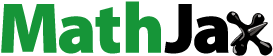
ABSTRACT
This study was to investigate the antioxidant activity of Komagataeibacter hansenii CGMCC 3917 in vivo and in vitro. In vitro study suggested that CGMCC 3917 exhibited strong scavenging abilities against DPPH, ABTS, OH free radicals and reducing power, suggesting significant anti-oxidant ability. In vivo supplementation of mice with CGMCC 3917 bacteria suspension at a dose of 10 mL/kg (108 or 106 CFUs/mL) dramatically decreased the elevated serum activities of aspartate aminotransferase (AST) and alanine aminotransferase (ALT) induced by alcohol treatment in mice (p< 0.01), and also significantly decreased the elevated levels of alkaline phosphatase (ALP) and hepatic malondialdehyde (MDA) induced by alcohol treatment in mice (p< 0.01). Furthermore, CGMCC 3917 supplement showed the preventive effects against the alcohol-induced elevation in serum triglycerides (TG) and low-density lipoprotein cholesterol (LDL-C), and the decrease in high-density lipoprotein cholesterol (HDL-C), as well as the decreased activities of glutathione peroxidase (GSH-Px) and superoxide dismutase (SOD) in mice. Additionally, supplementation with CGMCC 3917 displayed significant anti-inflammatory effects by lowering hepatic production of TNF-α, IL-1, and IL-6 in alcohol-treated mice. All these findings suggest that K. hansenii CGMCC 3917 may be a great potential nutritional resource against chronic alcohol-induced liver oxidative injury in mice.
Resumen
El presente estudio se propuso investigar la actividad antioxidante de Komagataeibacter hansenii CGMCC 3917 in vivo e in vitro. El estudio in vitro indicó que CGMCC 3917 exhibe una fuerte capacidad de eliminación de radicales DPPH, ABTS y OH, además de poder reductor. Ello sugiere que posee una significativa capacidad antioxidante. La suplementación in vivo de ratones tratados con alcohol con suspensión de bacterias CGMCC 3917 a una dosis de 10 ml/kg (108 o 106 UFC/ml) disminuyó drásticamente la elevada actividad sérica de la aspartato aminotransferasa (AST) y la alanina aminotransferasa (ALT) inducida por el tratamiento con alcohol (p<0.01). Además, redujo significativamente los niveles elevados de fosfatasa alcalina (ALP) y malondialdehído hepático (MDA) inducidos por el tratamiento con alcohol (p<0.01). Por otra parte, el suplemento CGMCC 3917 administrado a ratones mostró efectos preventivos de la elevación de triglicéridos (TG) en el suero, así como del colesterol de lipoproteínas de baja densidad (LDL-C), y de la disminución del colesterol de lipoproteínas de alta densidad (HDL-C), y de las actividades de la glutatión peroxidasa (GSH-Px) y la superóxido dismutasa (SOD) inducidas por el alcohol. Además, la suplementación con CGMCC 3917 mostró efectos antiinflamatorios significativos al disminuir la producción hepática de TNF-α, IL-1 e IL-6 en ratones tratados con alcohol. Estos hallazgos sugieren que K. hansenii CGMCC 3917 puede ser potencialmente un gran recurso nutricional contra la lesión oxidativa crónica del hígado de ratones inducida por alcohol.
1. Introduction
Liver disease has been subdivided into alcoholic liver disease (ALD) and nonalcoholic liver disease (NAFLD) according to its cause (Kim, Brown, Terrault, & El Serag, Citation2002; Yeh & Brunt, Citation2014). For the ALD, alcoholic abuse has been identified as one of the main determinants (Lu et al., Citation2015; Parry, Patra, & Rehm, Citation2011). It has been demonstrated that long-term alcohol intake induces hepatic steatohepatitis, followed by more severe lesions such as hepatic fibrosis and cirrhosis (Louvet & Mathurin, Citation2015). One of the most important pathologic mechanisms involved in ALD is the production of oxidative alcohol metabolites because alcohol can induce oxidative stress, lipid peroxidation, and free radicals (Dey & Cederbaum, Citation2006; Ghosh, Guria, & Das, Citation2016; Meagher et al., Citation1999; Nordmann, Ribière, & Rouach, Citation1992). To enhance hepatic antioxidant capacity and reduce alcoholic liver injury, a variety of synthetic liver-protection drugs have been widely used. However, the use of these drugs is limited because of their side-effects (Rao, Rao, Pushpangadan, & Shirwaikar, Citation2006). Therefore, it is of high significance to explore new, safe and effective natural antioxidants for alcohol consumers to prevent or slowdown the progression of ALD.
Recently, studies have reported that probiotics play an important role in the prevention of ALD in living organism (Kirpich & McClain, Citation2012; Shi et al., Citation2015; Zhao et al., Citation2015). Accumulating evidences has suggested that natural microorganisms exert various biological roles through several mechanisms, and their main function is the prevention of oxidative stress and related diseases such as ALD (Amaretti et al., Citation2013; Bullotterson et al., Citation2013). Potentiality of probiotics for exploitation as functional foods has also been reported. The probiotics and probiotic products are widely used in promoting human health and adjunctive therapy of human disease (Kirpich et al., Citation2008; Okoń, Stadnik, & Dolatowski, Citation2017). The protective mechanism may be explained on the basis of their antioxidant activities, radicals scavenging capacities, inhibiting DNA and RNA synthesis, anti-inflammation, or inhibiting the viral reproduction, etc. (Kirpich & McClain, Citation2012; Zhao et al., Citation2015). Therefore, there is an increasing number of studies trying to seek for safe probiotic bacteria against ALD. Komagataeibacter genus (formerly Gluconacetobacter genus) belonging to the acetic acid bacteria are known for their high acetic acid resistance, which have a wide range of use in the food industry (Barja, Andrés-Barrao., Pérez, Cabello, & Chappuis, Citation2016; Ge et al., Citation2011). Symbiosis of Komagataeibacter strains and fungi with bacterial cellulose (BC) is well known as traditional anti-alcoholism beverage in South Asia (Dufresne & Farnworth, Citation2000; Sengun & Karabiyikli, Citation2011). The strain CGMCC 3917 belongs to the family of Komagataeibacter, which was first isolated from homemade persimmon vinegar with high genetic stability and strong ability to produce BC (Lin, Li, Lopez-Sanchez, & Li, Citation2015). Additionally, K. hansenii CGMCC 3917 has a great ability to oxidize alcohols to acetic acid, which possesses potential in the field of functional foods for its edibleness and fermentation use (Ge et al., Citation2011; Lin et al., Citation2015). Several studies have reported that fermentation products (e.g. polysaccharides) from Komagataeibacter genus showed antioxidant activity in vitro (Ahmed et al., Citation2014; Srikanth et al., Citation2015). However, little is known about the antioxidant activity of K. hansenii CGMCC 3917 cells. It is also unclear whether cell components or compounds of K. hansenii CGMCC 3917 can ameliorate alcohol-induced liver injury including oxidative stress, hyperpermeability, and subsequent alcoholic steatohepatitis.
Hence, the present study aims to explore the antioxidant properties of K. hansenii CGMCC 3917 in vitro and in vivo. The radicals scavenging abilities against 1,1-diphenyl-2-picrylhydrazyl (DPPH), 2,2‘-azino-bis-3-ethylbenzthiazoline-6-sulphonic acid (ABTS), hydroxyl (OH) free radicals and reducing power were determined in vitro. Meanwhile a mouse model of chronic alcohol consumption in vivo was employed and correlative parameters of hepatic inflammatory cytokines and alcohol-induced oxidative stress were measured to investigate the protective effects of K. hansenii CGMCC 3917 against chronic alcohol-induced liver injury in mice.
2. Materials and methods
2.1. Bacterial strain and culture conditions
Bacterial strain (Komagataeibacter hansenii CGMCC 3917) used in this study, donated by Fermentation Technology Innovation Laboratory of Northwest A&F University (Yanglin, Shaanxi, China), was cultured at 30°C under stirred conditions in a fermentation medium (pH 5.0) containing glucose 2% (w/v), yeast extract 0.5% (w/v), KH2PO4 0.1% (w/v), MgSO4 ·7H2O 1.5% (w/v), and ethanol 2% (v/v (Ge et al., Citation2011)). All stock solutions were prepared with doubly distilled deionized water. The strain was activated in 250 mL Erlenmeyer flasks containing 100 mL of fermentation medium and stirred at 30°C in a rotary shaker incubator (150 ×g). Growth in liquid media was monitored by measuring the turbidity of the bacterial suspension at OD600 (Chen et al., Citation2012). After cultivation, bacteria were collected by centrifugation at 5000 ×g for 10 min, and then washed three times with 0.9% saline solution to prepare the required dose for in vitro and in vivo studies (Su et al., Citation2015). The concentration of bacteria suspension was quantified by counting colony forming units (CFUs) after plating serial dilutions of bacteria suspension onto fermentation medium with 1.7% agar (w/v (Montealegre, Singh, & Murray, Citation2016)).
2.2. Measurement of antioxidant properties in vitro
The antioxidant properties of K. hansenii CGMCC 3917 were determined by measuring free radicals-scavenging activities and total reducing power. Briefly, bacteria for the in vitro studies were grown on fermentation medium at 30°C for 45 h, suspended in sterile 0.9% saline, and adjusted to a concentration of 2 ×108 CFUs/mL (Su et al., Citation2015). Physical fragmentation of the strain CGMCC 3917 was used as the comparison of antioxidant activity with intact bacterial cells. The bacteria were disrupted using an ultra-high pressure homogenizer (F6/10-10G, Fluko, Shanghai, China) and centrifugated at 6000 ×g for 10 min (Su et al., Citation2015). For DPPH radical scavenging activity, a volume of 2.0 mL of bacterial suspension with varying concentrations (0–108 CFUs/mL) was mixed with 1.0 mL of DPPH (0.2 mM in absolute ethanol) and incubated at 30 ± 2°C for 30 min. The absorbance was determined at 517 nm (Floegel, Kim, Chung, Koo, & Chun, Citation2011). The ABTS radical-scavenging ability assay was based on the generation of a blue or green ABTS radical that can be reduced by antioxidants. A quantity of 5 mL of blue-green ABTS solution (7 mM in distilled water) was mixed with 88 µL of potassium persulphate solution (140 mM in distilled water) and diluted with anhydrous ethanol solution for ABTS working solution. A quantity of 1 mL of bacterial suspension with different concentrations (0–108 CFUs/mL) was mixed with 2 mL of ABTS working solution (0.2 mM). Absorbance at 734 nm was then determined after 5 min (Kozłowska, Ścibisz, Zaręba, & Ziarno, Citation2015; Müller, Fröhlich, & Böhm, Citation2011). The OH radical was assigned after generation by a Fenton-type reaction. The reaction mixture included 1 mL FeSO4 (2.5 mM), 1 mL 1,10-phenanthroline (2.5 mM, Zhengzhou Sigma Chemical Co., Ltd., China), 1 mL carbonate buffer (pH 7.4), and 1 mL H2O2 (20 mM). The reaction system was then kept for 30 min at 37°C in a water bath. Absorbance of the mixture was determined at 536 nm versus a prepared water blank (Li, Zhang, & Ma, Citation2011). The reducing power of the test bacterial samples was determined using Negi’s method (Negi & Jayaprakasha, Citation2003). The intact bacterial cells and fragmented bacteria suspensions (1 mL) with various concentrations (0–108 CFUs/mL) were mixed with 1 mL of phosphate buffer (0.2 mM, pH 6.6) and 1 mL of 1% potassium ferricyanide in a 10 mL test tubes. The mixtures were incubated for 20 min at 50°C. A quantity of 0.5 mL trichloroacetic acid (10%, w/v) was added to the mixtures and centrifuged at 3000 ×g for 10 min until the centrifugation was over. The upper layer (1 mL) was mixed with 1 mL of ferric chloride (0.1%, w/v) to incubate for 10 min subsequently, and the absorbance was measured at 700 nm. The increase in absorbance of the reaction indicates the reducing power of the samples, and total reducing power was measured by its absorbance at 700 nm (Negi & Jayaprakasha, Citation2003). Measurement of DPPH, ABTS and the OH radicals-scavenging activity (RSA) was calculated using the following formula.
The total reducing power (TRP) was calculated using U as the equivalent amount (OD at 700 nm). 1U/mL, by definition, is the unit when the molar attenuation coefficient was increased 0.01 by per mL sample at 30°C.
where = final volume make-up of the sample, mL.
= volume of the sample, mL.
= volume of bacterial samples before dilution, mL.
2.3. Animals and treatments
Male Kunming mice (18–22 g) were purchased from Laboratory Animal Center of Xi’an Jiaotong University, China. All mice received humane care in compliance with institutional guidelines to minimize animal suffering. The whole experiment was carried out complying with the Guidelines of Experimental Animal Administration published by the State Committee of Science and Technology of the People’s Republic of China.
Mice were housed in individually ventilated cage (IVC) system in a controlled environment (22 ± 2°C, humidity of 60 ± 5%, and 12 h light/dark cycle) and allowed free access to water and standard lab chow. After 1-week acclimation, all mice were randomly divided into four groups with 10 mice in each group. Group Ⅰ (n =10, normal group): mice were treated by gavage with normal saline at a dose of 10 mL/kg twice a day with an interval under 2 h. Group Ⅱ (n =10, alcohol-treated group): mice received 28% (v/v) ethanol (10 mL/kg BW) 2 h after normal saline by intragastric gavage (10 mL/kg BW). Groups III (n =10) and IV (n =10) are dose-dependent test groups, where mice were administered by intragastric gavage with 28% (v/v) ethanol (10 mL/kg BW) 2 h after administration of CGMCC 3917 bacterial suspension by intragastric gavage at a dose of 10 mL/kg BW (Bati, Celik, & Dogan, Citation2014). The concentrations of bacterial suspension were approximately 2 ×108 (high-dose group) and 2 ×106 CFUs (low-dose group), respectively. Mice were administrated every day and weighed weekly. All the administration was conducted for 10 weeks. All the experiments were executed on the basis of the Guidelines of Experimental Animal Administration published by the State Committee of Science and Technology of the People’s Republic of China.
2.4. Biological samples collection
After the administration described above, all the mice were fasted and only allowed free access to water for 12 h, and then anesthetized by the inhalation of isoflurane and sacrificed to collect blood and tissues. Blood was centrifuged at 3000 ×g for 15 min to collect the serum and then stored at −80°C for the biochemical analysis (Yu et al., Citation2017). The isolated tissues including abdominal fat and the liver were weighed and then stored at −80°C for subsequent evaluations of oxidative stress, biochemical and molecular assay. All the experiments were executed on the basis of the Guidelines of Experimental Animal Administration published by the State Committee of Science and Technology of the People’s Republic of China.
2.5. Measurement of serum protein and aminotransferase levels
Activities of serum alanine transaminase (ALT), aspartate aminotransferase (AST), and alkaline phosphatase (ALP) were analyzed by the corresponding commercial spectrophotometric kits according to the manufacturer’s instructions, respectively. Serum albumin (ALB) and total protein (TP) were also evaluated in an automated microplate reader device (Biotek Instruments, Inc., USA) using the corresponding commercial spectrophotometric kits, respectively. All commercial kits used here were purchased from Nanjing Jiancheng Bioengineering Institute (Nanjing, China).
2.6. Measurement of serum triglycerides, high-density lipoprotein, and low-density lipoprotein
Serum triglyceride (TG), low-density lipoprotein cholesterol (LDL-C) and high-density lipoprotein cholesterol (HDL-C) levels in mice were quantitatively determined by the corresponding commercial Kits (Nanjing Jiancheng Bioengineering Institute, Nanjing, China) according to the manufacturer’s protocols.
2.7. Histological assay
Liver tissues collected from four groups of mice were fixed with 10% formalin for 48 h at 4°C, embedded in paraffin wax and sectioned into 5 μm thick slices. Then, all the samples were stained with hematoxylin and eosin (H&E, Sigma-Aldrich Co.) or oil red O (Ding et al., Citation2015; Hsu et al., Citation2017). Morphological features of all samples were captured by light microscopy.
2.8. Determination of hepatic MDA, SOD and GSH-Px levels
Liver tissues were homogenized in nine volumes of cold saline solution and then centrifuged at 3000 ×g and 4°C for 15 min. Measurements of hepatic superoxide dismutase (SOD) and glutathione (GSH-Px) activities and malondialdehyde (MDA) levels were analyzed by commercial kits (Nanjing Jiancheng Bioengineering Institute, Nanjing, China) according to the manufacturer’s instructions.
2.9. Assay for hepatic cytokines
The concentrations of hepatic IL-1, IL-6 and TNF-α were measured using ELISA kits purchased from Nanjing Jiancheng Bioengineering Institute (Nanjing, China). Briefly, 40 µL and 100 µL of diluted HRP-conjugate reagent was added to 10 µL sample in each well, and then samples were covered with an adhesive strip and incubated for 60 min at 37°C, followed by washing. After that, the chromogen solutions were added before 50 µL stop solution to each well for 15 min at 37°C (Wang et al., Citation2013). The values were normalized to total protein and expressed as pg/mg of protein.
2.10. Statistical analysis
All data are expressed as mean±standard deviation (SD). Statistical evaluation of the results was performed using Analysis of Co-variance (ANCOVA) followed by Fisher’s LSD (SPSS 20.0, IBM). Differences between groups were considered significant at p<0.05. The significant difference was stressed with symbols as shown in Figureures.
3. Results and discussion
3.1. Antioxidant activities of strain CGMCC 3917 in vitro
The antioxidant activity of strain CGMCC 3917 was investigated by in vitro assay of scavenging activities against DPPH, ABTS, HO free radicals, and the total reducing power, where both intact and fragmented bacteria cells were prepared at a concentration of 108 CFUs/mL. As shown in , the intact bacteria cells displayed higher DPPH scavenging activity (23.94%) than fragmented cells (21.11%, p<0.01), which was consistent with the previous research on antioxidant properties of wine lactic acid bacteria (Su et al., Citation2015). For ABTS scavenging capacity, the intact cells showed a higher effect than the fragmented bacteria (p<0.05). Similarly, CGMCC 3917 with intact bacterial cells at the tested concentrations (108 CFUs/mL) exhibited higher total reducing power with the value of 2.68 U/mg than fragmented microorganisms (2.18 U/mg, p<0.01). In terms of HO radicals scavenging capacity, CGMCC 3917 with intact bacterial cells displayed slightly lower scavenging activity than fragmented bacteria cells (p<0.05). On the basis of the results, intact bacterial cells of strain CGMCC 3917 were selected for subsequent animal experiments to investigate the antioxidant capacity and protective effect on alcohol-induced liver injury in mice. The in vitro investigation indicates that both intact and fragmented bacteria cells of CGMCC 3917 exhibited antioxidant properties. Epidemiological data and randomized clinical trials provide abundant indications that many nonenzymatic antioxidants play a fundamental role in the prevention of oxidative stress and related diseases (Yin et al., Citation2012). Excessive studies have reported the development of probiotics exerting antioxidant activity and counteracting the oxidative stress. (Amaretti et al., Citation2013) Probiotic strains could protect against oxidative stress, decrease the risk of the accumulation of reactive oxygen species (ROS), such as the selected strains of lactobacilli and bifidobacteria which could present antioxidative properties (Amaretti et al., Citation2013; Kullisaar et al., Citation2002; Lin & Yen, Citation1999; Shimamura et al., Citation1992; Spyropoulos, Misiakos, Fotiadis, & Stoidis, Citation2011). Results of in vitro measurement reveal that CGMCC 3917 may act as scavengers of ROS and metal chelators that protect human cells and reduce oxidative damages.
Table 1. In vitro antioxidant effects of unprocessed CGMCC 3917 and fragmented bacteria. *p< 0.05, **p< 0.01 indicated statistically significant difference.
Tabla 1. Efectos antioxidantes in vitro de CGMCC 3917 sin procesar y bacterias fragmentadas. * p< 0.05, ** p< 0.01 indica una diferencia estadísticamente significativa.
3.2. Effects of strain CGMCC 3917 on body weight, liver weight, and liver index
The body weight, liver weight and liver index (liver weight/body weight, %) were compared among the four groups. As depicted in , the body weight in the ethanol-treated mice was slightly lower compared to that in the untreated normal mice (p>0.05). Administration with both high-dose and low-dose of bacterial suspension did not affect animal body weight and liver weight in comparison with the normal mice (p>0.05). However, the liver index of ethanol-treated mice was increased by 21.1% in comparison with that of the normal mice (p<0.01), suggesting that alcohol exposure for 10 weeks caused the lipid accumulation in the liver. Interestingly, this increase in the liver index of the ethanol-treated mice was prevented by supplementation with bacteria suspension of CGMCC 3917, especially the high-dose bacteria suspension (108 CFUs/mL, p<0.01).
Table 2. Body weight, liver weight and liver index of mice submitted to ethanol model and treated with vehicle or CGMCC 3917. #p < 0.05 indicated statistically significant difference with normal mice. *p < 0.05, **p < 0.01 indicated statistically significant difference with ethanol mice.
Tabla 2. Peso corporal, peso del hígado e índice hepático de ratones sometidos al tratamiento con etanol y tratados con vehículo o CGMCC 3917. #p< 0.05 indica una diferencia estadísticamente significativa en comparación con ratones normales. * p< 0.05, ** p< 0.01 indica una diferencia estadísticamente significativa en comparación con ratones tratados con etanol.
3.3. Effects of strain CGMCC 3917 on serum aminotransferase activities in mice
The activities of AST, ALT, and ALP in the serum are considered as reliable and sensitive indicators of ALD (Bati et al., Citation2014; Uyanoglu et al., Citation2014). AST and ALT are enzymes present in hepatocytes, and these intracellular enzymes are released into the circulation when injury is induced in the liver (Wang et al., Citation2014). As presented in , serum AST, ALT and ALP activities in ethanol-treated mice were dramatically elevated by 37.7%, 58.04% and 33.10% in comparison with those in normal mice, respectively (p<0.01), indicating that ethanol gavage for 10 weeks induced hepatotoxicity in mice. However, it was apparent that supplementation with suspensions of bacteria at either low-dose or high-dose was effective in suppressing the chronic alcohol-induced liver injury by decreasing the levels of serum AST, ALT and ALP activities in ethanol-administrated mice (p<0.05, p<0.01). For protein assay, as shown in , ethanol gavage for 10 weeks resulted in a substantial reduction in the serum TP levels (, p<0.01), and caused a 0.8-fold decrease in serum ALB levels, relative to the normal mice (, p<0.01). However, a significant elevation in the serum TP and ALB levels of mice was achieved by treatment with CGMCC 3917 at two tested doses compared to those of ethanol-administrated mice (p<0.01). The present results show that based on the evaluation of serum protein and aminotransferases, intragastrical administration with CGMCC 3917 significantly inhibited the alcohol-elevated increases in the serum AST, ALT and ALP activities, suggesting that daily administration with bacteria suspension of CGMCC 3917 (10 mL/kg) for 10 weeks ameliorated hepatic tissue damage caused by alcohol. This finding demonstrated that CGMCC 3917 may prevent liver damage caused by alcohol ingestion via inhibiting hepatocyte damage in mice.
Figure 1. Effects of CGMCC 3917 on the levels of the serum (a) AST, (b) ALT, (c) ALP. Groups are normal, ethanol, ethanol+CGMCC 3917 (108 CFUs/mL), ethanol+ CGMCC 3917 (106 CFUs/mL), respectively. Values are expressed as means ± SDs for 10 mice. ##p< 0.01, compared to the normal mice. *p< 0.05 and **p< 0.01, compared to the ethanol-treated mice, respectively.
Figura 1. Efectos de CGMCC 3917 en los niveles de suero (a) AST, (b) ALT, (c) ALP. Los grupos son normal, etanol, etanol + CGMCC 3917 (108 UFC/ml), etanol + CGMCC 3917 (106 UFC/ml), respectivamente. Los valores indican medias ± DE para 10 ratones. ## p< 0.01, en comparación con los ratones normales. * p< 0.05 y ** p< 0.01, en comparación con los ratones tratados con etanol, respectivamente.

Figure 2. Effects of CGMCC 3917 on the levels of the serum (a) TP, (b) ALB. Groups are normal, ethanol, ethanol+CGMCC 3917 (108 CFUs/mL), ethanol+CGMCC 3917 (106 CFUs/mL), respectively. Values are expressed as means ± SDs for 10 mice. ##p< 0.01, compared to the normal mice, **p< 0.01, compared to the ethanol-treated mice.
Figura 2. Efectos de CGMCC 3917 en los niveles de suero (a) TP, (b) ALB. Los grupos son normal, etanol, etanol + CGMCC 3917 (108 UFC/ml), etanol + CGMCC 3917 (106 UFC/ml), respectivamente. Los valores expresan medias ± DE para diez ratones. ## p< 0.01, en comparación con los ratones normales, ** p < 0.01, en comparación con los ratones tratados con etanol.

3.4. Strain CGMCC 3917 prevented chronic alcohol-induced lipid accumulation in mice
The levels of serum TG, HDL-C, and LDL-C in mice were measured as present in . Prominent elevation of TG and LDL-C levels and significant reduction of HDL-C level in ethanol-administrated mice were clearly observed in comparison with those in the untreated normal mice (p<0.01). However, the elevated TG and LDL-cholesterol levels in the ethanol-treated mice were dramatically decreased in the mice treated with CGMCC 3917 at 108 CFUs/mL or 106 CFUs/mL for 10 weeks ( and , p<0.05), and the reduced HDL-C level in the ethanol-treated mice was increased in the mice supplemented with bacteria suspensions of CGTMCC 3917 at 108 CFUs/mL or 106 CFUs/mL for 10 weeks (p<0.05, ).
Figure 3. Effects of CGMCC 3917 on the levels of the serum (a) TG, (b) HDL-C, (c) LDL-C. Groups are normal, ethanol, ethanol+CGMCC 3917 (108 CFUs/mL), ethanol+CGMCC 3917 (106 CFUs/mL), respectively. Values are expressed as means ± SDs for 10 mice. ##p< 0.01, compared to the normal mice. *p< 0.05 and **p< 0.01, compared to the ethanol-treated mice, respectively.
Figura 3. Efectos de CGMCC 3917 en los niveles de suero (a) TG, (b) HDL-C, (c) LDL-C. Los grupos son normal, etanol, etanol + CGMCC 3917 (108 UFC/ml), etanol + CGMCC 3917 (106 UFC/ml), respectivamente. Los valores expresan medias ± DE para 10 ratones. ## p< 0.01, en comparación con ratones normales. * p< 0.05 y ** p< 0.01, en comparación con ratones tratados con etanol, respectivamente.

Hepatic steatosis, characterized by excessive lipid accumulation in the cytoplasm of hepatocytes, is accelerated by the alcohol consumption from simple fatty liver steatosis to more severe liver pathologies (Carr, Dhir, Yin, Agarwal, & Ahima, Citation2013). Hepatic steatosis is an early pathologic feature of ALD, and most common response of the liver to acute or chronic alcohol exposure (Raynard et al., Citation2002). With the development of ALD, the excessive lipids dominate the cytoplasmic space of the hepatocytes, gradually progress to affect cellular function, break the balance of lipid synthesis and degradation (Carr et al., Citation2013; Raynard et al., Citation2002). In order to confirm the effect of bacteria suspension of CGMCC3917 on hepatic steatosis induced by chronic alcohol intake, histopathological morphologies of the livers were observed using H&E staining and oil red O staining. In ethanol-administrated mice, liver sections stained with hematoxylin–eosin revealed severe parenchymal disarrangement, such as lobular inflammation, hepatocellular swelling and macrovesicular necrosis, massive fatty changes, and the loss of cellular boundaries as compared with the normal mice (). However, the damage of hepatocyte morphology in mice treated with CGMCC 3917 was ameliorated, and especially mice treated with high dosage of bacteria suspension of strain CGMCC 3917 (108 CFUs/mL) showed well-preserved cytoplasm and legible nucleoli (). Additionally, the liver tissue stained with Oil Red O showed widespread deposition of lipid droplets inside the parenchyma cells in ethanol-treated mice (). However, the liver tissues in CGMCC 3917 treated mice showed scattered droplets of fat ( and ). The results together with biochemical tests demonstrated that K. hansenii CGMCC 3917 could prevent adipose tissue lipolysis and reduce hepatic fatty acid uptake capability induced by ingestion of ethanol.
Figure 4. Effects of CGMCC 3917 on ethanol-induced hepatotoxicity in mice. (a) normal mice (b) ethanol mice, (c) ethanol+CGMCC 3917 (108 CFUs/mL) treated mice, and (d) ethanol+CGMCC 3917 treated mice (106 CFUs/mL) (200 × magnification).
Figura 4. Efectos de CGMCC 3917 sobre la hepatotoxicidad inducida por etanol en ratones. (a) ratones normales (b) ratones tratados con etanol, (c) ratones tratados con etanol + CGMCC 3917 (108 UFC/ml) y (d) ratones tratados con etanol + CGMCC 3917 (106 UFC/ml) (200 × aumentos).

3.5. Strain CGMCC 3917 prevented alcohol-induced oxidative stress in the liver
The hepatic levels of MDA, GSH-Px, and SOD are considered as typical indexes of antioxidant status of tissues (Farbiszewski, Radecka, Chwiecko, & Holownia, Citation1992). Numerous studies have confirmed that antioxidant enzymes such as SOD and GSH-Px provide protection against oxidative stress (Farbiszewski et al., Citation1992; Flores-Mateo et al., Citation2009). SOD is an antioxidant enzyme involved in the elimination of oxidative stress and serves as the first line of defense against oxidative damage (Rong et al., Citation2012). GSH-Px reduces hydroperoxides with reduced glutathione to form glutathione disulfide (Kim, Kim, & Min, Citation2015). Additionally, MDA is an end product of lipid peroxidation, which has been used as an indicator of oxidative stress (Jain, Ross, Levy, Little, & Duett, Citation1989). Alcohol metabolism with oxidative stress damage results in the formation of MDA that can bind to proteins to form adducts (Nielsen, Mikkelsen, Nielsen, Andersen, & Grandjean, Citation1997). Acetaldehyde and MDA react with proteins synergistically to form hybrid protein adducts called malondialdehyde-acetaldehyde (MAA) adducts (Nielsen et al., Citation1997). Circulating antibodies to MAA-adducts were detected in patients with alcoholic hepatitis and cirrhosis and correlated with the severity of liver injury (Nielsen et al., Citation1997). Therefore, the MDA level and activities of GSH-Px and SOD in the mouse liver were measured in the present work. As shown in , MDA level in ethanol-treated mice was about 1.4-fold higher than that of the untreated normal mice (p<0.01), indicating that ethanol administration for 10 weeks induced hepatic oxidative stress (). Ethanol treatment also resulted in the consumption of the antioxidant enzymes in mice, including hepatic GSH-Px and SOD activities ( and , p<0.01). Conversely, the elevation of hepatic MDA concentration in mice was considerably inhibited by oral administration with bacteria suspension of strain CGMCC 3917 at the two tested dosages (, p<0.01). In addition, supplementation with CGMCC 3917 at 108 CFUs/mL effectively prevented the attenuation in hepatic SOD and GSH-Px activities in the mice caused by ethanol gavage (p<0.05). These results confirmed that chronic ethanol gavage induced significant oxidative stress in the liver, and CGMCC 3917 supplementation effectively prevent the liver oxidative stress induced by ethanol treatment for 10 weeks. Herein, alcohol administration induced oxidative stress, thus resulting in the depletion of endogenous antioxidative defenses, and enzymatic antioxidants such as SOD and GSH-Px (Yang, Dong, & Ren, Citation2011). The pathogenesis of liver fibrosis is a very complex phenomenon involved different molecular and biological mechanisms. However, the literatures show that oxidative stress is a major pathogenetic factor in ALD (Dey & Cederbaum, Citation2006; Nordmann et al., Citation1992). Ethanol is mainly metabolized in the liver by alcohol dehydrogenase (ADH) and microsome ethanol oxidation system (MEOS) into acetaldehyde (Louvet & Mathurin, Citation2015). Therefore, chronic alcohol feeding increases the activation of NADPH oxidase in Kupffer cells, resulting in the generation of ROS, which can cause oxidative stress especially harmful to mitochondria. Moreover, ROS plays important roles in impaired gene expression, alterations in proteins synthesis, decreased mitochondrial content and impaired mitochondrial. Taken together, the oxidative stress leads to steatosis, inflammation, and apoptosis in the liver (Halina & Agata, Citation2014; Louvet & Mathurin, Citation2015; Nguyen, Green, & Mecsas, Citation2017). Current evidence indicates that various natural products mediate their protective effects against liver injury by decreasing the production of hepatic ROS and ameliorating the depletion of antioxidants (Ding et al., Citation2015; Lu et al., Citation2015). Although the exact mechanisms of beneficial effects of probiotics are not yet fully elucidated, it has been demonstrated that the main therapeutic effects of probiotics against liver injury and other diseases result from their anti-oxidative abilities (Abdellatef & Khalil, Citation2016; Amaretti et al., Citation2013). Our results demonstrated that K. hansenii CGMCC 3917 not only decreased the production of hepatic MDA induced by ethanol treatment for 10 weeks, but also ameliorated the depletion of antioxidants, including SOD and GSH-Px enzymes (, p<0.01), suggesting that supplementation with CGMCC 3917 may enhance the innate mechanisms of the antioxidant system to protect the liver of the tested mice from alcohol-induced oxidative stress.
Figure 5. Effects of CGMCC 3917 on hepatic (a) MDA, (b) SOD and (c) GSH-Px activities after ethanol administration in mice. Data are means ± SD (n = 10). ## p< 0.01, compared to the normal mice; **p< 0.01, compared to the ethanol-treated mice.
Figura 5. Efectos de CGMCC 3917 sobre la actividad hepática (a) MDA, (b) SOD y (c) GSH-Px después de la administración de etanol en ratones. Los datos indican medias ± DE (n = 10). ## p< 0.01, en comparación con ratones normales; ** p< 0.01, en comparación con ratones tratados con etanol.

3.6. Strain CGMCC 3917 reduced the production of hepatic inflammatory cytokines
Several inflammatory cytokines, including IL-1, IL-6, and TNF-α are considered as crucial mediators for the modulation of liver synthesis of acute phase proteins (Bailey et al., Citation2009;Batey, Cao, Madsen, Pang, & Clancy, Citation1995). The mechanisms of CGMCC 3917 protecting hepatocytes from inflammation were investigated in this work. Effects of CGMCC 3917 on inflammatory cytokines in the liver of mice under long-term ethanol administration are shown in . The ethanol gavage for 10 weeks exhibited hepatotoxicity in mice, as indicated by obvious increase in hepatic TNF-α and IL-1 levels, respectively, compared to those in normal mice ( and , p<0.01). And the administration with ethanol for 10 weeks caused a 1.2-times increase in hepatic IL-6 production in ethanol-treated mice as compared to that in normal mice (, p<0.01). However, supplementation with strain CGMCC 3917 at a dose of 108 CFUs/mL or 106 CFUs/mL resulted in the significant decrease in hepatic IL-1, IL-6, and TNF-α levels of ethanol-treated mice (p<0.05). The present work demonstrated that the levels of the hepatic inflammatory cytokines IL-1, IL-6, and TNF-α were significantly increased in alcohol-treated mice, and this increase was suppressed by CGMCC 3917 supplementation (, p<0.01). Our results suggest that K. hansenii CGMCC 3917 has beneficial effects on the mitigation of hepatic inflammation and apoptosis in cases of ALD.
Figure 6. CGMCC 3917 treatment inhibited hepatic productions of inflammatory cytokines (a) TNF-α, (b) IL-1 and (c) IL-6. Values are normalized to picogram protein content. ##p< 0.01, compared to the normal mice; **p< 0.01, compared to the ethanol-treated mice.
Figura 6. El tratamiento con CGMCC 3917 inhibió la producción hepática de citoquinas inflamatorias (a) TNF-α, (b) IL-1 y (c) IL-6. Los valores se normalizan al contenido de proteínas en picogramos. ## p< 0.01, en comparación con ratones normales; ** p< 0.01, en comparación con ratones tratados con etanol.

4. Conclusions
The present study for the first time highlights the antioxidant activity of Komagataeibacter hansenii CGMCC 3917 in vitro experiments as well as its ameliorating effects on ALD in vivo experiments using a mice model. The bacteria cells of K. hansenii CGMCC 3917 exhibited strong anti-oxidant ability in vitro. The in vivo results also indicate that supplementation of mice with K. hansenii CGMCC 3917 could attenuate oxidative stress, inflammatory reaction, and injury severity. These data suggest that K. hansenii CGMCC 3917 from edible vinegar may be an effective preventable agent or functional foods against alcohol-induced liver injury.
Ethical approval
All procedures performed in studies involving animals were in accordance with the ethical standards of Experimental Animal Administration published by the State Committee of Science and Technology of the People’s Republic of China.
Disclosure statement
No potential conflict of interest was reported by the authors.
Additional information
Funding
References
- Abdellatef, A. A., & Khalil, A. A. (2016). Ameliorated effects of Lactobacillus delbrueckii subsp. lactis DSM 20076 and Pediococcus acidilactici NNRL B-5627 on fumonisin B1-induced hepatotoxicity and nephrotoxicity in rats. Asian Journal of Pharmaceutical Sciences, 11(2), 326–336.
- Ahmed, K. B. A., Kalla, D., Uppuluri, K. B., & Anbazhagan, V. (2014). Green synthesis of silver and gold nanoparticles employing levan, a biopolymer from Acetobacter xylinum NCIM 2526, as a reducing agent and capping agent. Carbohydrate Polymers, 112(Supplement C), 539–545.
- Amaretti, A., Di Nunzio, M., Pompei, A., Raimondi, S., Rossi, M., & Bordoni, A. (2013). Antioxidant properties of potentially probiotic bacteria: In vitro and in vivo activities. Applied Microbiology and Biotechnology, 97(2), 809–817.
- Bailey, S. M., Mantena, S. K., Millender-Swain, T., Cakir, Y., Jhala, N. C., Chhieng, D., … Ballinger, S. W. (2009). Ethanol and tobacco smoke increase hepatic steatosis and hypoxia in the hypercholesterolemic apoE−/− mouse: Implications for a “multihit” hypothesis of fatty liver disease. Free Radical Biology and Medicine, 46(7), 928–938.
- Barja, F., Andrés-Barrao., C., Pérez, R. O., Cabello, E. M., & Chappuis, M. L. (2016). Physiology of Komagataeibacter spp. during acetic acid fermentation. Acetic Acid Bacteria, 201–221.
- Batey, R. G., Cao, Q., Madsen, G., Pang, G., & Clancy, R. (1995). Intrahepatic lymphocyte production of IL-1, IL-6 and TNF-α in alcoholic liver disease in the rat. Hepatology, 22(4), A365.
- Bati, B., Celik, I., & Dogan, A. (2014). Determination of hepatoprotective and antioxidant role of walnuts against ethanol-induced oxidative stress in rats. Cell Biochemistry and Biophysics, 71(2), 1191–1198.
- Bullotterson, L., Feng, W., Kirpich, I., Wang, Y., Qin, X., Liu, Y., … Petrosino, J. (2013). Metagenomic analyses of alcohol induced pathogenic alterations in the intestinal microbiome and the effect of Lactobacillus rhamnosus GG treatment. PloS One, 8(1), e53028.
- Carr, R. M., Dhir, R., Yin, X., Agarwal, B., & Ahima, R. S. (2013). Temporal effects of ethanol consumption on energy homeostasis, hepatic steatosis, and insulin sensitivity in mice. Alcoholism-Clinical and Experimental Research, 37(7), 1091–1099.
- Chen, S., Guo, Y., Chen, S., Ge, Z., Yang, H., & Tang, J. (2012). Fabrication of Cu/TiO2 nanocomposite: Toward an enhanced antibacterial performance in the absence of light. Materials Letters, 83, 154–157.
- Dey, A., & Cederbaum, A. I. (2006). Alcohol and oxidative liver injury. Hepatology, 43(2 Suppl 1), S63–74.
- Ding, R. B., Tian, K., Cao, Y. W., Bao, J. L., Wang, M., He, C., … Wan, J. B. (2015). Protective effect of Panax notoginseng saponins on acute ethanol-induced liver injury is associated with ameliorating hepatic lipid accumulation and reducing ethanol-mediated oxidative stress. Journal of Agricultural and Food Chemistry, 63(9), 2413–2422.
- Dufresne, C., & Farnworth, E. (2000). Tea, Kombucha, and health: A review. Food Research International, 33(6), 409–421.
- Farbiszewski, R., Radecka, A., Chwiecko, M., & Holownia, A. (1992). The effect of heparegen on antioxidant enzyme activities in ethanol-induced liver injury in rats. Alcohol, 9(5), 403–407.
- Floegel, A., Kim, D.-O., Chung, S.-J., Koo, S. I., & Chun, O. K. (2011). Comparison of ABTS/DPPH assays to measure antioxidant capacity in popular antioxidant-rich US foods. Journal of Food Composition and Analysis, 24(7), 1043–1048.
- Flores-Mateo, G., Carrillo-Santisteve, P., Elosua, R., Guallar, E., Marrugat, J., Bleys, J., & Covas, M. I. (2009). Antioxidant enzyme activity and coronary heart disease: Meta-analyses of observational studies. American Journal of Epidemiology, 170(2), 135–147.
- Ge, H. J., Du, S. K., Lin, D. H., Zhang, J. N., Xiang, J. L., & Li, Z. X. (2011). Gluconacetobacter hansenii subsp. nov., a high-yield bacterial cellulose producing strain induced by high hydrostatic pressure. Applied Biochemistry and Biotechnology, 165(7–8), 1519–1531.
- Ghosh, S., Guria, S., & Das, M. (2016). Alcohol as a risk factor for cancer burden: A review. Proceedings of the Zoological Society of London, 69(1), 32–37.
- Halina, C. L., & Agata, M. (2014). Oxidative stress as a crucial factor in liver diseases. World Journal of Gastroenterology, 20(25), 8082–8091.
- Hsu, T. C., Huang, C. Y., Liu, C. H., Hsu, K. C., Chen, Y. H., & Tzang, B. S. (2017). Lactobacillus paracasei GMNL-32, Lactobacillus reuteri GMNL-89 and L. reuteri GMNL-263 ameliorate hepatic injuries in lupus-prone mice. British Journal of Nutrition, 117(8), 1066–1074.
- Jain, S. K., Ross, J. D., Levy, G. J., Little, R. L., & Duett, J. (1989). The accumulation of malonyldialdehyde, an end product of membrane lipid peroxidation, can cause potassium leak in normal and sickle red blood cells. Biochemical Medicine and Metabolic Biology, 42(1), 60.
- Kim, S. Y., Kim, H., & Min, H. (2015). Effects of excessive dietary methionine on oxidative stress and dyslipidemia in chronic ethanol-treated rats. Nutrition Research and Practice, 9(2), 144–149.
- Kim, W. R., Brown, R. S., Jr, Terrault, N. A., & El Serag, H. (2002). Burden of liver disease in the United States: Summary of a workshop. Hepatology, 36(1), 227–242.
- Kirpich, I. A., & McClain, C. J. (2012). Probiotics in the treatment of the liver diseases. Journal of the American College of Nutrition, 31(1), 14–23.
- Kirpich, I. A., Solovieva, N. V., Leikhter, S. N., Shidakova, N. A., Lebedeva, O. V., Sidorov, P. I., … Cave, M. (2008). Probiotics restore bowel flora and improve liver enzymes in human alcohol-induced liver injury: A pilot study. Alcohol, 42(8), 675–682.
- Kozłowska, M., Ścibisz, I., Zaręba, D., & Ziarno, M. (2015). Antioxidant properties and effect on lactic acid bacterial growth of spice extracts. Cyta-Journal of Food, 13(4), 573–577.
- Kullisaar, T., Zilmer, M., Mikelsaar, M., Vihalemm, T., Annuk, H., Kairane, C., & Kilk, A. (2002). Two antioxidative lactobacilli strains as promising probiotics. International Journal of Food Microbiology, 72(3), 215–224.
- Li, H., Zhang, M., & Ma, G. (2011). Radical scavenging activity of flavonoids from Trollius chinensis Bunge. Nutrition, 27(10), 1061–1065.
- Lin, D., Li, R., Lopez-Sanchez, P., & Li, Z. (2015). Physical properties of bacterial cellulose aqueous suspensions treated by high pressure homogenizer. Food Hydrocolloids, 44, 435–442.
- Lin, M. Y., & Yen, C. L. (1999). Antioxidative ability of lactic acid bacteria. Journal of Agricultural and Food Chemistry, 47(4), 1460–1466.
- Louvet, A., & Mathurin, P. (2015). Alcoholic liver disease: Mechanisms of injury and targeted treatment. Nature Reviews Gastroenterology & Hepatology, 12(4), 231–242.
- Lu, C., Xu, W., Zhang, F., Jin, H., Chen, Q., Chen, L., … Zheng, S. (2015). Ligustrazine prevents alcohol-induced liver injury by attenuating hepatic steatosis and oxidative stress. International Immunopharmacology, 29(2), 613–621.
- Meagher, E. A., Barry, O. P., Burke, A., Lucey, M. R., Lawson, J. A., Rokach, J., & Fitzgerald, G. A. (1999). Alcohol-induced generation of lipid peroxidation products in humans. Journal of Clinical Investigation, 104(6), 805–813.
- Montealegre, M. C., Singh, K. V., & Murray, B. E. (2016). Gastrointestinal tract colonization dynamics by different Enterococcus faecium clades. The Journal of Infectious Diseases, 213(12), 1914–1922.
- Müller, L., Fröhlich, K., & Böhm, V. (2011). Comparative antioxidant activities of carotenoids measured by ferric reducing antioxidant power (FRAP), ABTS bleaching assay (αTEAC), DPPH assay and peroxyl radical scavenging assay. Food Chemistry, 129(1), 139–148.
- Negi, P. S., & Jayaprakasha, G. K. (2003). Antioxidant and antibacterial activities of Punica granatum peel extracts from Punica granatum L. Journal of Chemistry and Environment, 68(4), 1473–1477.
- Nguyen, G. T., Green, E. R., & Mecsas, J. (2017). Neutrophils to the ROScue: Mechanisms of NADPH oxidase activation and bacterial resistance. Frontiers in Cellular and Infection Microbiology, 7, 373.
- Nielsen, F., Mikkelsen, B. B., Nielsen, J. B., Andersen, H. R., & Grandjean, P. (1997). Plasma malondialdehyde as biomarker for oxidative stress: Reference interval and effects of life-style factors. Clinical Chemistry, 43(7), 1209–1214.
- Nordmann, R., Ribière, C., & Rouach, H. (1992). Implication of free radical mechanisms in ethanol-induced cellular injury. Free Radical Biology and Medicine, 12(3), 219.
- Okoń, A., Stadnik, J., & Dolatowski, Z. J. (2017). Effect of probiotic bacteria on antiradical activity of peptides isolated from dry-cured loins. Journal of Food, 15(3), 382–390.
- Parry, C. D., Patra, J., & Rehm, J. (2011). Alcohol consumption and non-communicable diseases: Epidemiology and policy implications. Addiction, 106(10), 1718–1724.
- Rao, G. M., Rao, C. V., Pushpangadan, P., & Shirwaikar, A. (2006). Hepatoprotective effects of rubiadin, a major constituent of Rubia cordifolia Linn. Journal of Ethnopharmacology, 103(3), 484–490.
- Raynard, B., Balian, A., Fallik, D., Capron, F., Bedossa, P., Chaput, J. C., & Naveau, S. (2002). Risk factors of fibrosis in alcohol-induced liver disease. Hepatology, 35(3), 635–638.
- Rong, S., Zhao, Y., Bao, W., Xiao, X., Wang, D., Nussler, A. K., … Liu, L. (2012). Curcumin prevents chronic alcohol-induced liver disease involving decreasing ROS generation and enhancing antioxidative capacity. Phytomedicine, 19(6), 545–550.
- Sengun, I. Y., & Karabiyikli, S. (2011). Importance of acetic acid bacteria in food industry. Food Control, 22(5), 647–656.
- Shi, X., Wei, X., Yin, X., Wang, Y., Zhang, M., Zhao, C., … Zhang, X. (2015). Hepatic and fecal metabolomic analysis of the effects of Lactobacillus rhamnosus GG on alcoholic fatty liver disease in mice. Journal of Proteome Research, 14(2), 1174–1182.
- Shimamura, S., Abe, F., Ishibashi, N., Miyakawa, H., Yaeshima, T., Araya, T., & Tomita., M. (1992). Relationship between oxygen sensitivity and oxygen metabolism of Bifidobacterium species. Journal of Dairy Science, 75(12), 3296.
- Spyropoulos, B. G., Misiakos, E. P., Fotiadis, C., & Stoidis, C. N. (2011). Antioxidant properties of probiotics and their protective effects in the pathogenesis of radiation-induced enteritis and colitis. Digestive Diseases and Sciences, 56(2), 285–294.
- Srikanth, R., Siddartha, G., Sundhar, R. C. H., Harish, B. S., Janaki, R. M., & Uppuluri, K. B. (2015). Antioxidant and anti-inflammatory levan produced from Acetobacter xylinum NCIM2526 and its statistical optimization. Carbohydrate Polymers, 123, 8–16.
- Su, J., Wang, T., Li, Y., Li, J., Zhang, Y., Wang, Y., … Li, H. (2015). Antioxidant properties of wine lactic acid bacteria: Oenococcus oeni. Applied Microbiology and Biotechnology, 99(12), 5189–5202.
- Uyanoglu, M., Canbek, M., Van Griensven, L., Yamac, M., Senturk, H., Kartkaya, K., … Kanbak, G. (2014). Effects of polysaccharide from fruiting bodies of Agaricus bisporus, Agaricus brasiliensis, and Phellinus linteus on alcoholic liver injury. International Journal of Food Sciences and Nutrition, 65(4), 482–488.
- Wang, X., Jiang, Z., Xing, M., Jing, F., Su, Y., Sun, L., & Zhang, L. (2014). Interleukin-17 mediates triptolide-induced liver injury in mice. Food and Chemical Toxicology, 71(8), 33.
- Wang, Y., Liu, Y., Kirpich, I., Ma, Z., Wang, C., Zhang, M., … Feng, W. (2013). Lactobacillus rhamnosus GG reduces hepatic TNF-alpha production and inflammation in chronic alcohol-induced liver injury. Journal of Nutritional Biochemistry, 24(9), 1609–1615.
- Yang, X., Dong, C., & Ren, G. (2011). Effect of soyasaponins-rich extract from soybean on acute alcohol-induced hepatotoxicity in mice. Journal of Agricultural and Food Chemistry, 59(4), 1138–1144.
- Yeh, M. M., & Brunt, E. M. (2014). Pathological features of fatty liver disease. Gastroenterology, 147(4), 754–764.
- Yin, J. J., Fu, P. P., Lutterodt, H., Zhou, Y. T., Antholine, W. E., & Wamer, W. (2012). Dual role of selected antioxidants found in dietary supplements: Crossover between anti- and pro-oxidant activities in the presence of copper. Journal of Agricultural and Food Chemistry, 60(10), 2554–2561.
- Yu, L., Zhao, X. K., Cheng, M. L., Yang, G. Z., Wang, B., Liu, H. J., … Mu, M. (2017). Saccharomyces boulardii administration changes gut microbiota and attenuates D-galactosamine-induced liver injury. Scientific Reports, 7(1), 1359.
- Zhao, H. Y., Zhao, C. Q., Dong, Y. Y., Zhang, M., Wang, Y. H., Li, F. Y., … Feng, W. K. (2015). Inhibition of miR122a by Lactobacillus rhamnosus GG culture supernatant increases intestinal occludin expression and protects mice from alcoholic liver disease. Toxicology Letters, 234(3), 194–200.