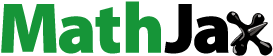
Abstract
A series of 20 newly designed (E)-1-(4-sulphamoylphenylethyl)-3-arylidene-5-aryl-1H-pyrrol-2(3H)-ones was synthesised and assessed as carbonic anhydrase (CA, EC 4.2.1.1) inhibitors towards four human isoforms of pharmaceutical interest, that is, hCA I, II, IX and XII. The compounds displayed low to high nanomolar potency against all the isoforms. Introducing strong electron withdrawing groups at the para position of the arylidene ring increased the binding affinity to the enzyme. All compounds showed acceptable pharmacokinetic range and physicochemical characteristics as determined by computational ADMET analysis. Density Functional Theory (DFT) calculations of 3n were carried to gain understanding on the stability of the E and Z isomers. The energy values clearly indicate the stability of E isomer over Z isomer by −8.2 kJ mol−1. Our findings indicate that these molecules are useful as leads for discovering new CA inhibitors.
Introduction
Carbonic anhydrases (EC 4.2.1.1; CA) are a superfamily of ubiquitous metalloenzymes, which play a vital role in several physiological processes in organisms belonging to all life kingdoms, by catalysing the reversible hydration of carbon dioxide into bicarbonate and a proton (CO2 +H2O ⇋ HCO3 - + H+)Citation1. Among the eight unrelated gene families of CAs (α, β, γ, δ, η, ζ, θ and ι) only the α-class was found in higher vertebrates. In Homo sapiens, 15 isoforms of α-CAs (hCAs) were identified, each differing by oligomeric state (most are monomers, with CA VI, IX and XII being dimers), molecular features, subcellular location, distribution in tissues and organs, expression levels, as well as kinetic propertiesCitation2,Citation3. hCA I, III, VII, and XIII are present in the cytosol, whereas hCA IV, IX, XII, and XIV are membrane-bound, hCA VA and VB are located in the mitochondria, isoform VI is secreted, and CA VIII, X, and XI are acatalytic, cytosolic isoforms. All the catalytically active isoforms are important for maintaining the intracellular and extracellular pH and CO2/HCO3- pool of cellsCitation4. CA isoforms play important roles in various metabolic reactions in mammals (i.e., gluconeogenesis, lipogenesis and ureagenesis), electrolyte secretion, pH and CO2 homeostasis, respiration, bone resorption and tumourigenicityCitation5. However, a variety of pathological conditions in humans have been linked to an altered expression/activity of these isoforms. Besides retinal and brain oedema, the cytosolic hCA I and II isoforms have been connected with glaucoma, altitude sickness and epilepsyCitation6. hCA IX and hCA XII have been related to inflammation and tumorigenesis. They control the extracellular and intracellular pH levels favouring the growth, progression and metastases formation of many tumour types. Their overexpression in many human malignancies makes them interesting new targets in the development of anticancer drugs for treating hypoxic tumoursCitation7. Thus, novel therapeutic alternatives may be obtained by interfering with CA activity in such conditions, bringing it to normal levels either through inhibition or activationCitation8.
The most investigated class of CA inhibitors (CAIs) are the zinc-binders, among which the primary sulphonamides have wide therapeutic applications, such as antiglaucoma agents, diuretics, antidiabetic, and anticancer therapyCitation9. In CA-catalysed processes, the inclusion of an aromatic or heteroaromatic scaffold harbouring the sulphonamide group greatly stabilizes the formation of the enzyme-ligand complexCitation10. These sulphonamide inhibitors bind to CAs by replacing a water molecule or hydroxide ion coordinated to the Zn2+ ion from the active site of enzyme, as in the acetazolamide (AAZ), a drug that has been used in medicines since 1954. The binding mode of the sulphonamides within various CA active sites has been explored by many X-ray crystallographic studies which showed the deprotonated SO2NH- moiety coordinated to the zinc ion, and two hydrogen bonds between the NH- and S = O groups with the side-chain OH and backbone NH of the Thr199, respectively a highly conserved amino acid in all α-CAs active sitesCitation11 ().
Figure 1. 2D-representation of the α-CA-benzenesulphonamide complex displaying the estimated active site region and residues participating in the recognition of the inhibitor component.Citation11
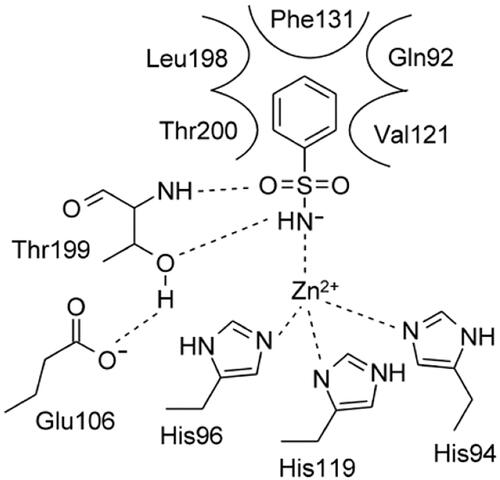
In a previous study, our team found that a set of benzenesulphonamides connected to the pyridazinone nucleus had an outstanding inhibitory effect against hCAI and II in the nanomolar rangeCitation12. We thus continued and broadened our research in this article to examine the impact of pyrrolone-substituted ethyl benzene sulphonamides on the inhibitory profile of the hCA I, II, IX, and XII isoforms in an effort to find new potent and selective CAIs.
Due to their significant pharmacological activity, pyrrolones (five-member heterocyclic Δ3or Δ4-lactams) have garnered a lot of interest in recent years. It has been reported that they have antibacterialCitation13, antifungalCitation14, anticancerCitation15,Citation16 and anti-inflammatoryCitation17activities ().
Materials and methods
Chemistry
Chemicals and starting materials were acquired from Sigma Aldrich, Merck (Germany) and Spectrochem. Open capillary tubes were used for melting point determination and are uncorrected. BIO-RAD FTS-135 spectrophotometer (Waltham, MA, USA) was used to record Fourier Transform Infra-Red (FTIR) by making use of KBr pellets and vmax values are given in cm−1. Bruker spectrospin DPX 400MHZ spectrometer (Fallanden, Switzerland) was used to record 1H NMR spectra by using internal standard as tetramethylsilane (TMS) and solvent as CDCl3 or (DMSO-d6). Coupling constants i.e., J values are expressed in Hertz and chemical shift values are given in δ (ppm) scale. Multiplicities in chemical shift are expressed as s = singlet, d = doublet, t = triplet, and m = multiplet. Bruker spectrospin DPX at 100MHZ was used to record 13C spectra. Managed by mass Lynx software (version 4.1), XEVO TQ-S triple quadrupole mass spectrometer (Waters, USA) was used to scan mass spectra (MS) and more intense peak values are specified by m/z ratio. TLC plates (silica gel G) were utilised for determining progress of reaction and further visualisation was done by exposing to vapours of iodine. To carry elemental analysis, CHNS Elementar (Vario EL III, Hanau Germany) was used.
General procedure for the synthesis of (E)-1–(4-sulfamoylphenylethyl)-3-arylidene-5-aryl-1H-pyrrol-2(3H)-ones (3a-t)
The following two steps were involved in the synthesis of compounds:
Synthesis of γ-keto-4-sulfamoylphenylethylamide:
Using Friedel Crafts succinoylation, β-aroylpropionic acids were synthesised from the appropriate aromatic hydrocarbons. The required 2(3H)-furanone derivatives (1a-t) were prepared by refluxing the equimolar amount of appropriate β-aroylpropionic acids and aromatic aldehydes in acetic anhydride. Then 2(3H)-Furanone (3 mmol) and 4-(2-aminoethyl) benzenesulphonamide (4 mmol) were refluxed for 2–5 h in dry benzene. After completion of reaction, the volume of the reaction mixture was reduced to half by removing excess benzene through distillation. The mixture was left at room temperature to give crystals that were filtered off, washed with little volume of dry benzene and then dried to give 2a-t.
2. Cyclisation of γ-keto-4-sulfamoylphenylethylamide:
The crystals of γ-keto-4-sulphamoylphenylethylamide (3 mmol) obtained from above experiment were refluxed for 1 h in 6 M hydrochloric acid (20 ml). After cooling the contents, the solid obtained was filtered off, washed with water followed by recrystallisation with methanol to give pure TLC (toluene: ethylacetate: formic acid: 5: 4:1) of compounds (3a-t).
(E)-4-(2-(3-benzylidene-2-oxo-5-phenyl-2,3-dihydro-1H-pyrrol-1-yl)ethyl) benzenesulphonamide (3a)
Light yellow crystals, yield = 80%, m.p. 167–168 °C, Rf = 0.74[toluene: ethyl acetate: formic acid::5:4:1]. IRυmax (KBr, incm−1): 3743 and 3207 (NH2), 1676 (C = O), 1330 and 1150 (SO2N). 1H NMR (400 MHz, DMSO-d6, δ): 2.72 (2H, t, PhCH2CH2N), 3.89 (2H, t, PhCH2CH2N), 6.39 (1H, s, H-4 pyrrolone ring), 7.12 (2H, d, J = 8.00 Hz, H-3′, H-5′), 7.25–7.64 (13H, m, SO2NH2, olefinic H and aromatic protons of Ar1, Ar2 and sulphamoyl phenyl ring), 7.74 (2H, d, J = 8.4 Hz, H-3′′, H-5′′). 13C NMR (100 MHz, DMSO-d6, δ): 34.55, 41.86, 100.58, 123.60(2 C), 126.17, 128.11(2 C), 129.29(4 C), 129.46 (2 C), 130.08, 130.25(2 C), 130.40, 131.19, 132.46, 132.73, 134.82, 142.79, 150.24, 170.06. ESI-MS (m/z): 430[M+], 431[M++1], 432[M + 2]. CHNS Analysis for C25H22N2O3S Found (Calculated): C: 69.75(69.89), H: 5.15(5.17), N:6.51 (6.53), O:11.15 (11.11), S: 7.45 (7.55).
(E)-4-(2-(3-(4-fluorobenzylidene)-2-oxo-5-phenyl-2,3-dihydro-1H-pyrrol-1-yl)ethyl)benzenesulphonamide (3b)
Light yellow crystals, yield = 85%, m.p. 170–171 °C, Rf = 0.73[toluene: ethyl acetate: formic acid; 5:4:1]. IRυmax (KBr, incm−1): 3743 and 3207 (NH2), 1676 (C = O), 1330 and 1150 (SO2N). 1H NMR (400 MHz, DMSO-d6, δ): 2.71(2H, t, PhCH2CH2N), 3.89 (2H, t, PhCH2CH2N), 6.39 (1H, s, H-4 pyrrolone ring), 7.12 (2H, d, J = 8.4 Hz, H-3, H-5), 7.25–7.64 (12H, m, SO2NH2, olefinic H and aromatic protons of Ar1, Ar2 and sulphamoyl phenyl ring), 7.74 (2H, d, J = 8.4 Hz, H-3′′, H-5′′).13C NMR (100 MHz, DMSO-d6, δ): 34.55, 41.86, 100.58, 123.60 (2 C), 126.17, 128.12 (2 C), 129.29 (4 C), 129.46 (2 C), 130.08, 130.25 (2 C), 130.40, 131.20, 132.46, 132.74, 134.83, 142.79, 150.25, 170.07. ESI-MS (m/z): 448[M+], 449[M++1]. CHNS Analysis for C25H21FN2O3S Found (Calculated): C: 66.95(66.94), H: 4.72(4.73), F: 4.24(4.23); N:6.25 (6.27), O:10.70 (10.71), S: 7.15 (7.25).
(E)-4-(2-(3-(4-chlorobenzylidene)-2-oxo-5-phenyl-2,3-dihydro-1H-pyrrol-1-yl)ethyl)benzenesulphonamide (3c)
Light brown crystals, yield = 94%, m.p 192–193 °C, Rf = 0.72 [toluene: ethyl acetate: formic acid; 5:4:1]. IRυmax (KBr, in cm−1): 3743 and 3207 (NH2), 1676 (C = O), 1330 and 1150 (SO2N). 1H NMR (300 MHz, CDCl3, δ): 2.82 (2H, t, PhCH2CH2N), 3.93 (2H, t, PhCH2CH2N), 4.81 (2H, s, SO2NH2), 6.08 (1H, s, H-4 pyrrolone ring), 7.07 (2H, d, J = 8.1 Hz, H-3′, H-5′), 7.36–7.44 (8H, m, aromatic protons of Ar1, Ar2, sulphamoyl phenyl ring and olefinic H), 7.55 (2H, d, J = 8.1 Hz, H-2′, H-6′), 7.71 (2H, d, J = 8.1 Hz, H-3′′, H-5′′). ESI-MS (m/z): 464 [M+], 465[M++1], 467[M++3]. CHNS Analysis for C25H21ClN2O3S. Found (Calculated): C: 64.58(64.59), H:4.55 (4.51), Cl: 7.62(7.63), N:6.02(6.04), O:10.32 (10.31),S: 6.90 (6.93).
(E)-4-(2-(3-(4-bromobenzylidene)-2-oxo-5-phenyl-2,3-dihydro-1H-pyrrol-1-yl)ethyl)benzenesulphonamide (3d)
Dark brown crystals, yield = 89%, m.p. 214–215 °C, Rf = 0.71[toluene: ethyl acetate: formic acid; 5:4:1]. IRυmax (KBr, incm−1): 3743 and 3207 (NH2), 1676 (C = O), 1330 and 1150 (SO2N). 1H NMR (400 MHz, DMSO-d6, δ): 2.71 (2H, t, PhCH2CH2N), 3.89 (2H, t, PhCH2CH2N), 6.39 (1H, s, H-4 pyrrolone ring), 7.12 (2H, d, J = 8.00 Hz, H-3′, H-5′), 7.25–7.64 (12H, m, SO2NH2, olefinic H and aromatic protons of Ar1, Ar2 and sulphamoyl phenyl ring), 7.74 (2H, d, J = 8.00 Hz, H-3′′, H-5′′). 13C NMR (100 MHz, DMSO-d6, δ): 34.55, 41.86, 100.58, 123.60 (2 C), 126.17, 128.12 (2 C), 129.29(4 C), 129.46(2 C), 130.08, 130.25(2 C), 130.41, 131.20, 132.46, 132.74, 134.82, 142.79, 150.25, 170.20. ESI-MS (m/z): 509 [M+], 511[M++2], 512[M++3]. CHNS Analysis for C25H21BrN2O3S Found (Calculated): C: 58.94(58.91), H:4.16 (4.15), Br: 15.69(15.63), N:5.50(5.48), O: 9.42 (9.40), S: 6.29 (6.38).
(E)-4-(2-(3-benzylidene-2-oxo-5-(p-tolyl)-2,3-dihydro-1H-pyrrol-1-yl)ethyl)benzenesulphonamide (3e)
Light Yellow crystals, yield = 95%, m.p. 189-190 °C, Rf = 0.73[toluene: ethyl acetate: formic acid; 5:4:1]. IRυmax (KBr, in cm−1): 3745 and 3191 (NH2), 1654 (C = O), 1331 and 1156 (SO2N). 1H NMR (400 MHz, DMSO-d6, δ): 2.50 (3H, s, CH3), 2.73 (2H, t, PhCH2CH2N), 3.89 (2H, t, PhCH2CH2N), 6.37(1H, s, H-4 pyrrolone ring), 7.15(2H, d, J = 8.4 Hz, H-3′ and H-5′ Ar1 ring), 7.27-7.66 (12H, m, SO2NH2, olefinic H, aromatic protons of Ar1, Ar2 and sulfamoyl phenyl ring), 7.77 (2H, d, J = 7.20 Hz, H-3′′, H-5′′). 13C NMR (100 MHz, DMSO-d6, δ): 21.44, 34.59, 41.84, 100.33, 126.17(3 C), 128.01(2 C), 128.44(2 C), 128.79(2 C), 129.47(2 C), 129.51(2 C), 129.79, 129.86, 130.15, 130.84, 131.49, 135.69, 139.72, 142.86, 170.24. ESI-MS (m/z): 444[M+], 445[M++1], 446[M++2], 447[M++3]. CHNS Analysis for C26H24N2O3S Found (Calculated): C: 70.25(70.31), H: 5.44(5.45), N: 6.30(6.34), O: 10.80(10.71), S: 7.21 (7.28).
(E)-4-(2-(3-(4-fluorobenzylidene)-2-oxo-5-(p-tolyl)-2,3-dihydro-1H-pyrrol-1-yl)ethyl)benzenesulphonamide (3f)
Light Yellow crystals, yield = 92%, m.p. 196–197 °C, Rf = 0.72 [toluene: ethyl acetate: formic acid; 5:4:1]. IRυmax (KBr, in cm−1): 3745 and 3191 (NH2), 1654 (C = O), 1331 and 1156 (SO2N). 1H NMR (400 MHz, DMSO-d6, δ): 2.38 (3H, s, CH3), 2.72 (2H, t, PhCH2CH2N), 3.88 (2H, t, PhCH2CH2N), 6.34 (1H, s, H-4 pyrrolone ring), 7.13–7.86 (15H, m, SO2NH2, olefinic H and aromatic protons of Ar1, Ar2 and sulphamoyl phenyl ring). ESI-MS (m/z): 462[M+]. CHNS Analysis for C26H23FN2O3S Found (Calculated): C: 67.51(67.41), H:5.01 (5.15), F:4.11(4.23), N: 6.06(6.14), O: 10.38 (10.37), S: 6.93 (6.92).
(E)-4-(2-(3-(4-chlorobenzylidene)-2-oxo-5-(p-tolyl)-2,3-dihydro-1H-pyrrol-1-yl)ethyl)benzenesulphonamide (3 g)
Dark brown crystals, yield = 96%; m.p. 224–225 °C, Rf = 0.71 [toluene: ethyl acetate: formic acid; 5:4:1]. IRυmax (KBr, in cm−1): 3745 and 3191 (NH2), 1654 (C = O), 1331 and 1156 (SO2N). 1H NMR (400 MHz, DMSO-d6, δ): 2.38 (3H, s, CH3), 2.72 (2H, t, PhCH2CH2N), 3.88 (2H, t, PhCH2CH2N), 6.34 (1H, s, H-4 pyrrolone ring), 7.14 (2H, d, J = 8.4 Hz, H-3′, H-5′), 7.23–7.38 (7H, m, SO2NH2, olefinic H and aromatic protons of Ar1 and Ar2), 7.48 (2H, d, J = 8.8 Hz, H-2′′, H-6′′), 7.63 (2H, d, J = 8.00 Hz, H-2, H-6), 7.79 (2H, d, J = 8.00 Hz, H-3′′, H-5′′). ESI-MS (m/z): 478[M+], 479[M++1], 481[M++3]. CHNS Analysis for C26H23ClN2O3S Found (Calculated): C: 65.20(65.23), H:4.84(4.85), Cl:7.40(7.43), N:5.85(5.84), O:10.02(10.04), S: 6.69(6.68).
(E)-4-(2-(3-(4-bromobenzylidene)-2-oxo-5-(p-tolyl)-2,3-dihydro-1H-pyrrol-1-yl)ethyl)benzenesulphonamide (3h)
Dark brown crystals, yield = 98%, m.p. 233–234 °C, Rf = 0.70[toluene: ethyl acetate: formic acid; 5:4:1]. IRυmax (KBr, in cm−1): 3745 and 3191(NH2), 1654 (C = O), 1331 and 1156 (SO2N). 1H NMR (400 MHz, DMSO-d6, δ): 2.38 (3H, s, CH3), 2.72 (2H, t, PhCH2CH2N), 3.88 (2H, t, PhCH2CH2N), 6.33 (1H, s, H-4 pyrrolone ring), 7.14 (2H, d, J = 8.4 Hz, H-3′ and H-5′ Ar1 ring), 7.21(s, 1H, olefinic H), 7.27–7.64 (10H, m, SO2NH2, aromatic protons of Ar1, Ar2 and sulphamoyl phenyl ring), 7.78 (2H, d, J = 8.00 Hz, H-3′′, H-5′′). ESI-MS(m/z):523[M+], 525[M++2]. CHNS Analysis for C26H23BrN2O3S Found (Calculated): C: 59.66(59.61), H:4.43 (4.45), Br:15.27(15.29), N: 5.35(5.34), O: 9.17 (9.07), S: 6.13(6.18).
(E)-4-(2-(3-benzylidene-5-(4-ethylphenyl)-2-oxo-2,3-dihydro-1H-pyrrol-1-yl)ethyl)benzenesulphonamide (3i)
Light yellow crystals, yield = 90%, m.p. 183–184 °C, Rf = 0.75[toluene: ethyl acetate: formic acid; 5:4:1]. IRυmax (KBr, in cm−1): 3390 and 3218 (NH2), 1653 (C = O), 1329 and 1155 (SO2N). 1H NMR (400 MHz, DMSO-d6, δ): 1.23 (3H, t, CH3CH2-), 2.65–2.74 (merged quartet of 2H of CH3CH2- with triplet of 2H of PhCH2CH2N), 3.89 (2H, t, PhCH2CH2N), 6.36 (1H, s, H-4 pyrrolone ring), 7.13 (2H, d, J = 7.60 Hz, H-3′ and H-5′), 7.24 (1H, s, olefinic H), 7.29 (2H, s, SO2NH2), 7.32–7.40 (5H, m, H-2′,H-6′, H-3, H-4, H-5), 7.48 (2H, d, J = 8.40 Hz, H-2′′ and H-6′′), 7.63(2H, d, J = 8.00 Hz, H-2 and H-6), 7.80(2H, d, J = 8.40 Hz, H-3′′ and H-5′′). 13C NMR (100 MHz, DMSO-d6, δ): 15.92, 28.51, 34.59, 41.85, 100.18, 126.16(2 C), 128.12(2 C), 128.59(2 C), 128.68(2 C), 129.45(2 C), 129.51, 129.88, 130.25, 132.47(2 C), 134.59, 134.62, 142.74, 142.82, 146.05, 150.31, 170.14. ESI-MS (m/z): 458[M+], 459[M++1]. CHNS Analysis for C27H26N2O3S Found (Calculated): C: 70.72(70.71), H:5.71(5.68), N: 6.11(6.24), O:10.47 (10.57), S: 6.99(6.89).
(E)-4-(2-(5-(4-ethylphenyl)-3-(4-fluorobenzylidene)-2-oxo-2,3-dihydro-1H-pyrrol-1-yl)ethyl)benzenesulphonamide (3j)
Light yellow crystals, yield = 88%; m.p. 185–186 °C, Rf = 0.74[toluene: ethyl acetate: formic acid; 5:4:1]. IRυmax (KBr, in cm−1): 3390 and 3218 (NH2), 1653 (C = O), 1329 and 1155 (SO2N). 1H NMR (400 MHz, DMSO-d6, δ): 1.19 (3H, t, CH3CH2-), 2.60–2.70 (quartet of 2H of CH3CH2 merged with triplet of 2H of PhCH2CH2N), 3.85 (2H, t, PhCH2CH2N), 6.33 (1H, s, H-4 pyrrolone ring), 7.09 (2H, d, J = 8.00 Hz, H-3′ and H-5′ Ar1 ring), 7.20 (1H, s, olefinic H), 7.26 (2H,s, SO2NH2), 7.30 (2H, d, J = 8.00 Hz, H-3 and H-5), 7.35 (2H, d, J = 8.00 Hz, H-2′ and H-6′), 7.45 (2H, d, J = 8.00 Hz, H-2′′ and H-6′′), 7.59 (2H, d, J = 8.00 Hz, H-2 and H-6), 7.77 (2H, d, J = 8.00 Hz, H-3′′ and H-5′′). ESI-MS (m/z):476[M+], 477[M++1], 478[M++2]. CHNS Analysis for C27H25FN2O3S Found (Calculated): C: 68.05(68.10), H:5.29 (5.39), F:3.99(3.89), N: 5.88(5.84), O: 10.07 (10.02), S: 6.73(6.78).
(E)-4-(2-(3-(4-chlorobenzylidene)-5-(4-ethylphenyl)-2-oxo-2,3-dihydro-1H-pyrrol-1-yl)ethyl)benzenesulphonamide (3k)
Dark brown crystals, yield = 95%, m.p. 186–187 °C, Rf = 0.73[toluene: ethyl acetate: formic acid; 5:4:1]. IRυmax (KBr, in cm−1): 3390 and 3218 (NH2), 1653 (C = O), 1329 and 1155 (SO2N). 1H NMR (400 MHz, DMSO-d6, δ): 1.22 (3H, t, CH3CH2-), 2.65–2.74 (quartet of 2H of CH3CH2 merged with triplet of 2H of PhCH2CH2N), 3.89 (2H, t, PhCH2CH2N), 6.37 (1H, s, H-4 pyrrolone ring), 7.13 (2H, d, J = 8.40 Hz, H-3′ and H-5′ Ar1 ring), 7.24 (1H, s, olefinic H), 7.313(2H,s, SO2NH2), 7.33 (2H, d, J = 8.00 Hz, H-2′ and H-6′ Ar1 ring), 7.39 (2H, d, J = 8.40 Hz, H-3 and H-5), 7.48(2H, d, J = 8.00 Hz, H-2′′ and H-6′′) , 7.63 (2H, d, J = 8.00 Hz, H-2 and H-6), 7.81 (2H, d, J = 8.40 Hz, H-3′′ and H-5′′). 13C NMR (100 MHz, DMSO-d6, δ): 15.96, 28.51, 34.58, 41.83, 100.18, 126.16(2 C), 128.11(2 C), 128.58(2 C), 128.69(2 C), 129.46(2 C), 129.51, 129.89, 130.20, 132.49(2 C), 134.57, 134.62, 142.71, 142.82, 146.04, 150.28, 170.13. ESI-MS(m/z): 493[M+], 495[M++2], 496[M++3]. CHNS Analysis for C27H25ClN2O3S Found (Calculated): C:65.78(65.61), H:5.11(5.15), Cl:7.19(7.13), N:5.68(5.71), O:9.74(9.77), S:6.50(6.58).
(E)-4-(2-(3-(4-bromobenzylidene)-5-(4-ethylphenyl)-2-oxo-2,3-dihydro-1H-pyrrol-1-yl)ethyl)benzenesulphonamide (3 l)
Dark brown crystals, yield =90%; m.p. 188–189 °C, Rf = 0.72[toluene: ethyl acetate: formic acid; 5:4:1]. IRυmax (KBr, in cm−1): 3390 and 3218 (NH2), 1653 (C = O), 1329 and 1155 (SO2N). 1H NMR (400 MHz, DMSO-d6, δ): 1.22 (3H, t, CH3CH2-), 2.67–2.73 (quartet of 2H of CH3CH2- merged with triplet of 2H of PhCH2CH2N), 3.88(2H, t, PhCH2CH2N), 6.35(1H, s, H-4 pyrrolone ring), 7.12(2H, d, J = 8.40 Hz, H-3′ and H-5′), 7.24(1H, s, olefinic H), 7.26 (2H, s, SO2NH2), 7.33(2H, d, J = 8.80 Hz, H-2′ and H-6′), 7.38(2H, d, J = 8.40 Hz, H-2′′ and H-6′′), 7.48(2H, d, J = 8.00 Hz, H3, H5), 7.62(2H, d, J = 8.00 Hz, H-2 and H-6), 7.80(2H, d, J = 8.80 Hz, H-3′′ and H-5′′). ESI-MS (m/z):537[M+], 539[M++2], 540[M++3]. CHNS Analysis for C27H25BrN2O3S Found (Calculated):C: 60.34(60.31), H:4.69 (4.65), Br:14.87(14.83), N: 5.21(5.34), O: 8.93(8.94), S: 5.97(5.88).
(E)-4-(2-(3-benzylidene-5-(4-chlorophenyl)-2-oxo-2,3-dihydro-1H-pyrrol-1-yl)ethyl)benzenesulphonamide (3 m)
Light yellow crystals, yield = 87%, m.p. 189–190 °C, Rf = 0.73[toluene: ethyl acetate: formic acid; 5:4:1]. IRυmax (KBr, in cm−1): 3456 and 3190 (NH2), 1670 (C = O), 1323 and 1148 cm−1 (SO2N).1H NMR (400 MHz, DMSO-d6, δ): 2.74 (2H, t, PhCH2CH2N), 3.89 (2H, t, PhCH2CH2N), 6.43 (1H, s, H-4 pyrrolone ring), 7.14 (2H, d, J = 8.00 Hz, H-2′ and H-6′), 7.31–7.46 (8H, m, SO2NH2, olefinic H and aromatic protons of Ar1, Ar2), 7.54 (2H, d, J = 8.40 Hz, H-2′′ and H-6′′),7.63(2H, d, J = 8.40 Hz, H-2 and H-6), 7.805 (2H, d, J = 8.00 Hz, H-3′′ and H-5′′). 13C NMR (125 MHz, DMSO-d6, δ): 34.54, 41.83, 100.1, 126.17(2 C), 129.34, 129.53(4 C), 129.91(2 C), 130.05(2 C), 130.40, 130.97(2 C), 132.40(2 C), 134.63, 135.52, 142.76, 142.81, 148.48, 170.10. ESI-MS(m/z): 464[M+], 465[M++1]. CHNS Analysis for C25H21ClN2O3S Found (Calculated): C:64.58(64.51), H:4.55(4.53), Cl:7.62(7.63), N: 6.02(6.04), O: 10.32 (10.37), S: 6.90(6.98).
(E)-4-(2-(5-(4-chlorophenyl)-3-(4-fluorobenzylidene)-2-oxo-2,3-dihydro-1H-pyrrol-1-yl)ethyl)benzenesulphonamide (3n)
Light yellow crystals, yield = 88%, m.p. 159-160 °C, Rf = 0.72[toluene: ethyl acetate: formic acid; 5:4:1]. IRυmax (KBr, in cm−1): 3456 and 3176 (NH2), 1670 (C = O), 1330 and 1156 cm−1 (SO2N). 1H NMR (400 MHz, DMSO-d6, δ): 2.74 (2H, t, PhCH2CH2N), 3.89 (2H, t, PhCH2CH2N), 6.43 (1H, s, H-4 pyrrolone ring), 7.17 (2H, d, J = 8.00 Hz, H-3 and H-5), 7.32 (2H, d, J = 8.00 Hz, H-2′ and H-6′), 7.43–7.47 (5H, m, SO2NH2, olefinic H and H-3′, H-5′), 7.54 (2H, d, J = 8.00 Hz, H-2′′ and H-6′′), 7.64(2H, d, J = 8.00 Hz, H-2 and H-6), 7.79 (2H, d, J = 8.00 Hz, H-3′′ and H-5′′). 13C NMR (125 MHz, DMSO-d6, δ): 34.59, 41.95, 100.94, 126.16(2 C), 129.36, 129.62(4 C), 129.95(2 C), 130.09(2 C), 130.44, 130.96(2 C), 132.33(2 C), 134.68, 135.54, 142.79, 142.87, 148.53, 170.10. ESI-MS(m/z): 482[M+], 483[M++1], 485[M++3]. CHNS Analysis for C25H20ClFN2O3S Found (Calculated): C: 62.17(62.21), H:4.17(4.25), Cl:7.34(7.43), F:3.93(3.87), N: 5.80(5.84), O: 9.94 (9.97),S: 6.64(6.68).
(E)-4-(2-(3-(4-chlorobenzylidene)-5-(4-chlorophenyl)-2-oxo-2,3-dihydro-1H-pyrrol-1-yl)ethyl)benzenesulphonamide (3o)
Light brown crystals, yield = 77%; m.p. 163–164 °C, Rf = 0.71[toluene: ethyl acetate: formic acid; 5:4:1]. IRυmax (KBr, in cm−1): 3456 and 3176 (NH2), 1670 (C = O), 1330 and 1156 cm−1 (SO2N). 1H NMR (400 MHz, DMSO-d6, δ): 2.73(2H, t, PhCH2CH2N), 3.87(2H, t, PhCH2CH2N), 6.41(1H, s, H-4 pyrrolone ring), 7.12(2H, d, J = 8.40 Hz, H-2′ and H-6′), 7.21–7.53 (9H, m, SO2NH2, olefinic H, H-3, H-5, H-2′′, H-6′′and H-3′, H-5′), 7.62(2H, d, J = 8.00 Hz, H-2 and H-6), 7.81(2H, d, J = 8.00 Hz, H-3′′ and H-5′′). ESI-MS (m/z): 499[M+], 500[M++1], 501[M++2]. CHNS Analysis for C25H20Cl2N2O3S Found (Calculated): C:60.12(60.13), H:4.04(4.05), Cl:14.20(14.23), N: 5.61(5.64), O: 9.61 (9.67), S: 6.42(6.43).
(E)-4-(2-(3-(4-bromobenzylidene)-5-(4-chlorophenyl)-2-oxo-2,3-dihydro-1H-pyrrol-1-yl)ethyl)benzenesulphonamide (3p)
Dark brown crystals, yield = 85%, m.p. 176–177 °C, Rf = 0.70 [toluene: ethyl acetate: formic acid; 5:4:1]. IRυmax (KBr, in cm−1): 3456 and 3176 (NH2), 1670 (C = O), 1330 and 1156 cm−1 (SO2N). 1H NMR (400 MHz, DMSO-d6, δ): 2.73 (2H, t, PhCH2CH2N), 3.87 (2H, t, PhCH2CH2N), 6.40(1H, s, H-4 pyrrolone ring), 7.12 (2H, d, J = 8.00 Hz, H-2′ and H-6′), 7.35 (1H, s, olefinic H), 7.37 (2H, s, SO2NH2), 7.44 (2H, d, J = 8.00 Hz, H-3′ and H-5′), 7.52 (2H, d, J = 8.00 Hz, H-2′′ and H-6′′), 7.61–7.65 (4H, m, H-2, H-3, H-5, H-6), 7.72 (2H, d, J = 8.00 Hz, H-3′′ and H-5′′). ESI-MS (m/z): 542[M+-1], 543[M+]. CHNS Analysis for C25H20BrClN2O3S Found(Calculated): C:55.21(55.31), H:3.71(3.75), Br:14.69(14.63), Cl:6.52(6.57), N: 5.15(5.14), O: 8.83(8.87), S: 5.90(5.88).
(E)-4-(2-(3-benzylidene-5-(4-(tert-butyl)phenyl)-2-oxo-2,3-dihydro-1H-pyrrol-1-yl)ethyl)benzenesulphonamide (3q)
Light brown crystals, yield = 87%, m.p. 183–184 °C, Rf = 0.71[toluene: ethyl acetate: formic acid; 5:4:1].IRυmax (KBr, in cm−1): 3747 and 3208 (NH2), 1675 (C = O), 1330 and 1151 cm−1 (SO2N). 1HNMR (400 MHz, DMSO-d6, δ): 1.28 (9H, s, 3 × CH3), 2.67(2H, t, PhCH2CH2N), 3.84 (2H, t, PhCH2CH2N), 6.33(1H, s, H-4 pyrrolone ring), 7.06 (2H, d, J = 8.00 Hz, H-3′ and H-5′), 7.21 (1H,s olefinic H), 7.24(2H, s, SO2NH2), 7.35 (2H, d, J = 8.00 Hz, H-2′ and H-6′), 7.44–7.48 (5H, m, H-3, H-4, H-5, H-2′′, H-6′′), 7.57 (2H, d, J = 8.00 Hz, H-2 and H-6), 7.77(2H, d, J = 8.00 Hz, H-3′′ and H-5′′). ESI-MS(m/z): 486[M+], 487[M++1]. CHNS Analysis for C29H30N2O3S Found (Calculated): C: 71.58(71.56), H:6.21(6.25), N: 5.76(5.84), O: 9.86 (9.97), S: 6.59(6.58).
(E)-4-(2-(5-(4-(tert-butyl)phenyl)-3-(4-fluorobenzylidene)-2-oxo-2,3-dihydro-1H-pyrrol-1-yl)ethyl)benzenesulphonamide (3r)
Light yellow crystals, yield = 77%, m.p. 187–188 °C, Rf = 0.71[toluene: ethyl acetate: formic acid; 5:4:1]. IRυmax (KBr, in cm−1): 3456 and 3286 (NH2), 1673 (C = O), 1375 and 1178 cm−1 (SO2N). 1H NMR (400 MHz, DMSO-d6, δ): 1.27 (9H, s, 3 × CH3), 2.67 (2H, t, PhCH2CH2N), 3.86 (2H, t, PhCH2CH2N), 6.34 (1H, s, H-4 pyrrolone ring), 7.06 (2H, d, J = 8.00 Hz, H-3 and H-5), 7.21 (1H, s, olefinic H), 7.26 (2H, s, SO2NH2), 7.35(2H, d, J = 8.00 Hz, H-3′ and H-5′), 7.44–7.48 (4H, m, H-2′, H-6′, H-2′′, H-6′′), 7.57 (2H, d, J = 8.00 Hz, H-2 and H-6), 7.77 (2H, d, J = 8.00 Hz, H-3′′ and H-5′′). ESI-MS(m/z): 504[M+], 505[M++1], 506[M++2]. CHNS Analysis for C29H29FN2O3S Found (Calculated): C: 69.02(69.07), H:5.79 (5.75), F:3.76(3.77), N: 5.55(5.54), O: 9.51 (9.57), S: 6.35(6.38).
(E)-4-(2-(5-(4-(tert-butyl)phenyl)-3-(4-chlorobenzylidene)-2-oxo-2,3-dihydro-1H-pyrrol-1-yl)ethyl)benzenesulphonamide (3s)
yellow crystals, yield = 95%, m.p. 196–197 °C, Rf = 0.70[toluene: ethyl acetate: formic acid; 5:4:1].IRυmax (KBr, in cm−1): 3456 and 3286 (NH2), 1673 (C = O), 1375 and 1178 cm−1 (SO2N). 1H NMR (400 MHz, DMSO-d6, δ): 1.30 (9H, s, 3 × CH3), 2.67 (2H, t, PhCH2CH2N), 3.86 (2H, t, PhCH2CH2N), 6.32(1H, s, H-4 pyrrolone ring), 7.06 (2H, d, J = 8.00 Hz, H-3′ and H-5′), 7.21 (1H, s, olefinic H), 7.25 (2H, s, SO2NH2), 7.35(2H, d, J = 8.00 Hz, H-2′ and H-6′), 7.40–7.48 (4H, m, H-3, H-5, H-2′′, H-6′′), 7.57(2H, d, J = 8.00 Hz, H-2 and H-6), 7.77 (2H, d, J = 8.00 Hz, H-3′′ and H-5′′). ESI-MS(m/z): 521[M+], 522[M++1]. CHNS Analysis for C29H29ClN2O3S Found (Calculated): C: 66.85(66.81), H:5.61 (5.65), Cl:6.80(6.87), N: 5.38(5.34), O: 9.21 (9.37), S: 6.15(6.18).
(E)-4-(2-(3-(4-bromobenzylidene)-5-(4-(tert-butyl)phenyl)-2-oxo-2,3-dihydro-1H-pyrrol-1-yl)ethyl)benzenesulphonamide (3t)
Dark brown crystals, yield = 92%, m.p. 204–205 °C, Rf = 0.70[toluene: ethyl acetate: formic acid; 5:4:1]. IRυmax (KBr, in cm−1): 3456 and 3286(NH2), 1673 (C = O), 1375 and 1178 cm−1 (SO2N). 1H NMR (400 MHz, DMSO-d6, δ): 1.28 (9H, s, 3 × CH3), 2.66 (2H, t, PhCH2CH2N), 3.85 (2H, t, PhCH2CH2N), 6.34 (1H, s, H-4 pyrrolone ring), 7.06 (2H, d, J = 8.00 Hz, H-3′ and H-5′), 7.21 (1H, s, olefinic H), 7.25 (2H, s, SO2NH2), 7.35 (2H, d, J = 8.00 Hz, H-2′ and H-6′), 7.43–7.48 (4H, m, H-3, H-5, H-2′′, H-6′′), 7.57 (2H, d, J = 8.00 Hz, H-2 and H-6), 7.77 (2H, d, J = 8.00 Hz, H-3′′ and H-5′′). ESI-MS(m/z): 565[M+], 567[M++2]. CHNS Analysis for C29H29BrN2O3S Found (Calculated): C: 61.59(61.56), H:5.17 (5.25), Br:14.13(14.17), N: 4.95(4.84), O: 8.49 (8.57),S: 5.67(5.68).
Carbonic anhydrase inhibition assay
By using Stopped-Flow CO2 hydrase assay, derivatives 3a–t were tested as inhibitors of the hCAs I, II, IX, and XII. Acetazolamide (AAZ), a commonly used sulphonamide inhibitor was used for comparing the inhibition data. The Inhibition effects of these compounds on enzyme activities were evaluated under in-vitro conditions. The CA catalysed CO2 hydration activity was measured using an Applied Photophysics stopped-flow instrument. Working at the maximum absorbance of 557 nm, phenol red (at a concentration of 0.2 mM) has been employed as an indicator, with 20 mM Na2SO4 (for maintaining constant the ionic strength) and 20 mM Hepes (pH 7.5) as buffer, following the initial rates of the CA-catalysed CO2 hydration reaction for a duration of 10–100 s. The CO2 concentrations used to determine the inhibition constants and kinetic parameters ranged from 1.7 to 17 mM. At least six traces of the initial 5–10% of the reaction have been used for measuring the initial velocity for every inhibitor. In the same way, the uncatalyzed rates were analysed and deducted from the total measured rates. Preparation of stock solutions of the inhibitor (0.1 mM) in distilled- deionised water was carried out, followed by the dilutions up to 0.05 nM by the assay buffer. The inhibitor and enzyme solutions were preincubated at room temperature for 15 min prior to the experiment to promote the formation of the E-I complex. By employing nonlinear least-squares methods using PRISM 3 and the Cheng-Prusoff equation, the inhibition constants were determined, as was previously reportedCitation18–20,Citation21 and indicate the average of at least three separate measurements that fall between the range of 5 and 10%. As reported earlierCitation22–24 all isoforms were recombinant ones obtained in house and their concentrations in the assay system was in the range of 6.8 − 12.4 nM.
ADMET Analysis
Using the Qikprop module of Maestro 9.6 Schrodinger programme, the ADMET evaluations of the compounds (3a-t) were carried out. It offers a set of parameters to compare a given molecule’s characteristics with 95% of all the known drugs. Various molecular descriptors were calculated that influence the physicochemical characteristics of a molecule. Based on the chemical structures of the molecules, the theoretical parameters were computed. Various descriptors such as human oral absorption, gut–blood barrier permeability, partition coefficient, CNS (central nervous system) activity were calculatedCitation25.
DFT study for E and Z isomers of compound 3n
Density functional theory (DFT) studies were carried for both the geometrical isomers (E and Z) of compound 3n using ORCA Program Package Version 4.2Citation26. We employed B3LYP functionalCitation27,Citation28 and def2-TZVPD3BJ basis setsCitation29 to perform the DFT calculations of the compounds.
Results and discussion
Chemistry
The current research is an expansion of our ongoing initiativeCitation12,Citation30–33 to create potentially and biologically active drugs by adopting a hybrid pharmacophore strategy. Designing and synthesising of hybrid molecules were achieved by joining the pyrrolone ring through two methylene groups with benzene sulphonamide. Here, we present the synthesis and inhibitory profile of twenty novel (E)-1–(4-sulfamoylphenylethyl)-3-arylidene-5-aryl-1H-pyrrol-2(3H)-ones (3a-t) against two cystosolic (hCA I and II) and two membrane bound, tumour-related hCA IX and XII isoforms. The exocyclic double bond in the target compounds (3a–t) allows for the possibility of either E or Z-isomers. The (E)-configuration appears to be suggested by calculations of δ values using incremental parameters for the hydrogen (semicyclic double bond). This is consistent with the results that have previously been reportedCitation14. A nuclear overhauser effect (NOE) experiment was carried to analyse the compound 3k in order to ascertain the geometry of the target compounds. The interaction between an olefinic proton and H-4 on the pyrrolone ring would be clearly visible through NOE analysis if 3k is the Z isomer. The fact that an olefinic proton did not engage in interaction with H-4 on the pyrrolone ring suggests the E form (Figure S1 and S2). The outcome of the inhibitory efficacy of such sulphonamide derivatives displayed good results and further strengthened the significance of the sulphonamides in the medicinal chemistry field of CAIs.
Synthesis of target compounds (3a-t) was accomplished using a four-step procedure described in Scheme 1. Cyclisation of appropriate γ-keto-4-sulfamoylphenylethylamide (2a-t) in HCl yields (E)-1–(4-sulphamoylphenylethyl)-3-arylidene-5-aryl-1H-pyrrol-2(3H)-ones (3a-t). Treatment of appropriate E form of 3-arylidene-5-(aryl)-furan-2(3H)-ones (1a-t) with equimolar quantity of 4–(2-aminoethyl)benzenesulphonamide in dry benzene yields compounds (2a-t).The desired intermediates i.e., E form of 3-arylidene-5-(aryl)-furan-2(3H)-ones (1a-t) were synthesised by fusion of appropriate 1,4-ketoacids (β-aroylpropionic acids) and aromatic aldehyde using acetic anhydride in presence of triethylamine through reported methodCitation34,Citation35. Compound purity was monitored using TLC plates (silica gel G), and was further detected by exposure to UV lamp and iodine vapours.
Scheme 1. Synthesis of pyrrolone substituted ethyl benzene sulphonamide derivatives (3a-t).
Reagents and conditions (a) Anhydrous AlCl3, stirring at room temperature, 6h; (b) Acetic anhydride, Triethylamine, reflux, 4-5 h; (c) Dry benzene, Reflux, 4h; (d) 6M HCl, reflux, 2h).
1a-3a, 1b-3b, 1c-3c, 1d-3d; R1=H,1e-3e, 1f-3f, 1g-3g, 1h-3h; R1= CH3, 1i-3i, 1j-3j, 1k-3k, 1l-3l; R1=C2H5, 1m-3m, 1n-3n, 1o-3o, 1p-3p; R1=Cl, 1q-3q, 1r-3r, 1s-3s, 1t-3t; R1=-C(CH3)3, 1a-3a, 1e-3e, 1i-3i, 1m-3m, 1q-3q; R2=H, 1b-3b, 1f-3f, 1j-3j, 1n-3n, 1r-3r; R2=F, 1c-3c, 1g-3g, 1k-3k, 1o-3o, 1s-3s; R2=Cl, 1d-3d, 1h-3h, 1l- 3l, 1p-3p, 1t-3t; R2=Br.
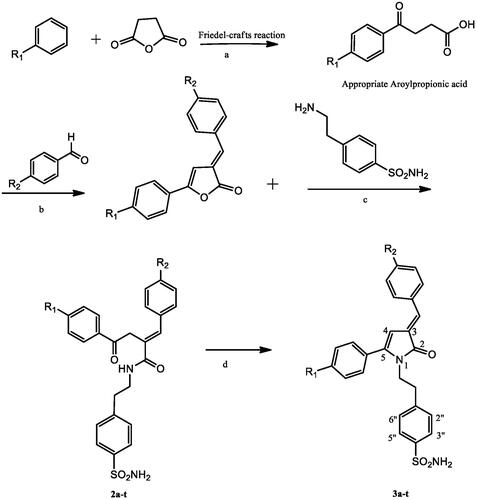
The structures of pyrrolone derivatives (3a-t) were confirmed by spectroscopic methods such as IR, 1H NMR, 13C NMR, MS and elemental analysis. Compounds were obtained in the good yield of around 70–95%. Characteristic stretching bands in the IR spectra for compounds 3a-t were observed: two bands for NH2 (3747–3176 cm−1 and 3227–3286 cm−1), one band for carbonyl group of pyrrolone moiety (1676–1653 cm−1) and two bands for SO2N (1375–1323 cm−1 and 1178–1148 cm−1). 1H NMR spectrum showed two protons triplets at δ = 2.60–2.82 and δ = 3.84–3.93 which can be ascribed to -CH2-CH2- unit. One proton singlet which appeared at δ = 6.08–6.43 can be assigned to H-4 of pyrrolone ring. SO2NH2 protons appeared as singlet at δ = 4.81–7.43. Another proton singlet appeared at δ = 7.20–7.36 and is accountable for olefinic-H. In addition, Density Functional Theory (DFT) calculations of 3n-E and 3n-Z were performed to gain more understanding about the stability of the E and Z isomers. The energy values clearly indicate the stability of E-isomer over the Z-isomer by −8.2 kJ mol−1 which was further supported by Frontier Molecular Orbital (FMO) analysis of these isomers. In the 13C NMR spectra, chemical shift value of carbon atoms, were noticed in the expected range with -CH2-CH2-N- unit carbon peaks appearing at (δ = 34.54–34.59) and (δ = 41.83–41.95) respectively while as C-4 of pyrrolone ring appeared in range of (δ =100.1–100.94) and the peak of C-2 appeared at (δ =170.06 − 170.24).
Carbonic anhydrase inhibition activity
In the current study, Carbonic anhydrase inhibitory effect of 20 novel synthesised compounds (3a-t) was tested under in vitro condition against two cystolic (hCA I and II) and membrane bound tumour related isoforms i.e., hCA IX and XII, along with the standard inhibitor acetazolamide (AAZ) in a stopped-flow CO2 hydrase assayCitation36. The inhibition data (Ki) was determined with the help of Cheng-Prusoff equation as mentioned in the Materials and methods section. In selecting these four isoforms it was taken into account that hCA II is a target site for glaucomaCitation37 and hCA IX and XII have been authenticated as hypoxic malignancy targetsCitation38,Citation39. However, for anti-glaucoma and anticancer CAIs therapeutic applicationsCitation6,Citation40 hCA I is considered as the essential off-target isoform.
Based on of the CA inhibition assay, following assumptions were made:
Table 1. Carbonic anhydrase inhibition data of compounds (3a-t).
The inhibition profile of the compounds against the four CA isoforms was affected by the substitution of halogen groups at the para position of the arylidene ring (C-3) directly attached to pyrrolone nucleus. Compound 3b was found to be most active inhibitor against membrane bound cytosolic hCAI and II isoform having KI values of 368.7 and 81.4 nM respectively while as 3n was found to be more effective against the tumour-associated hCA IX and XII isoforms having Ki value of 41.3 and 39.1 nM respectively. Hydrophobicity of the pyrrolone moiety may facilitate the inhibition of hCA I, II, IX and XII isozymes. It was further noted that by introducing a strong electron withdrawing group, that is, fluoro at the para position of arylidene ring (C-3) the inhibitory activity of such compounds increased because of strong binding affinity to the active site of enzyme thus making such compounds efficient and tight binding inhibitors than the rest of the compounds of the series.Citation41–43 Alternatively other functionalisations also adversely effect the inhibition potency, i.e. incorporation of less hindered groups at the para position of aryl ring (C-5) of pyrrolone nucleus, maintained certain degree of activity than more hindered groups. Overall, the SAR analysis reveals that hydrophobic and bulky substituents are detrimental to all isoforms; however, the presence of a fluorine atom always improves Ki values except for the simultaneous presence of tertiary butyl substituent.
All pyrrolone substituted ethyl benzene sulphonamides showed Kis between 368.7- 9272nM for the cytosolic isoform hCAI. Compound 3b had effective inhibition for this isoform (Ki = 368.7), while as 3a and 3j had moderate inhibition activity with (KiS = 634.1 and 836.5 nM) and 3q and 3t lead to weaker inhibition in hCAI isoform (KiS= 8.5 and 9.3µM) respectively.
hCAII isoform was inhibited more efficiently by sulphonamide derivatives (KiS=81.4- 4687nM) compared to hCAI. Compound 3b was more effective inhibitor for this isoform (Ki=81.4nM). However, compounds 3a, 3c, 3e, 3f, 3g, 3i, 3j, 3m, 3n had medium nanomolar inhibition activity (KiS=368.5, 718, 471.4, 146.9, 941.7, 901.5, 295.7, 780, 465.6nM) respectively, whereas compounds 3s and 3t (KiS=4.3 and 4.6µM) had weak inhibition activity.
All these primary sulphonamides exhibited significant potency towards the tumour associated isoform hCA IX among all the isoforms (Kis spanning between 41.3 – 468.5nM). Compound 3n was the most potent inhibitor for this isoform (Ki = 41.3 nM) relative to AAZ (Ki = 25.7nM). However, compounds 3a, 3b, 3g, 3h, 3i, 3j, 3l, 3m, 3o, 3p having (Kis=93.8, 72.7, 95.5, 84.2, 78.3, 71.4, 77.5, 54.6, 68.9, 74.3 nM) moderately inhibited the hCA IX isoform, whereas other compounds 3c, 3d, 3e, 3f, 3k, 3q, 3r, 3s, 3t (Kis = 108.3, 111.6, 116.7, 103.2, 101.6, 468.5, 417.7, 432.4, 391.2 nM) showed lower binding affinity, in comparison to other compounds of the series.
The other tumour-associated isoform, that is, hCA XII showed similar tendencies as described above for hCA IX, with Kis ranging from 39.1 to 396.2 nM. Compound 3n was the most potent inhibitor for this isoform (Ki = 39.1nM).Compounds 3a-m (Kis=62.1, 45.6, 76.5, 89.8, 58.4, 42.7, 73.4, 94.0, 83.9, 66.2, 91.1, 56.3, 61.7 nM), 3o-p (Kis = 78.9 and 85.4 nM) moderately inhibited hCA XII, whereas 3q-t (KiS=0.39, 0.35, 0.34, 0.30µM) showed lower binding affinity in comparison to other compounds of the series.
From the brief examination of the data, it is evident that the compounds that have been synthesised and investigated for the activity showed low to high nanomolar efficacy against cytosolic hCA II isoform while the efficiency towards hCA I decreased dramatically. Also the inhibitory efficacy towards tumour associated isoform i.e. hCA IX and hCA XII settled in the low nanomolar range, therefore, making this distinct series of compounds worthwhile, thus indicating new class of possible CAIs of medicinal interest.
ADMET Analysis
The ADMET analysis of 3a-t were carried with the Qikprop tool of Schrodinger. Molecular descriptors were calculated that impact the physicochemical characteristics of a compound. In the present ADMET analysis, drug-likeness properties and in silico ADME evaluation for all synthesised compounds were analysed. The different parameters predicted were; molecular weight (M. Wt.), number of hydrogen bond donor (HBD), number of hydrogen bond acceptor (HBA), octanol/water partition coefficient (log P) and Percentage of human oral absorption. Lipinski’s rule of five values are within their acceptable range, except for the molecular weight. Most of the titled compounds were following the parameters that were followed by majority of the approved drugs. Partition coefficient predicted values were within the acceptable range. Despite of all parameter, human oral absorption were found to be higher for all compounds and within the limit while as 3k and 3q had highest human oral absorption. The results are presented in .
Table 2. ADMET analysis of the compounds against hCAI, hCAII, hCAIX and hCAXII isozymes.
DFT study for E and Z isomers of compound 3n
Density Functional Theory (DFT) calculations were carried to gain more understanding about the stability of the 3n-E and 3n-Z isomers. Initial structures of both the isomers were visualised through UCSF Chimaera 1.12 Visualisation PackageCitation44 and then optimized using ORCA Program Package version 4.2Citation26 at B3LYP/def2-TZVP D3BJ level of theory.Citation27–29 The optimized structures and the corresponding optimized coordinates of both the isomers 3n-E and 3n-Z are shown in and Tables SX and SY of the Supporting Information (SI), respectively. After successful optimizations of both the isomers, the total energy of the 3n-E isomer is −2255.653129872 Ha, while as total energy of the 3n-Z isomer is found to be −2255.650017417Ha. The energy values clearly indicate that the stability of E-isomer over Z-isomer by −8.2 kJ mol−1. To get more insight in the stability of both the isomers, we also carried Frontier Molecular Orbital (FMO) analysis of these isomers at the same level of theory. FMO analysis has been found a useful tool in explaining reactivity and stability of various organic systems.Citation45–47 In the FMO Analysis, the energy gap (ΔE) is calculated from the difference between the Energy of Lowest Unoccupied Molecular Orbital (ELUMO) and the Energy of Highest Occupied Molecular Orbital (EHOMO) according to the Koopmans’ relation (). In Conceptual DFT terminology, this energy gap (ΔE) is also called as chemical hardness (
)Citation48,Citation49. presents the FMO plots of the optimised structures (3n-E and 3n-Z isomers). As shown in , the EHOMO and ELUMO of the E-isomer is found to be −5.62420 eV and −2.42361 eV respectively, whereas the EHOMO and ELUMO of the Z-isomer is found to be −5.53132 eV and −2.45462 eV. The computed energy gap (ΔE) or chemical hardness (
) for 3n-E and 3n-Z isomers is 3.20059 eV and 3.07671 eV respectively. Maximum hardness principleCitation50 states that, “the more the value of ΔE or
more stable is the system and less is the reactivity”. Therefore, it may be concluded that E-isomer is more stable i.e., chemically more hard and hence less susceptible to attack by different chemical species in the reaction mixture, while as the Z-isomer is comparatively chemically less hard or more soft. It suggests that soft chemical species are more susceptible to electrophilic or nucleophilic attack, hence possibility of isolating Z-isomer is less as it might have been dissociated in the reaction mixture because of its soft nature and less stability as discussed.
Conclusions
In the present study, 20 new hybrid molecules incorporating pyrrolone nucleus with ethyl benzenesulphonamide moiety 3a-t were synthesised. Spectroscopic data and elemental analysis support the structures proposed for the synthesised compounds. These compounds along with acetazolamide, were tested as inhibitors of the physiologically relevant hCA isoforms, that is, hCAI, II, IX and XII. The potency of all compounds ranged from low nanomolar to high nanomolar. The compound 3b behaved as more effective inhibitor against the human cytosolic isoforms hCA I and II having Ki values (368.7 and 81.4 nM) respectively, while 3n behaved as more effective inhibitor against the membrane bound cancer related isozyme hCA IX and XII, having Ki values (41.3 and 39.1 nM) respectively. The results of ADMET studies provided a clear picture that all compounds have acceptable pharmacokinetic range and physicochemical characteristics. Density Functional Theory (DFT) calculations of 3n were carried to gain more understanding about the stability of the E and Z isomers. The energy values clearly indicate the stability of E-isomer over Z-isomer by −8.2 kJ mol−1 which is further supported by Frontier Molecular Orbital (FMO) analysis of these isomers. Results of our investigation suggest another possible class of carbonic anhydrase inhibitors of medicinal importance, hence making these molecules as promising candidates for the discovery of new and effective inhibitor of carbonic anhydrase.
Supplemental Material
Download PDF (5 MB)Acknowledgements
The authors convey their profound sense of appreciation to Indian institute of Science, Bangalore, India, University Science instrumentation centre, Delhi university, New Delhi, India for providing NMR facility and Jamia Hamdard, New Delhi, India for providing Mass spectra Facility to carry out spectral analysis. The authors are also grateful to Dr. Mahesh Sundararajan, BARC, Mumbai, India for his valuable scientific inputs.
Disclosure statement
All authors except CTS report no conflict of interest. CT Supuran is Editor-in-Chief of the Journal of Enzyme Inhibition and Medicinal Chemistry. He was not involved in the assessment, peer review, or decision-making process of this paper. The authors have no relevant affiliations of financial involvement with any organisation or entity with a financial interest in or financial conflict with the subject matter or materials discussed in the manuscript. This includes employment, consultancies, honoraria, stock ownership or options, expert testimony, grants or patents received or pending, or royalties.
Correction Statement
This article has been republished with minor changes. These changes do not impact the academic content of the article.
Additional information
Funding
References
- Supuran CT. Carbonic anhydrases: novel therapeutic applications for inhibitors and activators. Nat Rev Drug Discov. 2008;7(2):168–181.
- Supuran CT. Carbon- versus sulphur-based zinc binding groups for carbonic anhydrase inhibitors? J Enzyme Inhib Med Chem. 2018;33(1):485–495.
- Carta F, Supuran CT, Scozzafava A. Sulfonamides and their isosters as carbonic anhydrase inhibitors. Future Med Chem. 2014;6(10):1149–1165.
- Supuran CT. Carbonic anhydrases as drug targets–an overview. Curr Top Med Chem. 2007;7(9):825–833.
- Nocentini A, Donald WA, Supuran CT. Human carbonic anhydrases: tissue distribution, physiological role, and druggability. In: Supran CT, Nocentini A, editors. Carbonic anhydrases. Cambridge: Academic press; 2019. p. 151–185.
- Aspatwar A, Parvathaneni NK, Barker H, Anduran E, Supuran CT, Dubois L, Lambin P, Parkkila S, Winum J-Y. Design, synthesis, in vitro inhibition and toxicological evaluation of human carbonic anhydrases I, II and IX inhibitors in 5-nitroimidazole series. J Enzyme Inhib Med Chem. 2020; 35(1):109–117.
- Supuran CT. Experimental Carbonic Anhydrase Inhibitors for the Treatment of Hypoxic Tumors. J Exp Pharmacol. 2020;12:603–617.
- Balaydin HT, Durdaği S, Ekinci D, Sentürk M, Göksu S, Menzek A. Inhibition of human carbonic anhydrase isozymes I, II and VI with a series of bisphenol, methoxy and bromophenol compounds. J Enzyme Inhib Med Chem. 2012;27(4):467–475.
- Oudah KH, Najm MAA, Roomi AB, Al-Saidy HA, Awadallah FM. The recent progress of sulfonamide in medicinal chemistry. Syst Rev Pharm. 2020; 11(12):1473–1477.
- Supuran CT. Carbonic anhydrases: from biomedical applications of the inhibitors and activators to biotechnological use for CO(2) capture. J Enzyme Inhib Med Chem. 2013;28(2):229–230.
- Alterio V, Vitale RM, Monti SM, Pedone C, Scozzafava A, Cecchi A, De Simone G, Supuran CT. Carbonic anhydrase inhibitors: X-ray and molecular modeling study for the interaction of a fluorescent antitumor sulfonamide with isozyme II and IX. J Am Chem Soc. 2006;128(25):8329–8335.
- Yaseen R, Ekinci D, Senturk M, Hameed AD, Ovais S, Rathore P, Samim M, Javed K, Supuran CT. Pyridazinone substituted benzenesulfonamides as potent carbonic anhydrase inhibitors. Bioorg Med Chem Lett. 2016;26(4):1337–1341.
- Ahmad A, Husain A, Khan SA, Mujeeb M, Bhandari A. Design, synthesis, molecular properties and antimicrobial activities of some novel 2 (3H) pyrrolone derivatives. J Saudi Chem Soc. 2015;196:340–346.
- Husain A, Khan MSY, Hasan SM, Alam MM. 2-Arylidene-4-(4-phenoxy-phenyl)but-3-en-4-olides: synthesis, reactions and biological activity. Eur J Med Chem. 2005;40(12):1394–1404.
- Zykova SS, Boĭchuk SV, Galembikova AR, Ramazanov BR, Mustafin IG, Igidov NM, et al. 3-Hydroxy-1,5-diaryl-4-pivaloyl-2,5-dihydro-2-pyrrolones induce the mitotic exit failure and cell death in tumor cells in vitro]. Tsitologiia. 2014;56:439–442.
- Olla S, Manetti F, Crespan E, Maga G, Angelucci A, Schenone S, Bologna M, Botta M. Indolyl-pyrrolone as a new scaffold for Pim1 inhibitors. Bioorg Med Chem Lett. 2009;19(5):1512–1516.
- Alam MM, Husain A, Hasan SM, Anwer T., Suruchi Synthesis and pharmacological evaluation of 2(3H)-furanones and 2(3H)-pyrrolones, combining analgesic and anti-inflammatory properties with reduced gastrointestinal toxicity and lipid peroxidation. Eur J Med Chem. 2009;44(6):2636–2642.
- Oudah KH, Najm MAA, Samir N, Serya RAT, Abouzid KAM. Design, synthesis and molecular docking of novel pyrazolo[1,5-a][1,3,5]triazine derivatives as CDK2 inhibitors. Bioorg Chem. 2019;92:103239.
- Yazdani E, Kazemi Miraki M, Salamatmanesh A, Azarnia J, Azizi K, Ghandi L, Heydari A. A magnetically recoverable copper–salen complex as a nano-catalytic system for amine protection via acetylation using thioacetic acid. Res Chem Intermed. 2019; 45(4):1775–1793.
- Das VK, Devi RR, Thakur AJ. Recyclable, highly efficient and low cost nano-MgO for amide synthesis under SFRC: A convenient and greener “NOSE” approach. Appl Catal A Gen. 2013;456:118–125.
- Song B, Xiao T, Qi X, Li L-N, Qin K, Nian S, Hu G-X, Yu Y, Liang G, Ye F. Design and synthesis of 8-substituted benzamido-phenylxanthine derivatives as MAO-B inhibitors. Bioorg Med Chem Lett. 2012;22(4):1739–1742.
- Gawel JM, Shouksmith AE, Raouf YS, Nawar N, Toutah K, Bukhari S, Manaswiyoungkul P, Olaoye OO, Israelian J, Radu TB, et al. PTG-0861: a novel HDAC6-selective inhibitor as a therapeutic strategy in acute myeloid leukaemia. Eur J Med Chem. 2020;201:112411.
- Basanagouda M, Kulkarni MV, Kalkhambkar RG, Kulkarni GM. ChemInform abstract: new, efficient, selective, and one-pot method for acylation of amines. ChemInform. 2008;38:2929–2940.
- Das VK, Devi RR, Raul PK, Thakur AJ. Nano rod-shaped and reusable basic Al2O3 catalyst for N-formylation of amines under solvent-free conditions: A novel, practical and con_venient NOSE’ approach. Green Chem. 2012;14:847–854.
- Sharma K, Neshat N, Sharma S, Giri N, Srivastava A, Almalki F, Saifullah K, Alam MM, Shaquiquzzaman M, Akhter M, et al. Identification of novel selective Mtb-DHFR inhibitors as antitubercular agents through structure-based computational techniques. Arch Pharm (Weinheim)). 2020;353(2):e1900287.
- Neese F, Wennmohs F, Becker U, Riplinger C. The ORCA quantum chemistry program package. J Chem Phys. 2020;152(22):224108.
- Axel DB. Density‐functional thermochemistry. III. The role of exact exchange. J Chem Physics. 1993;98:5648–5652.
- Lee C, Yang W, Parr RG. Development of the Colle-Salvetti correlation-energy formula into a functional of the electron density. Phys Rev B Condens Matter. 1988;37(2):785–789.
- Zarić MM, Bugarski BK. Best methods for calculating interaction energies in 2-butene and butane systems. Comput Theor Chem. 2017;1117:150–161.
- Bashir R, Ovais S, Yaseen S, Hamid H, Alam MS, Samim M, Singh S, Javed K. Synthesis of some new 1,3,5- trisubstituted pyrazolines bearing benzene sulfonamide as anticancer and anti-inflammatory agents. Bioorg Med Chem Lett. 2011;21(14):4301–4305.
- Rathish IG, Javed K, Ahmad S, Bano S, Alam MS, Akhter M, Pillai KK, Ovais S, Samim M. Synthesis and evaluation of anticancer activity of some novel 6-aryl-2-(p-sulfamylphenyl)-pyridazin-3(2H)-ones. Eur J Med Chem. 2012;49:304–309.
- Ovais S, Javed K, Yaseen S, Bashir R, Rathore P, Yaseen R, Hameed AD, Samim M. Synthesis, antiproliferative and anti-inflammatory activities of some novel 6-aryl-2-(p-(methanesulfonyl)phenyl)-4,5-dihydropyridazi-3(2H)-ones. Eur J Med Chem. 2013;67:352–358.
- Rathore P, Yaseen S, Ovais S, Bashir R, Yaseen R, Hameed AD, et al. Synthesis and evaluation of some new pyrazoline substituted benzenesulfonylureas as potential antiproliferative agents. Bioorg Med Chem Lett. 2014;24:1685–1691.
- Khan MSY, Husain A. Synthesis and reactions of some new 2-arylidene-4-(biphenyl-4-yl)-but-3-en-4-olides with a study of their biological activity. Pharmazie. 2002;57:448–452.
- Khan MSY, Husain A, Sharma S. Studies on butenolides: 2-Arylidene-4-(substituted aryl)but-3-ene-4-olides synthesis, reactions and biological activity. Indian J Chem. 2002 2002;41B:2160–2171.
- Khalifah RG. The carbon dioxide hydration activity of carbonic anhydrase. I. Stop-flow kinetic studies on the native human isoenzymes B and C. J Biol Chem. 1971;246:2561–2573.
- Singh P, Yadav Swain PP, Thacker B, Angeli PS, Supuran A, Arifuddin CT. M. Discovery of a novel series of indolylchalcone-benzenesulfonamide hybrids acting as selective carbonic anhydrase II inhibitors. Bioorg Chem. 2021;108:104647.
- Darin D, Kantin G, Kalinin S, Sharonova T, Bunev A, Ostapenko GI, Nocentini A, Sharoyko V, Supuran CT, Krasavin M, et al. investigation of 3-sulfamoyl coumarins against cancer_related IX and XII isoforms of human carbonic anhydrase as well as cancer cells leads to the discovery of 2-oxo-2H-ben_zo[h]chromene-3-sulfonamide – a new caspase-activating pro apoptotic agent. Eur J Med Chem. 2021;222:113589.
- Yamali CI, Gul, H, Ozli G, Angeli A, Ballar Kirmizibayrak PE, Tepedelen B, Sakagami H, Bua S. Supuran CT.Exploring of tumor-associated carbonic anhydrase isoenzyme IX and XII inhibitory effects and cytotoxicities of the novel N-aryl-1-(4-sulfamoylphenyl)-5-(thiophen-2-yl)-1H-pyrazole-3-carboxamides. Bioorg Chem. 2021;115:105194.
- Lolak N, Akocak S, Bua S, Sanku RKK, Supuran CT. Discovery of new ureido benzenesulfonamides incorporating 1,3,5-triazine moieties as carbonic anhydrase I, II, IX and XII inhibitors. Bioorg Med Chem. 2019;27:1588–1594.
- Kim C-Y, Chang JS, Doyon JB, Baird TT, Fierke CA, Jain A, Christianson DW. Contribution of fluorine to protein-ligand affinity in the binding of fluoroaromatic inhibitors to carbonic anhydrase II. J Am Chem Soc. 2000;122:12125–12134.
- Maren TH, Conroy CW. A new class of carbonic anhydrase inhibitors. J Biol Chem. 1993;268(35):26233–26239.
- Abbate F, Casini A, Scozzafava A, Supuran CT. Carbonic anhydrase inhibitors: X-ray crystallographic structure of the adduct of human isozyme II with the perfluorobenzoyl analogue of methazolamide Implications for the drug design of fluorinated inhibitors. J Enz Inhib Med Chem. 2003;18:303–308.
- Pettersen EF, Goddard TD, Huang CC, Couch GS, Greenblatt DM, Meng EC, et al. UCSF Chimera–a visualization system for exploratory research and analysis. J Comput Chem. 2004;25:1605–1612.
- Nabi SA, Ramzan F, Lone MS, Beg MA, Hamid A, Nainwal LM, Samim M, Shafi S, Bano S, Javed K. Synthesis, crystallographic study, molecular docking, ADMET, DFT and biological evaluation of new series of aurone derivatives as anti-leishmanial agents. J Mol Struct. 2022;1256:132528.
- Choudhary S, Yadav J, Mamta Pawar AP, Vanaparthi S, Mir NA, et al. Sequential multicomponent site-selective synthesis of 4-iodo and 5-iodopyrrole-3-carboxaldehydes from a common set of starting materials by tuning the conditions. Org Biomol Chem. 2020;18:1155–1164.
- Hamid A, Roy RK. Solvent effect on stabilization energy: An approach based on density functional reactivity theory. Int J Quantum Chem. 2019;119:e25909.
- Parr RG, Pearson RG. Absolute hardness: companion parameter to absolute electronegativity. J Am Chem Soc. 1983;105:7512–7516.
- Hamid A, Roy RK. Validation of Hammett’s Linear Free Energy Relationship Through an Unconventional Approach. J Phys Chem A. 2020;124:5775–5783.
- Pearson RG. The principle of maximum hardness. Acc Chem Res. 1993;26:250–255.