Abstract
Although T-cell-dependent antibody response (TDAR) assays with keyhole limpet hemocyanin (KLH) as specific antigen have many advantages, most experiments produce qualitative results based on antibody titers. It was hypothesized that if experimental conditions (like antigen coating concentration, serum dilution, and detecting [here, horseradish peroxidase-goat anti-mouse IgG] antibody dilution) could be optimized, the resulting measured value (here, optical density) could be used to directly analyze and evaluate the experimental results. This means specifically that the assay OD values could be used for approximate quantitative statistical analysis; it does not require a further conversion of the data into qualitative forms or require obtaining further titer data from additional experiments. As such, the use of this “improved” assay would: greatly reduce the complexity of experimental operations; improve overall sensitivity and practicality of traditional TDAR assays; and, allow for direct assessing of any immunosuppression caused by a test drug in a host. Here, KLH-immunized serum was obtained from BALB/c mice, and the means to detect serum anti-KLH antibodies by an indirect ELISA were optimized. The results indicated that in this system, the optimal KLH coating concentration was 80 μg/ml, the optimal dilution range of the serum (at immunization dose of 5 mg KLH/kg) was 1:200–1:800, and the optimal dilution of HRP-goat anti-mouse IgG antibody was 1:16,000. Methodology verification was performed and a regression model of y = 144.16x + 0.9815 (R2 = 0.9571, indicating very good linearity) was obtained. Intragroup precision was 7.51–9.40%; the intergroup coefficient of variation was 9.83–14.22%. The lower limit of detection was 0.1385. The present results showed this indirect ELISA exhibited very good linearity, accuracy, and precision.
Introduction
In recent years, the T-cell-dependent antibody response (TDAR) has been considered a functional test with good predictability in detecting potential immunotoxicity of some drugs. By using exogenous antigens, this technique can reflect the overall effect of a tested drug on the immune system, and can predict changes in immune function in addition to permitting an early prediction of the role of drugs in immunoregulation and immunotoxicity. With these advantages, the TDAR has been widely used in evaluations of drug immunotoxicity (Rhodes et al. Citation2012; Vandebriel et al. Citation2014). However, due to poor antigenic specificity, complex and labor intensive manipulation, and inherently high variability, traditional TDAR tests clearly display many defects, such as low specificity, sensitivity, precision, reproducibility, and end-user operability.
When compared with sheep red blood cells (SRBC), keyhole limpet hemocyanin (KLH) has several inherent advantages including those of high stability, simple standardization and ease of acquisition, and is thus regarded more suitable as a T-cell-dependent antibody since it displays decreased immunological variability and greater stability than SRBC (Kawai et al. Citation2013). Though the use of KLH as a specific antigen in TARD possesses many advantages, it also possesses several important problems. First, the need for differential immunological doses, routes of administration or cycles of immunization could provoke different and highly variable effects; thereby affecting the sensitivity of the assay. Second, most TDAR tests would use indirect ELISA, which only provides qualitative data; thus, they may not necessarily reflect the immunotoxicity of immunosuppressive drugs or agonists. Further, some known immunosuppressive agents, like chemotherapeutic drugs, have been confirmed to display immunosuppressive effects. Consequently, if the degree of immunosuppression requires investigation, the process would require other methods, including quantitative analyses.
Thus, if the experimental conditions (including antigen coating concentration, serum dilution, and detecting antibody [here, horseradish peroxidase-goat anti-mouse IgG]) dilution could be optimized, the resulting measured value (here, optical density) could be used to directly analyze and evaluate the experimental results. This means specifically that the assay OD values could be used for approximate quantitative statistical analysis; it does not require a further conversion of the data into qualitative forms or require obtaining further titer data from additional experiments. As such, the use of this current assay could greatly reduce the complexity of experimental operations, improve overall sensitivity and practicality of traditional TDAR assays, and allow for direct assessing of any immunosuppression caused by a test drug in a host. Accordingly, this study was undertaken to attempt to optimize experimental conditions for future TDAR assays and to validate this methodology.
Materials and methods
Reagents
ELISA plates were purchased from Jet Biofil (Guangzhou, China). Horseradish peroxidase (HRP)-conjugated goat anti-mouse IgG antibody was bought from Bioss (Beijing, China). Other reagents purchased were Tween-20 (Aladdin, Shanghai, China), PBS (phosphate- buffered saline [pH 7.4], Boster Biological Technology Co., Wuhan, China), KLH (Sigma, St. Louis, MO), and TMB (3,3′,5,5′-tetramethylbenzidine reagent for detecting presence of horse- radish peroxidase [Nanjing Senbeijia Biological Technology Co., Nanjing, China]).
Animals
BALB/c mice (18–22 g, both female and male, 6–8-weeks-of-age) were purchased from Hunan SJA Laboratory Animal Co. (Changsha, China). All animals were housed in polypropylene cages in specific pathogen-free rooms maintained at 20–26 °C with a 40–70% relative humidity and a 12-h light cycle. All animals had ad libitum access to standard rodent chow and filtered tap water. All experiments were approved and conducted under the Research Center for Drug Safety Evaluation of Hainan Province Animal Care and Use Committee.
KLH immunization
An intraperitoneal injection dose of KLH-saline solution at 5 mg KLH/kg was selected based on a preliminary study (data not shown) and the earlier study of Kawai et al. (Citation2013). Mice were immunized twice, at an interval of 7 d between each immunization (i.e. days 1 and 8). On day 15 after the first immunization, blood was sampled from the retro-orbital plexus, and the serum was separated and stored at –80 °C for further study of expected increases in circulating IgG in these hosts. For the studies below, to reduce the impact of any inter-animal variability, serum samples from all immunized mice were combined into a “mixed serum” sample that was then use for the analyses.
Optimizing an indirect ELISA method with KLH as specific antigen
The general methods used on all plates operated followed the sequence: well-coating, sample (i.e. serum) addition, addition of detecting antibody, plate development, and estimation of levels of bound detecting antibody (OD measurement).
Well coating
KLH was dissolved in coating solution (carbonate buffer, pH 9.6) and then added to the wells at 100 μl/well. After incubation overnight at 4 °C, the liquid in each well was discarded and the plates washed twice with 0.05% (v/v) Tween-20/PBS solution (PBS-T). The plates were then blocked with 5% skimmed milk/deionized water (200 μl/well) at room temperature (RT) for 1 h. Thereafter, the liquid was discarded and the plates used for the analyses below (or stored at 4 °C for future use).
Sample (i.e. test serum) addition
Before adding the test “mixed serum” sample, each well was again blocked with 5% skim milk solution (200 μl/well) for 1 h at RT. The liquid was then discarded and the plate washed twice with PBS-T. The mixed serum sample was then diluted to the desired titer in 1% BSA/PBS solution and then added to dedicated wells at a volume of 50 μl. Pre-immunization serum was used as a negative control and PBS alone was used as a blank control. The plates were then incubated at 37 °C for 1 h.
Detection antibody addition
Following either time period, each plate was washed three times with PBS-T. The wells in each plate then received a solution (50 μl/well) of HRP-goat anti-mouse IgG antibody that had been diluted to the desired titer in 1% BSA/PBS solution. Plates were then incubated at RT for 1 h, washed three times with PBS-T, soaked in washing liquid for 5 min, and then washed an additional three times with PBS-T.
Developing the plate and estimation of bound detection antibody
Each well on each plate then received TMB substrate solution (100 μl/well) and the plates were placed in the dark at RT for 10–20 min. Termination solution (50 μl 2 M H2SO4/well) was then added to each well and the plates were incubated a further 3–5 min. The OD value in each well was then measured at 450 nm in a 96-well plate reader (TECAN, Männedorf, Switzerland) with the reference wavelength set at 630 nm.
Determining optimal antigen well wall-coating concentration
While holding the levels of dilution of the test serum and the detecting antibody (i.e. HRP-goat anti-mouse IgG) fixed, the optimal antigen (KLH) coating concentration for use in the assay plates was determined. For these analyses, four dilutions of KLH were evaluated, i.e. 20, 40, 80, and 160 μg/ml coating solution. Using the general protocols below, the optimal coating concentration was then determined using a “mixed serum” sample generated from the pooled sera from all the KLH-immunized mice (diluted 1:800 in 1% BSA/PBS) and HRP-conjugated goat-anti-mouse IgG antibody (diluted at 1:16,000 in 1% BSA/PBS).
Determining optimal dilutions of serum and HRP-goat anti-mouse IgG
Matrix titration (chessboard titration) was used to determine the optimal dilutions of both the test serum (i.e. “mixed serum” generated from pooling of sera from all the KLH-immunized mice) and the detection antibody (i.e. HRP-goat anti-mouse IgG) for use in this modified assay. Using the optimal well coating concentration determined above, plates were coated, blocked, and then test serum added to dedicated wells at dilutions of 1:50, 1:100, 1:200, 1:400, 1:800, 1:1600, 1:3200, or 1:6400 in 1% BSA/PBS (each in triplicate for each subsequent dilution of detection antibody [i.e. 18 wells/serum dilution]). Following the 1 h incubation and then the washing of the plates, HRP-goat anti-mouse IgG was added to dedicated wells of each serum dilution; for each serum sample dilution, dedicated wells then received a fixed dilution of the detection antibody (i.e. 1:2000, 1:4000, 1:8000, 1:16,000, 1:32,000, or 1:64,000 in 1% BSA/PBS). After the 1 h incubation, the plates were developed to estimate levels of bound detection (OD values).
To define the optimal test serum dilution, the one that resulted in an ODserum sample close to 1 (i.e. 1 < OD < 2) was sought. The optimal detection antibody dilution was determined by calculating the ODserum sample/ODnegative control ratio. When the ratio was at its highest (for a given dilution of serum) and also > 2.1, this was deemed the optimal dilution.
Reproducibility
To test for reproducibility, the mixed serum sample from the KLH-immunized mice was diluted at high (1:200), medium (1:400), and low (1:800) concentrations within the linear range for quality control metrics. Each concentration within a group had six replicates, and each group had six parallel measurements. Coating, blocking, incubation, development, and measurements were performed using the now-established optimal conditions to measure intragroup precision as well as intergroup variation.
Lower limit of quantitation (LOQ)
By repeating measurements of the blank control (1:400) more than 20 times, OD values that corresponded to the mean + 10 standard deviations (SD) of the blank were subsequently measured. When the number of blank measurements (n) was ≥ 20, then the LOQ was equal to 4.6 σwb, where σwb was the SD of the blank parallel measurements (i.e. intragroup measures).
Assessing utility of the ELISA with serum from immunosuppressed hosts
To ascertain if this “improved” ELISA could be useful for evaluating responses in hosts that have also been exposed to an immunosuppressant, serum was collected from mice that had been exposed to cyclophosphamide (CTX) prior to beginning KLH immunizations. Specifically, a total of 36 BALB/c mice were obtained and randomly distributed into a negative control group, a parallel control group, and a cyclophosphamide (CTX) group. The parallel control and CTX mice were each given two daily intraperitoneal injections of 40 mg CTX/kg; negative control mice were injected with normal saline at the same volume (never exceeded 500 µl/mouse). This CTX dose was selected based on the studies of Chen et al. (Citation2019). On day 5 after the second CTX/saline injection, all mice then underwent the first of their two KLH immunizations (or received saline each time) that occurred 7 d apart as before. On day 15 after the first KLH/saline injection, blood was collected from all mice, and serum was separated for analyses.
TDAR OD value (quantitative) and qualitative analyses
OD value (quantitative) assay
Here, coating concentration used = 80 μg KLH/ml, dilution of serum = 1:400, and dilution of HRP-goat anti-mouse IgG = 1:16,000. All samples were evaluated in duplicate. Results are presented as OD values.
Qualitative assay
Here, coating concentration = 20 μg KLH/ml, serum dilution = 1:5, and dilution of HPR-goat anti-mouse IgG = 1:16,000. All samples were evaluated in duplicate. Results were deemed positive when (ODsample serum – ODblank control)/(ODnegative control – ODblank control) was > 2.1.
Statistical analysis
Data were analyzed using Office Excel 2007 data analysis package, and SPSS statistical analysis software (v(0).24, SPSS Inc., Cary, NC). The results were fitted to a linear regression model to analyze the linearity and linear range of the derived data, and the R2 > 0.95. Intragroup precision and intergroup variation of the data are presented as relative standard deviation (RSD). Intergroup comparisons of measured data were made using a one-way analysis of variance (ANOVA). A χ2-test was performed to analyze the qualitative data.
Results
Coating concentration, serum dilution, and HRP-goat anti-mouse IgG antibody dilution
The results of the studies to determine the optimal KLH antigen coating concentration showed that OD values increased with the level of coating antigen up to 80 μg/ml. Above this, OD values ceased to significantly increase. Thus, optimal coating concentration here was defined as 80 μg/ml (). Based on this, it was then seen that when the dilution of detection HRP-goat anti-mouse IgG antibody was 1:16,000, the ODsample/ODnegative control ratio was at its highest and > 2.1 (). Similarly, when the serum was diluted in the range of 1:100–1:1600, the results showed good linearity (results see below). Accordingly, the optimal dilution of the serum sample appeared to be in the 1:100–1:1600 range (but seemingly best at 1:200–1:800).
Figure 1. OD values obtained using different KLH coating concentrations. The results showed that when the concentration of coated antigen reached 80 µg/ml, the OD value did not increase significantly when higher concentrations were employed. For this assay, test sera dilution was fixed at 1:800 (in 1% BSA/PBS) and the HRP-conjugated goat-anti-mouse IgG antibody dilution was fixed at 1:16,000 (in 1% BSA/PBS).
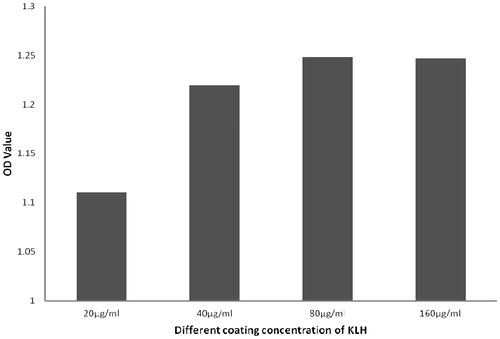
Table 1. ODsample/ODnegative ratios.
Linearity and linear range
The “mixed serum” sample for the assay was diluted (1:50, 1:100, 1:200, 1:400, 1:800, 1:1600, 1:3200, and 1:6400) in 1% BSA/PBS. Assay results were then fitted into a linear regression model to determine the linear range. When the serum was diluted in a range of 1:100–1:1600, the results showed good linearity, i.e. y = 144.16x + 0.9815 with an R2 = 0.9571 ().
Repeatability (intra- and between-group precision) and assay LOQ
The intra- and intergroup variability of the modified ELISA was analyzed using OD values derived with quality control samples. The data indicated that the intragroup precision of quality control samples diluted to 1:200, 1:400, and 1:800 was 7.51%, 9.40%, and 8.33%, respectively. The corresponding intergroup variation was 9.83%, 14.22%, and 14.10%. As the observed intragroup RSD was < 10% and the intergroup RSD < 15–20%, the indirect ELISA procedure established in this study was deemed to have exhibited high accuracy and precision ( and ).
Table 2. Within-group RSD.
Table 3. Between-group RSD.
In addition, after measuring the pre-immunized serum (1:400) more than 20-times, the OD of the mean blank and standard deviation was calculated to be 0.132 and 0.030, respectively. Thus, the LOQ was determined to be 0.138.
TDAR OD value (quantitative) versus qualitative analyses
According to observations derived from these specific analyses, the mean concentration of peripheral blood anti-KLH IgG was significantly lower in the CTX (received KLH) and parallel control (received CTX, no KLH) mice when compared to levels in the blood of the negative control (no CTX, received KLH) mice. However, χ2 analyses could not find significant differences between blood antibody levels in the CTX and negative control mice (χ2 = 0.374, p > 0.05). In comparison, blood levels of the antibody for the parallel vs. the CTX and the negative control mice differed significantly (χ2 = 24.89 and χ2 = 28.34, respectively, both p < 0.001) ( and ).
Table 4. OD value analysis of anti-KLH IgG in peripheral blood of mice.
Table 5. Analysis of anti-KLH IgG in peripheral blood of mice.
Discussion
The TDAR is an immune response initiated by B-cell activation following interaction with T-cell-dependent processed antigens. This process requires participation of CD4+ T-helper Type 2 (TH2) cells. Recently, TDAR has been considered as a functional test with favorable predictability in detecting the potential immunotoxicity of select drugs. Keyhole limpet hemocyanin (KLH) is a soluble antigenic protein extracted from marine organisms that in mammals is an allogenic protein with a high degree of immunogenicity. Compared to other common antigens like sheep red blood cells (SRBC) (Ladics Citation2018), KLH is more suited as a T-cell-dependent antigen because it displays greater stability in nature, is simple to be standardized, and is easy to be obtained. In studies by Lebrec et al. (Citation2014) and Peachee et al. (Citation2014), KLH was used as the specific antigen to detect TDAR in rats and pigs by ELISA. Those authors found that KLH resulted in less variability and more stable results when compared with outcomes in hosts given SRBC. Apart from the use of a different antigen to optimize measures, the advantages of using an ELISA over a hemolytic plaque test (PFC) was that the former provided standardized measurements suitable for automation in large-volume operations (Lebrec et al. Citation2014). The use of ELISA for measurements also allowed for a continuous collection of samples, and for serum samples to be frozen prior to analysis (thus, making analyses more flexible and less labor-intensive (namely, if doing PFC, for investigators).
Though the use of KLH as the specific antigen provides many advantages for a TDAR, there are still some problems (Swaminathan et al. Citation2014). The use of KLH might lead to low sensitivity (Plitnick and Herzyk Citation2010). Further, indirect ELISA is often used to measure KLH-specific antibodies, but KLH has a high molecular weight and many epitopes. So, the indirect ELISA only produces qualitative or semi-quantitative results, and the latter are often represented by antibody titers that might not accurately reflect effects of immunosuppressants (Bugelski and Kim Citation2007; Gehen et al. Citation2014), including chemotherapeutics. Thus, if results of a TDAR could be developed that could be qualified, and its OD values used to compare different immune effects between treatment groups, the sensitivity and applicability of that TDAR would be significantly improved (especially, if there is a need to compensate for any degree of effects from any immunosuppressants).
In the present study, conditions were optimized for this modified indirect ELISA and methodological verification was performed. The results showed that the linearity of the optimized TDAR was good, and the technical indexes such as inter-batch precision, inter-batch difference, and quantitative lower limit met the requirements of the experiment. It was also seen that the direct OD value analysis method of TDAR established according to the above optimization conditions could meet the requirements of accuracy and precision and could be used for statistical analyses among groups. Though the modified system could not report out the actual concentrations of anti-KLH antibody (i.e. X pg Ab/ml serum), the OD values measured still reflected the levels of the antibody in the host serum.
At present, there have been many studies on drug immunotoxicology that require TDAR detection. Most of these tests have been qualitative experiments and only a few have been semi-quantitative (i.e. analyses using antibody titers (Gallegos et al. Citation2013; Potter et al. Citation2018)). Quantitative analysis could be carried out by flow cytometry (i.e. bead array) to detect specific KLH antibodies (Ferbas et al. Citation2013). However, such detection methods are either expensive or are labor intensive. Quantitative analyses using indirect ELISAs have not been reported.
To test the utility of this modified assay, effects of a known immunosuppressant (cyclophosphamide) on anti-KLH antibody formation were evaluated in the now-optimized ELISA. When analyzed by estimates of the OD value and by qualitative TDAR, treatment of the hosts with cyclophosphamide resulted in quantitatively lower levels of peripheral blood anti-KLH antibodies when compared with levels in mice that received KLH only. On the other hand, no significant differences between these groups were noted when the qualitative analysis was performed. This suggested to us that, going forward, the TDAR OD value assay might better detect any effects of immunosuppressants overall. But this method also has some drawbacks, because microplates used to affect the adsorption of coated antigen and the optimal dilution of secondary (detection) antibody depends on its manufacturers and products, each test facility should always conduct validation of TDAR assay before immunotoxicity testing.
Even so, the “improved” TDAR described here could allow for a ready quantification of results and a significantly reduced workload. Therefore, this approach could be suitable to evaluate/detect the immunotoxicity of a variety of drugs, especially immunosuppressants. Beside establishing a reliable test procedure, and optimizing its conditions, the current study has also set out foundations by which others might develop more sensitive and reliable detection kits in the future.
Disclosure statement
No potential conflict of interest was reported by the authors.
Additional information
Funding
References
- Bugelski P, Kim C. 2007. T-Dependent antigen response (TDAR) tests: Meta-analysis of results generated across multiple laboratories. J Immunotoxicol. 4:159–164.
- Chen L, Qi Y, Qi Z, Gao K, Gong R, Shao Z, Liu S, Li S, Sun Y. 2019. A comparative study on the effects of different parts of panax ginseng on the immune activity of cyclophosphamide-induced immunosuppressed mice. Molecules. 4:1096.
- Ferbas J, Belouski S, Horner M, Kaliyaperumal A, Chen L, Boyce M, Colaço C, McHugh N, Quick V, Nicholl R. 2013. A novel assay to measure B-cell responses to keyhole limpet hemocyanin vaccination in healthy volunteers and subjects with systemic lupus erythematosus. Br J Clin Pharmacol. 76:188–202.
- Gallegos A, Hoerger M, Talbot N, Krasner M, Knight J, Moynihan J, Duberstein P. 2013. Toward identifying effects of specific components of mindfulness-based stress reduction on biologic and emotional outcomes among older adults. J Alternat Complement Med. 19:787–792.
- Gehen SC, Blacker AM, Boverhof DR, Hanley TR, Hastings CE, Ladics GS, Lu H, O’Neal FO. 2014. Retrospective evaluation of impact of functional immunotoxicity testing on pesticide hazard identification and risk assessment. Crit Rev Toxicol. 44:407–419.
- Kawai R, Ito S, Aida T, Hattori H, Kimura T, Furukawa T, Mori K, Sanbuissho A, Kawada T. 2013. Evaluation of primary and secondary responses to a T-cell-dependent antigen, keyhole limpet hemocyanin, in rats. J Immunotoxicol. 10:40–48.
- Ladics G. 2018. The sheep erythrocyte T-dependent antibody response (TDAR). Methods Mol Biol. 1803:83–94.
- Lebrec H, Hock M, Sundsmo J, Mytych D, Chow H, Carlock L, Joubert M, Reindel J, Zhou L, Bussiere J. 2014. T-Cell-dependent antibody responses in the rat: Forms and sources of keyhole limpet hemocyanin matter. J Immunotoxicol. 11:213–221.
- Lebrec H, Molinier B, Boverhof D, Collinge M, Freebern W, Henson K, Mytych DT, Ochs HD, Wange R, Yang Y, et al. 2014. The T-cell-dependent antibody response assay in non-clinical studies of pharmaceuticals and chemicals: Study design, data analysis, interpretation. Regul Toxicol Pharmacol. 69:7–21.
- Peachee V, Smith M, Beck M, Stump D, White K. 2014. Characterization of the T-dependent antibody response (TDAR) to keyhole limpet hemocyanin (KLH) in the Göttingen minipig. J Immunotoxicol. 11:376–382.
- Plitnick L, Herzyk D. 2010. The T-dependent antibody response to keyhole limpet hemocyanin in rodents. Methods Mol Biol. 598:159–171.
- Potter T, Neun B, Dobrovolskaia M. 2018. Methods for analysis of nanoparticle immunosup- pressive properties in vitro and in vivo. Meth Mol Biol. 1682:161–172.
- Rhodes M, Laffan S, Genell C, Gower J, Maier C, Fukushima T, Nichols G, Bassiri A. 2012. Assessing a theoretical risk of dolutegravir-induced developmental immunotoxicity in juvenile rats. Toxicol Sci. 130:70–81.
- Swaminathan A, Lucas R, Dear K, McMichael A. 2014. Keyhole limpet haemocyanin – a model antigen for human immunotoxicological studies. Br J Clin Pharmacol. 78:1135–1142.
- Vandebriel R, Tonk E, De la Fonteyne-Blankestijn L, Gremmer E, Verharen H, van der Ven L, van Loveren H, de Jong W. 2014. Immunotoxicity of silver nanoparticles in an intravenous 28-day repeated-dose toxicity study in rats. Part Fibre Toxicol. 11:21.