Abstract
A new series of macrocyclic complexes of type [M(TML)X]X2, where M = Cr(III), Mn(III), or Fe(III), TML is tetradentate macrocyclic ligand, and X = Cl−, NO3−, CH3COO− for Cr(III), Fe(III) and X = CH3COO− for Mn (III), has been synthesized by condensation of benzil and succinyldihydrazide in the presence of metal salt. The complexes have been so formulated due to the 1:2 electrolytic nature of these complexes as shown by conductivity measurements. The complexes have been characterized with the help of various physicochemical techniques such as elemental analysis, molar conductance, electronic and infrared spectral studies, and magnetic susceptibility. On the basis of these studies, a five-coordinate distorted square pyramidal geometry, in which two nitrogens and two carbonyl oxygen atoms are suitably placed for coordination toward the metal ion, has been proposed for all the complexes. The complexes have been tested for their in vitro antibacterial activity. Some of the complexes show remarkable antibacterial activities against some selected bacterial strains. The minimum inhibitory concentrations shown by these complexes have been compared with those shown by some standard antibiotics such as linezolid and cefaclor.
Abbreviations: | ||
MIC, | = | minimum inhibitory concentration |
MTCC, | = | microbial type culture collection |
MHA, | = | Muller–Hinton agar |
CFU, | = | colony forming unit |
BM, | = | Bohr magneton |
DMF, | = | N,N-dimethylformamide |
DMSO, | = | dimethyl sulfoxide |
BHI, | = | brain heart infusion |
Introduction
Transition metals play an important role in the development of new molecular materials which show magnetic properties and find applications in various fields. During the past few decades, the synthesis of macrocyclic complexes has been a fascinating area of research growing at a very fast pace, owing to their resemblance to naturally occurring macrocycles and analytical, industrial, and medical applicationsCitation1–5. Macrocyclic nickel complexes find use in DNA recognition and oxidationCitation6 while macrocyclic copper complexes find use in DNA binding and cleavageCitation7. Macrocyclic metal complexes of lanthanides, e.g. Gd3+ are used as magnetic resonance imaging (MRI) contrast agentsCitation8,Citation9. Macrocyclic metal chelating agents (DOTA) are useful for detecting tumor lesionsCitation10. The chemistry of macrocyclic complexes is also important due to their use as dyes and pigmentsCitation11 as well as nuclear magnetic resonance (NMR) shift reagentsCitation12. Some macrocyclic complexes have been found to exhibit potential antibacterial activitiesCitation13. Prompted by these, in the present article a new series of macrocyclic complexes of Cr(III), Fe(III), and Mn(III) obtained by template condensation reaction of succinyldihydrazide and benzil is reported. The complexes have been characterized with the help of infrared (IR) and magnetic susceptibilities, elemental analysis, and molar conductance. These complexes have also been tested for their in vitro antibacterial activities. Some complexes show remarkable antibacterial activity.
Experimental
Chemistry
Materials
Diethylsuccinate, hydrazine hydrate, and benzil were obtained from SRL Pvt. Ltd., Mumbai. All other chemicals used were of AnalR grade.
Analytical and physical measurements
Microanalyses of C, H, and N were carried out at the Sophisticated Analytical Instrument Facility, CDRI, Lucknow. The metal contents were determined by standard ethylenediaminetetraacetic acid (EDTA) methods. Electronic spectra (DMF) were recorded on a Cary 14 spectrophotometer. The magnetic susceptibility measurements were carried at IIT, Roorkee. The IR spectra were recorded on an infrared spectrophotometer in the range 4000– 200 cm−1. The conductivity was measured using a digital conductivity meter (HPG System, G-3001).
Isolation of complexes
All the reported complexes were prepared using the template method. To a stirring methanolic solution (~50 cm3) of succinyldihydrazide (10 mmol) was added trivalent chromium, manganese, and iron salt (10 mmol) dissolved in a minimum quantity of methanol (20 cm3). The resulting solution was refluxed for 0.5 h. After that, benzil (10 mmol) dissolved in ~20 mL of methanol was added to the refluxing mixture which was refluxed again for 6–8 h. On overnight cooling, a dark colored precipitate formed, which was filtered, washed with methanol, acetone, and diethyl ether, and dried in vacuo (yield 45%). The complexes were found to be soluble in DMF and DMSO, but were insoluble in common organic solvents and water. They were found to be thermally stable up to ~240°C and then they decomposed.
The template syntheses of the complexes may be represented by the scheme shown in , where M = Cr(III), Mn(III), Fe(III), and X = Cl−, NO3−, CH3COO− for Cr(III) and Fe(III) and CH3COO− for Mn (III).
Pharmacology
In vitro antibacterial activity
Some of the synthesized macrocyclic complexes were tested for in vitro antibacterial activity against some bacterial strains using spot-on-lawn on Muller–Hinton agar by a reported methodCitation14.
Test pathogens Four test pathogenic bacterial strains, viz. Bacillus cereus (MTCC 1272), Salmonella typhi (MTCC 733), Escherichia coli (MTCC 739), and Staphylococcus aureus (MTCC 1144), were considered for determination of the MIC (minimum inhibitory concentration) of selected complexes.
Culture conditions The test pathogens were subcultured aerobically using brain heart infusion agar (HiMedia, Mumbai, India) at 37°C/24 h. Working cultures were stored at 4°C in brain heart infusion (BHI) broth (HiMedia), while stock cultures were maintained at −70°C in BHI broth containing 15% (v/v) glycerol (Qualigens, Mumbai, India). Organism was grown overnight in 10 mL BHI broth, which was then centrifuged at 5000g for 10 min, and the pellet was suspended in 10 mL of phosphate buffered saline (PBS, pH 7.2). The optical density at 545 nm (OD-545) was adjusted to obtain 108 CFU/mL, followed by plating serial dilution onto plate count agar (HiMedia).
Determination of minimum inhibitory concentration The minimum inhibitory concentration (MIC) is the lowest concentration of the antimicrobial agent that prevents the development of viable growth after overnight incubation. The antimicrobial activity of the compounds was evaluated using the spot-on-lawn method on Muller–Hinton agar (MHA; HiMedia). Soft agar was prepared by adding 0.75% agar in Muller–Hinton broth (HiMedia). The soft agar was inoculated with 1% of 108 CFU/mL of the test pathogen, and 10 mL was overlaid on MHA. From a × 1000 solution of compound (1 mg/ml of DMSO), × 1, × 2, × 4, × 8, × 16, × 32, × 64, and × 128 solutions were prepared. Dilutions of standard antibiotics (linezolid and cefaclor) were also prepared in the same manner. Five microliters of the appropriate dilution was spotted on the soft agar and incubated at 37°C for 24 h. Zones of inhibition of the compounds were considered after subtraction of the inhibition zones of DMSO. A negative control (with no compound) was also observed.
Results and discussion
Chemistry
The analytical data show the formula of macrocyclic complexes as: [M(C18H16N4O2)X]X2, where M = Cr(III), Mn(III), Fe(III) and X = Cl−, NO3−, CH3COO− for Cr(III) and Fe(III) and X = CH3COO− for Mn(III). The test for anions was positive before and after decomposing the complexes with concentrated HNO3, indicating their presence inside as well as outside the coordination sphere. Conductivity measurements in DMSO indicated them to be electrolytic in nature (140–150 ohm−1 cm2 mol−1)Citation15. All compounds gave satisfactory elemental analysis results, as shown in .
Table 1. Analytical data for trivalent chromium, manganese, and iron complexes derived from succinyldihydrazide and benzil.
IR spectra
In the infrared spectrum of succinyldihydrazide, a pair of bands corresponding to ν(NH2) is present at ~3200 cm−1and ~3250 cm−1, but this was absent in the IR spectra of all the complexesCitation16. However, a single, broad, medium band at ~3350–3400 cm1 was observed in the spectra of all the complexes, which may be assigned to ν(NH)Citation17,Citation18. A strong peak at ~1665 cm−1 in the IR spectrum of succinyldihydrazide is assigned to the >C=O group of the CONH moiety. This peak was shifted to a lower frequency (~1625–1640 cm−1) in the spectra of all the complexesCitation19,Citation20, suggesting coordination of the oxygen of the carbonyl group with the metal. Further, no strong absorption band was observed near 1700 cm−1 in the IR spectra of all complexes, as observed in the spectrum of benzil. This indicates the absence of >C=O groups of the benzil moiety in the complexes. These facts confirm the condensation of carbonyl groups of the benzil and amino groups of succinyldihydrazideCitation21,Citation22. The IR spectra of the complexes showed a new strong absorption band in the region ~1590–1610 cm−1, which may be attributed to ν(C=N)Citation23,Citation24. These results provide strong evidence for the formation of a macrocyclic frameCitation25. The lower value of ν(C=N) indicates coordination of the nitrogens of azomethine and the metalCitation26. The bands present at ~1350– 1000 cm−1 are assigned to ν(C–N) vibration. The bands presents at ~ 3040 cm−1 may be assigned to ν (C–H) vibrations of the benzil moiety.
Far infrared spectra
The far infrared spectra showed bands in the region ~425– 445 cm−1 corresponding to ν(M–N) vibrations in all the complexesCitation27–29. The presence of a band in all the complexes at the ~425– 445 cm−1 region originates from (M–N) azomethine vibration modes and gives an idea about coordination of azomethine nitrogens with the metalCitation30. The bands present at ~300– 315 cm−1 are due to ν(M–Cl)Citation27,Citation29 and bands present at ~220– 250 cm−1 in all nitrato complexes to ν(M–O)Citation27.
Magnetic measurements and electronic spectra
Chromium complexes Magnetic moments of chromium complexes were found in the range of 4.25–4.60 BM. The electronic spectra of chromium complexes showed bands at ~9010–9260, 13,000–13,550, 17,460–18,300, 27,400–27,800, and 34, 850 cm−1. However, these spectral bands cannot be interpreted in terms of four or six coordinated environments around the metal atom. In turn, the spectra are comparable to those of five-coordinated Cr(III) complexes, whose structures have been confirmed with the help of X-ray measurementsCitation31. Thus, keeping in view the analytical data and electrolytic nature of these complexes, a five-coordinated square pyramidal geometry may be assigned to these complexes. Thus, assuming the symmetry C4V for these complexesCitation32, the various spectral bands may be assigned as: 4B1 → 4Ea, 4B1 → 4B2, 4B1 → 4A2, and 4B1 → 4Eb.
Manganese complex The magnetic moment of the manganese complex was found to be 4.80 BM. The electronic spectrum of the manganese complex showed three d–d bands which lay in the regions 12,200, 16,100, and 35, 400 cm−1. The higher energy band at 35, 400 cm−1 may be assigned to charge transfer transitions. The spectrum resembled those reported for five-coordinate square pyramidal manganese porphyrinsCitation32. This idea is further supported by the presence of the broad-ligand field band at 20, 400 cm−1, diagnostic of C4V symmetry, and thus the various bands may be assigned as follows: 5B1 → 5A1, 5B1 → 5B2, and 5B1 → 5E, respectively. The band assignment in single electron transition may be made as: dz2 → dx2−y2, dxy → dx2−y2, and dxy, dyz → dx2−y2, respectively, in order of increasing energy. However, the complexes do not have idealized C4V symmetry.
Iron complexes The magnetic moments of the iron complexes lay in the range 5.80–5.92 BM. The electronic spectra of the trivalent iron complexes showed various bands, 9800–9900, 15,500–15,550, 27,600–27,700 cm−1, and these bands do not suggest octahedral or tetrahedral geometry around the metal atom. The spectral bands are consistent with the range of those reported for five-coordinate square pyramidal iron(III) complexesCitation33. Assuming C4V symmetry for these complexes, the various bands can be assigned as: dxy → dxz, dyz and dxy → dz2. Any attempt to make an accurate assignment is difficult due to interactions of the metal–ligand pi-bond systems lifting the degeneracy of the dxz and dyz pair.
Based on elemental analyses, conductivity and magnetic measurements, and electronic, IR and far IR spectral studies, the general structure shown in may be proposed for these complexes.
Biology
The synthesized macrocyclic complexes were tested for their in vitro antibacterial activity against four test bacteria, Bacillus cereus (MTCC 1272), Salmonella typhi (MTCC 733), Escherichia coli (MTCC 739), and Staphylococcus aureus (MTCC 1144). The MIC (minimum inhibitory concentration) shown by the complexes against these bacterial strains was compared with the MIC shown by standard antibiotics linezolid and cefaclor ( and ). Complex 1 showed a minimum inhibitory concentration of 32 μg/mL against bacterial strain Salmonella typhi (MTCC 733), which is equal to the MIC shown by standard antibiotic linezolid against the same bacterial strain. Complex 3 registered a minimum inhibitory concentration of 4 μg/mL against bacterial strain Staphylococcus aureus (MTCC 1144), which is equal to the MIC shown by standard antibiotic linezolid against the same bacterial strain. Further, it showed a minimum inhibitory concentration of 16 μg/mL against bacterial strain Escherichia coli (MTCC 739), which is equal to the MIC shown by standard antibiotic linezolid against the same bacterial strain. Complex 4 registered a minimum inhibitory concentration ranging from 16 to 64 μg/mL. Complex 6 registered a minimum inhibitory concentration of 8 μg/mL against the bacterial strain Bacillus cereus (MTCC 1272), which is equal to the MIC shown by standard antibiotic cefaclor against the same bacterial strain. Complex 7 registered a minimum inhibitory concentration of 32 μg/mL against bacterial strain Salmonella typhi (MTCC 733), which is equal to the MIC shown by standard antibiotic linezolid against the same bacterial strain. Among the series under test for determination of the minimum inhibitory concentration, complex 3 was found to be the most potent. However, complexes 2, 4, and 5 showed poor antibacterial activity or no activity against all bacterial strains among the whole series.
Figure 3. Comparison of minimum inhibitory concentration (MIC) of complexes with those of standard antibiotics up to concentration of 64 μg/mL. a, Bacillus cereus (MTCC 1272); b, Staphylococcus aureus (MTCC 1144); c, Escherichia coli (MTCC 739); d, Salmonella typhi (MTCC 733); cefaclor and linezolid are standard antibiotics.
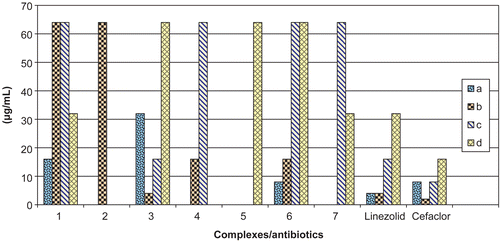
Table 2. Minimum inhibitory concentration (MIC) shown by complexes against test bacteria using agar dilution assay.
Conclusions
Based on elemental analyses, conductivity and magnetic measurements, and electronic, IR, and far IR spectral studies, the structure as shown in may be proposed for these complexes.
It has been suggested that chelation/coordination reduces the polarity of the metal ion mainly because of partial sharing of its positive charge with the donor group within the whole chelate ring system. This process of chelation thus increases the lipophilic nature of the central metal atom, which in turn favors its permeation through the lipid layer of the membrane, thus causing the metal complex to cross the bacterial membrane more effectively and hence increase the activity of the complex. Aside from this, many other factors such as solubility, dipole moment, and conductivity influenced by the metal ion may be possible reasons for the remarkable antibacterial activities of these complexesCitation34–37. It also has been observed that some moieties, such as the azomethine linkage or heteroaromatic nucleus, introduced into such compounds exhibit extensive biological activities that may be responsible for the increase in hydrophobic character and liposolubility of the molecules in crossing the cell membrane of the microorganism, thus enhancing the biological utilization ratio and activity of complexesCitation38,Citation39.
Acknowledgements
D. P. Singh thanks the University Grants Commission, New Delhi, for financial support in the form of a Major Research Project. Thanks are also due to the authorities of NIT, Kurukshetra, for providing necessary research facilities.
Declaration of interest: The authors report no conflicts of interest.
References
- Gloe K, ed. Macrocyclic Chemistry: Current Trends and Future Perspectives. Dordrecht: Springer, 2005.
- Lindoy LF, ed. The Chemistry of Macrocyclic Ligand Complexes. Cambridge: Cambridge University Press, 1989.
- Constable EC, ed. Coordination Chemistry of Macrocyclic Compounds. Oxford: Oxford University Press, 1999.
- Singh DP, Kumar R, Malik V, Tyagi P. Synthesis and characterization of complexes of Co(II), Ni(II), Cu(II), Zn(II), Cd(II) with macrocycle 3,4,11,12-tetraoxo-1,2,5,6,9,10,13,14-octaazacyclohexadeca-6,8,14,16-tetraene and their biological screening. Transition Met Chem 2007;32:1051–5.
- Singh DP, Kumar R, Malik V, Tyagi P. Template synthesis, spectroscopic studies and biological activities of macrocyclic complexes derived from thiocarbohydrazide and glyoxal. J Enzyme Inhib Med Chem 2007;22:177–82.
- Muller JG, Chen X, Dadiz AC, Rokita SE, Burrows CJ. Macrocyclic nickel complexes in DNA recognition and oxidation. Pure Appl Chem 1993;65:545–50.
- Liu J, Lu TB, Deng H, Ji LN, Qu LH, Zhou H. Synthesis DNA-binding and cleavage studies of macrocyclic copper(II) complexes. Transition Met Chem 2003;28:116–21.
- Paryzek WR, Krzyminiewska VP. Yttrium(III) complexes of pentadentate Schiff base macrocyclic ligands with N3O2 and N5 set of donor atoms. Polyhedron 1995;14:2059–62.
- Watson AD, Rockladge SM. In: Higgins CB, ed. Magnetic Resonance Imaging of the Body. New York: Raven Press, 1992.
- Kosmas C, Snook D, Gooden CS, Courtenay-Luck NS, McCall MJ, Claude F, Meares CF, Epenetos AA. Development of humoral immune responses against a macrocyclic chelating agent (DOTA) in cancer patients receiving radioimmunoconjugates for imaging and therapy. Cancer Res 1992;52:904–11.
- Seto J, Tamura S, Asai N, Kishii N, Kijima Y, Matsuzawa N. Macrocyclic functional dyes: applications to optical disk media, photochemical hole burning and non-linear optics. Pure Appl Chem 1996;68:1429–34.
- Dong W, Yang R, Yan L. Rare earth complexes of a new Schiff base macrocyclic ligand derived from 2,29-(ethylenedioxy) bisbenzaldehyde and 2,29-(ethylenedioxy)bisbenzyl amine. Indian J Chem 2001;40A:202–6.
- Singh DP, Kumar R, Tyagi P. Template synthesis, spectroscopic studies and biological screening of macrocyclic complexes derived from thiocarbohydrazide and benzil. Transition Met Chem 2006;31:970–3.
- Singh DP, Kumar R, Singh J. Biologically active trivalent metal macrocyclic complexes derived from oxalyldihydrazide and benzil: synthesis and spectroscopic approach. Eur J Med Chem 2009 (in press).
- Kumar R, Singh R. Chromium(III) complexes with different chromospheres macrocyclic ligand: synthesis and spectroscopic studies. Turk J Chem 2006;30:77–87.
- Prasad RN, Mathur M, Upadhyay A. Synthesis and spectroscopic studies of CrIII, FeIII and CoII complexes of hexaazamacrocycles. J Indian Chem Soc 2007;84:1202–4.
- Khan TA, Rather MA, Jahan N, Varkey SP, Shakir M. Tetraoxotetraamide macrocyclic complexes. Transition Met Chem 1998;23:283–5.
- Singh AK, Panwar A, Singh R, Beniwal S. New bis-macrocyclic complexes with transition metal ion. Transition Met Chem 2003;28:160–2.
- Pavia DL, Lampman GM, Kriz GS.Introduction to Spectroscopy. New York: Harcourt College Publishers, 2001.
- Nishat N, Ud-din R, Haq MM, Siddiqi KS. Synthesis and characterization of new 13- and 14-membered macrocycles and their transition metal complexes. Transition Met Chem 2003;28:948–53.
- Prasad RN, Mathur M. CrIII, FeIII, CoII, NiII, CuII and ZnII complexes of 26- and 28-membered tetraazamacrocycles. J Indian Chem Soc 2006;83:1208–13.
- Zeng Q, Sun J, Gou S, Zhou K, Fang J, Chen H. Synthesis and spectroscopic studies of dinuclear copper(II) complexes with new pendant-armed macrocyclic ligands. Transition Met Chem 1998;23:371–3.
- Singh AK, Singh R, Saxena P. Macrocyclic metal complexes: synthesis and characterization of 14- and 16-membered tetraaza macrocyclic complexes of transition metals. Transition Met Chem 2004;29:867–9.
- Gupta LK, Chandra S. Physicochemical and biological characterization of transition metal complexes with a nitrogen donor tetra-dentate novel macrocyclic ligand. Transition Met Chem 2006;31:368–73.
- Mohamed AK, Islam KS, Hasan SS, Shakir M. Metal ion directed synthesis of 14-16 membered tetraimine macrocyclic complexes. Transition Met Chem 1999;24:198–201.
- Lodeiro C, Basitida R, Bertolo E, Macias A, Rodriguez R. Synthesis and characterization of four novel NxOy-Schiff base macrocyclic ligands and their metal complexes. Transition Met Chem 2003;28:388–94.
- Shakir M, Islam KS, Mohamed AK, Shagufta M, Hasan SS. Macrocyclic complexes of transition metals with divalent polyaza units. Transition Met Chem 1999;24:577–80.
- Shakir M, Varkey SP. A new synthetic route for the preparation of a new series of 14–22-membered tetraoxomacrocyclic tetraamines and their transition metal complexes. Polyhedron 1995;14:1117–27.
- Aqra FMAM. New macrocyclic complexes containing amide, imine and secondary amine functions. Transition Met Chem 1999;24:337–9.
- Chandra S, Kumar R. Synthesis and spectral studies on mononuclear complexes of chromium(III) and manganese(II) with 12-membered tetradentate N2O2, N2S2 and N4 donor macrocyclic ligand. Transition Met Chem 2004;29:269–75.
- Wood JS. Stereochemical electronic structural aspects of five coordination. Prog Inorg Chem 1972;16:227.
- Singh DP and Rana VB. Binuclear chromium (III), manganese (III), iron (III) and cobalt (III) complexes bridged by diaminopyridine. Polyhedron. 1995; 14: 2901–2906.
- Lever ABP. Inorganic Electronic Spectroscopy. Amsterdam: Elsevier, 1984.
- Chohan ZH, Supuran CT. Structure and biological properties of first row d-transition metal complexes with N-substituted sulfonamides. J Enzyme Inhib Med Chem 2008;23:240–51.
- Chohan ZH. Metal-based sulfonamides: their preparation, characterization and in-vitro antibacterial, antifungal & cytotoxic properties. X-ray structure of 4-(2-hydroxybenzylidene) amino benzenesulfonamide. J Enzyme Inhib Med Chem 2008;23:120–30.
- Chohan ZH, Shad HA. Structural elucidation and biological significance of 2-hydroxy-1-naphthaldehyde derived sulfonamides and their first row d-transition metal chelates. J Enzyme Inhib Med Chem 2008;23:369–79.
- Chohan ZH. Synthesis of organometallic-based biologically active compounds: in vitro antibacterial, antifungal and cytotoxic properties of some sulfonamide incorporated ferrocences. J Enzyme Inhib Med Chem 2009;24:169–75..
- Chohan ZH, Supuran CT, Hadda TB, Nasim F, Khan KM. Metal based isatin-derived sulfonamides: their synthesis, characterization, coordination behavior and biological activity. J Enzyme Inhib Med Chem 2008 Sep 30:1. [Epub ahead of print]
- Singh K, Singh DP, Barwa MS, Tyagi P, Mirza Y. Antibacterial Co(II), Ni(II), Cu(II) and Zn(II) complexes of Schiff bases derived from fluorobenzaldehyde and triazoles. J Enzyme Inhib Med Chem 2006;21:557–62.