol, which also proved to be a tyrosinase inhibitor, possessing the ability to chelate one copper in the binuclear activity center of tyrosinase [Citation34, Citation35, Citation36]. Kamepferol inhibits the enzyme competitively and the inhibition mechanism is a classical competitive type. The presence of hydroxyl groups at positions 5, 7 and the 4-oxo group in the tiliroside molecule can chelate copper in tyrosinase [Citation12]. In addition, the free hydroxyl groups helped to bind the activity site of tyrosinase. This, however, did not rule out the possibility that the structural formula of tiliroside was associated in part with this mechanism of inhibition.
MTT assay
The MTT assay was performed to evaluate the cytotoxicity of tiliroside on B16 cells. This cell viability test was aimed at finding a relative safe dosage for further intracellular determination of melanin formation in B16 cells. In this study, different concentrations of tiliroside were examined by the MTT assay. As shown in , tiliroside gave relatively lower cytotoxicity with cell viablity about 80% in 0.168 mM, which had similar effects with arbutin at the same concentration. Based on the results of the MTT test, tiliroside was further examined for inhibition of intracellular tyrosinase activity and melanin content.
Table 1. The cell viability of tiliroside and arbutin on B16 cells (Mean ± SD, %).
The inhibitory activities of tiliroside against tyrosinase and melanin biogenesis in B16 cells
Based on the results of the MTT test, the concentrations ranging from 0.011 to 0.168 mM of tiliroside were chosen for tyrosinase- and melanin-reducing activities. Arbutin was used as positive control. The results are shown in . The results suggested that tiliroside had inhibitory effect on intracellular tyrosinase activity and showed dose-dependent bioactivity, which was consistent with its extracellular activities. In contrast, arbutin, at the same level (0.168 mM), showed lower inhibition. When the reduced melanin content of tiliroside was examined, a similar dose-dependent inhibition was observed (). Treatment with tiliroside at a concentration of 0.168 mM resulted in a significant reduction in the melanin (54.1%, p < 0.05), whereas was 29.5% (p < 0.05) inhibition in that of arbutin. Tiliroside presented higher activity against melanin production than arbutin. Although the death of cells would lead to the reduction of melanin biogenesis, tiliroside exhibited 14.5% (p < 0.05) inhibition of melanin biogenesis at 0.011 mM with little cytotoxicity on B16 cells. Melanin synthesis is a multi-step pathway. In addition to tyrosinase, it is controlled by many factors including UV exposure, prostaglandins, vitamins, growth factors, interleukins, interferons, and hormones [Citation37]. It is necessary to investigate tiliroside’s inhibition on this multi-step melanin synthesis pathway.
Table 2. The tyrosinase- and melanin-reducing activities of tiliroside on B16 cells.
Conclusion
In this study, tiliroside, a natural compound from raspberry, showed monophenolase and diphenolase inhibitory activity of mushroom tyrosinase. The inhibition mechanism of tilirosde on monophenolase and diphenolase activity of mushroom tyrosinase was elucidated as competitive. Furthermore, its inhibitory effect against intracellular tyrosinase activity and melanin synthesis were studied. Tiliroside could significantly inhibit intracellular tyrosinase activity and melanin production with low cytotoxicity on B16 cells in contrast to arbutin. Those evidently support that tiliroside could be a potential skin whitening agent in cosmetic or pharmaceutical industries. Moreover, future studies on derivatives of tiliroside from raspberry and structure-activity relationships are so necessary as to provide more evidence.
Acknowledgements
This work was supported by Shanghai Leading Academic Discipline Project (B505).
Declaration of interest: The authors report no conflicts of interest. The authors alone are responsible for the content and writing of the paper.
References
- Choia SY, Hwang JS, Kim SS, Kim Y. Synthesis, discovery and mechanism of 2, 6-dimethoxy-N-(4-methoxyphenyl) benzamide as potent depigmenting agent in the skin. Biochem Bioph Res Comm (2006); 349: 39–49.
- Nerya O, Vaya J, Musa R, Izrael S, Ben-Arie R, Tamir S. Glabrene and isoliquiritigenin as tyrosinase inhibitors from licorice roots. J Agric Food Chem (2003); 51: 1201–1207.
- Solano F, Briganti S, Picardo M, Ghanem GH. Hypopigmenting agents: an updated review on biological, chemical and clinical aspects. Pigm Cell Res (2006); 19: 550–571.
- Wang KH, Lin RD, Hsu FL, Huang YH, Chang HC, Huang CY, Lee MH. Cosmetic applications of selected traditional Chinese herbal medicines. J Ethnopharmacol (2006); 106: 353–359.
- Lin YP, Hsu FL, Chen CS, Chern JW, Lee MH. Constituents from the Formosan apple reduce tyrosinase activity in human epidermal melanocytes. Phytochemistry (2007); 68: 1189–1199.
- Zheng ZP, Cheng KW, Chao JF, Wu JJ, Wang MF. Tyrosinase inhibitors from paper mulberry (Broussonetia papyrifera). Food Chem (2008); 106: 529–535.
- Son SM, Moon KD, Lee CY. Rhubarb juice as a natural antibrowing agent. J Food Sci (2000); 65: 1288–1289.
- Kuliev ZA, Vdovin AD, Abdullaev ND, Makhmatkulov AB, Malikov VM. Study of the catechins and proanthocyanidins of Quercus robur. Chem Nat Compd (1997); 6: 642–652.
- The Pharmacopoeia Commission of PRC. Pharmacopoeia of the People’s Republic of China Version. Beijing: Chemical Industry Press; 2005. pp: 265.
- Tomczyk M, Gudej J. Polyphenolic compounds from Rubus saxatilis. Chem Nat Compd (2005); 41: 359–351.
- Schinella GR, Tournier H, Manez AS. Tiliroside and gnaphaliin inhibit human low density lipoprotein oxidation. Fitoterapia (2006); 9: 1–10.
- Sala A, Recio MC, Schinella GR. Assessment of the anti-inflammatory activity and free radical scavenger activity of tiliroside. Eur J Pharmacol (2003); 461: 53–61.
- Panizzi L, Caponi C, Catalano S, Cioni PL, Morelli I. In vitro antimicrobial activity of extracts and isolated constituents of Rubus ulmifolius. J Ethnopharmacol (2002); 79: 165–168.
- Chicaro P, Pinto E, Colepicolo PJ, Lopes LC, Lopes NP. Flavonoids from Lychnophora passerina (Asteraceae): potential antioxidants and UV-protectants. Biochem Syst Ecol (2004); 32: 239–243.
- Kostas D, Costas D, Sofia M, Marrios M, Theodoros T, Dimitrios K. Cytotoxic activity of kaempferol glycoside against human leukaemic cell lines in vitro. Pharm Res (2000); 41: 85–88.
- Anastasia K, Anastasia P, Nikolaos M, Helen S. Identification of tyrosinase inhibitors from Marrubium velutinum and Marrubium cylleneum. Bioorg Med Chem (2007); 15: 2708–2714.
- Budzianowski J, Skrzypczak L. Phenylpropanoid esters from Lamium album flowers. Phytochemistry (1995); 38: 997–1001.
- Chen QX, Song KK, Wang Q, Huang HJ. Inhibitory effects on mushroom tyrosinase by some alkylbenzaldehydes. J Enz Inhib Med Chem (2003); 18: 491–496.
- Wang Q, Qiu L, Chen XR, Song KK, Shi Y, Chen QX.Inhibitory effects of phloridzin dihydrate on the activity of mushroom (Agaricus bisporus) tyrosinase. Bioorg Med Chem. (2007); 15: 1568–1571.
- Zhang CW, Lu Y, Tao HL, Tao XY, Su XC, Wei DZ. Tyrosinase inhibitory effects and inhibition mechanisms of nobiletin and hesperidin from citrus peel crude extracts. J Enz Inhib Med Chem (2007); 22: 1–98.
- Gillian H, Carole T, Cresswell JE, Thody AJ. α-Melanocyte stimulating hormone and its analogue Nle4DPhe7α-MSH affect morphology, tyrosinase activity and melanogenesis in cultured human melanocytes. J Cell Sci (1994); 107: 205–211.
- Jung GD, Yang JY, Song ES. Stimulation of melanogenesis by glycyrrhizin in B16 melanoma cells. Exp Mol Med (2001); 33: l31–l35.
- Cheng DQ, Wei XD, Wang SQ, Xu AE. Effect of tradition Chinese medicine on the tyrosinase activity and melanogenesis in B-16 murine melanoma. Chin J Dermato (2000); 33: 173–174.
- Xu ZY, Zhang QH. Dual regulation of rhubarb on proliferation and tyrosinase in human epidermal melanocyte. Chin J Dermato (1997); 30: 404–405.
- Ando H, Itoh A, Mishima Y, Ichihashi M. Correlation between the number of melanosomes, tyrosinase mRNA levels, and tyrosinase activity in cultured murine melanoma TYHcells in response to various melanogenesis regulatory agents. J Cell Physiol (1995); 163: 608–614.
- Xie LP, Chen QX, Huang H, Liu XD, Zhang RQ. Inhibitory effects of cupferron on the monophenolase and diphenolase activity of mushroom tyrosinase. Int J Biochem Cell Biol (2003); 35: 1658–1666.
- Lorena GF, José NRL, Francisco GS, Pedro AG, Ramón R, Francisco V, José GCT. Analysis and interpretation of the action mechanism of mushroom tyrosinase on monophenols and diphenols generating highly unstable o-quinones. Biochim Biophys Acta (2001); 1548: 1–22.
- Sánchez-Ferrer A, Rodríguez-López J, García-Cánovas NF, García-Carmona F. Tyrosinase: a comprehensive review of its mechanism. Biochim Biophys Acta (1995); 1247: 1–11.
- Juan CE, Pedro AGR, José T, Francisco GC. Study of stereospecificity in mushroom tyrosinase. Biochem J. (1998); 331, 547–551
- Nerya O, Musa R, Khatib S, Tamir S, Vaya J. Chalcones as potent tyrosinase inhibitors: the effect of hydroxyl positions and numbers. Phytochemistry (2004); 65: 1389–1395.
- Song KK, Qiu L, Huang H, Chen QX. The inhibitory effect of tyrosinase by arbutin as cosmetic additive. J Xiamen University (Natural Science) (2003); 42: 791–794.
- Sabrina O, Delphine R, Sébastien B, Anne-Marie M, Eric P, Ahcéne B. Analogues of N-hydroxycinnamoylphenalkylamides as inhibitors of human melanocyte-tyrosinase. Bioorg Med Chem Lett (2006); 16: 2252–2255.
- Long Z, Hyang RP, Yun KP, Seung KL, Jeong HP, Man KP. Mushroom Tyrosinase Inhibition activity of some chromones. Chem Pharm Bull (2002); 50: 309–311.
- Himmelwright RS, Eickman NC, LuBien CD, Lerch K, Solomon EI. Chemical and spectroscopic studies of the binuclear copper active site of Neurospora tyrosinase: Comparison to hemocyanins. J Am Chem Soc (1980); 102: 7339–7344.
- Solomon EI. In Copper Proteins. New York: John Wiley and Sons; 1981. pp: 42–108.
- Wilcox DE, Porras AG, Hwang YT, Lerch K, Winkler ME, Solomon EI. Substrate analogue binding to the coupled binuclear copper active site in tyrosinase. J Am Chem Soc (1985); 107: 4015–4027.
- Lin YP, Hsu F, Chen CS, Chern JW, Lee MH. Constituents from the Formosan apple reduce tyrosinase activity in human epidermal melanocytes. Phytochemistry (2007); 68: 1189–1199.