Abstract
Background A one-step exchange of an endoprosthesis with periprosthetic infection requires effective antibiotics at high concentrations around the endoprosthesis. We evaluated the tissue distribution of vancomycin and Moxifloxacin in a standardized in vivo model of periprosthetic infection.
Methods 36 male rats with periprosthetic infection of the left hind leg, induced by a standardized procedure, received either antibiotic treatment with vancomycin or Moxifloxacin twice daily for 2 weeks, or a sham treatment. After the last administration, different tissues from each animal were evaluated for concentrations of antibiotic.
Results Compared to plasma, the tissue concentrations of Moxifloxacin were higher in all tissues investigated (lung, muscle, fat, bone) and the tissue-plasma ratio of Moxifloxacin was considerably higher than that of vancomycin. The concentrations of Moxifloxacin were equally high in the infected and the uninfected hind leg, whereas the vancomycin concentrations were significantly higher in the infected leg.
Interpretation The standardized model of periprosthetic infection described here can be extrapolated to different bacterial and mycotic pathogens, and also to different antibiotics or therapeutic regimes. It provides a way of correlating tissue concentrations with clinical outcome in future studies.
Periprosthetic infection is a severe complication. An overall infection rate of about 1% for primary surgery can be found in the literature, but even higher rates of 2.5–7% for primary surgery and 12–15% for revision surgery have been reported (Eveillard et al. Citation2001, Puolakka et al. Citation2001, Nguyen et al. Citation2002, Sharkey et al. Citation2002). Endoprosthesispreserving treatment and also one-step and twostep exchanges have been described. All procedures require thorough irrigation, debridement, and concomitant long-term antibiotic therapy lasting several months. There is, however, controversy surrounding these methods; none of them are generally accepted (Drobny and Munzinger Citation1991, Isiklar et al. Citation1999, Segawa et al. Citation1999, Kordelle et al. Citation2000, Silva et al. Citation2002). A critical issue in the one-step procedure is the (mostly) unknown bacterial pathogen during surgery and the appropriate first-line antibiotic (Della Valle et al. Citation1999, Spangehl et al. Citation1999a, Citationb). Antibiotics at effective concentrations in the surrounding tissues—and especially the bone—are crucial. The fourth-gen-eration quinolone Moxifloxacin, which has a broad spectrum of antibacterial activity and reaches high concentrations in tissues, might be considered as a first-line antibiotic in periprosthetic infections, which might in turn allow a safer one-step procedure (Dalhoff Citation1999, Hoogkamp-Korstanje and Roelofs-Willemse Citation2000, Kaatz et al. Citation2002, Dalhoff and Schmitz Citation2003, Ince et al. Citation2003). Vancomycin is an accepted standard antibiotic used in periprosthetic infection.
We evaluated the tissue distribution of vancomycin and Moxifloxacin in rats in a standardized in vivo model of periprosthetic infection caused by Staphylococcus aureus.
Methods
The protocol used was approved by the official local Institutional Animal Care and Protection Committee (Veterinary Department, government of the Oberpfalz, application no. 621-2531.1-12/03).
Animals and bacterial strain
36 male Wistar rats (Charles River, Sulzfeld, Germany) were used for the experiments. The animals were aged 12–14 weeks, had an average body weight (BW) of 452 g (SD 29), were housed one per cage in a room with controlled temperature (23–25°C) and a light-dark cycle of 12:12 h, and they had free access to a standard pellet diet and tap water. An oxacillin-sensitive Staphylococcus aureus strain (ATCC 29213) was used for bacterial contamination.
Surgical procedure
After a one-week settling-in period, each rat was anesthetized with an intraperitoneal injection of 90–120 mg/kg BW ketamine (10%) and 6–8 mg/kg BW xylazine. Surgery was performed under aseptic conditions. The implantation of the foreign bodies was carried out mimicking a retrograde femur nailing: the knee joint of the left hind leg was arthrotomized by parapatellar incision, the patella luxated medially, and the medullary cavity was then intercondylarly opened with K-wires, and gradually widened using K-wires of increasing diameter up to 1.6 mm. The rat femur was then inoculated with 100 µL of the S. aureus suspension (108 colony forming units/ml (cfu/mL)) and a sterile 16-G standard needle cut to a length of 15 mm was implanted intramedullary. The medullary cavity was closed with sterile bone wax, and the joint was irrigated with physiological saline and the wound sutured. A disinfecting wound ointment was applied. Before antibiotic treatment was started, animals were observed daily for a period of 1 week, and their activity, protection posture, and wound healing were recorded.
Therapeutic procedure and collection of samples
The antibiotics used were vancomycin and Moxifloxacin. On day 7, the rats were randomized into 3 groups of 12 animals each. Depending on the group, they received an intraperitoneal injection of either vancomycin 15 mg/kg BW or moxifloxacin 10 mg/kg BW, or physiological saline, twice daily (i.e. at 12-hour intervals) from day 7 to day 21 postoperatively. On day 21, the animals were killed—2 h after the last administration of antibiotic—in deep ether anesthesia by an intracardial overdose injection of pentobarbital. Immediately before administration of barbiturates, 1 mL blood (EDTA Monovette; Sarstedt, Nümbrecht, Germany) was taken by intracardial puncture. The blood was centrifuged, and the plasma was separated and stored at –25°C until further analysis. The treated knees were re-arthrotomized and infection was confirmed clinically and radiologically ( and ). The femora of both hind legs, soft tissue surrounding the knee joints (including capsule and some muscle) on both sides, and also lung and fatty tissue from the groin of the uninfected side were removed, frozen in liquid nitrogen, and stored at −25°C.
Determination of Moxifloxacin by high-perfor-mance liquid chromatography (HPLC)
Moxifloxacin was determined by HPLC and fluorimetric detection, adapted from a previously published method (Kees et al. Citation1988). Briefly, plasma was deproteinized with 4 volumes of methanol. Tissue (200–250 mg lung, fat, or muscle) was homogenized with an Ultra Turrax TP 18–10 (IKA, Laufen, Germany) in 10 volumes (w/v) of a mixture of water, methanol, 70% perchloric acid, and 85% ortho-phosphoric acid (ratio 500:500:10:1, v/v/v/v). Bone was deep-frozen in liquid nitrogen and pulverized in a chilled home-made stainless steel mortar with pestle. Then, the bone meal was transferred to a polypropylene tube, plugged, and extracted by shaking horizontally for 30 min in 8 volumes (w/v) of the homogenization solution. Gatifloxacin was used as an internal standard. For chromatography, an LC-10 series HPLC system was used and a RF-10AXL fluorimetric detector (Shimadzu, Duisburg, Germany) was set at ex/em 296/504 nm. Separation was performed using a Synergi Polar RP column (internal diameter 150 × 4.6 mm; Phenomenex, Aschaffenburg, Germany) and an eluent consisting of 1 L water, 1.0 mL 85% ortho-phosphoric acid, 1.0 g tetrabutyl ammonium hydrogen sulfate (adjusted to pH 3.0 with 10 M NaOH) and 200 ml acetonitrile. Moxifloxacin and the internal standard gatifloxacin eluted after appro-ximately 8 min and 6 min, respectively (flow rate 1.0 mL/min, column temperature 30°C). The recovery of gatifloxacin and Moxifloxacin was 100% from plasma and bone and 85–90% from lung, fat, and muscle. Linearity has been proven between 50 and 2,000 ng/mL by assaying spiked plasma specimens. Precision was better than 10% down to 50 ng/mL Moxifloxacin in plasma and 50 ng/g in tissue, respectively. The limit of detection of Moxifloxacin on the column (signal to noise ratio 3:1) corresponded to 80 pg Moxifloxacin injected. Due to the much higher concentrations in the specimens, no efforts were made to determine the lower limit.
Determination of vancomycin by HPLC
Vancomycin was assayed by adapting previously published methods (Luksa and Marusic Citation1995, Backes et al. Citation1998). 200 µL plasma was acidified with 200 µL 2.5% orthophosphoric acid, and mixed with 800 µL acetonitrile. After 15 min of incubation at 4°C, the precipitated proteins were separated by centrifugation. The supernatant was extracted with 2 mL dichloromethane, and the mixture was centrifuged again. An aliquot (10 µL) of the upper aqueous layer was injected onto the column. Similarly to the determination of Moxifloxacin, soft tissue (lung, fat, muscle) was homogenized and extracted with 8 volumes (w/v) of 1% ortho-phosphoric acid/acetonitrile (30:70, v/v). Pulverized bone was extracted with 5 volumes of homogenization buffer. The same chromatographic set-up was used as for Moxifloxacin, except for the fluorimetric detector which was replaced by an LC-10 AS photometric detector (Shimadzu, Duisburg, Germany) set at 240 nm. Separation was performed using a Hypersil ODS 3-µm column (internal diameter 200 × 4.6 mm; Bischoff, Leonberg, Germany) and an eluent consisting of 800 mL 50 mM sodium dihydrogen phosphate and 90 ml acetonitrile (adjusted to pH 3.65 with 85% ortho-phosphoric acid). Vancomycin was eluted after 5.1 min (flow rate 1.0 mL/min, column temperature 30°C). The recovery was 100% from plasma, 80% from bone, 80–90% from fat, 70% from lung, and 30% from muscle. Linearity has been proven between 1 and 50 µg/mL by assaying spiked plasma specimens.
For determination of tissue concentrations, calibration curves in duplicate were analyzed with each tissue assay. Bone was spiked with 0.5–10 µg/g whereas lung and muscle were spiked with 1–10 µg/g vancomycin. Due to the small quantity of fat from untreated rats, one-point calibration at 2.5 µg/g was done. Mean intra-assay and interassay precision and accuracy (as deduced from spiked samples) were better than 5%. A total of 3 lung specimens, 1 muscle specimen, and 2 bone specimens were assayed in duplicate. Mean intraassay variation of these real samples was 8.2% (range 0.6–18%). The limit of vancomycin detection corresponded to 330 pg vancomycin applied to the column.
Minimum inhibitory concentration (MIC) of vancomycin and Moxifloxacin
The MICs of vancomycin and Moxifloxacin for the Staphylococcus aureus strain used (ATCC 29213) were 0.5 mg/L and 0.03 mg/L, respectively, as determined by E-test.
Statistics
Statistical analysis was performed with the twotailed paired Student t-test and a p-value of < 0.05 was considered to be statistically significant.
Results
All treated knees showed clear signs of infection, such as pyorrhea after re-arthrotomy, osteomyelitis with pus around the implants, and bony defects. The implants stayed in place. The mean plasma concentrations 2 h after the last administration were 0.34 µg/mL for Moxifloxacin and 3.5 µg/mL for vancomycin (). The corresponding tissue concentrations of Moxifloxacin were higher than in plasma in all tissues investigated, being highest in muscle (2.0 µg/g) and lowest in fat (0.68 µg/g). In contrast, the tissue concentrations of vancomycin exceeded the plasma concentrations only in lung (5.4 µg/g) and in fat (4.4 µg/g). Accordingly, with the exception of fat, the tissue-to-plasma ratio for Moxifloxacin was considerably higher than that for vancomycin (). There were no differences in Moxifloxacin concentration between the infected left hind leg and the uninfected right hind leg, whereas the vancomycin concentrations were significantly higher in the infected leg ().
Figure 3. Tissue to plasma ratio (mean ± SD) of Moxifloxacin concentrations (open columns) and vancomycin concentrations (closed columns) in lung, fat from the groin, muscle, and bone of rats after a 2-week intraperitoneal treatment with Moxifloxacin 10 mg/kg body weight twice daily or vancomycin 15 mg/kg twice daily. Muscle and bone specimens were taken separately from the left (infected) and right hind legs.
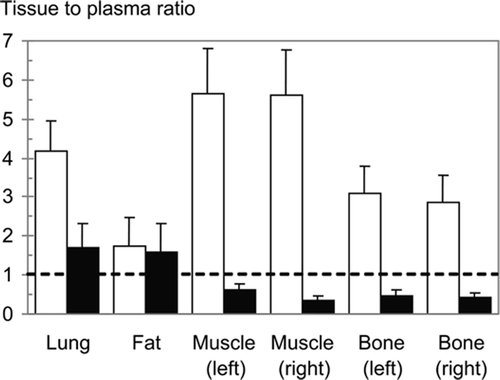
Plasma and tissue concentrations of Moxifloxacin and vancomycin in plasma, lung, fat from the groin, muscle and bone of rats following a 2–week intraperitoneal treatment with Moxifloxacin 10 mg/kg body weight twice daily or vancomycin 15 mg/kg daily. Muscle and bone specimens were taken separately from the left (infected) and right hind legs
Discussion
Experimental models based on rodents are widely acknowledged to be useful in evaluation of the pathogenesis of bacterial infections—including infections around the prosthesis itself—and also different therapeutic strategies. The complexity of the physiological defense mechanisms and reactions cannot be simulated in vitro. Rats have favorable anatomical proportions, which simplifies the surgical procedure described here and also the subsequent bacterial contamination, and enables standardization of the experimental operation. In order to ensure the development of a local infection, the inoculum of 108 cfu/mL Staphylococcus aureus used here was higher than in other experimental models (Blaser et al. Citation1995, Arens et al. Citation1996, Gracia et al. Citation1998, Monzon et al. Citation2002, Vaudaux et al. Citation2002). The local infection rate in the operated femur was 100% and no animal died of sepsis. Our model mimics well the natural development of a periprosthetic infection. The surgical procedure, contamination, the subsequent extraction for analysis, and the analysis of tissue concentrations could all be standardized by the model. The local concentrations of antibiotic in different tissues and their tissue-plasma ratios could be evaluated relatively easily. The selected dosages of Moxifloxacin 10 mg/kg BW and vancomycin 15 mg/kg BW twice daily were based on controlled multiple-dose investigations in guinea pigs with measurement of ciprofloxacin and vancomycin levels after intraperitoneal administration (Blaser et al. Citation1995). In the rat, mean plasma concentrations of 0.35 and 0.25 mg/L were measured 2 h after a single dose of Moxifloxacin at 4.6 mg/kg (intravenously) or 5 mg/kg (orally), respectively (Siefert et al. Citation1999). The half-life of elimination from plasma was approximately 1.5 h, indicating that there would be very little possibility of accumulation in rats after multiple dosing twice daily. The mean plasma concentration of 0.34 mg/L Moxifloxacin 2 h after intraperitoneal administration of 10 mg/kg that we found in our study is in concordance with previously published data, and it is above the minimum inhibitory concentration for the bacterial strain tested.
To our knowledge, no investigation of the penetration of Moxifloxacin in different rat tissues has been published, apart from one autoradiographic study that revealed distinctly higher concentrations of radioactivity in most organs and tissues (Siefert et al. Citation1999). In accordance with its clinical use in controlling respiratory tract infections, analyses of Moxifloxacin in tissue have focused on the lung, where tissue-to-serum ratios of 3–4 have been found in rats (Siefert et al. Citation1999) and in man (Breilh et al. Citation2003). Our data regarding the concentration of Moxifloxacin in the lung (tissue-to-plasma ratio 4:1) confirm these previous results. In addition, the relative tissue-to-plasma-con-centrations of Moxifloxacin in muscle (6:1), lung (4:1), bone (3:1), and fat (2:1) roughly follow the order shown for levofloxacin in tissues of orthopedic patients (von Baum et al. Citation2001). However, the tissue-to-plasma ratios for Moxifloxacin are generally higher than those for levofloxacin due to the higher apparent volume of distribution of the former (Matzke et al. Citation1986, Lew and Waldvogel Citation1999). According to previously poublished results in rats, vancomycin is quickly absorbed following intraperitoneal administration. Mean peak serum concentrations of 35 µg/mL were measured 0.5 h after the last dose of 25 mg/kg BW twice daily for 3 weeks (Haleem et al. Citation2004). However, the plasma half-life of vancomycin in rats in the first 8 h after intravenous injection is short, 1 hour (Mori et al. Citation1998). Thus, the mean vancomycin concentration (3.5 µg/mL) measured in this study 2 h after the last dose is plausible—and is above the MIC for the bacterial strain used. In addition, our data are in line with peak concentrations of 30–35 µg/mL 2 h after single subcutaneous administration of vancomycin at 160 mg/kg BW seen in another study (Catherall et al. Citation1992).
The tissue distribution of vancomycin was different from that of Moxifloxacin. The tissue-to-plasma ratio was generally lower and followed the order: lung < fat < muscle = bone. The concentration of vancomycin in fat tissue was high compared to plasma and highly vascularized muscle. As only limited data are available on the tissue distribution of vancomycin in rats, we must compare our results mainly with results from studies on humans. The tissue concentrations of vancomycin in heart, kidney, liver, and lung have been found to exceed the corresponding serum concentrations (Matzke et al. Citation1986). Concentrations in bone are lower than in serum (Wilson and Mader Citation1984, Graziani et al. Citation1988, Massias et al. Citation1992, Farin et al. Citation1998), and even lower concentrations than in bone have been found in fat (Farin et al. Citation1998). Our data relating to fat differ from these published data. However, all previous data have been obtained from studies using single-dose administration, whereas the rats in our study were treated for a period of 2 weeks before measurement. Thus, we conclude that fat behaves as a deep compartment with slow washout with appreciable accumulation potential for vancomycin. Accordingly, an increased apparent volume distribution of vancomycin has been reported in obese patients (Blouin et al. Citation1982, Bearden and Rodvold Citation2000). Also, higher bone-to-serum ratios of vancomycin were found after multiple dosing (Massias et al. Citation1992) than after single dosing (Wilson and Mader Citation1984, Vuorisalo et al. Citation2000), indicating accumulation of vancomycin also in bone. We cannot, however, exclude a bias due to the intraperitoneal administration. Some of the drug may diffuse directly into the adjacent groin fat and not into the systemic circulation.
There was no difference between the concentration of Moxifloxacin in the infected left leg and the uninfected right leg, whereas the concentration of vancomycin was significantly higher in the infected leg. In accordance with previous results, the concentration in the bone of the infected left knee was significantly higher than in the uninfected right knee (Wilson and Mader Citation1984, Graziani et al. Citation1988). The higher concentration of vancomycin in muscle of the infected leg confirms the results from bone. A plausible explanation would be that the higher concentrations may be due to an increased vascular supply to infected bone and joint, and thus increased delivery of vancomycin to the sampling site (Graziani et al. Citation1988). Furthermore, the tissue environment and milieu (such as pH value and permeability of vessels) may influence the local concentration of amphoteric antibiotics such as vancomycin. Consequently, the lower pH value in infected tissues than in uninfected tissues may have resulted in improved permeation of vessels and accumulation of vancomycin in the infected leg. On the other hand, similar concentrations of Moxifloxacin in the infected leg and the uninfected leg may be explained by the generally much higher tissue concentrations compared to plasma; thus, the influence of vascularization and pH value due to the infection is minor.
It is important to correlate the tissue-concentra-tion findings with clinical findings. As reported, the reduction in bacterial numbers could be evaluated directly by counting the colony-forming units (cfu) on standardized agar plates, and could therefore be directly correlated to the effectiveness of the antibiotic. A significant reduction in cfu could be expected for Moxifloxacin (Kalteis et al. Citation2006). Thus, Moxifloxacin represents a very promising alternative for concomitant antibiotic treatment of osteomyelitis and periprosthetic infections due to oxacillin-sensitive Staphylococcus aureus strains (Kalteis et al. Citation2006). From our findings, it appears that the model of periprosthetic infection used here and the methods we used to correlate tissue concentrations with clinical outcome can be applied to infections with various bacterial and mycotic pathogens, as well as to different antibiotics or therapeutic regimes. Further studies will be needed to find out the most effective treatment regimen.
We thank the keepers of the animals, the laboratory assistants of the Dept. of Pharmacology, and Dr. H. Linde and the laboratory assistants at the Department of Microbiology, University of Regensburg, for their excellent uncomplicated interinstitutional work. We also thank Bayer Vital GmbH, as the study was funded by an unrestricted research grant from this company.
Contributions of authors
JB: wrote the manuscript and carried out the study (surgery, processing, X-ray, photography). FK: was responsible for the pharmacological parts. JS and TK: helped carry out the study. NL: provided the microbiological strains. JG: supervised the study as head of the Orthopedic Clinic. KL: initiated the study and helped carry it out. The manuscript was proofread by all authors.
- Arens S, Schlegel U, Printzen G, Ziegler W J, Perren S M, Hansis M. Influence of materials for fixation implants on local infection. An experimental study of steel versus titanium DCP in rabbits. J Bone Joint Surg (Br) 1996; 78: 647–51
- Backes D W, Aboleneen H I, Simpson J A. Quantitation of vancomycin and its crystalline degradation product (CDP1) in human serum by high performance liquid chromatography. J Pharm Biomed Anal 1998; 16: 1281–7
- Bearden D T, Rodvold K A. Dosage adjustments for antibacterials in obese patients: applying clinical pharmacokinetics. Clin Pharmacokinet 2000; 38: 415–26
- Blaser J, Vergeres P, Widmer A F, Zimmerli W. In vivo verification of in vitro model of antibiotic treatment of device-related infection. Antimicrob Agents Chemother 1995; 39: 1134–9
- Blouin R A, Bauer L A, Miller D D, Record K E, Griffen W O, Jr. Vancomycin pharmacokinetics in normal and morbidly obese subjects. Antimicrob Agents Chemother 1982; 21: 575–80
- Breilh D, Jougon J, Djabarouti S, Gordien J B, Xuereb F, Velly J F, Arvis P, Landreau V, Saux M C. Diffusion of oral and intravenous 400 mg once-daily Moxifloxacin into lung tissue at pharmacokinetic steady-state. J Chemother 2003; 15: 558–62
- Catherall E J, Gillon V, Hurn S, Irwin R, Mizen L. Efficacy of ticarcillin-clavulanic acid for treatment of experimental Staphylococcus aureus endocarditis in rats. Antimicrob Agents Chemother 1992; 36: 458–62
- Dalhoff A. Pharmacodynamics of fluoroquinolones. J Antimicrob Chemother 1999; 43(Suppl B)51–9
- Dalhoff A, Schmitz F J. In vitro antibacterial activity and pharmacodynamics of new quinolones. Eur J Clin Microbiol Infect Dis 2003; 22: 203–21
- Della Valle C J, Scher D M, Kim Y H, Oxley C M, Desai P, Zuckerman J D, Di Cesare P E. The role of intraoperative Gram stain in revision total joint arthroplasty. J Arthroplasty 1999; 14: 500–4
- Drobny T K, Munzinger U. Problems of infected knee prosthesis. Orthopade 1991; 20: 239–43
- Eveillard M, Mertl P, Canarelli B, Lavenne J, Fave M H, Eb F, Vives P. Risk of deep infection in first-intention total hip replacement. Evaluation concerning a continuous series of 790 cases. Presse Med 2001; 30: 1868–75
- Farin D, Piva G A, Gozlan I, Kitzes-Cohen R. A modified HPLC method for the determination of vancomycin in plasma and tissues and comparison to FPIA (TDX). J Pharm Biomed Anal 1998; 18: 367–72
- Gracia E, Lacleriga A, Monzon M, Leiva J, Oteiza C, Amorena B. Application of a rat osteomyelitis model to compare in vivo and in vitro the antibiotic efficacy against bacteria with high capacity to form biofilms. J Surg Res 1998; 79: 146–53
- Graziani A L, Lawson L A, Gibson G A, Steinberg M A, MacGregor R R. Vancomycin concentrations in infected and noninfected human bone. Antimicrob Agents Chemother 1988; 32: 1320–2
- Haleem A A, Rouse M S, Lewallen D G, Hanssen A D, Steckelberg J M, Patel R. Gentamicin and vancomycin do not impair experimental fracture healing. Clin Orthop 2004, 427: 22–4
- Hoogkamp-Korstanje J A, Roelofs-Willemse J. Comparative in vitro activity of Moxifloxacin against Gram-positive clinical isolates. J Antimicrob Chemother 2000; 45: 31–9
- Ince D, Zhang X, Hooper D C. Activity of and resistance to Moxifloxacin in Staphylococcus aureus. Antimicrob Agents Chemother 2003; 47: 1410–5
- Isiklar Z U, Demirors H, Akpinar S, Tandogan R N, Alparslan M. Two-stage treatment of chronic staphylococcal orthopaedic implant-related infections using vancomycin impregnated PMMA spacer and rifampin containing antibiotic protocol. Bull Hosp Jt Dis 1999; 58: 79–85
- Kaatz G W, Moudgal V V, Seo S M. Identification and characterization of a novel efflux-related multidrug resistance phenotype in Staphylococcus aureus. J Antimicrob Chemother 2002; 50: 833–8
- Kalteis T, Beckmann J, Schroder H J, Handel M, Grifka J, Lehn N, Lerch K. Moxifloxacin superior to vancomycin for treatment of bone infections--a study in rats. Acta Orthop Scand 2006; 77: 315–9
- Kees F, Naber K G, Schumacher H, Grobecker H. Penetration of fleroxacin into prostatic secretion and prostatic adenoma tissue. Chemotherapy 1988; 34: 437–43
- Kordelle J, Frommelt L, Kluber D, Seemann K. [Results of one-stage endoprosthesis revision in periprosthetic infection cause by methicillin-resistant Staphylococcus aureus]. Ergebnisse einzeitiger Endoprothesenwechsel bei periprothetischer Infektion durch einen methicillinresistenten Staphylococcus aureus (MRSA). Z Orthop Ihre Grenzgeb 2000; 138(3)240–4
- Lew D P, Waldvogel F A. Use of quinolones in osteomyelitis and infected orthopaedic prosthesis. Drugs 1999; 58(Suppl 2)85–91
- Luksa J, Marusic A. Rapid high-performance liquid chromatographic determination of vancomycin in human plasma. J Chromatogr B Biomed Appl 1995; 667: 277–81
- Massias L, Dubois C, de Lentdecker P, Brodaty O, Fischler M, Farinotti R. Penetration of vancomycin in uninfected sternal bone. Antimicrob Agents Chemother 1992; 36: 2539–41
- Matzke G R, Zhanel G G, Guay D R. Clinical pharmacokinetics of vancomycin. Clin Pharmacokinet 1986; 11: 257–82
- Monzon M, Garcia-Alvarez F, Lacleriga A, Amorena B. Evaluation of four experimental osteomyelitis infection models by using precolonized implants and bacterial suspensions. Acta Orthop Scand 2002; 73: 11–9
- Mori H, Nakajima T, Nakayama A, Yamori M, Izushi F, Gomita Y. Interaction between levofloxacin and vancomycin in rats--study of serum and organ levels. Chemotherapy 1998; 44: 181–9
- Nguyen L L, Nelson C L, Saccente M, Smeltzer M S, Wassell D L, McLaren S G. Detecting bacterial colonization of implanted orthopaedic devices by ultrasonication. Clin Orthop 2002, 403: 29–37
- Puolakka T J, Pajamaki K J, Halonen P J, Pulkkinen P O, Paavolainen P, Nevalainen J K. The Finnish Arthroplasty Register: report of the hip register. Acta Orthop Scand 2001; 72: 433–41
- Segawa H, Tsukayama D T, Kyle R F, Becker D A, Gustilo R B. Infection after total knee arthroplasty. A retrospective study of the treatment of eighty-one infections. J Bone Joint Surg (Am) 1999; 81: 1434–45
- Sharkey P F, Hozack W J, Rothman R H, Shastri S, Jacoby S M. Insall Award paper. Why are total knee arthroplasties failing today?. Clin Orthop 2002, 404: 7–13
- Siefert H M, Kohlsdorfer C, Steinke W, Witt A. Pharmacokinetics of the 8-methoxyquinolone, Moxifloxacin: tissue distribution in male rats. J Antimicrob Chemother 1999; 43(Suppl B)61–7
- Silva M, Tharani R, Schmalzried T P. Results of direct exchange or debridement of the infected total knee arthroplasty. Clin Orthop 2002, 404: 125–31
- Spangehl M J, Masri B A, O’Connell J X, Duncan C P. Prospective analysis of preoperative and intraoperative investigations for the diagnosis of infection at the sites of two hundred and two revision total hip arthroplasties. J Bone Joint Surg (Am) 1999a; 81: 672–83
- Spangehl M J, Masterson E, Masri B A, O’Connell J X, Duncan C P. The role of intraoperative gram stain in the diagnosis of infection during revision total hip arthroplasty. J Arthroplasty 1999b; 14: 952–6
- Vaudaux P, Francois P, Bisognano C, Schrenzel J, Lew D P. Comparison of levofloxacin, alatrofloxacin, and vancomycin for prophylaxis and treatment of experimental foreign-body-associated infection by methicillin-resistant Staphylococcus aureus. Antimicrob Agents Chemother 2002; 46: 1503–9
- von Baum H, Bottcher S, Abel R, Gerner H J, Sonntag H G. Tissue and serum concentrations of levofloxacin in orthopaedic patients. Int J Antimicrob Agents 2001; 18: 335–40
- Vuorisalo S, Pokela R, Satta J, Syrjala H. Internal mammary artery harvesting and antibiotic concentrations in sternal bone during coronary artery bypass. Int J Angiology 2000; 9: 78–81
- Wilson K J, Mader J T. Concentrations of vancomycin in bone and serum of normal rabbits and those with osteomyelitis. Antimicrob Agents Chemother 1984; 25: 140–1