Abstract
Glyphosate, or N-phosphomethyl(glycine), is an organophosphorus compound and a competitive inhibitor of the shikimate pathway that allows aromatic amino acid biosynthesis in plants and microorganisms. Its utilization in broad-spectrum herbicides, such as RoundUp®, has continued to increase since 1974; glyphosate, as well as its primary metabolite aminomethylphosphonic acid, is measured in soils, water, plants, animals and food. In humans, glyphosate is detected in blood and urine, especially in exposed workers, and is excreted within a few days. It has long been regarded as harmless in animals, but growing literature has reported health risks associated with glyphosate and glyphosate-based herbicides. In 2017, the International Agency for Research on Cancer (IARC) classified glyphosate as “probably carcinogenic” in humans. However, other national agencies did not tighten their glyphosate restrictions and even prolonged authorizations of its use. There are also discrepancies between countries’ authorized levels, demonstrating an absence of a clear consensus on glyphosate to date. This review details the effects of glyphosate and glyphosate-based herbicides on fish and mammal health, focusing on the immune system. Increasing evidence shows that glyphosate and glyphosate-based herbicides exhibit cytotoxic and genotoxic effects, increase oxidative stress, disrupt the estrogen pathway, impair some cerebral functions, and allegedly correlate with some cancers. Glyphosate effects on the immune system appear to alter the complement cascade, phagocytic function, and lymphocyte responses, and increase the production of pro-inflammatory cytokines in fish. In mammals, including humans, glyphosate mainly has cytotoxic and genotoxic effects, causes inflammation, and affects lymphocyte functions and the interactions between microorganisms and the immune system. Importantly, even as many outcomes are still being debated, evidence points to a need for more studies to better decipher the risks from glyphosate and better regulation of its global utilization.
Introduction
Glyphosate (N-phosphomethyl[glycine]), is an organophosphorus compound with herbicide properties discovered in 1970. It is a competitive inhibitor of the 5-enolpyruvylshikimate-3-phosphate synthase, an enzyme involved in aromatic amino acid biosynthesis in plants and microorganisms () (Steinrücken and Amrhein Citation1984). In 1974, Monsanto started its commercialization as a broad-spectrum herbicide. This first glyphosate-based herbicide (GBH), RoundUp®, and the others that followed such as Glyphogan®, Touchdown®, or Glifloglex®, are mixtures of glyphosate and various adjuvants used to boost its penetration in plants and enhance its activity (Williams et al. Citation2000). Most of them are surfactants, such as polyethoxylated tallow amine (POEA). First used as a desiccant and in the pretreatment of crops, RoundUp® utilization spreads dramatically since 1995 with the development of RoundUp®-resistant plants (Benbrook Citation2016). Glyphosate is now the most used herbicide globally, and its usage keeps increasing with the emergence of weed resistance, from 16 million kg spread in the world in 1994 to 79 million kg spread in 2014, including 15% in the United States alone (Benbrook Citation2016).
Figure 1. Chemical structures underlying glyphosate herbicidal function, its metabolites and estrogenic potential. (A) Representation of the shikimate pathway that allows the biosynthesis of aromatic amino acids tryptophan, tyrosine and phenylalanine (represented in green) in plants and microorganisms, but not in animals. Glyphosate (represented in the red box) acts as a competitive inhibitor of 5-enolpyruvylshikimate-3-phosphate (EPSP) synthase, an enzyme with a central role in aromatic amino acid biosynthesis. (B) Chemical structures of glyphosate and its two primary metabolites, aminomethylphosphonic acid (AMPA) and methylphosphonic acid (MPA), as well as a rarer metabolite, glyoxylate. (C) Molecular structure of estrogen; glyphosate mimics its action and potentially uses the same molecular pathway.
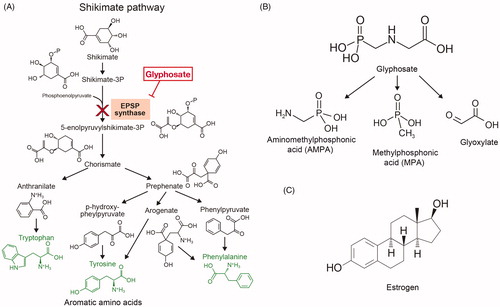
Once in the environment, glyphosate is metabolized by microorganisms into aminomethylphosphonic acid (AMPA; known as its most active metabolite) and methylphosphonic acid (MPA) () (Williams et al. Citation2000). Glyphosate and its metabolite AMPA can be found in soils, water, plants, food, and animals (Alferness and Iwata Citation2002; Caloni et al. Citation2016; El-Gendy et al. Citation2018). Glyphosate is detected in human urine, blood, and maternal milk, with urinary levels of 0.26–73.5 μg/L in exposed workers and 0.16–7.6 μg/L in the general population (Acquavella et al. Citation2004; Gillezeau et al. Citation2019). Glyphosate most likely enters the body via the dermal, oral and pulmonary routes (Martinez et al. Citation1990; Williams et al. Citation2000). Even if the dermal route allows a poor absorption (about 2%), it is the main reported route of entry in exposed farmers (Connolly et al. Citation2019). Glyphosate then seems to accumulate principally in the kidneys, liver, colon, and small intestine and is eliminated in the feces (90%) and urine within 48 h (Williams et al. Citation2000). Because of this omnipresence, its safety is of grave concern. Glyphosate has long been regarded as harmless allegedly because it targets an enzyme inexistent in animals, is supposedly degraded into CO2, and its formulation contains misleadingly-called “inert” ingredients. Nevertheless, there is growing literature that describes the risks for glyphosate and GBHs on human health (Vandenberg et al. Citation2017).
After more than 40 years of global use, glyphosate has been classified as “probably carcinogenic” in humans by the International Agency for Research on Cancer (IARC). Nonetheless, as of 2017, other national or international organizations, such as the European Commission or Health Canada, renewed their glyphosate use authorizations for 5 or 10 years, respectively, based on scientific evidence. However, many studies’ reliability is questioned with the 2017 “Monsanto Papers” scandal. Monsanto allegedly interfered with the publication of valuable information on glyphosate toxicity and ghost-wrote papers proving glyphosate safety (McHenry Citation2018). In this context, it seems relevant to give an overview of the reported effects of glyphosate and GBHs on animal health, from cellular to systemic effects, with a specific focus on the immune system of fish, non-human mammals and humans. The animal data is valuable per se, not only because numerous species are affected by glyphosate environmental contamination, which could eventually be passed along the food chain, but they also provide valuable information where human data may be scarce, considering that various mammals and fish are standard laboratory models and share highly conserved immune structures and defence mechanisms with humans (Secombes and Wang Citation2012).
Impacts and toxicity on animal health
Cellular effects
The cellular effects of glyphosate are somewhat disputable in the literature depending on the tested formulations and the studied cell types – immune cells being discussed separately later in this review. In a model of rat cardiac cells, no significant toxicity was shown at environmentally-relevant concentrations of glyphosate, while a dose-dependent toxicity was observed for a constant glyphosate concentration with increasing levels of TN20 surfactant (Kim et al. Citation2013); this suggests that GBH components other than glyphosate have toxic effects. Moreover, these authors determined that with glyphosate alone or in combination with low doses of TN20, cells were equally dying from apoptosis and necrosis, whereas the main cell death was apoptosis when TN20 doses were enhanced. In a study where rats were exposed for 18 months to a mixture of glyphosate and 12 other chemicals, some dose-dependent cytotoxic effects were noted in testis and kidney cells (Tsatsakis et al. Citation2019). However, it is difficult to determine to what extent glyphosate contributed to this cytotoxicity. In humans, on the one hand, studies on glyphosate alone did not show significant cytotoxicity at environmentally-relevant concentrations, in both healthy and fibrosarcoma human cells (Monroy et al. Citation2005). On the other hand, GBHs exhibit dose-dependent toxicity, even at sub-agricultural concentrations. RoundUp®, as well as another GBH Glyphogan®, increases cell death among human Sertoli cells (somatic cells of the testis), with more significant toxicity from Glyphogan® (Vanlaeys et al. Citation2018). Altogether, these results highlight the importance of studying GBHs rather than glyphosate alone.
Other studies have examined the genotoxicity associated with glyphosate and GBHs. These types of effects have mostly been tested by measuring DNA strand-breaks using a Comet assay; overall, results depended on the cell type. In erythrocytes of the Nile tilapia fish (Oreochromis niloticus), glyphosate alone appeared highly genotoxic (Alvarez-Moya et al. Citation2014) while in healthy and fibrosarcoma human cells, it was genotoxic only at high concentrations (Monroy et al. Citation2005). These results collectively demonstrate that glyphosate and GBHs can impart cytotoxicity, genotoxicity, or both, and such outcomes seem to depend on the cell type.
In Caenorhabditis elegans, increased hydrogen peroxide (H2O2) production was observed after Touchdown® exposure (Burchfield et al. Citation2019). Environmentally-realistic concentrations of GBHs also lower the activity of anti-oxidant enzymes, i.e. catalase, glutathione peroxidase, and superoxide dismutase, in rainbow trout (Oncorhynchus mykiss) (Topal et al. Citation2015) and goldfish (Carassius auratus) (Lushchak et al. Citation2009). Interestingly, Topal and colleagues showed that even though enzymatic activities were reduced, their expression was increased. The authors hypothesized GBHs caused oxidative stress, leading to the increased expression of anti-oxidant enzymes – but that their activities could be inhibited by glyphosate or other toxic ingredients in the herbicide formulations. When higher concentrations of pesticides were tested on two teleostean fish species, glutathione-S-transferase activity was reduced to 70–80% while catalase and lipid peroxidase activities increased to 150% and 130%, respectively (Samanta et al. Citation2014). In humans, glutathione-S-transferase activity was reduced in Sertoli cells exposed to RoundUp® or Glyphogan®, but not to glyphosate alone (Vanlaeys et al. Citation2018). When taken together, these results suggest that GBHs can, at least, cause mild oxidative stress.
Given their implication in oxidative stress, GBHs are inclined to alter energy metabolism and cellular biochemical processes as well. Indeed, in C. elegans exposed to environmentally-relevant concentrations of Touchdown®, a reduction in oxygen consumption and complex II activity was noted, suggesting a decrease in mitochondrial respiration; but complex IV seems unscathed, with its activity even increased (Burchfield et al. Citation2019). This observation could indicate that GBHs affect mitochondrial respiration upstream of complex IV. Both the proton gradient and the ATP levels were reduced in C. elegans (Burchfield et al. Citation2019), as a result of complex dysfunctions. A decrease in the activity of both complex II and III, as well as in mitochondrial membrane potentials, was observed in rat liver cells (Peixoto Citation2005). In human Sertoli cells, Vanlaeys et al. (Citation2018) also observed a reduction in the complex II activity.
There is also some evidence that lipid metabolism could be affected. In mice, a mapping of glyoxylate targets, a minor glyphosate metabolite (), found that the fatty acid metabolism, particularly the fatty acid oxidation, as the most down-regulated pathway (Ford et al. Citation2017). Consequently, GBHs seem able to lower both mitochondrial respiration and fatty acid oxidation, which in turn most likely modulate cellular and functional responses.
Reproductive, hormonal, and teratogenic effects
The deregulation of the estrogen pathway by glyphosate and GBHs has been largely documented. First, the aromatase production of estrogen is inhibited by RoundUp® in human placental and embryonic cell lines (Richard et al. Citation2005; Benachour et al. Citation2007). Competitive inhibition of the enzyme has been suggested for glyphosate, an effect boosted in herbicide formulations because adjuvants enhance glyphosate solubilization and action (Richard et al. Citation2005). In breast cancer cell lines, Mesnage et al. (Citation2017) showed that glyphosate also activated estrogen receptors (ER) – probably by an indirect mechanism, since it was structurally unable to bind ERα, as illustrated by the profoundly different molecular structures of glyphosate and estrogen (). However, Thongprakaisang et al. (Citation2013) suggested that glyphosate mimics estrogen activity through interaction with its two receptors, ERα and ERβ, because ER antagonists block glyphosate action. Moreover, even if glyphosate could lead up to 50% of the estrogen response and induce the proliferation of breast cancer cells as well as the expression of both ERs after 6 h of exposure and only ERα at 24 h, this compound acted as an antagonist in the presence of the endogenous estrogen. Hence, glyphosate may act as an endocrine disruptor of estrogen pathways.
Testosterone production is also reduced in male offspring of rats exposed to nontoxic doses of glyphosate. This endocrine disruption altered reproductive behavior, with modifications of sexual preference and a delay in the first mount of females to initiate reproduction (Romano et al. Citation2012). RoundUp® could also reduce progesterone production in human placental cells, but only at higher concentrations than ones causing cell death, suggesting that the effect on progesterone is probably related to the cytotoxicity (Young et al. Citation2015). Human pregnancies were also affected by GBHs, as shown by a correlation between GBH exposure and a higher frequency of miscarriages and pre-term births (Arbuckle et al. Citation2001). This phenomenon could be linked to glyphosate cytotoxicity, as such an effect was noted on umbilical, embryonic, and placental human cell lines (Benachour and Séralini Citation2009; Thongprakaisang et al. Citation2013).
Lastly, teratogenicity from GBHs has also been shown in fish (mostly as cardiac defects) as well as in rats, mice, and chickens (with, notably, bone and brain malformations) (Dallegrave et al. Citation2003; Fagan et al. Citation2012; Roy et al. Citation2016). In humans, GBH exposure of parents before the birth of their child was correlated with a higher frequency of various malformations, ranging from cleft lip to Down’s syndrome (Campaña et al. Citation2010). Based on the above, it appears that glyphosate and GBHs can cause perturbations of the reproductive system at different levels, from hormonal pathway disruption to potential teratogenicity.
Neurologic effects
Some GBH components can cross the blood-brain barrier, as shown by acute poisoning cases with neurological alterations. For instance, a 58-year-old woman who attempted suicide by ingesting a large amount of a glyphosate-surfactant herbicide subsequently developed aseptic meningitis with measurable glyphosate concentrations in her cerebrospinal fluid (Sato et al. Citation2011). A month after massive spraying of RoundUp® without protection, a 70-year-old man developed a vasculitic neuropathy, which was allegedly linked to the herbicide (Kawagashira et al. Citation2017). Hence, GBH acute poisoning or extensive exposure could promote neurological disease development.
Besides their unconventional use for suicidal purposes, a correlation between exposure to organophosphate pesticides and suicide rates has been suspected (London et al. Citation2005). Anxiety behaviors were observed in mice exposed intra-nasally to low doses of Glifloglex® (Baier et al. Citation2017). Likewise, Ait Bali et al. (Citation2017, Citation2018) showed that mouse chronic (12 weeks) and even sub-chronic (6 weeks) oral exposures to RoundUp® caused anxiety and depression-like behaviors, for instance, decreased grooming time or increased immobility in open-field experiments. The same depression-like behaviors were observed in rat offspring exposed to GBHs in utero and via lactation from dams given slightly-contaminated water (Cattani et al. Citation2017). The dopamine pathway was found to be altered in mouse brains after chronic exposure to RoundUp® (Ait Bali et al. Citation2017). In these hosts, there was a decrease in brain levels of tyrosine hydroxylase, the enzyme allowing the production of the dopamine precursor 3,4-dihydroxyphenylalanine (L-DOPA). After chronic or sub-chronic exposure, the brains of treated mice displayed decreased levels of serotonin (Ait Bali et al. Citation2017). This induced anxiety and depression-like behaviors also seem linked to gut microbiota dysbiosis (Ait Bali et al. Citation2018). Altogether, these studies suggest a causal effect of GBHs on the induction of anxiety and depression-like behaviors, at least in rodents.
The dopaminergic pathway being involved in locomotion and learning capabilities, GBH alterations of this pathway suggest a possible alteration of these two functions. Indeed, mice chronically- or sub-chronically-exposed to RoundUp® by gavage evidenced decreased locomotor activity (Ait Bali et al. Citation2017). Likewise, when pregnant rats were exposed to different levels of RoundUp® in drinking water, their offspring showed reduced mobility (Gallegos et al. Citation2016). Moreover, in mice fed RoundUp® chronically or sub-chronically or exposed intranasally to Glifloglex®, there was diminished memory, in particular in terms of recognition memory (Baier et al. Citation2017; Ait Bali et al. Citation2019). In their study, Ait Bali et al. (Citation2019) also observed a reduction in mouse learning abilities and acetylcholine esterase activity in the total brain as well as the hippocampus, the latter being known for its central role in memory formation. Glutamate neurotransmission was also affected in the hippocampus of the offspring of RoundUp®-exposed rat dams (Cattani et al. Citation2014). A diminution in acetylcholine esterase activity was also observed in treated fish (Samanta et al. Citation2014). Therefore, it appears that locomotion and memory, as well as several associated neurotransmitter pathways, are impeded by GBHs. As the two cerebral activities are jointly impaired in Parkinson’s disease (characterized by loss of dopaminergic neurons), it may not be surprising that there is a growing body of case studies that suggest a causal effect between exposure to GBHs and onset of Parkinson’s disease (Barbosa et al. Citation2001; Kamel et al. Citation2007; Zheng et al. Citation2018; Eriguchi et al. Citation2019).
Digestive effects
The shikimate pathway targeted by glyphosate in plants also exists in microorganisms, the latter being exposed to GBHs in the gut microbiota through oral ingestion, accidental or not. In a model of cattle rumen exposed to different concentrations of glyphosate, there was a decrease in some microorganism phyla in favor of others, and the dysbiosis benefited pathogens (Ackermann et al. Citation2015). In particular, glyphosate inhibits Enterococcus spp., the bacteria capable of reducing the growth of the pathogen Clostridium botulinum in cattle rumen (Krüger et al. Citation2013). Another study demonstrated that dairy cows highly exposed to glyphosate showed changes in their rumen fungal composition compared to non-exposed cattle, revealing an effect of glyphosate on mycobiota (Schrödl et al. Citation2014). In other models such as mice, it was shown that oral exposure to RoundUp® was associated with a dysbiosis (Ait Bali et al. Citation2018). Specifically, in these hosts, there was a decreased ratio of firmicutes/bacteroides, the balance of which is essential for host physiology. Therefore, studies strongly indicate that GBHs disturb the microbiota equilibrium.
The impact of glyphosate and GBHs on the liver, the critical organ in xenobiotic detoxification, is of great interest. Pathologies, ranging from mild liver lesions up to fibrosis, were observed in rainbow trout exposed to increasing GBH concentrations (Topal et al. Citation2015). Likewise, in rats exposed orally to increasing doses of RoundUp®, Pandey et al. (Citation2019) observed mild-to-severe fibrosis that was characterized by collagen accumulation as well as increased liver weight, and erratic glycogen storage. The authors concluded that subacute exposure to RoundUp® might cause a nonalcoholic fatty liver condition. In a human hepatic progenitor cell line, glyphosate alone slightly altered the metabolome; this was accompanied by a reduction in long-chain and polyunsaturated fatty acid levels (Mesnage et al. Citation2018). Surprisingly, this decrease was significant only at the lowest glyphosate concentration tested, suggesting a concentration-independent effect.
Concerning other components of the digestive system, studies have shown no histological alterations in the intestines of weaned piglets exposed to RoundUp® in their diet (Qiu et al. Citation2020), nor a positive correlation between GBH exposure and diabetes in GBH applicators (Montgomery et al. Citation2008). Moreover, despite the presence of glyphosate in their urine, there was no evidence of kidney injuries caused by this compound in children (Trasande et al. Citation2020). Collectively, these studies reveal GBH-induced liver damage both at histological and cellular levels, but no evidence of effects on other digestive organs.
Cardiovascular effects
The effects of glyphosate and GBHs on the cardiovascular system are numerous. When mice were given glyphosate in their drinking water for 72 weeks, their hemoglobin concentration became slightly decreased – resulting in non-significant anemia (Wang et al. Citation2019). In these hosts, both red blood cell counts and hematocrit levels were also diminished. Also, isolated rat aortic rings exposed to 1% of glyphosate presented a slight vasorelaxation that corresponded to 20% of a standard response. When isolated rat heart atria were exposed to 1% of glyphosate, they could be stimulated like controls, but their spasmodic spontaneous contractions were inhibited by 65% (Chan et al. Citation2007). This outcome was also observed in human cases of acute poisoning; Zouaoui et al. (Citation2013) reported that 4 out of 10 poisoned subjects developed heart arrhythmias. Thus, in conclusion, glyphosate alone seems to affect the cardiovascular system; similar impacts might be expected with GBHs, at least in acute poisoning cases.
Carcinogenic effects
Glyphosate carcinogenicity remains debated. In mice exposed topically to glyphosate in a skin carcinogenicity test, George et al. (Citation2010) showed no tumor-initiating potential but rather a tumor-promoting effect. Human epidemiological studies also demonstrated a non-significant correlation between GBH exposure and melanoma, as well as leukemia and colon, rectum, bladder, and kidney cancers (de Roos et al. Citation2005) or acute myeloid lymphoma (Andreotti et al. Citation2018). However, a significant correlation was shown between glyphosate exposure and multiple myeloma (de Roos et al. Citation2005), and large B-cell lymphoma (Leon et al. Citation2019). The link between GBH exposure and non-Hodgkin’s lymphoma (NHL) is the most discussed. Hardell et al. (Citation2002) noted that NHL was the first form of cancer shown to be more represented in populations highly exposed to glyphosate. However, some studies denied this correlation (de Roos et al. Citation2005; Andreotti et al. Citation2018; Leon et al. Citation2019), but Zhang et al. (Citation2019) demonstrated that GBH exposure increases the probability to develop NHL by 41%.
Surprisingly, since glyphosate is an analog of glycine, an amino acid crucial for tumor cell development, another study showed that glyphosate and AMPA could inhibit the growth of some cancer cell lines. In particular, AMPA could inhibit the S phase of the cell cycle and alter the expression of genes involved in this process or apoptosis in cancer cell lines but not in normal cell lines. Although only two normal cell lines were tested, the authors suggested that targeting the metabolism of glycine with glyphosate or AMPA could be promising in the development of new anti-tumor drugs (Li et al. Citation2013). Besides this result and albeit no consensus has been reached on the matter, GBH exposure is nonetheless suspected to be a risk factor in the development of some cancers, probably through a tumor-promoting potential.
Effects on fish immune system
Complement
The complement cascade is one of the first defense against pathogens in fish, and glyphosate could alter this critical part of the innate immune system. In European flounder (Platichthys flesus) exposed to environmentally-realistic concentrations of RoundUp®, AMPA, and three other pesticides, e.g. methylchlorophenoxypropionic acid (mecoprop), acetochlor, and 2,4-dichlorophenol, an increase in expression of the precursor of C1 inhibitor, a key negative regulator of the complement system, was observed after 62 days of exposure. Of note, no increased expression of the C1 inhibitor precursor was seen following exposure to RoundUp® and AMPA alone; thus, the above-noted effect may be due to the other pesticides (mecoprop, acetochlor, and 2,4-dichlorophenol) (Evrard et al. Citation2010). Interestingly, Ma et al. (Citation2015) showed a decrease in expression of the C3 component, as well as substantial damage in the kidney (a primary immune organ in fish) in the common carp (Cyprinus carpio) after 3 and 7 days of subacute glyphosate exposure, suggesting that glyphosate could indeed down-regulate the complement pathway. Therefore, current studies point to a mild inhibitory effect of glyphosate and GBHs on the host complement cascade.
Phagocytosis
Kreutz et al. (Citation2010) studied interactions between sub-lethal glyphosate exposure and a bacterial Aeromonas hydrophila infection in silver catfish (Rhamdia quelen). These authors showed the catfish were more susceptible to infection after glyphosate exposure (i.e. had higher mortality) and also had a reduced number of phagocytes and diminished phagocytic potentials for the same number of phagocytes. After sublethal RoundUp® exposure for 7 days, common carp phagocyte production of reactive oxygen species was also reduced, as assessed by nitroblue tetrazolium test (Kondera et al. Citation2018). Moreover, common carp sublethally-exposed to glyphosate showed an increase in innate nonspecific opsonin lysozyme expression after 24 h followed by decreases at 72 and 168 h (Ma et al. Citation2015); this could partially explain the global impact on phagocytosis seen at long-term. Likewise, both carp and European sheatfish (Silurus glanis) exposed to RoundUp® showed decreases in phagocytic activity – sustained for 2 weeks; this phenotype was rescued by the addition of lysozyme (Terech-Majewska et al. Citation2004). Together, these results indicate that glyphosate and GBHs exposures can lead to reduced phagocytosis, possibly in part via a decrease in lysozyme expression in the hosts.
Cytokine production
Glyphosate and GBH effects on pro- or anti-inflammatory cytokine and chemokine production diverge depending on fish species, organs, or cells studied (). Discrepancies among those studies could also be due to differences in glyphosate formulations containing POEA or other surfactants and techniques used to assess cytokine expression. In general, with few exceptions, the expression of pro-inflammatory cytokines, such as tumor necrosis factor (TNF), interferon (IFN)-γ, interleukin (IL)-1β, and the activity of the NF-κB pathway, seemed enhanced by glyphosate exposure. At the same time, there were no clear exposure-related trends for the expression of anti-inflammatory cytokines (such as tumor growth factor [TGF]-β or IL-10) nor chemokines (such as LECT2 and CXCL8/IL-8). Nonetheless, while it is clear that exposures to these agents can impact on the balance between anti- and pro-inflammatory cytokines in a host, a firmer determination as to which direction is favored overall remains to be determined by ongoing investigations.
Table 1. Changes in cytokine production in fish with glyphosate and glyphosate-based herbicide exposure.
Lymphocyte responses
Glyphosate and GBHs may also impact on adaptive immune responses by inducing alterations in lymphocytes. Lymphocytes from Bolti fish (Tilapia nilotica) exposed to 1/1000 of field concentration of RoundUp® for up to 4 weeks proliferated ex vivo gradually less in response to lipopolysaccharide (LPS) mitogen (El-Gendy et al. Citation1998). Antibody production by these host cells was also affected, as assessed by plaque-forming assays. When common carp were exposed for 7 days to a glyphosate-POEA mixture, the mRNA levels of IgM (primary antibody type in fish; Secombes and Wang Citation2012) were lower as compared to in controls after 24 h; IgM protein contents were also lower at every timepoint, suggesting that antibody-mediated immunity became less efficient (Ma et al. Citation2015). In contrast, Kreutz et al. (Citation2014) noted an unexpected increase in the immunoglobulin concentrations in silver catfish exposed to glyphosate and then challenged with an inactivated form of Aeromonas hydrophila. While the studies above suggested lymphocyte responses were deteriorated by exposures to glyphosate or GBHs, the surprising results from the Kreutz group indicated that more studies are needed to apprehend further the full impact of these agents on fish lymphocyte function.
Effects on non-human mammal immune system
Genotoxicity and oxidative stress
The effects of glyphosate and GBHs on the genotoxicity and oxidative stress in non-immune cells suggest similar potential effects on immune cells. Lioi et al. (Citation1998) observed that bovine lymphocytes cultured with different glyphosate concentrations exhibit increasing dose-dependent chromosome aberrations and sister-chromatid exchanges. In another study on bovine lymphocytes cultured with increasing RoundUp® doses, there were increases in sister-chromatid exchanges but no significant changes in levels of chromosomal aberrations; it was also reported that there was an inhibition of mitotic activity at high RoundUp® doses and a delay in initiation of the cell cycle (Siviková and Dianovsky Citation2006). The use of RoundUp®, which was more cytotoxic than glyphosate alone, in conjunction with a cell cycle slowdown, could explain the differences between the conclusions of the above-noted studies.
In peripheral blood lymphocytes from the armadillo Chaetophractus villosus, exposure to RoundUp® induced more sister-chromatid exchanges and chromosomal aberrations (mostly chromatid breaks) (Luaces et al. Citation2017). Those authors interpreted these outcomes as a sign of alterations in chromosome replication; this was supported by an observation of a slight reduction in the replication index. Regarding oxidative stress, Lioi et al. (Citation1998) noted that bovine lymphocytes had significantly increased activity of the glucose 6-phosphate dehydrogenase (G6PDH; anti-oxidant enzyme), suggesting that oxidative stress was also induced in these cells. In conclusion, glyphosate and RoundUp® can impart some genotoxic and oxidative stress effects on mammalian lymphocytes.
Local inflammation and associated cytokine production
The inflammatory responses associated with glyphosate and GBHs that were seen in fish could most likely occur in non-human mammals. When rats were injected intraperitoneally with RoundUp® or glyphosate alone on alternative days for 1 or 2 weeks, their serum TNF levels increased (El-Shenawy Citation2009). In a study where glyphosate-rich air samples were collected in agricultural fields regularly sprayed with various GBHs and the filtered materials then injected intranasally into mice, eosinophil and neutrophil enrichment, as well as mast cell degranulation, was seen in the lungs (Kumar et al. Citation2014). This effect could be partly mediated through ERα and ERβ glyphosate-mimicked activation (Thongprakaisang et al. Citation2013) since these receptors are involved in respiratory inflammation in mice, in particular the eosinophil infiltration (Watanabe et al. Citation2019). In rats fed increasing concentrations of RoundUp®, Pandey et al. (Citation2019) noted an increase in levels of pro-inflammatory cytokines IL-1β, TNF, and IL-6, as well as C-reactive protein in the liver and adipose tissue. Likewise, in piglets exposed to different RoundUp® concentrations in their diet, Qiu et al. (Citation2020) showed an alteration of the jejunum and duodenum barrier that was associated with an increase in mRNA levels of IL-6 and NF-κB, whereas no differences in protein levels were observed for IL-6, IL-1β, or TNF in these organs. When taken together, these studies demonstrated that GBHs cause inflammation in mammals, in particular in the organs most directly impacted upon by route of exposure.
Lymphocyte responses
Lymphocyte responses seem altered by glyphosate and GBH exposure. For instance, in mice given glyphosate in their water for 72 weeks, Wang et al. (Citation2019) observed an increase in serum levels of IgG as well as in plasma cell populations that expand in the spleen and the bone marrow. However, in piglets fed a diet enriched in RoundUp® for 35 days, no significant differences were observed in duodenal and jejunal contents of secreted IgA, the main isotype secreted in mucous membranes (Qiu et al. Citation2020). Regarding T-lymphocytes, in mice exposed intranasally to glyphosate-rich air samples, a T-helper (TH) Type 2 response seemed to be activated, with increased production of the TH2-specific cytokines IL-33, IL-13, and IL-5 appearing in their lungs – even as systemic IL-4 concentrations remained unaffected (Kumar et al. Citation2014). It is worth noting that the IL-33 production could be increased by glyphosate via its ERα and ERβ activation, as suggested by studies from Watanabe et al. (Citation2018, Citation2019). Overall, the evidence suggests that GBH and glyphosate can reshape, to a certain extent, lymphocyte responses in mammalian hosts.
Interactions with pathogen infections
As a specific inhibitor of the shikimate metabolic pathway crucial for aromatic amino acid biosynthesis in plants and microorganisms, glyphosate has been studied in bacterial and fungal infections. In mice contaminated with Cryptococcus neoformans (a pathogenic fungus that causes meningoencephalitis – especially in AIDS patients), glyphosate injected intraperitoneally prolonged mouse survival and diminished colony-forming units in lung extracts. In vitro, glyphosate inhibited C. neoformans growth and production of melanin, a compound necessary for virulence (Nosanchuk et al. Citation2001). Likewise, in mice, intranasal spraying of glyphosate limited an infection by Klebsiella pneumoniae (commensal bacterium in the intestine, but pathogenic elsewhere) (Silver et al. Citation2019). Though aware of the hazards from the use of glyphosate as a treatment, the authors acknowledged that the agent could be a useful tool to test the therapeutic potentials of drugs that could target aromatic amino acid production.
Effects on human immune system
Genotoxicity
The genotoxicity of glyphosate, glyphosate metabolites, and GBHs on human lymphocytes and peripheral blood mononuclear cells (PBMCs) has been long investigated. Vigfusson and Vyse (Citation1980) first observed an increase in sister-chromatid exchanges in human lymphocytes cultured with RoundUp®. Regarding chromosomal aberrations in lymphocytes, AMPA caused an augmentation, but the effect of glyphosate was debatable since Mañas et al. (Citation2009a, Citation2009b) showed no impact while Santovito et al. (Citation2018) reported a dose-dependent increase. Santovito and colleagues also observed an amplification of micronuclei formation in lymphocytes cultured with low concentrations of glyphosate; Mladinic et al. (Citation2009a) achieved this result only with high doses. Moreover, lymphocytes cultured with increasing concentrations of glyphosate showed genotoxic damage in Comet assays and enhanced necrosis and apoptosis at high doses. However, no evidence for DNA base oxidation was found (Mladinic et al. Citation2009b). Still, in lymphocytes, non-cytotoxic concentrations of glyphosate, but not AMPA, caused double-strand breaks and phosphorylation of Ku80, a protein involved in the error-prone DNA repair by non-homologous end joining. Hence, the authors raised the concern that prolonged exposure could cause cumulative damage, potentially leading to leukemia or lymphoma (Suárez-Larios et al. Citation2017).
In PBMCs, RoundUp®, glyphosate, and AMPA induced single-strand breaks, and both RoundUp® and high doses of glyphosate caused double-strand breaks too, which are repaired after 2 h, except for the highest RoundUp® concentration (Kwiatkowska et al. Citation2017; Woźniak et al. Citation2018). Another study comparing genotoxicity in lymphocytes from populations exposed to GBH spraying showed a slightly higher frequency of binucleated cells with micronuclei (Bolognesi et al. Citation2009); as this effect decreased 4 months after the last GBH spraying, the authors concluded GBH caused low transient genotoxicity. Overall, while GBHs, glyphosate, and AMPA appear to pose a mild genotoxic hazard at environmentally-relevant concentrations, this still could be a long-term concern.
A decrease in global methylation was also observed in PBMCs cultured with glyphosate. Promoter methylation of tumor suppressor p53 and p21 increased and decreased, respectively, which translated in a decrease and increase in their expression (Kwiatkowska et al. Citation2017; Woźniak et al. Citation2020). The Woźniak study also described an augmentation of the proto-oncogene Bcl2 and the cyclin D CCDN1 expression, both of which correlated with a reduction of CCDN1 inhibitor p16 expression. All these occurred with no significant changes in methylation states. In conclusion, glyphosate seems to be capable of altering DNA methylation in PBMCs, thereby impacting on tumor suppressor gene, cell cycle gene, and proto-oncogene expression.
Energy metabolism and oxidative stress
As a growing number of studies have been using energy metabolism as a marker to assess the overall function of immune cells, the impact of glyphosate and GBHs on energy metabolism in these cells is of great relevance. In PBMCs, ATP levels were decreased by glyphosate, but less-so by AMPA and MPA. An impurity of GBH fabrication, phosphonomethyliminodiacetic acid (PMIDA), actually produced the most considerable effect on PBMC ATP levels (Kwiatkowska et al. Citation2017). Moreover, PBMC production of reactive oxygen species (especially radical hydroxyl) was enhanced by glyphosate and RoundUp®, with a more substantial effect from the latter, while AMPA had no impact (Woźniak et al. Citation2018). Hence, exposure to these agents clearly can impact on energy metabolism by human PBMC.
Interaction between the immune system and microorganisms
Recent studies suggest that glyphosate and GBHs interact with microorganisms and affect their interaction with the immune system. Mendler et al. (Citation2020) studied the relationship between mucosal-associated invariant T-cells (MAIT) and gut bacteria Escherichia coli and Lactobacillus reuteri, which respectively induce and inhibit MAIT TNF and IFNγ production to control gut microbiota tolerance. Using a model with bacteria pretreated with glyphosate and subsequently cultured with MAIT, the authors showed that treated E. coli still induced the same MAIT TNF and IFNγ responses whereas treated L. reuteris increased MAIT production of TNF but not IFNγ. Hence, in this model, glyphosate reduced microbiota tolerance. A case report study described a Clostridium tertium infection after GBH ingestion for suicidal purposes in a 44-year-old woman (You et al. Citation2015). The authors suspected that the intestinal mucosa deterioration seen in this woman was caused by the herbicide that had allowed the bacterial infection to evolve. In conclusion, glyphosate seems to be able to disrupt interactions between bacteria and the immune system, at least at the gut interface. These changes might lead to lower microbiota tolerance or even infection development.
Inflammatory diseases
Some studies have reported a link between exposure to glyphosate and GBHs and the development of inflammatory diseases. For instance, de Raadt et al. (Citation2015) described the case of a woman affected twice by acute eosinophilic pneumonia, each time a couple of weeks after an acute RoundUp® exposure, within a 4-year gap. Although the woman was a smoker, the authors suspect the herbicide was a causative agent for the disease. In epidemiological studies, Hoppin et al. (Citation2008, Citation2017) found a correlation between GBH exposure and the development of allergic and non-allergic wheeze in male farmers, as well as atopic asthma in farm women. Those analyses suggested then that chronic GBH inhalation could promote various types of airway inflammation in the long-term. Moreover, a review reported that an accumulation of GBHs (more specifically RoundUp®) as a result of continual unintended ingestion might present a potential risk for the development of endometriosis since oxidative stress and estrogen pathway disruptions are each involved in this chronic inflammatory disease (Aris and Paris Citation2010). From the results presented above, it would seem that GBH exposure could play a part in the development of inflammatory syndromes, whether chronic or acute, in humans.
Conclusions
A comprehensive review of the current literature provides strong evidence of the impact of glyphosate and GBHs on fish and mammals. In particular, these agents impart dose-dependent cytotoxic and genotoxic effects, increase oxidative stress, disrupt the estrogen pathway, impair some cerebral functions, and are allegedly correlated to the development of some cancers (). Regarding the immune system, common outcomes among tested species appear to be increased inflammatory states. These are demonstrable as induction of enhanced pro-inflammatory cytokine production in fish, local organ inflammation in rodents, or acute or chronic inflammatory syndromes in humans ().
Figure 2. Impacts of glyphosate and glyphosate-based herbicides on animal health. Individual circles summarize the reported cellular, carcinogenic, reproductive, cardiovascular, cerebral and digestive effects of glyphosate and glyphosate-based herbicides on fish and mammal health.
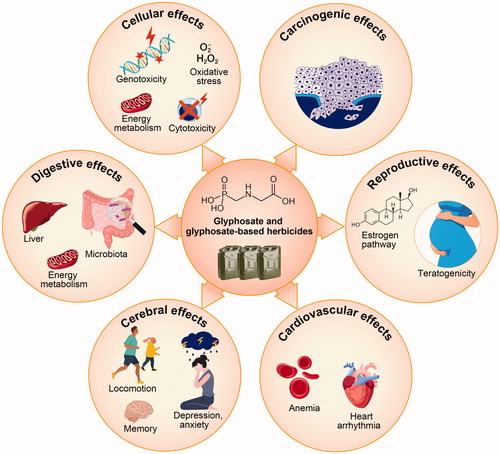
Figure 3. Impacts of glyphosate and glyphosate-based herbicides on fish and mammal immune system. Venn diagram summarizing the reported effects of glyphosate and glyphosate-based herbicides on the immune system of humans, non-human mammals and fishes, respectively represented in pink, green and blue circles. Arrows indicate the direction of the alteration: ↑ indicates a tendency in favor of an increase in the phenomenon, ↓ indicates a tendency in favor of a decrease in the phenomenon, ⇅ indicates no clear tendency, with some reports of an increase and some reports of a decrease in this phenomenon.
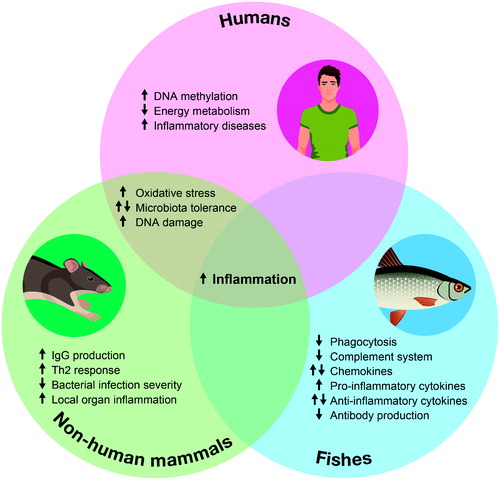
Despite this evidence, regulation of the use of glyphosate and GBHs can be seen as still rather permissive, especially in countries that renewed use authorizations for several additional years. Clearly, with growing populations to feed, one must take into consideration the increasing need for herbicides to explain the reluctance to ban glyphosate.
Since glyphosate is ubiquitous and an understanding of its hazards is crucial, the paucity of studies, in particular effects on the human immune system, is notable. Depending on the specific endpoint, there are, on the one hand, scattered studies that are hard to compare with one another; on the other hand, there are several studies on the same topic but whose divergent outcomes result in the absence of consensus. The difficulties in performing relevant experiments with human samples, as well as the ‘Monsanto Papers’ scandal, could explain to a certain extent the limited results so far. There is a clear need for more studies, especially on the effects of GBHs, since glyphosate is rarely used alone. Such studies could help standardize glyphosate regulations among organizations (that differ from one country to another for now), and settle once and for all the matter of glyphosate toxicity status among regulatory agencies.
Acknowledgments
The authors would like to thank Philippe A. Tessier (CRCHU de Québec-Université Laval) and Ingrid Saba for critical review of the manuscript, as well as France Couture (CRCHU de Québec-Université Laval) for the generation of the infographic images.
Disclosure statement
The authors declare no conflict of interest. The authors alone are responsible for the content of this manuscript.
Correction Statement
This article has been corrected with minor changes. These changes do not impact the academic content of the article.
Additional information
Funding
References
- Ackermann W, Coenen M, Schrödl W, Shehata A, Krüger M. 2015. The influence of glyphosate on the microbiota and production of botulinum neurotoxin during ruminal fermentation. Curr Microbiol. 70(3):374–382.
- Acquavella J, Alexander B, Mandel J, Gustin C, Baker B, Chapman P, Bleeke M. 2004. Glyphosate biomonitoring for farmers and their families: Results from the Farm Family Exposure Study. Environ Health Perspect. 112(3):321–326.
- Ait Bali Y, Ba-M’hamed S, Elhidar N, Nafis A, Soraa N, Bennis M. 2018. Glyphosate-based herbicide exposure affects gut microbiota, anxiety and depression-like behaviors in mice. Neurotoxicol Teratol. 67:44–49.
- Ait Bali Y, Ba-Mhamed S, Bennis M. 2017. Behavioral and immunohistochemical study of the effects of subchronic and chronic exposure to glyphosate in mice. Front Behav Neurosci. 11:146.
- Ait Bali Y, Kaikai N-E, Ba-M’hamed S, Bennis M. 2019. Learning and memory impairments associated to acetylcholinesterase inhibition and oxidative stress following glyphosate based-herbicide exposure in mice. Toxicology. 415:18–25.
- Alferness P, Iwata Y. 2002. Determination of glyphosate and (aminomethyl)phosphonic acid in soil, plant and animal matrixes, and water by capillary gas chromatography with mass-selective detection. Am Chem Soc. 42:2751–2759.
- Alvarez-Moya C, Silva M, Ramírez C, Gallardo D, Sánchez R, Aguirre A, Velasco A. 2014. Comparison of the in vivo and in vitro genotoxicity of glyphosate isopropylamine salt in three different organisms. Genet Mol Biol. 37(1):105–110.
- Andreotti G, Koutros S, Hofmann JN, Sandler DP, Lubin JH, Lynch CF, Lerro CC, De Roos AJ, Parks CG, Alavanja MC, et al. 2018. Glyphosate use and cancer incidence in the Agricultural Health Study. J Natl Cancer Inst. 110(5):509–516.
- Arbuckle T, Lin Z, Mery L. 2001. An exploratory analysis of the effect of pesticide exposure on the risk of spontaneous abortion in an Ontario farm population. Environ Health Perspect. 109(8):851–857.
- Aris A, Paris K. 2010. Hypothetical link between endometriosis and xenobiotics-associated genetically modified food. Gynecol Obstet Fertil. 38(12):747–753.
- Baier C, Gallegos C, Raisman-Vozari R, Minetti A. 2017. Behavioral impairments following repeated intranasal glyphosate-based herbicide administration in mice. Neurotoxicol Teratol. 64:63–72.
- Barbosa E, Leiros da Costa M, Bacheschi L, Scaff M, Leite C. 2001. Parkinsonism after glycine-derivate exposure. Mov Disord. 16(3):565–568.
- Benachour N, Séralini G. 2009. Glyphosate formulations induce apoptosis and necrosis in human umbilical, embryonic, and placental cells. Chem Res Toxicol. 22(1):97–105.
- Benachour N, Sipahutar H, Moslemi S, Gasnier C, Travert C, Séralini G. 2007. Time- and dose-dependent effects of Roundup on human embryonic and placental cells. Arch Environ Contam Toxicol. 53(1):126–133.
- Benbrook C. 2016. Trends in glyphosate herbicide use in the United States and globally. Environ Sci Eur. 28(1):3.
- Bolognesi C, Carrasquilla G, Volpi S, Solomon K, Marshall E. 2009. Biomonitoring of genotoxic risk in agricultural workers from five Colombian Regions: Association to occupational exposure to glyphosate. J Toxicol Environ Health. 72(15–16):986–997.
- Burchfield S, Bailey D, Todt C, Denney R, Negga R, Fitsanakis V. 2019. Acute exposure to a glyphosate-containing herbicide formulation inhibits Complex II and increases hydrogen peroxide in model organism Caenorhabditis elegans. Environ Toxicol Pharmacol. 66:36–42.
- Caloni F, Cortinovis C, Rivolta M, Davanzo F. 2016. Suspected poisoning of domestic animals by pesticides. Sci Total Environ. 539:331–336.
- Campaña H, Pawluk M, López Camelo J, Grupo de Estudio del E. 2010. Births prevalence of 27 selected congenital anomalies in seven geographic regions of Argentina. Arch Argent Pediatr. 108:409–417.
- Cattani D, Cesconetto PA, Tavares MK, Parisotto EB, De Oliveira PA, Rieg CEH, Leite MC, Prediger RDS, Wendt NC, Razzera G, et al. 2017. Developmental exposure to glyphosate-based herbicide and depressive-like behavior in adult offspring: Implication of glutamate excitotoxicity and oxidative stress. Toxicology. 387:67–80.
- Cattani D, de Liz Oliveira Cavalli V, Heinz Rieg C, Domingues J, Dal-Cim T, Tasca C, Mena Barreto Silva F, Zamoner A. 2014. Mechanisms underlying the neurotoxicity induced by glyphosate-based herbicide in immature rat hippocampus: Involvement of glutamate excitotoxicity. Toxicology. 320:34–45.
- Chan Y, Chang S, Hsuan S, Chien M, Lee W, Kang J, Wang S, Liao J. 2007. Cardiovascular effects of herbicides and formulated adjuvants on isolated rat aorta and heart. Toxicol in Vitro. 21(4):595–603.
- Connolly A, Coggins M, Galea K, Jones K, Kenny L, McGowan P, Basinas I. 2019. Evaluating glyphosate exposure routes and their contribution to total body burden: A study among amenity horticulturalists. Ann Work Expo Health. 63(2):133–147.
- Dallegrave E, Mantese F, Coelho R, Pereira J, Dalsenter P, Langeloh A. 2003. The teratogenic potential of the herbicide glyphosate-Roundup in Wistar rats. Toxicol Lett. 142(1–2):45–52.
- de Raadt W, Wijnen P, Bast A, Bekers O, Drent M. 2015. Acute eosinophilic pneumonia associated with glyphosate-surfactant exposure. Sarcoidosis Vasculitis Diffuse Lung Dis. 32:172–175.
- de Roos A, Blair A, Rusiecki J, Hoppin J, Svec M, Dosemeci M, Dale P, Sandler D, Alavanja M. 2005. Cancer incidence among glyphosate-exposed pesticide applicators in the Agricultural Health Study. Environ Health Perspect. 113(1):49–54.
- El-Gendy K, Aly N, El-Sebae A. 1998. Effects of edifenphos and glyphosate on the immune response and protein biosynthesis of bolti fish (Tilapia nilotica). J Environ Sci Health B. 33(2):135–149.
- El-Gendy K, Mosallam E, Ahmed N, Aly N. 2018. Determination of glyphosate residues in Egyptian soil samples. Anal Biochem. 557:1–6.
- El-Shenawy N. 2009. Oxidative stress responses of rats exposed to Roundup and its active ingredient glyphosate. Environ Toxicol Pharmacol. 28(3):379–385.
- Eriguchi M, Iida K, Ikeda S, Osoegawa M, Nishioka K, Hattori N, Hiroshi Nagayama H, Hara H. 2019. Parkinsonism relating to intoxication with glyphosate. Intern Med. 58(13):1935–1938.
- Evrard E, Marchand J, Theron M, Pichavant-Rafini K, Durand G, Quiniou L, Laroche J. 2010. Impacts of mixtures of herbicides on molecular and physiological responses of the European flounder Platichthys flesus. Comp Biochem Physiol C Toxicol Pharmacol. 152(3):321–331.
- Fagan J, Antoniou M, Habib M, Howard C, Jennings R, Leifert C, Nodari R, Fagan J, Robinson C. 2012. Teratogenic effects of glyphosate-based herbicides: Divergence of regulatory decisions from scientific evidence. J Environ Anal Toxicol. S4:006.
- Ford B, Bateman L, Gutierrez-Palominos L, Park R, Nomura D. 2017. Mapping proteome-wide targets of glyphosate in mice. Cell Chem Biol. 24(2):133–140.
- Gallegos C, Bartos M, Bras C, Gumilar F, Antonelli M, Minetti A. 2016. Exposure to a glyphosate-based herbicide during pregnancy and lactation induces neurobehavioral alterations in rat offspring. Neurotoxicology. 53:20–28.
- George J, Prasad S, Mahmood Z, Shukla Y. 2010. Studies on glyphosate-induced carcinogenicity in mouse skin: A proteomic approach. J Proteomics. 73(5):951–964.
- Gillezeau C, van Gerwen M, Shaffer R, Rana I, Zhang L, Sheppard L, Taioli E. 2019. The evidence of human exposure to glyphosate: A review. Environ Health. 18(1):2.
- Hardell L, Eriksson M, Nordstrom M. 2002. Exposure to pesticides as risk factor for non-Hodgkin’s lymphoma and hairy cell leukemia: Pooled analysis of two Swedish case-control studies. Leuk Lymphoma. 43(5):1043–1049.
- Hoppin J, Umbach D, London S, Henneberger P, Kullman G, Alavanja M, Sandler D. 2008. Pesticides and atopic and nonatopic asthma among farm women in the Agricultural Health Study. Am J Respir Crit Care Med. 177(1):11–18.
- Hoppin J, Umbach D, Long S, London S, Henneberger P, Blair A, Alavanja M, Freeman L, Sandler D. 2017. Pesticides are associated with allergic and non-allergic wheeze among male farmers. Environ. Health Perspect. 125(4):535–543.
- Kamel F, Tanner C, Umbach D, Hoppin J, Alavanja M, Blair A, Comyns K, Goldman S, Korell M, Langston J, et al. 2007. Pesticide exposure and self-reported Parkinson’s disease in the agricultural health study. Am J Epidemiol. 165(4):364–374.
- Kawagashira Y, Koike H, Kawabata K, Takahashi M, Ohyama K, Hashimoto R, Iijima M, Katsuno M, Sobue G. 2017. Vasculitic neuropathy following exposure to a glyphosate-based herbicide. Intern Med. 56(11):1431–1434.
- Kim Y, Hong J, Gil H, Song H, Hong S. 2013. Mixtures of glyphosate and surfactant TN20 accelerate cell death via mitochondrial damage-induced apoptosis and necrosis. Toxicol in Vitro. 27(1):191–197.
- Kondera E, Teodorczuk B, Ługowska K, Witeska M. 2018. Effect of glyphosate-based herbicide on hematological and hemopoietic parameters in common carp (Cyprinus Carpio L). Fish Physiol Biochem. 44(3):1011–1018.
- Kreutz L, Barcellos L, Marteninghe A, Dos Santos E, Zanatta R. 2010. Exposure to Sublethal Concentration of glyphosate or atrazine-based herbicides alters the phagocytic function and increases the susceptibility of silver catfish fingerlings (Rhamdia quelen) to Aeromonas hydrophila challenge. Fish Shellfish Immunol. 29(4):694–697.
- Kreutz L, Pavan T, Alves A, Correia A, Barriquel B, Dos Santos E, Barcellos L. 2014. Increased immunoglobulin production in silver catfish (Rhamdia quelen) exposed to o agrichemicals. Braz J Med Biol Res. 47(6):499–504.
- Krüger M, Shehata A, Schrödl W, Rodloff A. 2013. Glyphosate suppresses the antagonistic effect of Enterococcus Spp. on Clostridium botulinum. Anaerobe. 20:74–78.
- Kumar S, Khodoun M, Kettleson E, McKnight C, Reponen T, Grinshpun S, Adhikari A. 2014. Glyphosate-rich air samples induce IL-33, TSLP and generate IL-13 dependent airway inflammation. Toxicology. 325:42–51.
- Kwiatkowska M, Reszka E, Woźniak K, Jabłońska E, Michałowicz J, Bukowska B. 2017. DNA damage and methylation induced by glyphosate in human peripheral blood mononuclear cells (in vitro study). Food Chem Toxicol. 105:93–98.
- Leon ME, Schinasi LH, Lebailly P, Beane Freeman LE, Nordby K-C, Ferro G, Monnereau A, Brouwer M, Tual S, Baldi I, et al. 2019. Pesticide use and risk of Non-Hodgkin lymphoid malignancies in agricultural cohorts from France, Norway and the USA: A pooled analysis from the AGRICOH consortium . Int J Epidemiol. 48(5):1519–1535.
- Li Q, Lambrechts M, Zhang Q, Liu S, Ge D, Yin R, Xi M, You Z. 2013. Glyphosate and AMPA inhibit cancer cell growth through inhibiting intracellular glycine synthesis. Drug Des Devel Ther. 7:635–643.
- Lioi M, Scarfì M, Santoro A, Barbieri R, Zeni O, Di Berardino D, Ursini M. 1998. Genotoxicity and oxidative stress induced by pesticide exposure in bovine lymphocyte cultures in vitro. Mutat Res. 403(1–2):13–20.
- London L, Flisher A, Wesseling C, Mergler D, Kromhout H. 2005. Suicide and exposure to organophosphate insecticides: Cause or effect? Am J Ind Med. 47(4):308–321.
- Luaces J, Rossi L, Chirino M, Browne M, Merani M, Mudry M. 2017. Genotoxic effects of Roundup Full II® on lymphocytes of Chaetophractus villosus (Xenarthra, Mammalia): In vitro studies. PLoS One. 12(8):e0182911.
- Lushchak O, Kubrak O, Storey J, Storey K, Lushchak V. 2009. Low toxic herbicide Roundup induces mild oxidative stress in goldfish tissues. Chemosphere. 76(7):932–937.
- Ma J, Bu Y, Li X. 2015. Immunological and histopathological responses of the kidney of common carp (Cyprinus carpio L.) sublethally exposed to glyphosate . Environ Toxicol Pharmacol. 39(1):1–8.
- Ma J, Li X. 2015. Alteration in the cytokine levels and histopathological damage in common carp induced by glyphosate. Chemosphere. 128:293–298.
- Ma J, Zhu J, Wang W, Ruan P, Rajeshkumar S, Li X. 2019. Biochemical and molecular impacts of glyphosate-based herbicide on the gills of common carp. Environ Pollut. 252(Pt B):1288–1300.
- Mañas F, Peralta L, Raviolo J, García Ovando H, Weyers A, Ugnia L, Gonzalez Cid M, Larripa I, Gorla N. 2009a. Genotoxicity of AMPA, environmental metabolite of glyphosate, assessed by Comet Assay and cytogenetic tests. Ecotoxicol Environ Saf. 72(3):834–837.
- Mañas F, Peralta L, Raviolo J, Ovando H, Weyers A, Ugnia L, Cid M, Larripa I, Gorla N. 2009b. Genotoxicity of glyphosate assessed by Comet Assay and cytogenetic tests. Environ. Toxicol. Pharmacol. 28(1):37–41.
- Martinez T, Long W, Hiller R. 1990. Comparison of the toxicology of the herbicide Roundup by oral and pulmonary routes of exposure. Proc West Pharmacol Soc. 33:193–197.
- McHenry L. 2018. The Monsanto Papers: Poisoning the scientific well. Int J Risk Saf Med. 29(3–4):193–205.
- Mendler A, Geier F, Haange S, Pierzchalski A, Krause J, Nijenhuis I, Froment J, Jehmlich N, Berger U, Ackermann G, et al. 2020. Mucosal-associated invariant T-Cell (MAIT) activation is altered by chlorpyrifos- and glyphosate-treated commensal gut bacteria. J Immunotoxicol. 17(1):10–20.
- Mesnage R, Biserni M, Wozniak E, Xenakis T, Mein C, Antoniou M. 2018. Comparison of transcriptome responses to glyphosate, isoxaflutole, quizalofop-p-ethyl and mesotrione in the HepaRG cell line. Toxicol Rep. 5:819–826.
- Mesnage R, Phedonos A, Biserni M, Arno M, Balu S, Corton J, Ugarte R, Antoniou M. 2017. Evaluation of estrogen receptor alpha activation by glyphosate-based herbicide constituents. Food Chem Toxicol. 108(Pt A):30–42.
- Mladinic M, Berend S, Vrdoljak A, Kopjar N, Radic B, Zeljezic D. 2009b. Evaluation of genome damage and its relation to oxidative stress induced by glyphosate in human lymphocytes in vitro. Environ Mol Mutagen. 50(9):800–807.
- Mladinic M, Perkovic P, Zeljezic D. 2009a. Characterization of chromatin instabilities induced by glyphosate, terbuthylazine and carbofuran using Cytome FISH assay. Toxicol Lett. 189(2):130–137.
- Monroy C, Cortés A, Sicard D, de Restrepo H. 2005. Cytotoxicity and genotoxicity of human cells exposed in vitro to glyphosate. Biomedica. 25(3):335–345.
- Montgomery M, Kamel F, Saldana T, Alavanja M, Sandler D. 2008. Incident diabetes and pesticide exposure among licensed pesticide applicators: Agricultural Health Study, 1993–2003. Am J Epidemiol. 167(10):1235–1246.
- Nosanchuk J, Ovalle R, Casadevall A. 2001. Glyphosate inhibits melanization of Cryptococcus neoformans and prolongs survival of mice after systemic infection. J Infect Dis. 183(7):1093–1099.
- Pandey A, Dhabade P, Kumarasamy A. 2019. Inflammatory effects of subacute exposure of Roundup in rat liver and adipose tissue. Dose Response. 17:1559.
- Peixoto F. 2005. Comparative effects of the roundup and glyphosate on mitochondrial oxidative phosphorylation. Chemosphere. 61(8):1115–1122.
- Qiu S, Fu H, Zhou R, Yang Z, Bai G, Shi B. 2020. Toxic effects of glyphosate on intestinal morphology, anti-oxidant capacity and barrier function in weaned piglets. Ecotoxicol Environ Safety. 187:109846.
- Richard S, Moslemi S, Sipahutar H, Benachour N, Seralini G. 2005. Differential effects of glyphosate and Roundup on human placental cells and aromatase. Environ Health Perspect. 113(6):716–720.
- Richard S, Prévot-D’Alvise N, Bunet R, Simide R, Couvray S, Coupé S, Grillasca J. 2014. Effect of a glyphosate-based herbicide on gene expressions of the cytokines IL-1β and IL-10 and of heme oxygenase-1 in European sea bass. Bull Environ Contam Toxicol. 92(3):294–299.
- Romano M, Romano R, Santos L, Wisniewski P, Campos D, de Souza P, Viau P, Bernardi M, Nunes M, de Oliveira C. 2012. Glyphosate impairs male offspring reproductive development by disrupting gonadotropin expression. Arch Toxicol. 86(4):663–673.
- Roy N, Ochs J, Zambrzycka E, Anderson A. 2016. Glyphosate induces cardiovascular toxicity in Danio rerio. Environ Toxicol Pharmacol. 46:292–300.
- Samanta P, Pal S, Mukherjee A, Ghosh A. 2014. Biochemical effects of glyphosate based herbicide, Excel Mera 71 on enzyme activities of acetylcholinesterase (AChE), lipid peroxidation (LPO), catalase (CAT), glutathione-S-transferase (GST) and protein content on teleostean fishes. Ecotoxicol Environ Saf. 107:120–125.
- Santovito A, Ruberto S, Gendusa C, Cervella P. 2018. In vitro evaluation of genomic damage induced by glyphosate on human lymphocytes. Environ Sci Pollut Res Int. 25(34):34693–34700.
- Sato C, Kamijo Y, Yoshimura K, Ide T. 2011. Aseptic meningitis in association with glyphosate-surfactant herbicide poisoning. Clin Toxicol (Phila). 49(2):118–120.
- Schrödl W, Krüger S, Konstantinova-Müller T, Shehata A, Rulff R, Krüger M. 2014. Possible effects of glyphosate on Mucorales abundance in the rumen of dairy cows in Germany. Curr Microbiol. 69(6):817–823.
- Secombes C, Wang T, Eds. 2012. The innate and adaptive immune system of fish. In: Infectious disease in aquaculture: Prevention and control. Cambridge (UK): Woodhead Publications Ltd; p. 3–68.
- Silver R, Paczosa M, McCabe A, Balada-Llasat J, Baleja J, Mecsas J. 2019. Amino acid biosynthetic pathways are required for Klebsiella pneumoniae growth in immunocompromised lungs and are druggable targets during infection. Antimicrob Agents Chemother. 63:e02674.
- Siviková K, Dianovsky J. 2006. Cytogenetic effect of technical glyphosate on cultivated bovine peripheral lymphocytes. Intl J Hyg Environ Health. 209:15–20.
- Steinrücken H, Amrhein N. 1984. 5-Enolpyruvylshikimate-3-phosphate synthase of Klebsiella pneumoniae 2. Inhibition by glyphosate [N-(phosphonomethyl)glycine]. Eur J Biochem. 143(2):351–357.
- Suárez-Larios K, Salazar-Martínez A, Montero-Montoya R. 2017. Screening of pesticides with the potential of inducing DSB and successive recombinational repair. J Toxicol. 2017:3574840.
- Terech-Majewska E, Siwicki A, Szweda W. 2004. Modulative influence of lysozyme dimer on defense mechanisms in the carp (Cyprinus carpio) and European sheatfish (Silurus glanis) after suppression induced by herbicide roundup. Polish J Vet Sci. 7:123–128.
- Thongprakaisang S, Thiantanawat A, Rangkadilok N, Suriyo T, Satayavivad J. 2013. Glyphosate induces human breast cancer cells growth via estrogen receptors. Food Chem Toxicol. 59:129–136.
- Topal A, Atamanalp M, Uçar A, Oruç E, Kocaman EM, Sulukan E, Akdemir F, Beydemir Ş, Kılınç N, Erdoğan O, et al. 2015. Effects of glyphosate on juvenile rainbow trout (Oncorhynchus mykiss): Transcriptional and enzymatic analyses of anti-oxidant defense system, histopathological liver damage and swimming performance. Ecotoxicol Environ Safety. 111:206–214.
- Trasande L, Aldana SI, Trachtman H, Kannan K, Morrison D, Christakis DA, Whitlock K, Messito MJ, Gross RS, Karthikraj R, et al. 2020. Glyphosate exposures and kidney injury biomarkers in infants and young children. Environ Pollut. 256:113334.
- Tsatsakis A, Docea AO, Constantin C, Calina D, Zlatian O, Nikolouzakis TK, Stivaktakis PD, Kalogeraki A, Liesivuori J, Tzanakakis G, et al. 2019. Genotoxic, cytotoxic, and cytopathological effects in rats exposed for 18 months to a mixture of 13 chemicals in doses below NOAEL levels. Toxicol Lett. 316:154–170.
- Vandenberg LN, Blumberg B, Antoniou MN, Benbrook CM, Carroll L, Colborn T, Everett LG, Hansen M, Landrigan PJ, Lanphear BP, et al. 2017. Is it time to reassess current safety standards for glyphosate-based herbicides? J Epidemiol Community Health. 71(6):613–618.
- Vanlaeys A, Dubuisson F, Seralini G, Travert C. 2018. Formulants of glyphosate-based herbicides have more deleterious impact than glyphosate on TM4 sertoli cells. Toxicol in Vitro. 52:14–22.
- Vigfusson N, Vyse E. 1980. The effect of the pesticides, dexon, captan and Roundup, on sister-chromatid exchanges in human lymphocytes in vitro. Mutat Res. 79(1):53–57.
- Wang W, Qingqing L, Tianyi Z, Lu C, Shu L, Shiwen X. 2020. Glyphosate induces lymphocyte cell dysfunction and apoptosis via regulation of miR-203 targeting of PIK3R1 in common carp (Cyprinus carpio L.). Fish Shellfish Immunol. 101:51–57.
- Wang L, Qipan D, Hui H, Ming L, Zhaojian G, Shanshan Z, Zijun Y. 2019. Glyphosate induces benign monoclonal gammopathy and promotes multiple myeloma progression in mice. J Hematol Oncol. 12(1):70.
- Watanabe Y, Makino E, Tajiki-Nishino R, Koyama A, Tajima H, Ishimota M, Fukuyama T. 2018. Involvement of estrogen receptor α in pro-pruritic and pro-inflammatory responses in a mouse model of allergic dermatitis. Toxicol Appl Pharmacol. 355:226–237.
- Watanabe Y, Tajiki-Nishino R, Tajima H, Fukuyama T. 2019. Role of estrogen receptors α and β in the development of allergic airway inflammation in mice: A possible involvement of interleukin 33 and eosinophils. Toxicology. 411:93–100.
- Williams G, Kroes R, Munro I. 2000. Safety evaluation and risk assessment of the herbicide Roundup and its active ingredient, glyphosate, for humans. Regul Toxicol Pharmacol. 31(2 Pt 1):117–165.
- Woźniak E, Reszka E, Jabłońska E, Balcerczyk A, Broncel M, Bukowska B. 2020. Glyphosate affects methylation in the promoter regions of selected tumor suppressors as well as expression of major cell cycle and apoptosis drivers in PBMCs (in vitro study). Toxicol in Vitro. 63:104736.
- Woźniak E, Sicińska P, Michałowicz J, Woźniak K, Reszka E, Huras B, Zakrzewski J, Bukowska B. 2018. The mechanism of DNA damage induced by Roundup 360 PLUS, glyphosate and AMPA in human peripheral blood mononuclear cells – genotoxic risk assessment. Food Chem Toxicol. 120:510–522.
- You M, Shin G, Seop Lee C. 2015. Clostridium tertium bacteremia in a patient with glyphosate ingestion. Am J Case Rep. 16:4–7.
- Young F, Dao H, Danielle G, Edwards V. 2015. Integrative pharmacology, toxicology and genotoxicology. Integrat Pharmacol Toxicl Genotoxicol. 1:70–76.
- Zhang L, Rana I, Shaffer R, Taioli E, Sheppard L. 2019. Exposure to glyphosate-based herbicides and risk for Non-Hodgkin Lymphoma: A meta-analysis and supporting evidence. Mutat Res. 781:186–206.
- Zheng Q, Yin J, Zhu L, Jiao L, Xu Z. 2018. Reversible Parkinsonism induced by acute exposure glyphosate. Parkinsonism Relat Disord. 50:121.
- Zouaoui K, Dulaurent S, Gaulier J, Moesch C, Lachâtre G. 2013. Determination of glyphosate and AMPA in blood and urine from humans: About 13 cases of acute intoxication. Forensic Sci Int. 226(1–3):e20–e25.