Abstract
Novel immunotherapies for cancer and other diseases aim to trigger the immune system to produce durable responses, while overcoming the immunosuppression that may contribute to disease severity, and in parallel considering immunosafety aspects. Interleukin-2 (IL-2) was one of the first cytokines that the FDA approved as a cancer-targeting immunotherapy. However, in the past years, IL-2 immunotherapy is not actively offered to patients, due to limited efficacy, when compared to other novel immunotherapies, and the associated severe adverse events. In order to design improved in vitro and in vivo models, able to predict the efficacy and safety of novel IL-2 alternatives, it is important to delineate the mechanistic immunological events triggered by IL-2. Particularly, in this review we will discuss the effects IL-2 has with the bridging cell type of the innate and adaptive immune responses, dendritic cells. The pathways involved in the regulation of IL-2 by dendritic cells and T-cells in cancer and autoimmune disease will also be explored.
Introduction
Around 40 years ago, recombinant human Interleukin-2 (IL-2) was first used for treating a patient with metastatic melanoma, and its T-cell stimulatory capacities led to complete elimination of the cancer for some patients (Rosenberg et al. Citation1985). These findings paved the way to an FDA approval for the use of high-dose IL-2, called aldesleukin and marketed as Proleukin®, in metastatic renal cell cancer and metastatic melanoma patients in 1992 and 1998 respectively (Rosenberg Citation2021).
The use of high dose recombinant human IL-2 was a promising candidate for cancer immunotherapy, however the associated toxicities led to the cease of the treatment in the clinic. The IL-2-mediated adverse events observed were associated with toxicities in the heart, skin, gastrointestinal tract, lungs, endocrine system, blood, kidney and others, and were all related to the route of administration and dose (Siegel and Puri Citation1991). Capillary leak syndrome has been one of the most challenging associated toxicities, as it leads to medical complications (eg. subsequent lung and liver dysfunction) and was linked to administration of a high dose aldesleukin. Even to date, it poses a limiting factor for the development of safe novel IL-2 therapeutics. Experimental studies and medical practices have led to the mitigation and relief from some of these toxicities in the clinic, however the pathophysiological mechanisms behind these are poorly understood. Understanding the processes leading to the manifestation of side effects is important; a potential fine-tuning of dosage and administration route might augment the efficacy and improve safety of new IL-2 treatments. It is crucial though, to tweak at the appropriate dose IL-2, as a too high dose will activate T-effector- and natural killer (NK)- cells with a simultaneous generation of toxicities, however a too low dose may favor T-regulatory cell bias with a concomitant triggering of other immune cells that may additionally lead to a cytokine secretion imbalance (Kehrl et al. Citation1988; Fontenot et al. Citation2005; Barron et al. Citation2010).
IL-2 is a pleiotropic cytokine, originally discovered as a T lymphocyte growth factor (Smith Citation1988). Effector T-cells are the main producers of IL-2, which confers proliferative and cytotoxic properties, along with aiding in the development of memory T-cells and overall T-cell homeostasis (Nelson Citation2004; Ross and Cantrell Citation2018). However more immune cell types have been identified as sources of IL-2, e.g. dendritic cells (DC), NK cells, B-cells and mast cells (Bendickova and Fric Citation2020). Apart from the immune stimulating role of IL-2, the cytokine is also a critical player in the prevention of autoimmune disorders, as demonstrated in mice deficient in IL-2 or its α- or β- receptors (Sadlack et al. Citation1995; Suzuki et al. Citation1995).
Among the cells that orchestrate the immune system, DC play a pivotal role in bridging the innate and the adaptive immune response against invading pathogenic organisms. In this review, we will explore the IL-2 and DC interplay, following through on the initial discoveries that DCs induce IL-2 production by T-cells, which trigger a downstream array of helper functions (Inaba et al. Citation1983), and the observation that DC also secrete IL-2 in response to bacterial stimulation (Granucci et al. Citation2001). Moreover, the use of IL-2 within the cancer immunotherapy scope is discussed, with the aim of clarifying its synergistic-to-DC effects in the cancer microenvironment.
IL-2 Regulation of the T cell:DC axis
Cellular source of IL-2
IL-2 is a 15.5 kDa cytokine with T-cell modulating capacities in the thymus and in periphery (Ross and Cantrell Citation2018). Its various effects and functions in the thymus, periphery and gut are depicted in . IL-2 is an important player in the proliferation of naïve T-cells and maturation of T-regulatory (Treg) cells in the thymus; however, it is still unclear which cellular component (B-cells, T-cells, or DC) secretes IL-2 in that context. In an ex vivo thymic slice model, antigen-bearing cells, DC, were shown to be the main IL-2 secreting cells, promoting the Treg cell development in an antigen-specific manner (Weist et al. Citation2015). In a separate in vivo study, it was observed that T-cells, and not DC or B-cells, are the main producers of IL-2, supporting the development and homeostasis of Treg cells in the thymus (Owen et al. Citation2018).
Figure 1. Cellular source and functions of IL-2 in the thymus, periphery, and gut. In the thymus, (a) DCs bearing antigen secrete IL-2, which drives the development of Treg cells, but also (b) T-cells are shown to promote the Treg cell development and homeostasis. In periphery, (a) T effector cells act in an autocrine manner, producing IL-2, and thus regulating their proliferation and differentiation. Further, (b) Treg cell homeostasis is maintained due to IL-2 paracrine signaling, where IL-2 suppression and elevated CD25 expression enhance the Treg cell self-renewal and metabolic activity. Lastly, (c) mature DC, bearing antigen, release IL-2 that enhances T cell proliferation. In the gut, (a) endogenous IL-2 by conventional T-cells maintains tolerance, but also it has been shown that (b) CD103+ DC are able to induce the development of Treg cells, via IL-2 secretion. This figure was created with Biorender.com.
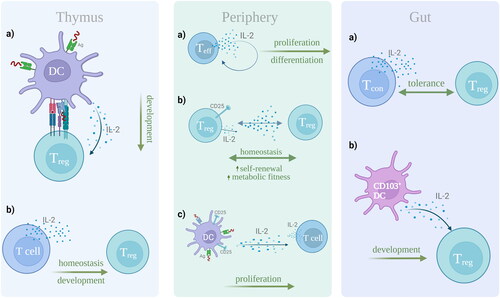
In periphery, effector T-cells are the main IL-2-secreting cells, acting in an autocrine manner, enhancing their proliferation and differentiation (Kalia and Sarkar Citation2018). In steady state resting conditions, with CD4+ T-cells, and to a lesser extent CD8+ T-cells, are the dominant producers of IL-2 (Boyman and Sprent Citation2012). Nevertheless, in vivo experiments and gene expression analyses revealed that Treg cell homeostasis is subject to paracrine IL-2 signaling, where a suppression of IL-2 production and an enhanced CD25 (IL-2Rα) expression result in Treg cell self-renewal and enhanced metabolic activity (Fontenot et al. Citation2005). Further, an in vitro syngeneic coculture model of primary human mature DC (mDC) loaded with antigen, and T-cells, revealed that directional IL-2 release at the immunological synapse has the ability to enhance T-cell proliferation, in an antigen-dependent manner, via binding of the IL-2 released by T-cells, and of the CD25 expressed by mDCs (Wuest et al. Citation2011).
Evidently, studies in different tissues and organs have prompted the question as to whether the IL-2 cellular source varies according to the microenvironment. In the gut mucosa, tolerance is maintained via Treg cells and the endogenous IL-2 production by conventional T-cells (Hsu et al. Citation2018). However, it is also reported that mucosal CD103+ DC drive the induction of Treg cells, dependent on the presence of IL-2 in vitro (Coombes et al. Citation2007).
Mechanisms involved in the IL-2 regulation by DCs
DC bridge the innate and adaptive immune system, due to their ability to recognize and internalize infectious agents and inflammatory products. They process peptides and display them on their surface in the context of the Major Histocompatibility Complex (MHC), migrate to lymphoid organs, the spleen and the lymph nodes, and present the MHC-peptide complex to naïve T-cells which will become activated in an antigen-specific manner (Banchereau and Steinman Citation1998; Morel and Butterfield Citation2015). DC were initially discovered by Ralf Steinman in 1973 (Steinman and Cohn Citation1973), and their function has been further explored in the past 25 years (Banchereau and Steinman Citation1998). DC employ pattern recognition receptors (PRRs) to recognize the foreign antigens, which then trigger signal transduction pathways and activation of various transcription factors, one of them being the Nuclear Factor of Activated T-cells (NFAT) (Zanoni et al. Citation2009). Mouse DC stimulation with lipopolysaccharides (LPS) induces NFAT translocation via CD14, which is critical for the apoptosis of terminally differentiated DC, self-tolerance and prevention of autoimmunity (Chen et al. Citation2006; Zanoni et al. Citation2009). Gene expression analysis of murine DC in response to Gram negative bacteria, identified IL-2 as one of the gene products regulated by NFAT in DC (Granucci et al. Citation2001). Under resting conditions, NFAT remains phosphorylated in the DC cytoplasm. Upon PRR trigger, calcium (Ca2+) flux activates calcineurin, a serine/threonine phosphatase, to dephosphorylate NFAT, which will translocate to the nucleus (Zaslavsky et al. Citation2013) and promote IL-2 transcription and release (Zanoni et al. Citation2009; Zelante et al. Citation2012).
In the immunological synapse of DC and T-cells, IL-2 is recognized by CD25 on the surface of DC and presented in trans to T-cells (Wuest et al. Citation2011). In vitro and in vivo studies in mice confirmed that stimulating DCs with LPS, other bacteria or zymosan, but not inflammatory cytokines, also induces the production of IL-2 by DCs, which are capable of priming naïve T-cells in response. Other DC subtypes, epidermal Langerhans cells, CD8a+ and CD8a- splenic DC, were also able to confer the same property (Granucci et al. Citation2003). In vitro, human monocyte-derived DC (moDC) were found to secrete IL-2, upon differentiation with IL-15 and with a T-cell contact via CD40L (Feau et al. Citation2005). Thus, although in mouse models a microbial stimulation can alone activate DC to secrete IL-2, in human in vitro models, DC-derived IL-2 is T-cell-dependent.
The regulation of the anti-microbial response by DC and the dependence on IL-2 was further highlighted in an in vivo study by Goodridge et al. (Goodridge et al. Citation2007), where zymosan-induced gene expression via Dectin-1 was demonstrated to trigger NFAT activation and a concomitant IL-2 production.
Dectin-1, a C-type lectin receptor, plays a pivotal role in the recognition of zymosan and other pathogenic fungi and yeast by DC, and the receptor belongs to the family of type II transmembrane proteins, with a characteristic immunoreceptor tyrosine-based activation motif (ITAM) found on its cytoplasmic tail. ITAM regulates activation and signaling to the B cell receptor and T cell receptor (Schorey and Lawrence Citation2008). Upon DC activation, the ITAM’s Tyr residues are phosphorylated by the Src family kinases- Syk and Zap70, which initiate the signaling cascade. Inhibition of Syk prevents zymosan-stimulated DC to produce IL-2 as shown by in vivo Syk blockade experiments (Rogers et al. Citation2005; Slack et al. Citation2007) underling its importance in Dectin-1 signaling. Based on the above, it is suggested that IL-2 regulation by DC occurs via the NFAT and Src kinases.
The biological role of DC-derived IL-2
Taking into consideration the effects that IL-2 has on the DC secretory milieu, it is intriguing to explore the systemic effects that it may also regulate in response to disease. A study by Mencarelli et al. investigated the role of IL-2, produced by different myeloid cell populations, in the intestine. They identified CD103+ DCs as the main IL-2 producers in the murine colon, unlike CD64+ F4/80± macrophages. Further, mice deficient in calcineurin or IL-2 expression in CD11c+ cells showed a severe intestinal inflammation with a decreased Treg cell number and a dysregulated CD4+ T-cell function (Mencarelli et al. Citation2018). When comparing mice deficient in IL-2 expression in CD4+ T-cells (IL-2CD4) or CD11c+ cells (IL-2CD11c) and IL-2 knockout (IL-2KO) mice, they observed severe anemia, splenomegaly, aberrant proliferation of T-cells, with a decreased Treg cell number and loss of B-cells in the IL-2CD4 and IL-2KO, indicative of the role IL-2 has in maintaining peripheral immune homeostasis. IL-2CD11c mice exhibited an increased transmural infiltration of mononuclear cells. However, in the intestinal microenvironment, IL-2CD11c showed a higher susceptibility to colitis, with an increased CD4+ T-cell population secreting IFNγ and IL-17, and a lower Treg cell population (Mencarelli et al. Citation2018). Additionally, they identified two signaling pathways involved in the overall IL-2 production by DC: the calcineurin-NFAT pathway and the TRAF6-NFκB. The first was identified as the major contributor in the induction of Treg cell homeostasis in the gut, whereas TRAF6-deficient DC in mice were responsible for a reduced inflammatory cytokine secretion by DCs and a T=helper 2 (TH2)—cell mediated enteritis, due to partial loss of IL-2 expression (Mencarelli et al. Citation2018). These observations are in agreement with an earlier study where mice with TRAF-6 deleted from DC, also presented a TH2-mediated eosinophilic enteritis, with a downregulated Treg cell proliferation and activity in the intestine (Han et al. Citation2013). The importance of DC-produced IL-2 in the Treg cell homeostasis in the gut was confirmed when these mice were injected with exogenous IL-2 and the Treg cell population and function were restored (Han et al. Citation2013).
Consistent with the finding that CD103+ DC are the main IL-2-producing cells in the gut, a separate in vivo study confirmed that the same population is responsible for the highest IL-2 production in the lung following Aspergillus fumigatus infection (Zelante et al. Citation2015). Additionally, mice deficient of IL-2 in CD11c+ DC, produced significantly higher levels of IL-17 and IL-23 compared to wild-type mice, driving the TH17 pathologic response and presenting a lower survival rate (Zelante et al. Citation2015).
A separate study however, identified Type 3 innate lymphoid cells (ILC3) as the highest secreting IL-2 cells in the small intestine which consequently regulate the Treg cell homeostasis in the gut (Zhou et al. Citation2019). Dysregulation of the IL-2 production by ILC3s, contributed to higher inflammation of the intestine and Crohn’s disease (Zhou et al. Citation2019).
Cyclosporine A is a calcineurin inhibitor, used as an immunosuppressant in transplantation to prevent graft vs host disease (GVHD) and in a range of other immune-mediated diseases. Cyclosporine A blocks the NFAT-directed transcription in T-cells (Flanagan et al. Citation1991), and its use post- transplantation has been associated with increased rates of fungal infections, predominantly Aspergillus infections. In recent years studies have shown that the inhibition of the calcineurin/NFAT signaling plays a pivotal role in the control of the myeloid-related immune response, as it is considered a risk factor for the manifestation of fungal infections post-transplantation (Seyedmousavi and Davis Citation2017). The use of Cyclosporine A has been shown to inhibit the secretion of IL-2 by DC, which leads to decreased ability of DCs to activate naïve T-cells, which in turn may explain the allograft acceptance in transplantation (Sauma et al. Citation2003).
The interplay of DCs with IL-2 in anti-tumor response
IL-2 was one of the first cytokine immunotherapies that the FDA approved for the treatment of cancer, with durable anti-tumor responses in metastatic melanoma and metastatic renal cell carcinoma. The exact interplay of IL-2 with DCs in mediating the anti-tumor immune response is still being under investigation, however some proposed DC- other immune cell interactions upon IL-2 administration are explored (). Immunotherapy with IL-2 promotes the expansion of conventional DC (cDC) in the spleens and lymph nodes of wild-type mice (Raeber et al. Citation2020). In vivo mechanistic studies in humans and mice injected with IL-2, identified three growth factors - FMS-like tyrosine kinase 3 ligand (FLT3L), colony-stimulating factor 2 (CSF-2) and tumor necrosis factor (TNF) - which upon production by ILCs, NK cells and T-cells, stimulate expansion of a specific subset of Type 1 cDC (cDC1) (BATF3+ IRF8+ CD103+ cDC1), that regulates anti-tumoral responses by CD8+ T-cells. A concomitant increase in Type 2 cDC (cDC2) is also observed, which in turn primes the CD4+ T-cell anti-tumoral response (Raeber et al. Citation2020).cDC1 in humans and mice are specializing in cross-presentation of tumor antigens to CD8+ T-cells, thus enhancing the antigen-specific anti-tumor immune response (Noubade et al. Citation2019). A high cDC1 infiltration at the tumor microenvironment (TME) is considered a good prognostic factor in cancer (Broz et al. Citation2014; Spranger et al. Citation2017; Barry et al. Citation2018).
Figure 2. Proposed interactions between DC and other immune cells in the TME, following IL-2 immunotherapy. Upon IL-2 administration, T-effector cells, ILCs and NK cells expand the cDC1 population in the tumor microenvironment, through the increased production of FLT3L, CSF-2 and TNF. Subsequently, cDC1-mediated IL-2 secretion aids the NK cell production of IFNγ, TNFα and GM-CSF. In this bi-directional crosstalk, NK cells further recruit cDC1 cells which lead to a concomitant increase in the cDC2 population, leading to an anti-tumoral priming of cytotoxic CD8+ T-cells and CD4+ T-cells respectively. A combinatorial treatment with IL-2 and for example anti-CD40 or all-trans retinoic acid, has shown that DC are able to promote a Treg cell homeostasis in the TME, following DC-derived IL-2 secretion, and an inhibition of suppressive cell populations like MDSC, further augmenting the anti-tumoral immune response. This figure was created with Biorender.com.
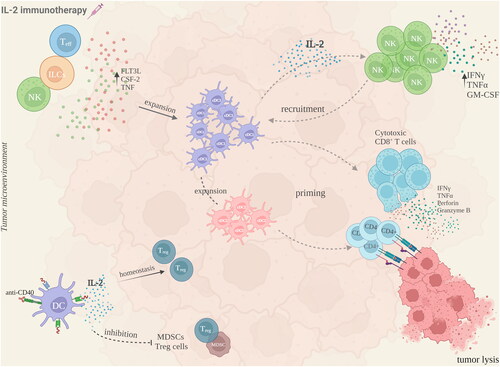
Apart from their interaction with T-cells, cDC1 also interact with NK cells and the crosstalk between them is bidirectional. cDC1 can be recruited in the TME by NK cells, via the chemokines CCL5 and XCL1 (Böttcher et al. Citation2018), and mature DC produce among other cytokines, IL-2, that aids NK cell production of IFNγ, TNFα, or GM-CSF which also promote DC maturation (Gerosa et al. Citation2002).
The importance of DC-derived IL-2 in the NK cell-mediated anti-tumoral response was highlighted by Granucci et al. (Granucci et al. Citation2004), in a melanoma mouse model with lung metastases. It was observed that priming NK cells with bacterially activated DCs which produce IL-2, is required for the NK cell production of IFNγ and the subsequent tumor lysis. Priming NK cells with DCs that were deficient of IL-2, led to an increased lung metastasis growth rate, when compared to priming of NK cells with wild-type DC. Thus, it was concluded that NK cell cytotoxicity is elicited in an IL-2 dependent manner, where bacterially induced DC are the main IL-2 cell providers (Granucci et al. Citation2004).
Immunotherapy with IL-2 and a parallel DC vaccination has also been explored in the context of cancer (Wang et al. Citation2020). Although IL-2 does not directly enhance the DC-vaccine-stimulated anti-tumoral effects, it may circumvent immunosuppressive factors (eg. Treg cells) in the TME that may curtail the efficacy of such vaccines and offer a synergistic anti-tumoral effect.
Treg cells promote an immunosuppressive TME by downregulating DC-therapy induced immune cell populations like CD8+ or CD4+ T-cells, through the secretion of immunosuppressive cytokines (eg. TGFβ or IL-10) or via inhibitory receptor-ligand interactions (e.g., Programmed cell death-1 and its ligand) (Tanaka and Sakaguchi Citation2017). Considering that DC-derived IL-2 may aid Treg cell homeostasis in an organ-specific context as mentioned earlier, a successful DC-targeted immunotherapy would entail the use of additional agents that downregulate the immunosuppressive Treg cell population in the TME and promote T-cell functions. The latter was investigated in metastatic melanoma patients who were treated with autologous DC pulsed with tumor-specific peptides, combined with IL-2, cyclophosphamide and a COX-2 inhibitor, in a phase II clinical trial. Although this combinatorial regimen deemed safe and well tolerated by patients, with a median overall survival rate of 9.4 months, the Treg cell population was not decreased despite the use of cyclophosphamide, a chemotherapeutic immunosuppressive drug (Ellebaek et al. Citation2012). Conversely, in another study with 17 advanced melanoma patients, a significant reduction of Treg cells was observed in patients with partial response or stable disease, following pretreatment with Temozolomide, and a tumor-lysate DC therapy combined with IL-2 (Ridolfi et al. Citation2013). The enigma remains as to whether the Treg cell reduction relates to the treatment alone, or if it also has a clinical relevance in terms of treatment efficacy and patient survival. A Phase I/II clinical trial in ovarian cancer patients, who were vaccinated with autologous moDC supplemented with IL-2, resulted in a 4-year survival rate for the complete responders (50%). A subsequent decreased Treg cell proportion in the periphery was also observed, however it was inconclusive whether this result correlated with the clinical response to treatment and survival rate (Baek et al. Citation2015).
Another cell population in the TME presenting an immunosuppressive activity are the myeloid-derived suppressor cells (MDSC) (Kumar et al. Citation2016; Umansky et al. Citation2016). They are bone marrow-derived and considered antigen-naïve cells, unable to differentiate to DC or macrophages, due to the inhibiting profile they present. They are divided into two groups, based on their morphology and phenotype. Polymorphonuclear MDSC (PMN-MDSC) resemble neutrophils, whereas monocytic MDSC (M-MDSC) resemble monocytes (Kumar et al. Citation2016). Their high abundance in the circulation or the TME has been associated with a higher tumor burden and poor prognosis in a range of cancers (Wang et al. Citation2013; Zhang et al. Citation2013; Jiang et al. Citation2015). A successful IL-2 immunotherapy would also target the MDSC’ tumorigenic effects and efforts toward this have also been reported. In renal cell carcinoma, a combinatorial therapy of IL-2 with all-trans retinoic acid, was able to modulate the DC function and antigen-specific immune response by downregulating the MDSC frequency in the TME (Mirza et al. Citation2006). In another renal adenocarcinoma mouse model, treatment with anti-CD40 and IL-2 induced an anti-tumoral immune response as indicated by the IFNγ-mediated recruitment of tumor-infiltrating leukocytes in the TME and a downregulated Treg and MDSC frequency (Weiss et al. Citation2009). This effect was achieved via FAS-mediated apoptosis (Weiss et al. Citation2014).
Conclusion and perspectives
IL-2 is an immune -stimulatory and -regulatory cytokine with a wide range of effects on innate and adaptive immune cells, dependent on the biological context. It was originally proposed as a T-cell growth cytokine, however, it is now clear that IL-2 affects many other cell types dependent on the disease context.
Harnessing the mechanistic interactions that occur between IL-2, DCs and T-cells are of high value in cancer therapy, where IL-2 can induce DC expansion with a cumulative anti-tumoral effect of T-cells and circumvent immunosuppressive factors in the TME. However, in transplantation, IL-2 blockade may favor the allograft acceptance. On these grounds, it is important to delineate the interactions that occur between IL-2 and immune cells in a specific disease context, prior to translating novel IL-2 alternatives in the clinic. Underpinning the events that lead to a successful immunomodulatory drug, would allow for the future design of safer and more efficacious immunotherapies. Moreover, it is critical to limit the adverse side effects related to the IL-2 treatment. This requires a very fine-tuning of the dose and the route of administration of novel IL-2 therapies. A better understanding of the pathophysiological mechanisms behind the adverse toxicities occurring upon IL-2 therapy may aid in the optimal dose selection and the prediction or prevention of related toxicities. Ultimately, we may come up with insights that will assist in future IL-2-, and other cytokine therapies and improve the selection criteria of patients who will benefit from such treatments.
Disclosure statement
No potential conflict of interest was reported by the author(s).
Correction Statement
This article was originally published with errors, which have now been corrected in the online version. Please see Correction (http://dx.doi.org/10.1080/1547691X.2024.2342736)
Additional information
Funding
References
- Baek S, Kim Y-M, Kim S-B, Kim C-S, Kwon S-W, Kim Y, Kim H, Lee H. 2015. Therapeutic DC vaccination with IL-2 as a consolidation therapy for ovarian cancer patients: A Phase I/II trial. Cell Mol Immunol. 12(1):87–95. doi: 10.1038/cmi.2014.40.
- Banchereau J, Steinman RM. 1998. Dendritic cells and the control of immunity. Nature. 392(6673):245–252. doi: 10.1038/32588.
- Barron L, Dooms H, Hoyer KK, Kuswanto W, Hofmann J, O‘Gorman WE, Abbas AK. 2010. Cutting edge: Mechanisms of IL-2-dependent maintenance of functional regulatory T-cells. J Immunol. 185(11):6426–6430. doi: 10.4049/jimmunol.0903940.
- Barry KC, Hsu J, Broz ML, Cueto FJ, Binnewies M, Combes AJ, Nelson AE, Loo K, Kumar R, Rosenblum MD, et al. 2018. A natural killer-dendritic cell axis defines checkpoint therapy-responsive tumor microenvironments. Nat Med. 24(8):1178–1191. doi: 10.1038/s41591-018-0085-8.
- Bendickova K, Fric J. 2020. Roles of IL-2 in bridging adaptive and innate immunity, and as a tool for cellular immunotherapy. J Leukoc Biol. 108(1):427–437. doi: 10.1002/JLB.5MIR0420-055R.
- Böttcher JP, Bonavita E, Chakravarty P, Blees H, Cabeza-Cabrerizo M, Sammicheli S, Rogers NC, Sahai E, Zelenay S, Reis e Sousa C, et al. 2018. NK cells stimulate recruitment of cDC1 into the tumor microenvironment promoting cancer immune control. Cell. 172(5):1022–1037 e14. doi: 10.1016/j.cell.2018.01.004.
- Boyman O, Sprent J. 2012. The role of interleukin-2 during homeostasis and activation of the immune system. Nat Rev Immunol. 12(3):180–190. doi: 10.1038/nri3156.
- Broz ML, Binnewies M, Boldajipour B, Nelson AE, Pollack JL, Erle DJ, Barczak A, Rosenblum MD, Daud A, Barber DL, et al. 2014. Dissecting the tumor myeloid compartment reveals rare activating antigen-presenting cells critical for T-cell immunity. Cancer Cell. 26(6):938. doi: 10.1016/j.ccell.2014.11.010.
- Chen M, Wang Y-H, Wang Y, Huang L, Sandoval H, Liu Y-J, Wang J. 2006. Dendritic cell apoptosis in the maintenance of immune tolerance. Science. 311(5764):1160–1164. doi: 10.1126/science.1122545.
- Coombes JL, Siddiqui KRR, Arancibia-Cárcamo CV, Hall J, Sun C-M, Belkaid Y, Powrie F. 2007. A functionally specialized population of mucosal CD103+ DC induces Foxp3+ regulatory T-cells via a TGFb and retinoic acid-dependent mechanism. J Exp Med. 204(8):1757–1764. doi: 10.1084/jem.20070590.
- Ellebaek E, Engell-Noerregaard L, Iversen TZ, Froesig TM, Munir S, Hadrup SR, Andersen MH, Svane IM. 2012. Metastatic melanoma patients treated with dendritic cell vaccination, IL-2, and metronomic cyclophosphamide: Results from a Phase II trial. Cancer Immunol Immunother. 61(10):1791–1804. doi: 10.1007/s00262-012-1242-4.
- Feau S, Facchinetti V, Granucci F, Citterio S, Jarrossay D, Seresini S, Protti MP, Lanzavecchia A, Ricciardi-Castagnoli P. 2005. Dendritic cell-derived IL-2 production is regulated by IL-15 in humans and in mice. Blood. 105(2):697–702. doi: 10.1182/blood-2004-03-1059.
- Flanagan WM, Corthésy B, Bram RJ, Crabtree GR. 1991. Nuclear association of a T-cell transcription factor blocked by FK-506 and cyclosporin A. Nature. 352(6338):803–807. doi: 10.1038/352803a0.
- Fontenot JD, Rasmussen JP, Gavin MA, Rudensky AY. 2005. A function for IL-2 in Foxp3-expressing regulatory T-cells. Nat Immunol. 6(11):1142–1151. doi: 10.1038/ni1263.
- Gerosa F, Baldani-Guerra B, Nisii C, Marchesini V, Carra G, Trinchieri G. 2002. Reciprocal activating interaction between natural killer cells and dendritic cells. J Exp Med. 195(3):327–333. doi: 10.1084/jem.20010938.
- Goodridge HS, Simmons RM, Underhill DM. 2007. Dectin-1 stimulation by Candida albicans yeast or zymosan triggers NFAT activation in macrophages and dendritic cells. J Immunol. 178(5):3107–3115. doi: 10.4049/jimmunol.178.5.3107.
- Granucci F, Feau S, Angeli V, Trottein F, Ricciardi-Castagnoli P. 2003. Early IL-2 production by mouse dendritic cells is the result of microbial-induced priming. J Immunol. 170(10):5075–5081. doi: 10.4049/jimmunol.170.10.5075.
- Granucci F, Vizzardelli C, Pavelka N, Feau S, Persico M, Virzi E, Rescigno M, Moro G, Ricciardi-Castagnoli P. 2001. Inducible IL-2 production by dendritic cells revealed by global gene expression analysis. Nat Immunol. 2(9):882–888. doi: 10.1038/ni0901-882.
- Granucci F, Zanoni I, Pavelka N, Van Dommelen SLH, Andoniou CE, Belardelli F, Degli Esposti MA, Ricciardi-Castagnoli P. 2004. A contribution of mouse dendritic cell-derived IL-2 for NK cell activation. J Exp Med. 200(3):287–295. doi: 10.1084/jem.20040370.
- Han D, Walsh MC, Cejas PJ, Dang NN, Kim YF, Kim J, Charrier-Hisamuddin L, Chau L, Zhang Q, Bittinger K, et al. 2013. Dendritic cell expression of the signaling molecule TRAF6 is critical for gut microbiota-dependent immune tolerance. Immunity. 38(6):1211–1222. doi: 10.1016/j.immuni.2013.05.012.
- Hsu PS, Lai CL, Hu M, Santner-Nanan B, Dahlstrom JE, Lee CH, Ajmal A, Bullman A, Arbuckle S, Al Saedi A, et al. 2018. IL-2 enhances gut homing potential of human naive regulatory T-cells early in life. J Immunol. 200(12):3970–3980. doi: 10.4049/jimmunol.1701533.
- Inaba K, Granelli-Piperno A, Steinman RM. 1983. Dendritic cells induce T-lymphocytes to release B-cell-stimulating factors by an IL-2-dependent mechanism. J Exp Med. 158(6):2040–2057. doi: 10.1084/jem.158.6.2040.
- Jiang H, Gebhardt C, Umansky L, Beckhove P, Schulze TJ, Utikal J, Umansky V. 2015. Elevated chronic inflammatory factors and myeloid-derived suppressor cells indicate poor prognosis in advanced melanoma patients. Int J Cancer. 136(10):2352–2360. doi: 10.1002/ijc.29297.
- Kalia V, Sarkar S. 2018. Regulation of effector and memory CD8 T-cell differentiation by IL-2-A balancing act. Front Immunol. 9:2987. doi: 10.3389/fimmu.2018.02987.
- Kehrl JH, Dukovich M, Whalen G, Katz P, Fauci AS, Greene WC. 1988. Novel interleukin 2 (IL-2) receptor appears to mediate IL-2-induced activation of natural killer cells. J Clin Invest. 81(1):200–205.
- Kumar V, Patel S, Tcyganov E, Gabrilovich DI. 2016. The nature of myeloid-derived suppressor cells in the tumor microenvironment. Trends Immunol. 37(3):208–220. doi: 10.1016/j.it.2016.01.004.
- Mencarelli A, Khameneh HJ, Fric J, Vacca M, El Daker S, Janela B, Tang JP, Nabti S, Balachander A, Lim TS, et al. 2018. Calcineurin-mediated IL-2 production by CD11cMHCII myeloid cells is crucial for intestinal immune homeostasis. Nat Commun. 9(1):1102. doi: 10.1038/s41467-018-03495-3.
- Mirza N, Fishman M, Fricke I, Dunn M, Neuger AM, Frost TJ, Lush RM, Antonia S, Gabrilovich DI. 2006. All-trans-retinoic acid improves differentiation of myeloid cells and immune response in cancer patients. Cancer Res. 66(18):9299–9307. doi: 10.1158/0008-5472.CAN-06-1690.
- Morel PA, Butterfield LH. 2015. Dendritic cell control of immune responses. Front Immunol. 6:42. doi: 10.3389/fimmu.2015.00042.
- Nelson BH. 2004. IL-2, regulatory T-cells, and tolerance. J Immunol. 172(7):3983–3988. doi: 10.4049/jimmunol.172.7.3983.
- Noubade R, Majri-Morrison S, Tarbell KV. 2019. Beyond cDC1: Emerging roles of DC crosstalk in cancer immunity. Front Immunol. 10:1014. doi: 10.3389/fimmu.2019.01014.
- Owen DL, Mahmud SA, Vang KB, Kelly RM, Blazar BR, Smith KA, Farrar MA. 2018. Identification of cellular sources of IL-2 needed for regulatory T-cell development and homeostasis. J Immunol. 200(12):3926–3933. doi: 10.4049/jimmunol.1800097.
- Raeber ME, Rosalia RA, Schmid D, Karakus U, Boyman O. 2020. Interleukin-2 signals converge in a lymphoid-dendritic cell pathway that promotes anticancer immunity. Sci Transl Med. 12:561. doi: 10.1126/scitranslmed.aba5464.
- Ridolfi L, Petrini M, Granato AM, Gentilcore G, Simeone E, Ascierto PA, Pancisi E, Ancarani V, Fiammenghi L, Guidoboni M, et al. 2013. Low-dose temozolomide before dendritic-cell vaccination reduces (specifically) CD4+CD25FoxP3+ regulatory T-cells in advanced melanoma patients. J Transl Med. 11(1):135. doi: 10.1186/1479-5876-11-135.
- Rogers NC, Slack EC, Edwards AD, Nolte MA, Schulz O, Schweighoffer E, Williams DL, Gordon S, Tybulewicz VL, Brown GD, et al. 2005. Syk-dependent cytokine induction by Dectin-1 reveals a novel pattern recognition pathway for C type lectins. Immunity. 22(4):507–517. doi: 10.1016/j.immuni.2005.03.004.
- Rosenberg SA, Lotze MT, Muul LM, Leitman S, Chang AE, Ettinghausen SE, Matory YL, Skibber JM, Shiloni E, Vetto JT, et al. 1985. Observations on the systemic administration of autologous lymphokine-activated killer cells and recombinant interleukin-2 to patients with metastatic cancer. N Engl J Med. 313(23):1485–1492. doi: 10.1056/NEJM198512053132327.
- Rosenberg SA. 2021. Correction to: A Journey in Science: Immersion in the search for effective cancer immunotherapies. Mol Med. 27(1):140. doi: 10.1186/s10020-021-00405-0.
- Ross SH, Cantrell DA. 2018. Signaling and function of IL-2 in T-lymphocytes. Annu Rev Immunol. 36(1):411–433. doi: 10.1146/annurev-immunol-042617-053352.
- Sadlack B, Löhler J, Schorle H, Klebb G, Haber H, Sickel E, Noelle RJ, Horak I. 1995. Generalized autoimmune disease in IL-2-deficient mice is triggered by an uncontrolled activation and proliferation of CD4+ T-cells. Eur J Immunol. 25(11):3053–3059. doi: 10.1002/eji.1830251111.
- Sauma D, Fierro A, Mora JR, Lennon-Duménil AM, Bono MR, Rosemblatt M, Morales J. 2003. Cyclosporine preconditions dendritic cells during differentiation and reduces IL-2 and IL-12 production following activation: A potential tolerogenic effect. Transplant Proc. 35(7):2515–2517. doi: 10.1016/j.transproceed.2003.09.020.
- Schorey JS, Lawrence C. 2008. The pattern recognition receptor Dectin-1: From fungi to mycobacteria. Curr Drug Targets. 9(2):123–129. doi: 10.2174/138945008783502430.
- Seyedmousavi S, Davis MJ. 2017. Defective calcineurin/NFAT signaling in myeloid cells and susceptibility to aspergillosis in post-transplant patients. Virulence. 8(8):1498–1501. doi: 10.1080/21505594.2017.1380143.
- Siegel JP, Puri RK. 1991. Interleukin-2 toxicity. J Clin Oncol. 9(4):694–704. doi: 10.1200/JCO.1991.9.4.694.
- Slack EC, Robinson MJ, Hernanz-Falcón P, Brown GD, Williams DL, Schweighoffer E, Tybulewicz VL, Reis e Sousa C. 2007. Syk-dependent ERK activation regulates IL-2 and IL-10 production by DC stimulated with zymosan. Eur J Immunol. 37(6):1600–1612. doi: 10.1002/eji.200636830.
- Smith KA. 1988. Interleukin-2: Inception, impact, and implications. Science. 240(4856):1169–1176. doi: 10.1126/science.3131876.
- Spranger S, Dai D, Horton B, Gajewski TF. 2017. Tumor-residing Batf3 dendritic cells are required for effector T-cell trafficking and adoptive T-cell therapy. Cancer Cell. 31(5):711–723 e4. doi: 10.1016/j.ccell.2017.04.003.
- Steinman RM, Cohn ZA. 1973. Identification of a novel cell type in peripheral lymphoid organs of mice. I. Morphology, quantitation, tissue distribution. J Exp Med. 137(5):1142–1162. doi: 10.1084/jem.137.5.1142.
- Suzuki H, Kündig TM, Furlonger C, Wakeham A, Timms E, Matsuyama T, Schmits R, Simard JJ, Ohashi PS, Griesser H, et al. 1995. De-regulated T-cell activation and autoimmunity in mice lacking IL-2 receptor beta. Science. 268(5216):1472–1476. doi: 10.1126/science.7770771.
- Tanaka A, Sakaguchi S. 2017. Regulatory T-cells in cancer immunotherapy. Cell Res. 27(1):109–118. doi: 10.1038/cr.2016.151.
- Umansky V, Blattner C, Gebhardt C, Utikal J. 2016. The role of Myeloid-Derived Suppressor Cells (MDSC) in cancer progression. Vaccines. 4(4):36. doi: 10.3390/vaccines4040036.
- Wang L, Chang EWY, Wong SC, Ong S-M, Chong DQY, Ling KL. 2013. Increased myeloid-derived suppressor cells in gastric cancer correlate with cancer stage and plasma S100A8/A9 proinflammatory proteins. J Immunol. 190(2):794–804. doi: 10.4049/jimmunol.1202088.
- Wang Y, Xiang Y, Xin VW, Wang X-W, Peng X-C, Liu X-Q, Wang D, Li N, Cheng J-T, Lyv Y-N, et al. 2020. Dendritic cell biology and its role in tumor immunotherapy. J Hematol Oncol. 13(1):107. doi: 10.1186/s13045-020-00939-6.
- Weiss JM, Back TC, Scarzello AJ, Subleski JJ, Hall VL, Stauffer JK, Chen X, Micic D, Alderson K, Murphy WJ, et al. 2009. Successful immunotherapy with IL-2/anti-CD40 induces the chemokine-mediated mitigation of an immunosuppressive tumor microenvironment. Proc Natl Acad Sci USA. 106(46):19455–19460. doi: 10.1073/pnas.0909474106.
- Weiss JM, Subleski JJ, Back T, Chen X, Watkins SK, Yagita H, Sayers TJ, Murphy WJ, Wiltrout RH. 2014. Regulatory T-cells and myeloid-derived suppressor cells in the tumor microenvironment undergo Fas-dependent cell death during IL-2/alphaCD40 therapy. J Immunol. 192(12):5821–5829. doi: 10.4049/jimmunol.1400404.
- Weist BM, Kurd N, Boussier J, Chan SW, Robey EA. 2015. Thymic regulatory T-cell niche size is dictated by limiting IL-2 from antigen-bearing dendritic cells and feedback competition. Nat Immunol. 16(6):635–641. doi: 10.1038/ni.3171.
- Wuest SC, Edwan JH, Martin JF, Han S, Perry JSA, Cartagena CM, Matsuura E, Maric D, Waldmann TA, Bielekova B, et al. 2011. A role for IL-2 trans-presentation in dendritic cell-mediated T-cell activation in humans, as revealed by daclizumab therapy. Nat Med. 17(5):604–609. doi: 10.1038/nm.2365.
- Zanoni I, Ostuni R, Capuano G, Collini M, Caccia M, Ronchi AE, Rocchetti M, Mingozzi F, Foti M, Chirico G, et al. 2009. CD14 regulates the dendritic cell life cycle after LPS exposure through NFAT activation. Nature. 460(7252):264–268. doi: 10.1038/nature08118.
- Zaslavsky A, Chou ST, Schadler K, Lieberman A, Pimkin M, Kim YJ, Baek K-H, Aird WC, Weiss MJ, Ryeom S, et al. 2013. The calcineurin-NFAT pathway negatively regulates megakaryopoiesis. Blood. 121(16):3205–3215. doi: 10.1182/blood-2012-04-421172.
- Zelante T, Fric J, Wong AYW, Ricciardi-Castagnoli P. 2012. Interleukin-2 production by dendritic cells and its immuno-regulatory functions. Front Immunol. 3:161. doi: 10.3389/fimmu.2012.00161.
- Zelante T, Wong AYW, Ping TJ, Chen J, Sumatoh HR, Viganò E, Hong Bing Y, Lee B, Zolezzi F, Fric J, et al. 2015. CD103 dendritic cells control TH17 cell function in the lung. Cell Rep. 12(11):1789–1801. doi: 10.1016/j.celrep.2015.08.030.
- Zhang B, Wang Z, Wu L, Zhang M, Li W, Ding J, Zhu J, Wei H, Zhao K. 2013. Circulating and tumor-infiltrating myeloid-derived suppressor cells in patients with colorectal carcinoma. PLoS One. 8(2):e57114. doi: 10.1371/journal.pone.0057114.
- Zhou L, Chu C, Teng F, Bessman NJ, Goc J, Santosa EK, Putzel GG, Kabata H, Kelsen JR, Baldassano RN, et al. 2019. Innate lymphoid cells support regulatory T-cells in the intestine through interleukin-2. Nature. 568(7752):405–409. doi: 10.1038/s41586-019-1082-x.