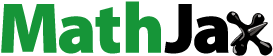
Abstract
A novel series of multifunctional pyrazolo[3,4-d]pyrimidine-based glutamate analogs (6a–l and 7a,b) have been designed and synthesized as antifolate anticancer agents. Among the tested compounds, 6i exhibited the most potent anti-proliferative activity towards NSCLC, CNS, Ovarian, Prostate, Colon, Melanoma, Breast, and Renal cancers with good to weak cytostatic activity and non-lethal actions. 6i demonstrated higher selectivity for cancer than normal cells. 6i could significantly increase the accumulation of S-phase cells during the cell cycle distribution of cancer cells with high potency in the induction of apoptosis. The results unveiled that 6i probably acts through dual inhibition of DHFR and TS enzymes (IC50 = 2.41 and 8.88 µM, correspondingly). Docking studies of 6i displayed that N1-p-bromophenyl and C3-Methyl groups participate in substantial hydrophobic interactions. The drug-likeness features inferred that 6i met the acceptance criteria of Pfizer. Taking together, 6i could be a promising prototype for further optimization as an effective anticancer drug.
Keywords:
Introduction
During the process of DNA synthesis, a significant number of endogenic forces can challenge, and cells harbour a series of pathways to disseminate genome integrityCitation1. Dihydrofolate reductase (DHFR) and thymidylate synthetase (TS) are key enzymes in nucleic acid synthesis, and they have long been evidenced as a crucial target of cancer chemotherapyCitation2,Citation3. DHFR reduces dihydrofolate (DHF) to tetrahydrofolate (THF) using NADPH, while TS catalyses the de novo synthesis of deoxythymidine monophosphate (dTMP) from deoxyuridine monophosphate (dUMP) using 5,10-Methylene THF as a cofactor, and thus initiates DNA synthesis and cell proliferationCitation2,Citation3. Therefore, DHFR and TS inhibitors cause THF and dTMP deficiency, resulting in RNA and DNA synthesis interference and apoptosisCitation4. However, the dose-limiting toxicities, low solubility, short plasma half-life, low specificity, rapid diffusion throughout the body, weak brain permeability unless at high doses (1–8 g/m2), low absorption and drug resistance development are the main drawbacks of the first-in-class DHFR and TS inhibitorsCitation3–5. A major mechanism of drug resistance by target cells to clinically useful classical antifolates () is based on their need for polyglutamylation via the enzyme folylpoly-gamma-glutamate synthetase (FPGS), which catalyses the addition of several equivalents of glutamic acid to the γ-carboxyl group in the side chain and hence prevents drug efflux from the cellCitation6. Therefore, developing a novel antifolate class of low toxicity and tumour resistance remains one of the major challenges that incessantly attracts intense interestCitation2,Citation3,Citation5,Citation7–10.
Figure 1. Structures of some antifolates and design strategy of novel Glu-based pyrazolo[3,4-d]pyrimidine analogues.
![Figure 1. Structures of some antifolates and design strategy of novel Glu-based pyrazolo[3,4-d]pyrimidine analogues.](/cms/asset/6fca3432-36a3-4e68-98cd-f11ad0d0066d/ienz_a_2203879_f0001_c.jpg)
The basic pharmacophoric features of classical antifolates such as methotrexate (MTX)Citation3, pralatrexate (PDX)Citation3, pemetrexed (PMX)Citation3, and raltitrexed (RTX)Citation3 could be divided into four regions as shown in : (1) the fused heterocyclic system (substituted pteridine, pyrrolo[2,3-d]pyrimidine and quinazoline scaffolds) which occupies the pteridine binding domain; (2) the heteroalkyl/alkyl chain (N-methyl aminomethyl, ethyl and sec-pentyne moieties) which occupies the bridge region and allows a tortuous “L” structure formation to fit tightly into the pocket of DHFR; (3) the benzoyl group which occupies the benzoyl region; and (4) the glutamate tail which occupies the l-glutamic acid binding siteCitation3. The SAR studies of classical antifolates usually vary in these regions.
The next generation of antifolates, as plevitrexed, revealed similar pharmacophoric properties of classical ones in which the free γ-carboxylic group was replaced with bioisosteric tetrazole moiety which, in turn, either improved the drug absorption or did not need to be polyglutamylated by FPGS and hence allows more decrease in drug resistance. In this context, the nonclassical antifolates such as nolatrexed and piritrexim (PTX) were designed as they are more lipophilic and non-polyglutamatable agentsCitation11,Citation12. Modifications in the antifolates structures have helped delineate the structural influence on the interaction with DHFR, TS, and FPGS utilisation. Therefore, intense interest has been in developing a new class of antifolate agents with these crucial structural pharmacophoric properties.
On the basis of isosterism and combination principles, as shown in , the 6-5 fused system, pyrazolo[3,4-d]pyrimidine, was selected as pteridine core isostere since it has recently been reported to display potent anti-tumour activities against different cancer cells with multitarget propertiesCitation13–16. The pyrazolo[3,4-d]pyrimidine core varied at N1, C3, and C6 and bridged at N5 with an acetamide linker which ended up with pharmacophoric benzoyl glutamate tailCitation3. The linker acetamide was used to improve binding affinity through the formation of a tortuous “L” structure to fit tightly inside the active site. In the glutamate region, both α and γ free carboxyl groups were blocked by making the corresponding ester in the form of a prodrug as a chemical approach catalysed by metabolic enzymes to minimise the undesirable physicochemical properties and enhance lipophilicity and obtain non-polyglutamatable agentsCitation4,Citation17. Moreover, the fused pyrimidine scaffold was replaced by a pyrimidine core to study the effect of a 6-5 fused system on biological activity. Therefore, we aim to develop novel hDHFR and TS dual inhibitors as a new generation of novel effective multitarget antifolate prodrugs with fewer side effects. Herein, a new lipophilic Glu-based pyrazolo[3,4-d]pyrimidine hybrid prodrug candidates were designed, prepared, and characterised by different spectroscopic techniques. The synthesised analogues were examined for their growth inhibitory (GI) effect on 59 cancer cell lines. The most active analogue was further subjected for its in vitro anti-proliferative activity against the most sensitive tumour and normal cell lines to investigate its selectivity towards cancer cells. In addition, cell cycle analysis and apoptosis were also examined. Finally, the in-silico molecular modelling and ADMET analysis were reported.
Results and discussions
Chemistry
The Glu-based pyrazolo[3,4-d]pyrimidine analogues 6a–l were prepared as cited in Scheme 1. The reaction cascade began with the cyclisation of alkylidene malononitrile 1a,b to 5-amino-4-cyano-pyrazole derivatives 2a–f using arylhydrazine derivativesCitation18,Citation19. The intermolecular cyclisation of 5-amino-4-cyano-pyrazole derivatives 2a–f using different aliphatic acids with a catalytic amount of phosphorous oxychloride afforded the precursors pyrazolo[3,4-d]pyrimidine-4-ones 3a–lCitation20. On the other hand, the glutamate chloroacetamide intermediate 5 was obtained from the chloroacetylation of glutamate anilinic derivative 4 with chloroacetyl chloride. Finally, the desired analogues 6a–n were synthesised through N5-alkylation of the precursor pyrazolo[3,4-d]pyrimidine-4-ones 3a–l with diethyl glutamate chloroacetamide intermediate 5.
Scheme 1. Synthesis of target compounds 6a–l. (i) R2-NHNH2, EtOH, reflux, 6 h; (ii) R3COOH, POCl3, reflux, 2h; (iii) ClCH2COCl, THF, reflux, 1 h; (iv) 5, DMF, K2CO3, NaI, 80 °C, 1–2 h.

Moreover, as shown in Scheme 2, carboxylic acid derivatives 7a and 7b were prepared through alkaline hydrolysis of the corresponding diethyl ester analogues 6c and 6d, respectively. On the other hand, the Biginelli reaction was utilised to synthesise the 1,4-dihydropyrimidine derivatives 9a–c through S-alkylation of 2-thioxo-1,4-dihydropyrimidine intermediates 8a–c with chloroacetamide glutamate derivative 5 as depicted in Scheme 3. Both spectral and elemental analysis data are depicted in the Supporting Information and have been fully consistent with the postulated structures of the prepared compounds.
Biological evaluation
Preliminary NCI-59 cancer cells single-dose screen
The growth percentage (G%) and cell viability of the herein target compounds 6a–l, 7a,b, and 9a–c have been screened towards a full panel of 59 cancer cells at NCI’s Developmental Therapeutics Program (NCI-DTP, MD, USA)Citation21. The submitted analogues were initially examined for preliminary in vitro anticancer activity using sulforhodamine B (SRB) assay at 10 µM as a single dose to evaluate cell growth and viability according to the procedure of Drug Evaluation BranchCitation22,Citation23.
Different types of tumours – colon, NSCLC, leukaemia, CNS, renal, breast, ovarian, melanoma, and prostate cancers – were investigated as a full panel of 59 cell lines, and the attained results are cited in Figures S1–S17 (see Supplementary Material) where the value recorded is the growth relative to that of both the negative control and zero-time number of cells in which both growth inhibition (GI%; 0–100%) and lethality (L%; 0–100%) percentages can be identified. The growth inhibition means percentages (MGI%) for the examined target analogues towards the different treated 59 cancer cells were presented in .
The attained results disclosed that compound 6k failed to demonstrate MGI%, while compounds 6a–h, 6j, 6 l, 7a,b, and 9a showed weak anticancer activity with MGI% ranging from 1.93% to 11.40%. Compounds 9b and 9c demonstrated moderate anticancer activity, with MGI% equal to 33.89% and 36.88%, respectively, while compound 6i exerted the significant mean growth inhibition percentage of 62.03% among the herein examined series. Superiorly, compound 6i emerged as the most efficient anticancer analogue eliciting high potency with broad-spectrum anticancer activity against all the herein subsets of cell panels, except against the A498 (Renal cancer) cell line ().
The inhibitory growth percentages (GI%) of compound 6i against the herein examined 59 cell panel are presented in . Surprisingly, exploring the results hinted that compound 6i exhibited excellent anti-proliferative activities towards Breast (MDA-MB-468 and MCF7), CNS (SF-295 and SF-268), NSCLC (NCI-H23), Ovarian (OVCAR-3 and OVCAR-8), Prostate (DU-145 and PC-3) and Renal (786-0) cancer cell lines with GI% of 92.44, 97.72, 89.78, 85.11, 87.19, 96.83, 87.86, 85.62, 91.71, and 82.93%, respectively. In addition, compound 6i displayed good inhibitory activity against Breast cancer (HS 578 T and BT-549), CNS cancer (SNB-19), Colon cancer (HCT-116, COLO 205, and SW-620), Leukaemia (CCRF-CEM), Melanoma (CCRF-CEM, LOX IMVI, SK-MEL-28, M14, and UACC-62), NSCLC (HOP-92, EKVX, and A549/ATCC) and Ovarian cancer (OVCAR-4) cell lines with GI% equals to 75.80, 66.64, 61.23, 70.04, 71.69, 62.17, 70.05, 79.78, 78.49, 75.54, 74.15, 63.82, 78.29, 78.12, 71.36, and 66.09, correspondingly. Furthermore, compound 6i demonstrated weak inhibitory activity against CNS cancer (SF-539 and U251), Colon cancer (HT29), Leukaemia (RPMI-8226 and MOLT-4), Melanoma (MDA-MB-435), NSCLC (NCI-H522), Ovarian cancer (NCI/ADR-RES) and Renal cancer (SN12C) cell lines with GI% of 54.23, 53.30, 59.90, 53.52, 52.51, 57.10, 52.34, 51.78, and 57.69, respectively. Moreover, the growth inhibitory percentages of 6i towards the other cell lines ranged from 9.83 to 48.82%. It is worth underlining that the lethality (L) of compound 6i was detected against some cell lines such as NSCLC (NCI-H226 and HOP-62), Renal (RXF 393), and Melanoma (SK-MEL-5) cancer cell lines with L% of 37.32, 8.41, 22.73, and 1.38%, respectively. Therefore, compound 6i exerted a remarkable anticancer profile in the One-Dose assay towards the herein tumour screening panel.
NCI 59 cancer cell five-dose screen
Remarkably, compound 6i (NSC: 838397) exhibited significant growth inhibition properties in the One-Dose (10 µM) screening assay; hence it was chosen for further evaluation at five-dose levels (0.01–100 µM) towards the herein examined tumour cell panelsCitation21 (Figures S53–S56 in Supporting Information). This assay evaluates GI50, TGI, and LC50 parameters, as cited in . Their values correspondingly represent the GI level, cytostatic impact, and cytotoxicity parameter. Furthermore, based on the GI50 values, the mean graph midpoints (MG-MID) for both full NCI 59 cell panel and subpanel cancer cell lines were evaluated, affording a median potency parameter for the examined compound 6i, as depicted in .
Table 1. NCI-59 cancer cell five-dose screening results of compound 6i (NSC: 838397).
Table 2. MG-MID values and selectivity index for compound 6i towards the herein examined subpanels.
Interestingly, the target compound 6i displayed potent anticancer activity at a single-digit micromolar concentration towards NSCLC (HOP-62, A549/ATCC, HOP-92, NCI-H23, and NCI-H226), CNS cancer (SF-268), Colon cancer (HCT-116), Ovarian cancer (OVCAR-8), Melanoma (LOX IMVI), Prostate cancer (DU-145 and PC-3) Renal cancer (786-0), and Breast cancer (BT-549 and MCF7) cells with GI50 spanning in the range: 3.93–9.85 µM as shown in . In addition, compound 6i showed good to weak anti-proliferative action against the residual herein examined cell lines with GI50 spanning in the interval from 10.10 to 71.00 µM, except for Leukaemia (HL-60(TB), K-562, MOLT-4, and CCRF-CEM) and Melanoma (M14) cancer cell lines which had GI50 more than 100 µM. While compound 6i revealed good to weak cytostatic action towards 41 of the herein examined tumour cell lines with TGI spanning in the range: 16.50–89.10 µM, it had no impact (TGI more than 100 µM) towards Leukaemia (HL-60(TB), CCRF-CEM, MOLT-4, K-562, SR, and RPMI-8226), Colon (HCC-2998, HCT-15, HCT-116, and KM12), CNS (U250), Melanoma (M14 and UACC-257), Ovarian (OVCAR-8), Renal (SN12C and 786-0), and Breast (T-47D and BT-549) cancer cells ().
Furthermore, the target analogue 6i was found to be a non-lethal compound (LC50 > 100 µM) towards most of the herein examined cancer cells, except for NSCLC (EKVX, HOP-62, HOP-92, NCI-H460, NCI-H522, and NCI-H23), Colon (COLO 205), CNS (SF-539, SNB-19, and SF-295), Melanoma (MALME-3M, LOX IMVI, SK-MEL-28, MDA-MB-435, UACC-62, and SK-MEL-5), Renal (ACHN, CAKI-1, and A498), Ovarian (OVCAR-4), Breast (MDA-MB-231/ATCC and MCF7) and Prostate (PC-3) cancer cell lines with LC50 interval: 47.0–96.1 µM ().
On the other hand, compound 6i had relatively homologous GI activity towards the cancer screening panel exploring the diverse cancer cells sensitivity, with efficient individual subpanel MG-MID values spanning from 6.63 to 68.03 µM and promising full panel MG-MID equals 20.45 µM. Interestingly, the most vulnerable subpanels to the impact of compound 6i were Non-Small Cell Lung and Prostate cancers with MG-MID equal 10.62 and 6.63 µM, correspondingly, .
Moreover, the selectivity index (SI) of the most efficient analogue 6i was calculated from the division of full-panel MG-MID by subpanel MG-MID. A value greater than 6 implies high selectivity, whereas a ratio from 3 to 6 denotes moderate selectivity. Otherwise, the compound is referred to as non-selective.Citation24 Hence, the examined compound 6i has emerged as a potent non-selective broad-spectrum anti-proliferative agent against all the herein examined subpanels with SI spanning in the interval: 0.3–1.9, except for Prostate cancer cell lines which stood out as a moderately selective agent with SI of 3.08, as depicted in . Therefore, the outstanding anti-cancer profile of compound 6i against the herein examined NCI-59 cell panels inspired us to explore its mechanism of action further.
Preliminary SAR study of the designed compounds
As the tested compounds demonstrated, variable anti-proliferative potencies towards cancer cell lines and structure activity relationships (SAR) about these compounds could be summarised as follows. Firstly, the introduction of the methyl group at C6 of 6a,c,e,g,i and 7a (afforded 6b,d,f,h,j and 7b) would decrease their anti-proliferative effects while in compound 6k (afforded 6 l), the inhibitory activity was increased by 8.18%. Secondly, the inhibitory activity of 6a,b, 6i, and 6l indicated that introducing substitution to the C3, especially methyl group, could significantly increase the inhibitory effects against the cancer cell lines. Thirdly, increasing lipophilicity at N1 by installing a p-bromophenyl substituent would significantly increase the inhibitory activity as in 6i. Fourthly, the inhibitory activity of 7a and 7b showed that hydrolysis of carboxylate ester of benzoyl glutamate tail of 6c and 6d would not improve the inhibitory effects. In turn, they could moderately decrease the inhibitory activities against most cancer cell lines evidencing that the ester prodrug form is more potent. Fifthly, replacing the pteridine isosteric 6-5 fused system, pyrazolo[3,4-d]pyrimidine, with 1,4-dihydropyrimidine core would significantly increase the inhibitory effect in compounds 9b and 9c. Also, the inhibitory effect of 9a, 9b, and 9c indicated that installing p-bromophenyl substituent at C4 in a pyrimidine ring could significantly increase the inhibitory effects against most of the cancer cells as in 6i, demonstrating the importance of this moiety on the anti-proliferative activity. Finally, 6i established better anti-proliferative effects among the tested analogues against NCI-59 cancer cells. In brief, we could conclude that bridging of pyrazolo[3,4-d]pyrimidine core at C5 with diethyl glutamate tail through acetamide linker and installation of a more lipophilic group at N1 and a hydrophobic group at C3 along with no substitution at C6 could potentially impact the anti-proliferative activity ().
Anti-proliferative activity towards human cancer MCF-7, PC-3, and OVCAR-3 cell lines
The future growth-inhibiting effects of the most active derivative 6i were investigated towards PC-3 (Prostate cancer), MCF-7 (Breast cancer), OVCAR-3 (Ovarian Cancer), as well as HSF (Normal human skin fibroblast) cells, using MTT assayCitation25–31. The cell viability was measured after incubation with the test compound for 48 h, and MTX was co-assayed as a reference antitumor drug. As shown in , the investigation hinted that compound 6i exhibited potent growth inhibitory activity, with IC50 values of 4.40 ± 0.26, 12.42 ± 0.62, and 11.52 ± 0.58 µM against MCF-7, PC-3, and OVCAR-3, correspondingly. In addition, compound 6i had about seven-fold, four-fold, and two-fold dwindled efficiency compared to MTX towards MCF-7, PC-3, and OVCAR-3 cancer cells, respectively. Furthermore, the cytotoxicity of 6a and MTX in normal fibroblast cells (HSF) was also studied, and the selectivity index (SI) was calculated. The results hinted that 6i elicited stronger cytotoxicity and higher selectivity for cancer than normal cells.
DHFR and TS inhibitory activity
To identify the effect on the targeted enzymes, hDHFR and TS inhibition assay was performed on the most potent anticancer analogue 6i. The assay was performed as reported elsewhereCitation32–35 using MTX and 5-FU as reference drugs, respectively. As shown in , the DHFR inhibition results established that 6i showed inhibitory potency of an IC50 of 2.41 µM compared to MTX (IC50 = 0.11 µM). In contrast, the TS inhibition activity was comparable to that of 5-FU (IC50 8.88 and 3.60 μM, respectively).
Cell cycle analysis in MCF-7 cells
The cell cycle distribution modulation and apoptosis induction were investigated to verify the mechanism of action of the most potent compound, 6i, in MCF-7 cells which were treated with 6i at 10 µM for 48 h along with an untreated DMSO control, washed with PBS, then fixed with 70% ice-cold ethanol for 12 h followed by staining with propidium iodide (PI), and analysed by flow cytometryCitation36,Citation37. The results showed that the percentage of S-phase cells increased to 36.29% for 6i from 24.95% (DMSO control) (. These results indicated that 6i could effectively induce S-phase arrest.
Figure 5. Flow cytometric analyses: cell cycle distribution analysis using PI staining method in which MCF-7 cells were treated by 6i (10 µM) and DMSO (negative control; NC) for 48 h; (A) 6i, (B) DMSO, (C) cells percentage in different phases (G0-G1, S, G2/M); apoptotic induction using annexin-V-FITC/PI staining method in which cancer cells were treated by 6i (10 µM) and DMSO (negative control; NC) for 24 h; (D) 6i, (E) DMSO, (F) histogram for induction of apoptosis.

Analysis of apoptosis
Again, MCF-7 cells were treated with 6i (10 µM) to assess its effect on cell apoptosis. As shown in , the fraction of cells in early apoptosis were 26.27% and 0.43%, while in late apoptosis, they were 8.90% and 0.29% for compound 6i and DMSO control, respectively. The obtained results hinted that compound 6i could induce apoptotic pathways. However, the ratio of apoptosis inferred that the anti-proliferative effect of compound 6i was largely dependent on apoptosis.
In silico molecular modelling studies
Molecular modelling analyses were accomplished by OpenEye® Scientific software (ver. 2021.2.1) to investigate the docking mode of the most active analogue 6i inside the active site of both hDHFR (PDB ID: 1U72) and TS (PDB ID: 1JU6)Citation19,Citation38–43. The reference drugs, MTX and PMX, were re-docked using the same procedure as compound 6i to validate the docking results and rationalise the predictive protein-ligand interactions within the active siteCitation18,Citation44–46. As shown in Figures S36 and S37 (in Supplementary Material), the docking pose of the co-crystallised ligand showing interactions similar to those existing in the crystal structure, and the hydrophobic interactions were roughly the same.
As seen in , the docked pose of compound 6i (grey) showed an overlay with the cocrystallised ligand MTX (green) in the hDHFR active siteCitation47. The results display that the pyrazolo[3,4-d]pyrimidine core of 6i displayed only hydrophobic interactions: alkyl, pi-pi T-shaped, and pi-alkyl. In contrast, there are three hydrogen bonds for MTX in the corresponding binding site. The NH of the acetamide bridge showed a hydrogen bond with Asp21, while the benzoyl moiety demonstrated pi-alkyl hydrophobic interactions with Pro61:A and Pro26:A residues. The benzoylglutamate tail of 6i formed two hydrogen bonds from the γ-carboxylate group with Asn64:A and Lys63:A residues, which differ from MTX′s. Also, compound 6i showed a FRED chemgauss4 score of −5.06, while MTX had a value of −13.38. The overlay of the docked pose of 6i with the crystal structure of MTX indicated that 6i established, to some extent, a similar binding mode with that of MTX, especially the formation of tortuous “L” structure, which allows tightly fitting in the active site of DHFR. This offered a plausible rationale for why 6i showed the best anti-cancer profile among the series tested, as established by NCI screening and molecular mechanisms. Furthermore, the molecular docking results infer that the hydrophobic interactions or hydrogen bonds of 6i were weaker than MTX′s. Consequently, 6i could be an inhibitor of DHFR but should be less potent than MTX. Overall, the Fred docking results proposed modifications or extensions on the N7 and benzoyl moiety as they projected towards the protein cavity.
Figure 6. Binding modes to the active sites of DHFR and TS enzymes: (A) 3D representation of 6i displaying an overlay with the crystal structure of MTX in the active site of DHFR; (B) 3D representation of 6i demonstrating an overlay with the crystal structure of PMX in the active site of TS; (C) 2D diagram of 6i in DHFR active site; (D) 2D diagram of 6i in TS active site.

also reveals an overlay of the docked pose of compound 6i (grey) with the crystal structure of PMX (green) in the human TS active siteCitation48. Like that of human DHFR, the TS active site consists of four regions: the pteridine, the bridge, the benzoyl, and the glutamate regions. The results display that the pyrazolo[3,4-d]pyrimidine core forms a hydrogen bond between bromine and Arg50:A and hydrophobic interactions: pi-pi T-shaped, alkyl, and pi-alkyl. The acetamide linker allows an overlay with PMX crystal structure through tortuous “L” structure formation. The benzoyl moiety formed mixed pi-alkyl hydrophobic interactions with Leu221:A. Finally, the glutamate tail of 6i forms van der Waals attractions in the benzoylglutamate region. The binding pose of 6i indicates that the pyrazolo[3,4-d]pyrimidine motif and benzoylglutamate tail form a tortuous “L” structure through the acetamide bridge region and spatially stacked well with the docked pose of PMX. Furthermore, the modelling study implies that 6i could be an inhibitor of TS but should be less potent than PMX, which is aligned with the results of inhibitory enzyme assays. Overall, the Fred docking results proposed modifications or extensions on the N1, N7, benzoyl moiety, and γ-carboxylic ester group as they project towards the protein cavity.
Drug-likeness and ADMET prediction
Drug-likeness and pharmacokinetics ADMET properties for the most active compound 6i were calculated using ADMETlab 2.0 predictorCitation49,Citation50. As illustrated in , Only one violation from Lipinski’s rule of five is permitted for a compound to obey the rule. It can be observed that compound 6i revealed two violations from Lipinski’s rule while Pfizer accepts 6i criteria as a good prodrug candidate. The ADMET prediction data of compound 6i are cited in . The results revealed that 6i has a good synthetic accessibility score (SA score 3.14), a high GIT absorption rate since the human intestinal absorption (HIA) is 90% or more, and moderate solubility in water that helps in solubilisation in GIT fluids before absorption process as indicated by the estimated log S value (−5.07). In addition, the tested compound 6i demonstrated more than 20% bioavailability, as indicated by the F20% result, while the plasma protein binding was 91.72%. Also, it is expected to be a non-inhibitor of CYP1A2, CYP2C19, and CYP2D6 enzymes, suggesting a low probability of causing drug-drug interaction. Furthermore, carcinogenicity and acute toxicity tests showed that compound 6i is neither carcinogenic nor toxic, evidencing the good ADMET profile.
Table 3. Growth inhibitory activity of compound 6i against MCF-7, PC-3, OVCAR-3, and HSF cells.
Table 4. DHFR and TS inhibitory activity of compound 6i and reference drugs.
Table 5. Lipinski’s rule of five calculated parameters for the most active anti-cancer compound 6i.
Table 6. Predicted ADMET properties for the most active anti-cancer compound 6i.
Experimental part
Chemistry
Bruker Avance III 400 MHz HD FT-high resolution-NMR spectrometer was used for recording the spectra of 1H and 13C NMR in deuterated solvents DMSO-d6 and CDCl3 using the residual peaks at δH = 2.50 ppm and δH = 7.27 ppm as an internal standard for 1H NMR spectra. In contrast, δC = 39.51 ppm and δC = 77.23 ppm were used for 13C NMR spectra, respectively. The multiplicity of signals was verified as singlet (s), doublet (d), doublet of doublets (dd), triplet (t), quartette (q), multiplet (m), or broad (br). The J-coupling constants (in Hz) were recorded. For reaction monitoring, silica gel 60 F254 pre-coated plates (Merck) were used for TLC using UV light (254 nm) for visualisation. The purification processes of the prepared analogues were accomplished by either recrystallisation from an appropriate solvent or chromatographic separation utilising silica gel for column (150–250 μm). All melting points were determined on an electro thermal melting point apparatus (Stuart Scientific, Model SMP1, UK) and were uncorrected. Mass spectrum was carried out on Direct Inlet part to mass analyser in Thermo Scientific GC-MS model ISQ (Waltham, MA, USA). Vario elemental analyser III (Vario, Germany) was used for analysing C, H, and N for all designed analogues. The findings agreed with the proposed structures within ±0.4% of the theoretical values. Dry solvents and chemicals were purchased from commercial suppliers and used as received. Compounds 2a–fCitation18,Citation19,Citation51–53, 3a–eCitation18,Citation19,Citation51,Citation54–58, 3g–lCitation18,Citation19,Citation51,Citation54–58, 5Citation59 and 8a–cCitation60–64 were synthesised as described.
General procedure for synthesis of compounds 3a–l
To a suspension of 1-aryl-5-amino-4-cyanopyrazoles 2a–f (0.006 mol) in aliphatic acid (18 ml), POCl3 (1.2 ml) was added instantaneously. The mixture was refluxed for 2 hCitation20 and then cooled to 0 °C, poured slowly to crushed ice, filtered, and recrystallised from formic acid (85%) to provide the intermediates 3a–l in good yields.
General procedure for the synthesis of N5 appended analogues 6a–l
To a solution of pyrazolo[3,4-d]pyrimidinone derivatives 3a–l (0.001 mol) in DMF (2 ml), diethyl (4-(2-chloroacetamido)benzoyl)glutamate 5 (0.3988 g, 0.001 mol), anhydrous K2CO3 (0.1382 g, 0.001 mol), and sodium iodide (0.015 g, 0.0005 mol) were added. The reaction was heated at 65° for 1–2 h as monitored by TLCCitation65. The reaction mixture was cooled to 25 °C and then poured dropwise onto crushed ice containing brine (5 ml). Finally, the formed product was filtered and crystallised from the appropriate solvent to provide the N5-derived analogues 6a–l.
General procedure for the synthesis of acid derivatives (7a,b)
A suspension of 6c,d (0.002 mol) in 5 ml aqueous sodium hydroxide (1 mol/l) was stirred at 25 °C for 2 h (TLC monitoring)Citation3. The reaction mixture was then filtered and acidified carefully with 0.5 mol/l HCl. The formed product was filtered and crystallised from the appropriate solvent to give 7a,b.
General procedure for preparation of 1,4-dihydropyrimidine derivatives (9a–c)
To a stirred solution of 2-thioxo-1,4-dihydropyrimidine intermediates 8a–c (0.001 mol) in DMF (2 ml), diethyl (4-(2-chloroacetamido)benzoyl)glutamate 5 (0.3988 g, 0.001 mol), anhydrous potassium carbonate (0.138 g, 0.001 mol), and sodium iodide (0.015 g, 0.0005 mol) were added. The reaction mixture was stirred at room temperature for 8 h, as indicated by TLCCitation65. The reaction mixture was then poured dropwise onto crushed ice containing 5 ml of brine. The formed precipitate was filtered and crystallised from the appropriate solvent to yield the desired analogues 9a–c.
The key intermediate (3f) and target compounds (6a–l, 7a,b, and 9a–c) were fully characterised in the Supplementary Material.
Biological activity
NCI-60 screening methodology
The designed analogues were submitted to NCI-Chemotherapeutic Agents Repository (MD, USA) for screening on a panel of 59 cancer cell lines. All compounds were initially examined at 10 µM concentration (Single-Dose Screen), and then the most efficient analogue 6i was screened further at 0.01–100 µM (Five-Dose Screen). The screening procedures were performed according to NCI-Drug Evaluation Branch protocol using SRB protein assay, as described previouslyCitation21,Citation66. The experimental procedures were detailed in the Supporting Information.
MTT cytotoxicity assay
The MTT assay was carried out according to the procedure cited earlierCitation25–31. Briefly, in a 96-well plate, either cancer or normal cells were seeded (106 cells/cm2; 100 µL/well) and incubated for 12 h at 37 °C and CO2 (5%), then serial dilutions of compound 6i were added and incubated for 48 h using DMSO control (0.5% V/V). MTT (3-(4,5-dimethylthiazoyl)-2,5-diphenyl-tetrazolium bromide; Cat. No. M-5655) 5 mg/mL was then added. After 4 h incubation, the formed MTT formazan crystals were solubilised by 10% Triton X-100 plus 0.1 N HCl in anhydrous isopropanol. The absorbance was read using a Bioline plate reader at λ570–630 nmCitation25–29. The selectivity of 6i towards HSF cells was also determined as followsCitation30,Citation31:
Cell cycle analysis
The cell cycle assay was carried out according to the previously reportedCitation36,Citation37. Briefly, in a six-well plate, 2 ml of MCF-7 cells were seeded per well and incubated overnight at 37 °C and CO2 (5%). After 12 h incubation with serum-free medium, they were treated with 10 μM of 6i, incubated for 48 h, then washed twice with 1× PBS, trypsinised and centrifuged for 5 min (500 xg) at 4 °C. And then the cell pellet, in ice-cold 1× PBS, was fixed with absolute ethanol at −20 °C for 2 h, washed with 1× PBS ice-cold solution and finally stained with propidium iodide (PI)/RNase (Abcam, ab139418) for 0.5 h at 25 °C in the dark. flow cytometry determined the DNA content in cell phases using BD FACS CaliburCitation36,Citation37.
Apoptosis analysis
The MCF-7 cells (106 cells/ml) were treated with either 10 µM of 6i or DMSO (NC) for 24 h. After that, the cells were trypsinised, collected, washed, and stained with PE-Annexin-V (BioVision, K101-25) in the presence of PICitation67. The induction of apoptosis was analysed by flow cytometry using BD FACS CaliburCitation67.
DHFR inhibitory assay
DHFR inhibition assay kit (BioVision, K247–100) supplied with recombinant hDHFR was utilised in this assay. The assay was performed as instructed in assay kitCitation32. Briefly, in a 96-well clear plate, serial dilutions of the test compound (2 μL) were loaded. DHFR was diluted in assay buffer (400-fold), and 98 μL was added to the assigned wells for compound 6i and enzyme control. An assay buffer (100 µl) was used as a Background Control. Then, 40 μL of diluted NADPH in assay buffer (40-fold dilution) was added and mixed well. At 25 °C in the dark, the plate was incubated for 15 min. After that, the diluted substrate in assay buffer at 15-fold dilution (60 μL) was added to assigned wells. Instantaneously, the absorbance was read at 340 nm for 10–20 min at 25 °C in kinetic mode by Robonik ELISA plate reader. Finally, the activity and relative inhibition percentages were measured using the equations indicated in the assay kitCitation33–35.
TS inhibitory assay
The assay was performed as instructed in the assay kit (ProFoldin, TMK100KE, MA, USA). Briefly, in a standard black well plate (Matrix 4318), water (12 µl), 10 × Buffer (3 µl), 10 × dTMP (3 µl), 10 × ATP (3 µl) and 10 × kinase (3 µl) were mixed well. After 2 min, 10 × MUK Reagent A (3 µl) and 10 × MUK Reagent B (3 µl). The mixture was then incubated at 37 °C for 1 h, and 1 × fluorescence dye (30 µl) was added. Finally, the fluorescence intensity was read at 535 nm with excitation at 485 nm utilising a Robonik ELISA plate reader.
Drug-likeness and ADMET analyses
Drug-likeness, physicochemical and pharmacokinetic characters of the most potent analogue 6i were predicted using an accurate, comprehensive, and efficient web-based tool ADMETlab 2.0. They were utilising a high-quality database and a framework for multi-task graph attentionCitation49,Citation50.
Molecular modelling studies
The straightforward OpenEye® scientific software (2021.2.1) was used for molecular docking studiesCitation19,Citation38–42. A library of the most potent compound 6i, MTX, and PMX was built, and MMFF94 force field was used for energy minimisation then generation of multi-conformers was offered by OMEGA® moduleCitation68. Both hDHFR (PDB ID 1U72) and TS (PDB ID 1JU6) receptors were prepared by the Spruce® application, and then FRED® module was utilised for molecular modelling, and hence FRED Chemgauss4 score generationCitation69. The Vida® module was used to visualise the tested compounds′ poses to both DHFR and TS active sitesCitation70. Also, a 2D diagram of binding interactions was generated by the Discovery Studio Visualisation tool (DS)Citation71,Citation72. The Docking-Report module generated the docking report through the command lines stated in OpenEye documentationCitation73.
Conclusion
In this research, a novel series of multifunctional pyrazolo[3,4-d]pyrimidine and 1,4-dihydropyrimidine Glu-based derivatives 6a–l, 7a,b, and 9a–c were designed and synthesised as potential antifolate agents. The NCI-59 Screening assay displayed that compounds 6i and 9a–c showed the best MGI% among the series investigated. At the NCI five-dose assay, compound 6i exhibited potent anti-proliferative activity against the herein tumour screening panel with GI50 spanning in the range: 3.93–9.85 µM. Compound 6i revealed good to weak cytostatic activity with TGI spanning in the range: 16.50–89.10 µM, and it was found to be a non-lethal compound (LC50 > 100 µM) towards most of the herein examined cancer cell lines. Moreover, compound 6i elicited broad-spectrum anticancer activity with effective subpanel MG-MID interval: 6.63–68.03 µM and promising full panel MG-MID of 20.45 µM with moderate selectivity towards Prostate cancer cell lines. The target analogue 6i, as the most potent derivative, demonstrated high selectivity towards cancer cell lines with IC50 values equal to 4.40, 12.42, and 11.50 against MCF-7, PC-3, and OVCAR-3 cells, respectively. Furthermore, compound 6i showed potent hDHFR inhibitory activity with an IC50 equal to 2.41 µM compared to MTX (IC50 = 0.11 µM), while the inhibitory activity towards TS was 8.88 µM compared to 5-FU (IC50 = 3.6 µM). The target compound 6i probably acts through dual inhibition of DHFR and TS enzymes. Compound 6i could significantly arrest the cells at the S-phase of the cell cycle and potentially induce apoptosis. The in silico molecular docking studies implied that compound 6i had an overlay with the crystal structure of MTX and PMX in their active sites of DHFR and TS, respectively, forming a tortuous “L” structure through the acetamide bridge region. The drug-likeness and ADMET analysis revealed that 6i met the Pfizer acceptance criteria. These results thoroughly delineate 6i as a good lead compound and multi-targeted antifolate agent for further study.
Supplemental Material
Download PDF (8 MB)Acknowledgements
The authors would like to express their gratitude and thanks to the Deanship of Scientific Research at Najran University for Funding this work under the National Research Priority program grant code (NU/DRP/MRC/12/1). The authors extend their appreciation to OpenEye Scientific Software for providing the academic licence.
Disclosure statement
The authors report no conflicts of interest.
Additional information
Funding
References
- Boyer AS, Walter D, Sørensen CS. DNA replication and cancer: From dysfunctional replication origin activities to therapeutic opportunities. Semin Cancer Biol. 2016;37-38:16–25.
- Raimondi MV, Randazzo O, La Franca M, Barone G, Vignoni E, Rossi D, Collina S. DHFR inhibitors: reading the past for discovering novel anticancer agents. Molecules. 2019;24(6):1140–1159.
- Tian C, Wang M, Han Z, Fang F, Zhang Z, Wang X, Liu J. Design, synthesis and biological evaluation of novel 6-substituted pyrrolo [3,2-d] pyrimidine analogues as antifolate antitumor agents. Eur J Med Chem. 2017;138:630–643.
- Fattahi N, Ramazani A, Hamidi M, Parsa M, Rostamizadeh K, Rashidzadeh H. Enhancement of the brain delivery of methotrexate with administration of mid-chain ester prodrugs: in vitro and in vivo studies. Int J Pharm. 2021;600:120479–120488.
- Gangjee A, Jain DH, Kurup S. Recent advances in classical and non-classical antifolates as antitumor and antiopportunistic infection agents: part I. Anticancer Agents Med Chem. 2007;7(5):524–542.
- Moran RG. Roles of folylpoly-gamma-glutamate synthetase in therapeutics with tetrahydrofolate antimetabolites: an overview. Semin Oncol. 1999;26(2 Suppl 6):24–32.
- Ewida MA, Abou El Ella DA, Lasheen DS, Ewida HA, El-Gazzar YI, El-Subbagh HI. Thiazolo[4,5-d]pyridazine analogues as a new class of dihydrofolate reductase (DHFR) inhibitors: synthesis, biological evaluation and molecular modeling study. Bioorg Chem. 2017;74:228–237.
- García DS, Saturansky EI, Poncino D, Martínez-Artola Y, Rosenberg S, Abritta G, Ascimani-Peña C, Cravero A. Hepatic toxicity by methotrexate with weekly single doses associated with folic acid in rheumatoid and psoriatic arthritis. What is its real frequency? Ann Hepatol. 2019;18(5):765–769.
- Mao Z, Pan J, Kalman TI. Design and synthesis of histidine analogues of folic acid and methotrexate as potential folylpolyglutamate synthetase inhibitors. J Med Chem. 1996;39(21):4340–4344.
- Patel TS, Bhatt JD, Dixit RB, Chudasama CJ, Patel BD, Dixit BC. Design and synthesis of leucine‐linked quinazoline‐4 (3H)‐one‐sulphonamide molecules distorting malarial reductase activity in the folate pathway. Arch Pharm Chem Life Sci. 2019;352(9):1900099.
- Marsham PR, Wardleworth JM, Boyle FT, Hennequin LF, Kimbell R, Brown M, Jackman AL. Design and synthesis of potent non-polyglutamatable quinazoline antifolate thymidylate synthase inhibitors. J Med Chem. 1999;42(19):3809–3820.
- Bavetsias V, Jackman AL. Nonpolyglutamatable antifolates as inhibitors of thymidylate synthase (TS) and potential antitumour agents. CMC. 1998;5(4):265–288.
- Wang S, Yuan X-H, Wang S-Q, Zhao W, Chen X-B, Yu B. FDA-approved pyrimidine-fused bicyclic heterocycles for cancer therapy: synthesis and clinical application. Eur J Med Chem. 2021;214:113218–113239.
- Ayati A, Moghimi S, Toolabi M, Foroumadi A. Pyrimidine-based EGFR TK inhibitors in targeted cancer therapy. Eur J Med Chem. 2021;221:113523–113542.
- Alberti MJ, Auten EP, Lackey KE, McDonald OB, Wood ER, Preugschat F, Cutler GJ, Kane-Carson L, Liu W, Jung DK. Discovery and in vitro evaluation of potent kinase inhibitors: pyrido[1′,2′:1,5]pyrazolo[3,4-d]pyrimidines. Bioorg Med Chem Lett. 2005;15(16):3778–3781.
- Abdelgawad MA, Bakr RB, Alkhoja OA, Mohamed WR. Design, synthesis and antitumor activity of novel pyrazolo[3,4-d]pyrimidine derivatives as EGFR-TK inhibitors. Bioorg Chem. 2016;66:88–96.
- Karaman R. Prodrugs design based on inter- and intramolecular chemical processes. Chem Biol Drug Des. 2013;82(6):643–668.
- Mahnashi MH, El-Senduny FF, Alshahrani MA, Abou-Salim MA. Design, synthesis, and biological evaluation of a novel VEGFR-2 inhibitor based on a 1,2,5-oxadiazole-2-oxide scaffold with MAPK signaling pathway inhibition. Pharmaceuticals. 2022;15(2):246–281.
- Elshaier YAMM, Shaaban MA, Abd El Hamid MK, Abdelrahman MH, Abou-Salim MA, Elgazwi SM, Halaweish F. Design and synthesis of pyrazolo[3,4-d]pyrimidines: nitric oxide releasing compounds targeting hepatocellular carcinoma. Bioorg Med Chem. 2017;25(12):2956–2970.
- Zhang X, Lin Q, Zhong P. A facile one-pot synthesis of 1-arylpyrazolo [3, 4-d] pyrimidin-4-ones. Molecules. 2010;15(5):3079–3086.
- National Cancer Institute. NCI-60 Screening Methodology. [accessed 2022 Jun 1]. https://dtp.cancer.gov/discovery_development/nci-60/methodology.htm.
- Monks A, Scudiero D, Skehan P, Shoemaker R, Paull K, Vistica D, Hose C, Langley J, Cronise P, Vaigro-Wolff A. Feasibility of a high-flux anticancer drug screen using a diverse panel of cultured human tumor cell lines. J Natl Cancer Inst. 1991;83(11):757–766.
- Boyd MR, Paull KD. Some practical considerations and applications of the National Cancer Institute in vitro anticancer drug discovery screen. Drug Dev Res. 1995;34(2):91–109.
- Al-Warhi T, Abo-Ashour MF, Almahli H, Alotaibi OJ, Al-Sanea MM, Al-Ansary GH, Ahmed HY, Elaasser MM, Eldehna WM, Abdel-Aziz HA. Novel [(N-alkyl-3-indolylmethylene)hydrazono]oxindoles arrest cell cycle and induce cell apoptosis by inhibiting CDK2 and Bcl-2: synthesis, biological evaluation and in silico studies. J Enzyme Inhib Med Chem. 2020;35(1):1300–1309.
- Skehan P, Storeng R, Scudiero D, Monks A, McMahon J, Vistica D, Warren JT, Bokesch H, Kenney S, Boyd MR. New colorimetric cytotoxicity assay for anticancer-drug screening. J Natl Cancer Inst. 1990;82(13):1107–1112.
- Plumb JA. Cell sensitivity assays: The MTT assay. In: Langdon SP, editor. Cancer cell culture: Methods and Protocols. Totowa, NJ: Humana Press Inc., Springer; 2004. p. 165–169.
- Bézivin C, Tomasi S, Lohézic-Le Dévéhat F, Boustie JJP. Cytotoxic activity of some lichen extracts on murine and human cancer cell lines. Phytomedicine. 2003;10(6-7):499–503.
- Prayong P, Barusrux S, Weerapreeyakul N. Cytotoxic activity screening of some indigenous Thai plants. Fitoterapia. 2008;79(7-8):598–601.
- El-Senduny FF, Zidane MM, Youssef MM, Badria F-C. An approach to treatment of liver cancer by novel glycyrrhizin derivative. Anticancer Agents Med Chem. 2019;19(15):1863–1873.
- Singh K, Gangrade A, Jana A, Mandal BB, Das N. Design, synthesis, characterization, and antiproliferative activity of organoplatinum compounds bearing a 1,2,3-triazole ring. ACS Omega. 2019;4(1):835–841.
- Bartmańska A, Tronina T, Popłoński J, Milczarek M, Filip-Psurska B, Wietrzyk J. Highly cancer selective antiproliferative activity of natural prenylated flavonoids. Molecules. 2018;23(11):2922–2936.
- Azzam RA, Elsayed RE, Elgemeie GH. Design, synthesis, and antimicrobial evaluation of a new series of N-sulfonamide 2-pyridones as dual inhibitors of DHPS and DHFR enzymes. ACS Omega. 2020;5(18):10401–10414.
- el-Subbagh HI, Abadi AH, Al-Khamees HA. Synthesis and antitumor activity of 9-anilino, phenylhydrazino, and sulphonamido analogs of 2- or 4-methoxy-6-nitroacridines. Arch Pharm. 1997;330(9-10):277–284.
- Bilal Tufail M, Aamir Javed M, Ikram M, Mahnashi MH, Alyami BA, Alqahtani YS, Sadiq A, Rashid U. Synthesis, pharmacological evaluation and molecular modelling studies of pregnenolone derivatives as inhibitors of human dihydrofolate reductase. Steroids. 2021;168:108801–108811.
- Sabry MA, Ghaly MA, Maarouf AR, El-Subbagh HI. New thiazole-based derivatives as EGFR/HER2 and DHFR inhibitors: synthesis, molecular modeling simulations and anticancer activity. Eur J Med Chem. 2022;241:114661–114682.
- Henriksen M, Miller B, Newmark J, Al-Kofahi Y, Holden E, Chapter 7. Laser scanning cytometry and its applications: a pioneering technology in the field of quantitative imaging cytometry. In: Darzynkiewicz Z, Holden E, Orfao A, Telford W, Wlodkowic D, editors. Methods in cell biology. Vol. 102. New York (NY): Academic Press; 2011. p. 159–205.
- Gray JW, Dolbeare F, Pallavicini MG, Beisker W, Waldman F. Cell cycle analysis using flow cytometry. Int J Radiat Biol Relat Stud Phys Chem Med. 1986;49(2):237–255.
- Ahmed MS, Kopel LC, Halaweish FT. Structural optimization and biological screening of a steroidal scaffold possessing cucurbitacin‐like functionalities as B‐raf inhibitors. ChemMedChem. 2014;9(7):1361–1367.
- Noolvi MN, Patel HM. A comparative QSAR analysis and molecular docking studies of quinazoline derivatives as tyrosine kinase (EGFR) inhibitors: a rational approach to anticancer drug design. J Saudi Chem Soc. 2013;17(4):361–379.
- Yang Y-A, Tang W-J, Zhang X, Yuan J-W, Liu X-H, Zhu H-L. Synthesis, molecular docking and biological evaluation of glycyrrhizin analogs as anticancer agents targeting EGFR. Molecules. 2014;19(5):6368–6381.
- Rajendra Prasad VVS, Deepak Reddy G, Kathmann I, Amareswararao M, Peters GJ. Nitric oxide releasing acridone carboxamide derivatives as reverters of doxorubicin resistance in MCF7/Dx cancer cells. Bioorg Chem. 2016;64:51–58.
- Mahnashi M, Elgazwi SM, Ahmed MS, Halaweish FT. Cucurbitacins inspired organic synthesis: potential dual inhibitors targeting EGFR – MAPK pathway. Eur J Med Chem. 2019;173:294–304. [InsertedFromOnli
- Alsaif NA, Dahab MA, Alanazi MM, Obaidullah AJ, Al-Mehizia AA, Alanazi MM, Aldawas S, Mahdy HA, Elkady H. New quinoxaline derivatives as VEGFR-2 inhibitors with anticancer and apoptotic activity: design, molecular modeling, and synthesis. Bioorg Chem. 2021;110:104807–104828.
- Yousef RG, Elkady H, Elkaeed EB, Gobaara IMM, Al-Ghulikah HA, Husein DZ, Ibrahim IM, Metwaly AM, Eissa IH. (E)-N-(3-(1-(2-(4-(2,2,2-Trifluoroacetamido)benzoyl)hydrazono)ethyl)phenyl)nicotinamide: a novel pyridine derivative for inhibiting vascular endothelial growth factor receptor-2: synthesis, computational, and anticancer studies. Molecules. 2022;27(22):7719–7741.
- Elkaeed EB, Yousef RG, Elkady H, Mehany ABM, Alsfouk BA, Husein DZ, Ibrahim IM, Metwaly AM, Eissa IH. In silico, in vitro VEGFR-2 inhibition, and anticancer activity of a 3-(hydrazonomethyl)naphthalene-2-ol derivative. J Biomol Struct Dyn. 2022:1–16.
- Alsaif NA, Taghour MS, Alanazi MM, Obaidullah AJ, Al-Mehizia AA, Alanazi MM, Aldawas S, Elwan A, Elkady H. Discovery of new VEGFR-2 inhibitors based on bis([1, 2, 4]triazolo)[4,3-a:3',4'-c]quinoxaline derivatives as anticancer agents and apoptosis inducers. J Enzyme Inhib Med Chem. 2021;36(1):1093–1114.
- Cody V, Luft JR, Pangborn W. Understanding the role of Leu22 variants in methotrexate resistance: comparison of wild-type and Leu22Arg variant mouse and human dihydrofolate reductase ternary crystal complexes with methotrexate and NADPH. Acta Crystallogr D Biol Crystallogr. 2005;61(Pt 2):147–155.
- Sayre PH, Finer-Moore JS, Fritz TA, Biermann D, Gates SB, MacKellar WC, Patel VF, Stroud RM. Multi-targeted antifolates aimed at avoiding drug resistance form covalent closed inhibitory complexes with human and Escherichia coli thymidylate synthases. J Mol Biol. 2001;313(4):813–829.
- Xiong G, Wu Z, Yi J, Fu L, Yang Z, Hsieh C, Yin M, Zeng X, Wu C, Lu A, et al. ADMETlab 2.0: an integrated online platform for accurate and comprehensive predictions of ADMET properties. Nucleic Acids Res. 2021;4(W1)9:W5–w14.
- ADMETLAB2.0. ADMET Evaluation. 2020; [accessed 2022 May 20]. https://admetmesh.scbdd.com/.
- Cheng C, Robins RK. Potential purine antagonists. VI. Synthesis of 1-alkyl-and 1-aryl-4-substituted pyrazolo[3,4-d]pyrimidines1, 2. J Org Chem. 1956;21(11):1240–1256.
- Kreutzberger A, Burgwitz K. Antimykotische Wirkstoffe, 11. Mitt. 4-Amino-3-methylpyrazolo[3,4-d]pyrimidine. Arch Pharm Pharm Med Chem. 1980;313(11):906–912.
- Silvestri R, Cascio MG, La Regina G, Piscitelli F, Lavecchia A, Brizzi A, Pasquini S, Botta M, Novellino E, Di Marzo V, et al. Synthesis, cannabinoid receptor affinity, and molecular modeling studies of substituted 1-aryl-5-(1H-pyrrol-1-yl)-1H-pyrazole-3-carboxamides. J Med Chem. 2008;51(6):1560–1576.
- Tavakoli-Hoseini N, Moloudi R, Davoodnia A, Shaker M. Synthesis of pyrazolo[3,4-d]pyrimidin-4-ones catalyzed by bronsted-acidic ionic liquids as highly efficient and reusable catalysts. Chin J Chem. 2011;29(11):2421–2426.
- Schmiedeberg N, Furet P, Imbach P, Holzer P. 1,4-Substituted pyrazolopyrimidines as kinase inhibitors. WO2006050946A1; 2006.
- Severina A, Georgiyants V, Shtrygol SY, Kavraiskyi D. Synthesis and alkylation of 1-aryl-1, 5-dihydro-4H-pyrazolo [3, 4-d] pyrimidin-4-ones as possible anticonvulsant agents. Der Pharma Chemica. 2015;7:43–48.
- Bakr RB, Abdelall EK, Abdel-Hamid MK, Kandeel MM. Design and synthesis of new EGFR-tyrosine kinase inhibitors containing pyrazolo[3,4-d]pyrimidine cores as anticancer agents. Bull Pharm Sci Assiut Univ. 2012(1);35:27–42.
- Davoodnia A, Zhiani R, Tavakoli-Hoseini N. Synthesis of pyrazolo[4,3-e][1,2,4]triazolo[4,3-c]pyrimidines. Monatsh Chem. 2008;139(11):1405–1407.
- Budeanu D. Analele Stiintifice ale Universitatii Al I Cuza din Iasi. Sectiunea 1c: Chimie. 1965;11:147–153.
- Yao B-J, Wu W-X, Ding L-G, Dong Y-B. Sulfonic acid and ionic liquid functionalized covalent organic framework for efficient catalysis of the Biginelli reaction. J Org Chem. (3)2021;86:3024–3032.
- Ranu BC, Hajra A, Jana U. Indium(III) chloride-catalyzed one-pot synthesis of dihydropyrimidinones by a three-component coupling of 1,3-dicarbonyl compounds, aldehydes, and urea: an improved procedure for the Biginelli reaction. J Org Chem. 2000;65(19):6270–6272.
- Murata H, Ishitani H, Iwamoto M. Synthesis of Biginelli dihydropyrimidinone derivatives with various substituents on aluminium-planted mesoporous silica catalyst. Org Biomol Chem. 2010;8(5):1202–1211.
- Fekri ZL, Movaghari M. 1,4-Diazabicyclo[2.2.2]octanium diacetate: as a new, effective and reusable catalyst for the synthesis of 3,4- dihydropyrimidin-2(1H)-ones and -thiones. LOC. 2016;13(6):406–413.
- Shaibuna M, Kuniyil MJK, Sreekumar K. Deep eutectic solvent assisted synthesis of dihydropyrimidinones/thiones via Biginelli reaction: theoretical investigations on their electronic and global reactivity descriptors. New J Chem. 2021;45(44):20765–20775.
- Shaaban MA, Elshaier YAMM, Hammad AH, Farag NA, Hassan Haredy H, AbdEl-Ghany AA, Mohamed KO. Design and synthesis of pyrazolo[3,4-d]pyrimidinone derivatives: discovery of selective phosphodiesterase-5 inhibitors. Bioorg Med Chem Lett. 2020;30(16):127337.
- Elbadawi MM, Eldehna WM, Wang W, Agama KK, Pommier Y, Abe M. Discovery of 4-alkoxy-2-aryl-6,7-dimethoxyquinolines as a new class of topoisomerase I inhibitors endowed with potent in vitro anticancer activity. Eur J Med Chem. 2021;215:113261–113281.
- Elmore S. Apoptosis: a review of programmed cell death. Toxicol Pathol. 2007;35(4):495–516.
- OpenEye. OMEGA. Version 4.2.1.1. Santa Fe (NM): OpenEye Scientific Software. 2022; [accessed 2022 Jul 20]. https://docs.eyesopen.com/applications/omega/index.html.
- OpenEye. Chemgauss4; OpenEye Scientific Software documents. 2022; [accessed 2022 Jul 20]. https://docs.eyesopen.com/applications/oedocking/theory/hybrid_theory.html#chemgauss4.
- OpenEye. VIDA. Version 5.0.3.1. Santa Fe (NM): OpenEye Scientific Software. 2022; [accessed 2022 Jul 25]. http://www.eyesopen.com.
- BIOVIA Discovery Studio Visualizer. 2021; [accessed 2022 Jul 10]. https://discover.3ds.com/discovery-studio-visualizer-download.
- Patil SM, Maruthi KR, Bajpe SN, Vyshali VM, Sushmitha S, Akhila C, Ramu R. Comparative molecular docking and simulation analysis of molnupiravir and remdesivir with SARS-CoV-2 RNA dependent RNA polymerase (RdRp). Bioinformation. 2021;17(11):932–939.
- OpenEye. DockingReport. Version 4.2.0.1. Santa Fe (NM): OpenEye Scientific Software. 2022; [accessed 2022 Jul 20]. https://docs.eyesopen.com/applications/oedocking/DockingReport.html#docking-report.