ABSTRACT
The effects of dairy and dairy-derived products on the human gut microbiota remains understudied. A systematic literature search was conducted using Medline, CINAHL, Embase, Scopus, and PubMed databases with the aim of collating evidence on the intakes of all types of dairy and their effects on the gut microbiota in adults. Risk of bias was assessed using the Cochrane risk-of-bias tool.The search resulted in 6,592 studies, of which eight randomized controlled trials (RCTs) met pre-determined eligibility criteria for inclusion, consisting of a total of 468 participants. Seven studies assessed the effect of type of dairy (milk, yogurt, and kefir) and dairy derivatives (whey and casein) on the gut microbiota, and one study assessed the effect of the quantity of dairy (high dairy vs low dairy). Three studies showed that dairy types consumed (milk, yogurt, and kefir) increased the abundance of beneficial genera Lactobacillus and Bifidobacterium. One study showed that yogurt reduced the abundance of Bacteroides fragilis, a pathogenic strain. Whey and casein isolates and the quantity of dairy consumed did not prompt changes to the gut microbiota composition. All but one study reported no changes to bacterial diversity in response to dairy interventions and one study reported reduction in bacterial diversity in response to milk intake.In conclusion, the results of this review suggest that dairy products such as milk, yogurt, and kefir may modulate the gut microbiota composition in favor to the host. However, the broader health implications of these findings remain unclear and warrant further studies.
Introduction
Dairy and dairy-derived products are a common component of many diets and influence physiological functions.Citation1-Citation4 Dairy products, including milk, yogurt and cheese, are generally considered nutrient-dense foods that contain proteins, calcium, and other essential nutrients such as magnesium, potassium, phosphorus, zinc, and B vitamins and their intake is associated with higher diet quality.Citation5,Citation6 The health properties of dairy foods have been the focus of intense research interest, evident from the substantial volume of published studies investigating the associations between dairy consumption and diseases such as cardiovascular disease (CVD), type 2 diabetes mellitus (T2DM), obesity, and osteoporosis.Citation1,Citation7-Citation18 However, the influence of dairy consumption on health outcomes is equivocal and often controversial, with some studies suggesting a detrimental impact on health outcomes such as fracturesCitation19 and even clinical depressionCitation20 and both positive and inverse associations observed between dairy intake and CVD,Citation7-Citation10 T2DMCitation11-Citation14 and obesity.Citation15,Citation16 This may be largely due to the complex nutritional composition of dairy products, which also poses challenges in understanding the underlying mechanism driving these observed associations.
The majority of the health impacts of dairy may be related to its nutritional composition and caloric content, however, dairy products may also influence health outcomes via the gut microbiota. It is increasingly understood that modulating the gut microbiota is a key pathway by which dietary intakes may influence health outcomes.Citation21 The gut microbiota is a dynamic metabolic organ that exerts diverse functions both localized to the gut and extending to peripheral organs.Citation22,Citation23 Diet in conjunction with other factors such as host genetics, age, sex and medication use, influences the structure and function of the gut microbiota.Citation22,Citation24-Citation27 Although there is emerging evidence that overall diet qualityCitation28,Citation29 and individual macronutrients and micronutrients of diet have a role in influencing the gut microbiota composition,Citation24,Citation30 the particular influence of dairy consumption on the gut microbiota composition is yet unclear.
Generally, a total of 3–4 serves/day of dairy products is recommended for adults, although this amount may vary depending on age, sex, and other physical requirements.Citation31,Citation32 Consumers in many countries can access a wide range of dairy products, including milk with varying fat content and fortified with additional vitamins and minerals, fermented milk/dairy (e.g. kefir, yogurt, cheese) and milk/dairy derivatives (e.g. casein, whey). Each of these products possesses distinct biological profiles and nutrient characteristics that may differentially influence the gut microbiota composition. Evidence from animal studies show that components of milk (e.g. fatCitation33 and proteinCitation34) and dairy derivatives (e.g. casein and whey isolatesCitation35) can prompt compositional changes to the gut microbiota, whilst there is some limited evidence in humans to show the impacts of some dairy groups (e.g. yogurt, acidified milk) on the gut microbiota.Citation36 Therefore, we aimed to undertake a comprehensive systematic literature review of extant intervention studies examining the influence of type of dairy products and dairy derivatives, including the quantity consumed, on human gut microbiota composition. The primary aim of our study was to investigate changes in the gut microbiota composition, measured in feces, in terms of relative abundance, colony forming units (CFU), or bacterial diversity (alpha and beta diversity) in response to dairy product consumption. Also, we further elaborated on mechanistic pathways by which dairy products may influence the gut microbiota composition.
Methods
Literature search
This review was written in accordance with the PRISMA (Preferred Reporting Items for Systematic reviews and Meta-Analyzes) statementCitation37 and was registered on PROSPERO (CRD42019137318). A search strategy was developed based on the research question (). Studies were identified using the following databases: Medline, CINAHL, Embase, Scopus and PubMed. Medical subject headings (MeSH) terms used in the search were related to milk and milk derived products (bovine milk OR cow* milk OR milk product* OR dairy product* OR cultured milk product* OR cheese OR yogurt OR kefir OR fermented dairy OR milk cream OR casein OR casein isolates OR casein concentrates OR whey OR whey isolates OR whey concentrates OR beta-casein) and gut microbiota (gut microb* OR fecal microb* OR gastrointestinal microbiome OR 16sRNA sequencing OR meta-genomics). The search strategy identified articles published since journal inception up to February 2019.
Table 1. PICOS criteria for inclusion.
The inclusion criteria were: randomized controlled trials (RCTs), including cross-over studies; RCTs that included both healthy and diseased subjects (clinical); published in English; included cow’s milk/milk-derived products as intervention (e.g. fermented or non-fermented dairy, whey protein, and milk protein supplements); and gut microbiota as the primary or secondary outcome (). Plant proteins, animal proteins, other sources of milk (sheep, goat, donkey, and human), and standard diets were considered as the comparator. Controls with no intervention/placebo were also considered as comparators. Additionally, studies that compared the effects of the quantity of dairy consumed were included. However, studies that examined the effects of non-dairy ingredients (e.g. prebiotic and probiotic supplement) that used dairy products as a medium were excluded (e.g. a yogurt enriched with a prebiotics/probiotics vs yogurt without prebiotic/probiotic supplement). Studies that investigated the changes in the gut microbiota composition (i.e. relative abundance, CFU, bacterial diversity) using any analysis techniques such as 16S rRNA, meta-genomics, qPCR, and culture dependant techniques were qualified to be included.
Data extraction
Titles and/or abstracts of studies were retrieved using the search strategy and two independent review authors (HA, VC) identified studies that met the inclusion criteria. The full texts of these potentially eligible studies were retrieved and independently assessed by two authors. Any disagreement was resolved through discussion with a third reviewer (WM, TR, AL).
A standardized, piloted form was used to extract data from the included studies for the assessment of study quality and evidence synthesis. Extracted study information included: study design; setting, sample size; study period; participant characteristics (age, sex, comorbidities); details of the intervention and comparator (quantity, duration); changes in the gut microbiota (CFU, relative abundance, single species, alpha, or beta diversity) and time points of assessment; and information for assessment of risk of bias. Four authors (HA, SD, MW, EM) extracted data independently and discrepancies identified were resolved through discussion with a fifth author (WM).
Risk of bias assessment
Risk of bias was assessed by four independent authors (HA, SD, MW, EM) using the Cochrane risk-of-bias tool for randomized controlled trials.Citation38 This is a 5-domain tool with signaling questions that assesses the risk of bias due to randomization, deviations from intended interventions, missing outcome data, measurement of the outcome, and selection of reported results. The signaling questions in each domain will assist in judging the risk of bias in the relevant domain (i.e. high risk, low risk and some concerns) with the aid of a scoring algorithm. Finally, the overall risk of bias of the study was judged as low risk of bias, some concerns, or high risk of bias based on the judgment received in each individual domain. Conflicting judgment for studies were resolved collaboratively.
Study results
As represented in , the search strategy resulted in 5093 de-duplicated studies that were screened to identify eight eligible studies for inclusion.
Study characteristics
A total of 468 participants were enrolled in the included studies. Trials ran for between 3 and 24 weeks with 12 weeks being the most common trial length (n = 3/8). Of the eight studies included, seven assessed the effect of dairy type (milk, yogurt, and kefir) and dairy derivatives (whey and casein) on the gut microbiota,Citation39-Citation45 and one study assessed the effect of consumed dairy quantity (high dairy vs low dairy) on the gut microbiota.Citation46 The mean age of the participants was 39 years (range 32–44 years). Seven out of eight studies included both males and femalesCitation40-Citation46 with one study including only males.Citation39 Studies were conducted in several countries including Switzerland, France, Denmark, Turkey, Japan, United States of America, and Canada (). Two studies were led by the same researcher.Citation41,Citation42 Risk of bias varied across studies: two studies were considered low risk of bias,Citation44,Citation45 five studies were considered to have some concerns,Citation39,Citation41-Citation43,Citation46 and one study had a very high risk of bias.Citation40
Table 2. Population characteristics.
Interventions
Of the seven studies that assessed the effect of various types of dairy and/or dairy derivatives, four assessed the effect of fermented dairy including yogurt and kefir,Citation40-Citation43 two assessed the effect of whey and casein proteins,Citation44,Citation45 and one assessed the effect of bovine milk.Citation39 Only one study investigated the quantity of dairy (low vs high intake) consumed.Citation46
Microbiome quantification
Two studies used quantitative polymerase chain reaction (qPCR)Citation42,Citation43 and four used 16S rRNA sequencing.Citation41,Citation44-Citation46 The seventh study used both qPCR and bacterial Tag-encoded FLX amplicon pyrosequencing (bTEFAP) sequencing.Citation39 A culture dependant method was used by another study by Link-Amster et al.Citation40 ().
Table 3. Methodological characteristics of articles reviewed.
The effects of the type of dairy and dairy derivatives on the gut microbiota
Milk
Fernandez-Raudales et al.Citation39 compared the effect of three-months of bovine milk consumption to two types of soymilk (low glycine soymilk and conventional soymilk) consumption on the gut microbiota composition in obese participants, using qPCR and bTEFAP. The qPCR results demonstrated that there was a significant increase in Lactobacillus and a non-significant increase in Bifidobacterium copy numbers in the bovine milk group compared to the soymilk groups at the end of the intervention. The bTEFAP showed that bacterial diversity was reduced at the end of intervention in all three groups, independent of the type of milk consumed. Similarly, bacterial richness, estimated using ACE and Chao1 indices, declined in all three groups. In the bovine milk group, the Firmicutes to Bacteroidetes ratio remained unchanged, while Roseburia tended to increase and Prevotella tended to decrease ().
Table 4. Changes in gut microbiota from feces according to dairy intervention.
Fermented dairy
Four RCTs assessed the effects of fermented dairy (yogurt and kefir) on the gut microbiota.Citation40-Citation43 Of these, threeCitation40-Citation42 reported the effect of yogurt and oneCitation43 study reported the effect of kefir on the gut microbiota composition. Link-Amster et al.Citation40 reported that consumption of fermented yogurt increased Lactobacillus and Bifidobacterium counts during the three week intervention period compared to the control arm, which consumed a usual diet but no fermented dairy/placebo. Additionally, a significant four-fold increase in specific serum IgA titer against Salmonella typhi Ty21a was observed in the intervention arm.Citation40 Odamaki et al.Citation41 demonstrated that yogurt intake reversed the shift created in the microbiota composition due to the intake of a mainly animal-based diet. All participants in this trial received a diet based mainly of animal products for five days, followed by a ‘balanced-diet’ for 14 days. Participants were randomized to one of three groups: the first group ingested 200 g of yogurt during both the animal and balanced diet period, while subjects in the second group ingested 200 g yogurt only during the balanced-diet period. Subjects in the third (control) group did not receive yogurt during either of the dietary phases. No differences in the alpha or beta diversity of the fecal microbiota were observed during the study for any groups during or following the dietary interventions.Citation42 However, at the end of the animal-based diet phase, the relative abundance of genera Odoribacter, Bilophila, Dorea, and Ruminococcus increased, and the genus Bifidobacterium decreased, in participants who had not yet received the yogurt intervention (i.e. second group ingesting yogurt in the ‘balanced diet’ phase only, and the control group). The relative abundances of these genera were significantly associated with the fat, carbohydrate, and fiber contents of the animal-based diet. Such changes were not observed in those receiving yogurt alongside the animal-based diet, prompting the authors to conclude that the effects of the animal-based diet on gut bacteria were mitigated by the yogurt consumption. In the two intervention groups, the microbiota composition returned to baseline levels following the balanced diet phase, but not in the control group (). The third study showed that yogurt intake for the period of 8 weeks significantly decreased the cell number of enterotoxigenic Bacteroides fragilis compared to the control arm that consumed milk.Citation42
The RCT conducted by Yilmaz et al.Citation43 investigated the effect of kefir on the gut microbiota in patients with inflammatory bowel disease. The test group consumed 400 mL/d of kefir for 4 weeks while the control group did not consume any fermented dairy (no placebo was provided either). The genera Lactobacillus was higher in the test group compared to control group () at the end of the study period.
Dairy derivatives
Beaumont et al.Citation44 randomly assigned healthy overweight participants into three groups to receive a dietary supplement composed of soy protein, casein protein, or maltodextrin for 5 weeks. There were no significant changes in alpha or beta diversity within or between the three groups over the period of intervention.Citation44 Another RCT using whey protein reported similar results. Reimer et al.Citation45 randomized obese subjects to four groups to receive isocaloric snack bars with different ingredients over a period of 12 weeks: (1) control bar; (2) inulin prebiotic bar; (3) whey protein bar; and (4) a combination whey protein and inulin bar. At the end of the intervention, there were changes in overall microbial structure in group two (inulin bar) and four (combination of whey protein and inulin bar), while the abundance of genus Bifidobacterium increased, and alpha diversity also decreased in these two groups (). In contrast, there were no differences reported in either group one (control bar) or three (whey protein bar).
The effects of the quantity of dairy consumed on the gut microbiota
In a 24-week RCT, Bendtsen et al.Citation46 observed that the quantity of dairy consumed did not result in significant changes to gut microbiota composition (genus or taxa level) in obese subjects. Participants in this trial were randomized to receive either a hypocaloric diet with high dairy intake or hypocaloric diet with low dairy intake. In this study, the quantity of dairy consumed was defined by calcium. The high dairy group consumed dairy in an amount equivalent to 1500 mg/d of calcium and the low dairy group consumed dairy equivalent to 600 mg/d of calcium. There were no significant changes observed in the gut microbiota composition or alpha diversity between the two groups. However, the relative abundance of genus Veillonella reduced between baseline and week 24 in the low dairy group ().Citation46
Discussion
To our knowledge, this is the first systematic literature review to collate evidence on all types of dairy and dairy-derived products and evaluate their possible effects on human gut microbiota composition. Our study results revealed that milk intake increased the relative abundance of genera Lactobacillus and Bifidobacterium and reduced the bacterial diversity.Citation39 Similarly, fermented dairy intake (i.e. yogurt and kefir) increased the abundance of genera Lactobacillus and Bifidobacterium.Citation40,Citation41,Citation43 Moreover, two studies indicated that yogurt consumption was protective against pathogenic bacterial strains i.e. Bacteroides fragilis and Salmonella typhi.Citation40,Citation42 However, it was demonstrated that neither casein or whey nor quantity of dairy consumed prompted changes to the gut bacterial taxa from phylum to species level and overall diversity.Citation44-Citation46 Nevertheless, it was indicative that milk and fermented dairy products may modulated the gut microbiota in a manner that may benefit the host by facilitating the growth of Lactobacillus and Bifidobacterium, which are considered probiotic species that – by definition – benefit host health.Citation47
In our study, the changes that dairy products may prompt to the gut microbiota was analyzed in relation to the biological profile and quantity of dairy consumed; this was taking in to consideration the distinct biological profile of each dairy products (i.e. unfermented dairy, fermented dairy, dairy derivatives) and the differing pathways by which they might influence the gut microbiota. Although some dairy products (i.e. milk, yogurt, kefir) demonstrated commonality in enhancing the growth of certain bacterial taxa (i.e. Lactobacillus, Bifidobacterium), some studies reported differential effects of dairy products on the gut microbiota. Reduced bacterial diversity – a marker often associated with host healthCitation48,Citation49 – was reduced in participants consuming bovine milk, as well as in the control conditions, and there were indications of reductions in Prevotella in the bovine milk group. Although Prevotella is considered a commensal phylotype, some studies have reported a link between certain strains of Prevotella and inflammatory status.Citation50,Citation51 However, neither the quantity of dairy consumed, nor dairy derivatives such as casein or whey isolates, had a meaningful impact on the human gut microbiota composition. Thus, the impact of dairy consumption on the gut microbiota remains unclear.
Limitations and strengths of the study
Some of the exclusion criteria that were deployed in designing this study such as excluding studies that are not published in English and excluding abstracts (i.e. no full text available in English, no full text available) may have eliminated eligible studies and this is a limitation (). Only three RCTs were double blindedCitation39,Citation44,Citation45 and others were open-label studies.Citation40-Citation43,Citation46 The unblinded trials are prone to bias estimates of treatment effects.Citation52 However, in RCTs with biological outcomes this is less of an issue. Although only RCTs were included in this systematic literature review, there were methodological inconsistencies and heterogeneity in trial settings, study subjects, microbiota analysis techniques (e.g. 16s RNA, culture-dependant methods), microbial outcomes (relative abundance, alpha, and beta-diversity), sample sizes, and study products; this precluded us from conducting a meta-analysis.
The use of varying microbial analysis techniques is another problematic characteristic of included studies; firstly, the use of culture-dependant analyses in one studyCitation40 reduced the comparability with other studies, which used culture-independent techniques such as qPCR and 16s rRNA.Citation39,Citation41-Citation46 Although five studies used 16s RNA high throughput sequencing, the analysis methods encompassed different variable regions, different pipelines, and different databases that may have influenced the result to varying extents ().Citation39,Citation41,Citation44-Citation46 Moreover, fecal sample collection methods, sample processing methods, and DNA extraction methods influence the output generated.Citation53-Citation55 RNA later stabilizer solution was used for sample collection in one study,Citation41and others employed cold-chain collection methods (i.e. no stabilizer). Importantly, the use of stabilizer kits (i.e. RNA later) may lead to disruptions in sequencing results, such as decreased species relative abundance.Citation56,Citation57 Additionally, studies differed significantly in terms of methods used to extract bacterial DNA, which can influence DNA yield, purity, and ultimately, microbiome outcomes.Citation58,Citation59As such, this may have also influenced the results. Additionally, three studies limited the analysis of the gut microbiota a priori to certain phylotypes: Firmicutes (Lactobacillus) and Actinobacteria (Bifidobacterium)Citation40,Citation43 or Bacteroidetes (Bacteroides fragilis),Citation42 making comparisons challenging. Whilst there are emerging methodologies for power calculations of microbiome studies, there is no established practice for microbiome outcomes in RCTs.Citation60 Besides, none of the included studies undertook a power calculation for microbiome outcomes, thus it is not possible to establish whether they were adequately powered to detect differences at the various taxonomic levels. This is a limitation of all studies included. Also, all studies were small and given the inherent multiple testing and corresponding false discovery rate corrections required for microbiome analyses it is possible that all included studies were underpowered.
Host characteristics are key in determining the gut microbiota composition and disparities in sex, population type (e.g. healthy, obese or overweight, inflammatory bowel disease) and geographical location differently influence the gut microbiota composition.Citation22,Citation24-Citation27 The RCTs that were included in evidence synthesis were conducted in different geographical regions and on different populations (i.e. diseased and healthy), which may have also affected the gut microbiota outcomes. Of the eight studies, six studiesCitation39,Citation40,Citation43-Citation46 considered a range of external key factors including use of antibiotics, medications, probiotics, and supplements that may also influence the gut microbiota.Citation61,Citation62 The impacts from these factors were controlled or omitted by entailing stringent inclusion/exclusion criteria (e.g. excluding participants with antibiotics use in the past three months) (). Moreover, twoCitation39,Citation41 studies monitored conditions (e.g. illness, use antibiotic, diet) that may impact the gut microbiota whilst participants were engaged in the intervention. All but three studiesCitation43,Citation45,Citation46 had a washout/run-in-period that preceded the intervention phase, which ensured dietary normalization and exclusion of any residual impacts from dairy prior to baseline; this is important given that the interventions comprised dairy products (). However, the RCTs included in this review did not assess compliance to the intervention/study products in both the experimental and control groups; consequently, noncompliance (i.e. consuming dairy external to the intervention) may be unaccounted and this is a limitation of all studies.
Components of dairy (e.g. protein, fat) and type of dairy (e.g. fermented and unfermented) may influence the gut microbiota differently; therefore, it is important to stipulate detailed description of the dairy product composition used in the intervention (e.g. low fat milk or full cream milk) in order to assess its impact on the gut microbiota composition. Two studies did not provide details on dairy compositionCitation39 or the type of dairyCitation46 used as intervention, which precluded meaningful interpretations.
Of note, four studiesCitation40-Citation43 assessed the impact of fermented dairy products that were produced by inoculating probiotic cultures; this also makes the generalization of conclusions challenging as different cultures are likely to have differential impacts on the gut microbiota. Moreover, during the process of fermentation, a myriad of biologically active molecules are synthesized including bio-active peptides, vitamins, and prebiotics, adding more functional and nutritive value to the original substrate, which in turn may influence the gut microbiota differentially.Citation63 When assessing the impacts of fermented dairy on the gut microbiota in humans, distinguishing the effects exerted by dairy components versus bacterial cultures is not possible due to synergistic effects.
Finally, all studies included in this systematic review used stool samples as a proxy to determine the gut microbiota composition. The microbiota in the stool sample may not reflect the species in the whole gastrointestinal (GI) tract, because stools mostly represent the bacterial species in the lumen and the lower GI tract.Citation23,Citation64 Thus, stool samples may not reflect the change to the bacterial species in the upper GI tract in response to external (e.g. diet, medications) or internal (e.g. disease) determinants.
Mechanisms by which dairy may influence the gut microbiota
The gut microbiota is constructed of five primary bacterial phyla (Firmicutes, Bacteroidetes, Actinobacteria, Proteobacteria and Fusobacteria), although Firmicutes and Bacteroidetes comprise 90% of the gut microbiome.Citation65 However, this proportion may not be similar in all individuals due to inter-personal variations created by environment, genetics, diet, and disease.Citation22,Citation24-Citation27,Citation66 Diet is increasingly recognized as a modulator of the gut microbiota.Citation21 Dairy products contain an array of nutrients including proteins, lipids, carbohydrates, amino acids, minerals and vitamins; any of these components may have potential to influence the gut microbiota composition.Citation36,Citation67,Citation68 However, we speculate that dairy products may influence the gut microbiota via mechanistic pathways such as: (1) facilitating the growth of beneficial strains,Citation69 (2) suppressing the growth of pathogenic strains,Citation70 and (3) altering the gastrointestinal (GI) environment ().Citation33,Citation71,Citation72
Figure 2. Mechanistic pathways by which dairy and its components may impact the gastrointestinal environment and the gut microbiota composition.
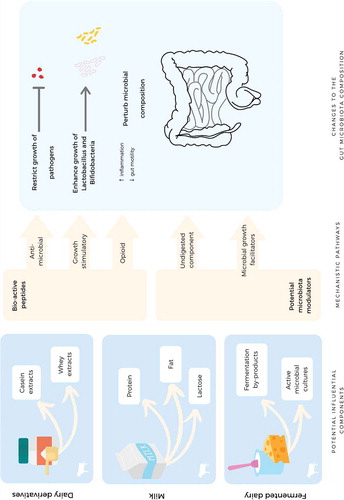
Promoting the growth of beneficial bacteria
Five studiesCitation39-Citation43 included in this review demonstrated the potential of dairy products including milk and fermented dairy (i.e. yogurt and kefir) to increase the growth of so-called beneficial bacteria.Citation40-Citation43 Lactobacillus and Bifidobacterium are two major bacterial genera that are considered to be ‘probiotics’ due to their demonstrated benefits to host health, such as improving metabolic disequilibrium, immune modulation and regulating inflammation.Citation73 Components of dairy such as lactose and protein possess potential to stimulate the growth of these bacterial genera. Lactose is the key milk carbohydrate; it exists in a concentration of 53 g/L and is often referred to as ‘milk sugar’.Citation74 Both in vitro and in vivo studies have demonstrated that lactose increased the growth of Bifidobacterium and Lactobacillus.Citation67,Citation75 Lactose also contains a prebiotic index of 5.75, which is similar to many other prebiotics.Citation69,Citation76 Prebiotics are a fermentable ingredient that instigates specific changes to both the composition and/or activity of the gastrointestinal microbes rendering health benefits. Milk proteins also hold potential to facilitate the growth of Bifidobacterium and Lactobacillus. Casein forms 80% of milk protein and is composed of four sub-units (α-caseinS1, α-caseinS2, β-casein, κ-casein). A κ-casein derived hydrolyzates demonstrated growth stimulatory effects on Bifidobacterium bifidum in a synthetic culture media.Citation68 Whey protein forms 20% of milk proteins and include the water-soluble protein fraction: α-lactoalbumin, β-lactoglobulin, immunoglobulins (Ig), lactoferrin, serum albumin, lactoperoxidase and lysozymes. Short peptides produced by the proteolytic digestion of β-lactoglobulin showed growth proliferation effects on Bifidobacterium and Lactobacillus spp.Citation77 Further, lactoferrin hydrolyzates increased the growth of Bifidobacterium adolescentis B-1 in a dose dependant manner.Citation78
Suppressing the growth of pathogens
Two studies that were included in this review demonstrated the potential of dairy products to retard the growth of pathogenic strains.Citation40,Citation42 The study by Odamaki et al.Citation42 showed that consumption of yogurt reduced Bacteroides fragilis, a strain that is associated with diarrheal disease, inflammatory bowel disease and colorectal cancer.Citation79,Citation80 Link-Amster et al.Citation40 reported that consumption of fermented yogurt increased specific serum IgA titer against an enteropathogenic strain, Salmonella typhi. Dairy products may suppress the growth of pathogenic strains via two pathways: (1) competitive exclusion of pathogens by facilitating the growth of specific bacterial strains; and (2) yielding bio-active peptides (antimicrobial peptides) that retard the growth of pathogenic strains.
As discussed previously, certain components of dairy itself (e.g. lactose, bio-active molecules produced by fermentation and peptides) facilitate the growth of probiotic bacteria, which in turn aids in pathogen elimination via creating competition for adhesion sites and producing anti-microbial peptides.Citation47 When subjected to enzymatic digestion, milk proteins can yield bio-active peptides that contain potential antimicrobial properties. Isracidin, a peptide that is produced by enzymatic digestion of α-caseinS1, demonstrates a broad spectrum of antibacterial action by restricting the growth of pathogenic strains such as Staphylococcus aureus, Streptococcus pyogenes and Listeria monocytogenes.Citation70 Casocidin is another antimicrobial peptide that is produced by the enzymatic digestion of α-caseinS2 and demonstrates potential to inhibit Escherichia coli and Staphylococcus carnosus.Citation70 One animal study showed that rats fed a casein-prebiotic mix had lower numbers of Clostridium perfringens compared to those fed a soy-prebiotic mix.Citation34 Additionally, whey protein yields antimicrobial peptides and effectively arrests the growth of Escherichia coli, Klebsiella pneumoniae, Staphylococcus aureus, Staphylococcus epidermidis, Staphylococci and Candida albicans.Citation81,Citation82
Altering the gastrointestinal environment
Dairy components, in particular milk proteins and fats, are capable of changing the luminal environment and thereby instigating changes to the gut microbiota composition.Citation33,Citation71,Citation72 The A1 beta-casein protein fraction in dairy has shown to alter the GI transit time and trigger GI inflammation by producing opioid peptides, which in turn may influence the gut microbiota composition.Citation83-Citation85 Although evidence do exist to show the potential of A1 beta-casein to increase GI transit time and trigger inflammation, the subsequent impact of these events on the gut microbiota has not been studied yet in both animal and human studies.Citation86-Citation92 Our study did include research that assessed the impact of casein extracts on the gut microbiota,Citation44 however, revealed no impact on the gut microbiota. Evidence from animal models demonstrates that milk fat intake reduced the abundance of certain bacterial taxa (i.e. Tenericutes) with a concomitant elevation in inflammatory markers.Citation33 However, in our systematic review most of the studies failed to provide the nutritional composition of dairy products, limiting the understanding of the specific contribution of dairy fats toward the changes in the gut microbiota composition.
Conclusion
The results of this systematic review suggest that some types of dairy modulate the gut microbiota composition in a manner that may benefit the host. Milk, yogurt and kefir consumption appeared to facilitate the growth of functional phylotypes i.e. Lactobacillus, Bifidobacterium that have been related to improved host health. However, the quantity of dairy and dairy derivatives (i.e. casein and whey) revealed negligible impacts on the gut microbiota. Given the heterogeneity in study methods and outcome reporting, and the unblinded nature of trials, it is difficult to draw robust conclusions. Future trials may benefit from encompassing rigorous blinded study design and a priori power calculation that ensures adequate sample size. To date the extant evidence on dairy and its implication on the gut microbiota is limited, relative to the widespread application and the different types of dairy available for consumers. Moreover, the impacts of dairy products on the gut microbiota in relation to dairy composition (e.g. full fat, low fat) are understudied. Therefore, further studies are warranted to better understand the broader impacts of dairy products on the gut microbiota.
Author contribution
HA developed the protocol, conducted the literature search, screened articles, extracted data, assessed study quality and drafted the manuscript. WM, TR, AL, AR, SD, JAP and FNJ contributed to the interpretation of data, critical appraisal of the manuscript and revised for intellectual contents.VC, SD, MW and EM extracted data and assessed the study quality. All authors read and approved the final draft for submission.
Disclosure of potential conflicts of interest
The Food & Mood Centre has received funding from Fernwood Foundation, Wilson Foundation, the A2 Milk Company, and Be Fit Foods. The A2 Milk Company has provided funding for an investigator-initiated randomized controlled trial (2018- 2020). The agreement strictly ensures the independence of the researchers at the Food & Mood Centre and the A2 Milk Company does not influence the design, content or outcomes of research arising from the Food & Mood Centre. Authors HA, AR, JAP and FNJ all acknowledge this conflict of interest. Other authors confirm no conflict of interest.
Additional information
Funding
References
- Heaney RP. Calcium, dairy products and osteoporosis. J Am Coll Nutr. 2000;19:83S–19. doi:10.1080/07315724.2000.10718088.
- Murphy S, Khaw K-T, May H, Compston JE. Milk consumption and bone mineral density in middle aged and elderly women. BMJ. 1994;308:939–941. doi:10.1136/bmj.308.6934.939.
- Barr SI, McCARRON DA, Heaney RP, Dawson-Hughes B, Berga SL, Stern JS, Oparil S. Effects of increased consumption of fluid milk on energy and nutrient intake, body weight, and cardiovascular risk factors in healthy older adults. J Am Diet Assoc. 2000;100:810–817. doi:10.1016/S0002-8223(00)00236-4.
- Elwood PC, Pickering JE, Fehily AM. Milk and dairy consumption, diabetes and the metabolic syndrome: the Caerphilly prospective study. J Epidemiol Community Health. 2007;61:695–698. doi:10.1136/jech.2006.053157.
- Rangan AM, Flood VM, Denyer G, Webb K, Marks GB, Gill TP. Dairy consumption and diet quality in a sample of Australian children. J Am Coll Nutr. 2012;31:185–193. doi:10.1080/07315724.2012.10720026.
- Fulgoni III VL, Keast DR, Auestad N, Quann EE. Nutrients from dairy foods are difficult to replace in diets of Americans: food pattern modeling and an analyses of the national health and nutrition examination survey 2003–2006. Nutr Res. 2011;31:759–765. doi:10.1016/j.nutres.2011.09.017.
- Alexander DD, Bylsma LC, Vargas AJ, Cohen SS, Doucette a, Mohamed M, Irvin SR, Miller PE, Watson H, Fryzek JP. Dairy consumption and CVD: a systematic review and meta-analysis. Br J Nutr. 2016;115:737–750. doi:10.1017/S0007114515005000.
- Lamarche B, Givens DI, Soedamah-Muthu S, Krauss RM, Jakobsen MU, Bischoff-Ferrari HA, Pan a, Després J-P. Does milk consumption contribute to cardiometabolic health and overall diet quality? Can J Cardiol. 2016;32:1026–1032. doi:10.1016/j.cjca.2015.12.033.
- Soedamah-Muthu SS, Ding EL, Al-Delaimy WK, Hu FB, Engberink MF, Willett WC, Geleijnse JM. Milk and dairy consumption and incidence of cardiovascular diseases and all-cause mortality: dose-response meta-analysis of prospective cohort studies. Am J Clin Nutr. 2011;93:158–171. doi:10.3945/ajcn.2010.29866.
- Tapsell LC. Fermented dairy food and CVD risk. Br J Nutr. 2015;113:S131–S135. doi:10.1017/S0007114514002359.
- Louie J, Flood V, Rangan a, Burlutsky G, Gill T, Gopinath B, Mitchell P. Higher regular fat dairy consumption is associated with lower incidence of metabolic syndrome but not type 2 diabetes. Nutr Metab Cardiovas Dis. 2013;23:816–821. doi:10.1016/j.numecd.2012.08.004.
- Soedamah-Muthu SS, Masset G, Verberne L, Geleijnse JM, Brunner EJ. Consumption of dairy products and associations with incident diabetes, CHD and mortality in the Whitehall II study. Br J Nutr. 2013;109:718–726. doi:10.1017/S0007114512001845.
- Sluijs I, Forouhi NG, Beulens JW, van der Schouw YT, Agnoli C, Arriola L, Balkau B, Barricarte a, Boeing H, Bueno-de-Mesquita HB. The amount and type of dairy product intake and incident type 2 diabetes: results from the EPIC-InterAct Study. Am J Clin Nutr. 2012;96:382–390. doi:10.3945/ajcn.111.021907.
- Drehmer M, Pereira MA, Schmidt MI, Molina MDCB, Alvim S, Lotufo PA, Duncan BB. Associations of dairy intake with glycemia and insulinemia, independent of obesity, in Brazilian adults: the Brazilian longitudinal study of adult health (ELSA-Brasil). Am J Clin Nutr. 2015;101:775–782. doi:10.3945/ajcn.114.102152.
- Marques-Vidal P, Goncalves a, Dias C. Milk intake is inversely related to obesity in men and in young women: data from the Portuguese health interview survey 1998–1999. Int J Obes. 2006;30:88–93. doi:10.1038/sj.ijo.0803045.
- Barba G, Troiano E, Russo P, Venezia a, Siani a. Inverse association between body mass and frequency of milk consumption in children. Br J Nutr. 2005;93:15–19. doi:10.1079/BJN20041300.
- International Osteoporosis Foundation Fact sheet: milk and dairy products are good for bone health. 2015.
- Ebeling PR, Eisman J. Recommendations from the vitamin D and calcium forum. 2005.
- Michaelsson K, Wolk a, Langenskiold S, Basu S, Lemming EW, Melhus H, Byberg L. Milk intake and risk of mortality and fractures in women and men: cohort studies. BMJ. 2014;349:g6015. doi:10.1136/bmj.g6015.
- Pasco JA, Williams LJ, Brennan-Olsen SL, Berk M, Jacka FN. Milk consumption and the risk for incident major depressive disorder. Psychother Psychosom. 2015;84:384–386. doi:10.1159/000381831.
- Singh RK, Chang H-W, Yan D, Lee KM, Ucmak D, Wong K, Abrouk M, Farahnik B, Nakamura M, Zhu TH. Influence of diet on the gut microbiome and implications for human health. J Transl Med. 2017;15:73. doi:10.1186/s12967-017-1175-y.
- Lynch SV, Pedersen O. The human intestinal microbiome in health and disease. N Engl J Med. 2016;375:2369–2379. doi:10.1056/NEJMra1600266.
- Lyte M. Microbial endocrinology in the microbiome-gut-brain axis: how bacterial production and utilization of neurochemicals influence behavior. PLoS Pathog. 2013;9:e1003726. doi:10.1371/journal.ppat.1003726.
- Lopez-Legarrea P, Fuller NR, Zulet MA, Martinez JA, Caterson ID. The influence of Mediterranean, carbohydrate and high protein diets on gut microbiota composition in the treatment of obesity and associated inflammatory state. Asia Pac J Clin Nutr. 2014;23:360–368. doi:10.6133/apjcn.2014.23.3.16.
- Ussar S, Griffin NW, Bezy O, Fujisaka S, Vienberg S, Softic S, Deng L, Bry L, Gordon JI, Kahn CR. Interactions between gut microbiota, host genetics and diet modulate the predisposition to obesity and metabolic syndrome. Cell Metab. 2015;22:516–530. doi:10.1016/j.cmet.2015.07.007.
- Schmidt TS, Raes J, Bork P. The human gut microbiome: from association to modulation. Cell. 2018;172:1198–1215. doi:10.1016/j.cell.2018.02.044.
- de Vos WM, de Vos EA. Role of the intestinal microbiome in health and disease: from correlation to causation. Nutr Rev. 2012;70:S45–S56. doi:10.1111/j.1753-4887.2012.00505.x.
- De Filippo C, Cavalieri D, Di Paola M, Ramazzotti M, Poullet JB, Massart S, Collini S, Pieraccini G, Lionetti P. Impact of diet in shaping gut microbiota revealed by a comparative study in children from Europe and rural Africa. Proc Natl Acad Sci. U.S.a. 2010;107:14691–14696. doi:10.1073/pnas.1005963107.
- De Filippis F, Pellegrini N, Vannini L, Jeffery IB, La Storia a, Laghi L, Serrazanetti DI, Di Cagno R, Ferrocino I, Lazzi C. High-level adherence to a Mediterranean diet beneficially impacts the gut microbiota and associated metabolome. Gut. 2016;65:1812–1821. doi:10.1136/gutjnl-2015-309957.
- Biesalski HK. Nutrition meets the microbiome: micronutrients and the microbiota. Ann N Y Acad Sci. 2016;1372:53–64. doi:10.1111/nyas.13145.
- National Health and Medical Research Council. Australian dietary guidelines national health and medical research council. 2013.
- Agriculture. USDoHaHSaUSDo. Dietary Guidelines for Americans; 2015.
- Huang EY, Leone VA, Devkota S, Wang Y, Brady MJ, Chang EB. Composition of dietary fat source shapes gut microbiota architecture and alters host inflammatory mediators in mouse adipose tissue. J Parenter Enter Nut. 2013;37:746–754. doi:10.1177/0148607113486931.
- Bai G, Ni K, Tsuruta T, Nishino N. Dietary casein and soy protein isolate modulate the effects of raffinose and fructooligosaccharides on the composition and fermentation of gut microbiota in rats. J Food Sci. 2016;81:H2093–H2098. doi:10.1111/1750-3841.13391.
- Masarwi M, Solnik HI, Phillip M, Yaron S, Shamir R, Pasmanic-Chor M, Gat-Yablonski G. Food restriction followed by refeeding with a casein-or whey-based diet differentially affects the gut microbiota of pre-pubertal male rats. J Nutr Biochem. 2018;51:27–39. doi:10.1016/j.jnutbio.2017.08.014.
- Burton KJ, Rosikiewicz M, Pimentel G, Bütikofer U, von Ah U, Voirol M-J, Croxatto a, Aeby S, Drai J, McTernan PG. Probiotic yogurt and acidified milk similarly reduce postprandial inflammation and both alter the gut microbiota of healthy, young men. Br J Nutr. 2017;117:1312–1322. doi:10.1017/S0007114517000885.
- Moher D, Liberati a, Tetzlaff J, Altman DG. Preferred reporting items for systematic reviews and meta-analyses: the PRISMA statement. Int J Surg. 2010;8:336–341. doi:10.1016/j.ijsu.2010.02.007.
- Cochrane Methods Bias. 2019. [cited 2020 Jun 1]. https://methods.cochrane.org/bias/resources/rob-2-revised-cochrane-risk-bias-tool-randomized-trials.
- Fernandez-Raudales D, Hoeflinger JL, Bringe NA, Cox SB, Dowd SE, Miller MJ, Gonzalez de Mejia E. Consumption of different soymilk formulations differentially affects the gut microbiomes of overweight and obese men. Gut Microbes. 2012;3:490–500. doi:10.4161/gmic.21578.
- Link-Amster H, Rochat F, Saudan K, Mignot O, Aeschlimann J. Modulation of a specific humoral immune response and changes in intestinal flora mediated through fermented milk intake. FEMS Immunol Med Microbiol. 1994;10:55–63. doi:10.1111/j.1574-695X.1994.tb00011.x.
- Odamaki T, Kato K, Sugahara H, Xiao J, Abe F, Benno Y. Effect of probiotic yoghurt on animal-based diet-induced change in gut microbiota: an open, randomised, parallel-group study. Benef Microbes. 2016;7:473–484. doi:10.3920/BM2015.0173.
- Odamaki T, Sugahara H, Yonezawa S, Yaeshima T, Iwatsuki K, Tanabe S, Tominaga T, Togashi H, Benno Y, Xiao J-Z. Effect of the oral intake of yogurt containing Bifidobacterium longum BB536 on the cell numbers of enterotoxigenic Bacteroides fragilis in microbiota. Anaerobe. 2012;18:14–18. doi:10.1016/j.anaerobe.2011.11.004.
- Yılmaz İ, Dolar ME, Özpınar H. Effect of administering kefir on the changes in fecal microbiota and symptoms of inflammatory bowel disease: a randomized controlled trial. Turk J Gastroenterol. 2019;30:242. doi:10.5152/tjg.2018.18227.
- Beaumont M, Portune KJ, Steuer N, Lan a, Cerrudo V, Audebert M, Dumont F, Mancano G, Khodorova N, Andriamihaja M. Quantity and source of dietary protein influence metabolite production by gut microbiota and rectal mucosa gene expression: a randomized, parallel, double-blind trial in overweight humans. Am J Clin Nutr. 2017;106:1005–1019. doi:10.3945/ajcn.117.158816.
- Reimer RA, Willis HJ, Tunnicliffe JM, Park H, Madsen KL, Soto‐Vaca a. Inulin‐type fructans and whey protein both modulate appetite but only fructans alter gut microbiota in adults with overweight/obesity: a randomized controlled trial. Mol Nutr Food Res. 2017;61:1700484. doi:10.1002/mnfr.201700484.
- Bendtsen LQ, Blædel T, Holm JB, Lorenzen JK, Mark AB, Kiilerich P, Kristiansen K, Astrup a, Larsen LH. High intake of dairy during energy restriction does not affect energy balance or the intestinal microflora compared with low dairy intake in overweight individuals in a randomized controlled trial. Appl Physiol Nutr Metab. 2018;43:1–10. doi:10.1139/apnm-2017-0234.
- Rastall RA, Gibson GR, Gill HS, Guarner F, Klaenhammer TR, Pot B, Reid G, Rowland IR, Sanders ME. Modulation of the microbial ecology of the human colon by probiotics, prebiotics and synbiotics to enhance human health: an overview of enabling science and potential applications. FEMS Microbiol Ecol. 2005;52:145–152. doi:10.1016/j.femsec.2005.01.003.
- Le Chatelier E, Nielsen T, Qin J, Prifti E, Hildebrand F, Falony G, Almeida M, Arumugam M, Batto J-M, Kennedy S. Richness of human gut microbiome correlates with metabolic markers. Nature. 2013;500:541–546. doi:10.1038/nature12506.
- Nowak P, Troseid M, Avershina E, Barqasho B, Neogi U, Holm K, Hov JR, Noyan K, Vesterbacka J, Svärd J. Gut microbiota diversity predicts immune status in HIV-1 infection. AIDS. 2015;29:2409–2418. doi:10.1097/QAD.0000000000000869.
- Larsen JM. The immune response to Prevotella bacteria in chronic inflammatory disease. Immunology. 2017;151:363–374. doi:10.1111/imm.12760.
- Field TR, Sibley CD, Parkins MD, Rabin HR, Surette MG. The genus Prevotella in cystic fibrosis airways. Anaerobe. 2010;16:337–344. doi:10.1016/j.anaerobe.2010.04.002.
- Kahan BC, Cro S, Doré CJ, Bratton DJ, Rehal S, Maskell NA, Rahman N, Jairath V. Reducing bias in open-label trials where blinded outcome assessment is not feasible: strategies from two randomised trials. Trials. 2014;15:456. doi:10.1186/1745-6215-15-456.
- Byrd DA, Sinha R, Hoffman KL, Chen J, Hua X, Shi J, Chia N, Petrosino J, Vogtmann E. Comparison of methods to collect fecal samples for microbiome studies using whole-genome shotgun metagenomic sequencing. Msphere. 2020;5. doi:10.1128/mSphere.00827-19.
- Wu W-K, Chen -C-C, Panyod S, Chen R-a, Wu M-S, Sheen L-Y, Chang S-C. Optimization of fecal sample processing for microbiome study—the journey from bathroom to bench. J Formos Med Assoc. 2019;118:545–555. doi:10.1016/j.jfma.2018.02.005.
- Costea PI, Zeller G, Sunagawa S, Pelletier E, Alberti a, Levenez F, Tramontano M, Driessen M, Hercog R, Jung F-E. Towards standards for human fecal sample processing in metagenomic studies. Nat Biotechnol. 2017;35:1069. doi:10.1038/nbt.3960.
- Chen -C-C, Wu W-K, Chang C-M, Panyod S, Lu T-P, Liou J-M, Fang Y-J, Chuang EY, Wu M-S. Comparison of DNA stabilizers and storage conditions on preserving fecal microbiota profiles. J Formos Med Assoc. 2020;25;S0929-6646(20)30017-6. doi:10.1016/j.jfma.2020.01.013
- Liang Y, Dong T, Chen M, He L, Wang T, Liu X, Chang H, Mao J-H, Hang B, Snijders AM. Systematic analysis of impact of sampling regions and storage methods on fecal gut microbiome and metabolome profiles. mSphere. 2020;5. doi:10.1128/mSphere.00763-19.
- Szopinska JW, Gresse R, van der Marel S, Boekhorst J, Lukovac S, van Swam I, Franke B, Timmerman H, Belzer C, Vasquez AA. Reliability of a participant-friendly fecal collection method for microbiome analyses: a step towards large sample size investigation. BMC Microbiol. 2018;18:110. doi:10.1186/s12866-018-1249-x.
- Videnska P, Smerkova K, Zwinsova B, Popovici V, Micenkova L, Sedlar K, Budinska E. Stool sampling and DNA isolation kits affect DNA quality and bacterial composition following 16S rRNA gene sequencing using MiSeq Illumina platform. Sci Rep. 2019;9:1–14. doi:10.1038/s41598-019-49520-3.
- Mattiello F, Verbist B, Faust K, Raes J, Shannon WD, Bijnens L, Thas O. a web application for sample size and power calculation in case-control microbiome studies. Bioinformatics. 2016;32:2038–2040. doi:10.1093/bioinformatics/btw099.
- Zhang S, Chen D-C. Facing a new challenge: the adverse effects of antibiotics on gut microbiota and host immunity. Chin Med J. 2019;132:1135. doi:10.1097/CM9.0000000000000245.
- Hemarajata P, Versalovic J. Effects of probiotics on gut microbiota: mechanisms of intestinal immunomodulation and neuromodulation. Ther Adv Gastroenterol. 2013;6:39–51. doi:10.1177/1756283X12459294.
- Aslam H, Green J, Jacka FN, Collier F, Berk M, Pasco J, Dawson SL. Fermented foods, the gut and mental health: a mechanistic overview with implications for depression and anxiety. Nutr Neurosci. 2018;1–13. doi:10.1080/1028415X.2018.1544332.
- Swidsinski a, Loening-Baucke V, Lochs H, Hale LP. Spatial organization of bacterial flora in normal and inflamed intestine: a fluorescence in situ hybridization study in mice. World J Gastroenterol. 2005;11:1131. doi:10.3748/wjg.v11.i8.1131.
- Rinninella E, Raoul P, Cintoni M, Franceschi F, Miggiano GAD, Gasbarrini a, Mele MC. What is the healthy gut microbiota composition? A changing ecosystem across age, environment, diet, and diseases. Microorganisms. 2019;7:14. doi:10.3390/microorganisms7010014.
- McBurney MI, Davis C, Fraser CM, Schneeman BO, Huttenhower C, Verbeke K, Walter J, Latulippe ME. Establishing what constitutes a healthy human gut microbiome: state of the science, regulatory considerations, and future directions. J Nutr. 2019;149:1882–1895. doi:10.1093/jn/nxz154.
- MAeKIVUOKKO HA, Saarinen MT, Ouwehand AC, Rautonen NE. Effects of lactose on colon microbial community structure and function in a four-stage semi-continuous culture system. Biosci Biotechnol Biochem. 2006;70:2056–2063. doi:10.1271/bbb.60022.
- KEHAGIAS C, Jao Y, Mikolajcik E, Hansen P. Growth response of Bifidobacterium bifidum to a hydrolytic product isolated from bovine casein. J Food Sci. 1977;42:146–150. doi:10.1111/j.1365-2621.1977.tb01238.x.
- Sanz ML, Gibson GR, Rastall RA. Influence of disaccharide structure on prebiotic selectivity in vitro. J Agric Food Chem. 2005;53:5192–5199. doi:10.1021/jf050276w.
- Nagpal R, Behare P, Rana R, Kumar a, Kumar M, Arora S, Morotta F, Jain S, Yadav H. Bioactive peptides derived from milk proteins and their health beneficial potentials: an update. Food Funct. 2011;2:18–27. doi:10.1039/C0FO00016G.
- Kaminski S, Cieslinska a, Kostyra E. Polymorphism of bovine beta-casein and its potential effect on human health. J Appl Genet. 2007;48:189–198. doi:10.1007/BF03195213.
- Haq MRU, Kapila R, Shandilya UK, Kapila S. Impact of milk derived β-casomorphins on physiological functions and trends in research: a review. Int J Food Prop. 2014;17:1726–1741. doi:10.1080/10942912.2012.712077.
- Turroni F, Ventura M, Buttó LF, Duranti S, O’Toole PW, Motherway MOC, van Sinderen D. Molecular dialogue between the human gut microbiota and the host: a Lactobacillus and Bifidobacterium perspective. Cell Mol Life Sci. 2014;71:183–203. doi:10.1007/s00018-013-1318-0.
- Haug a, Hostmark AT, Harstad OM. Bovine milk in human nutrition–a review. Lipids Health Dis. 2007;6:25. doi:10.1186/1476-511X-6-25.
- Daly K, Darby AC, Hall N, Nau a, Bravo D, Shirazi-Beechey SP. Dietary supplementation with lactose or artificial sweetener enhances swine gut Lactobacillus population abundance. Br J Nutr. 2014;111:S30–S35. doi:10.1017/S0007114513002274.
- Szilagyi a. Adaptation to lactose in lactase non persistent people: effects on intolerance and the relationship between dairy food consumption and evaluation of diseases. Nutrients. 2015;7:6751–6779. doi:10.3390/nu7085309.
- Poltronieri P, Cappello MS, D’Urso OF Bioactive peptides with health benefit and their differential content in whey of different origin. Whey Types, Composition and Health Implications; 2012:153–168.
- Titov EI, Tikhomirova NA, Ionova II, Gorlov IF, Slozhenkina MI, Mosolova NI, Zlobina EY. Growth stimulating effect of bovine milk lactoferrin on dermal cells and probiotic bacteria. Emir J Food Agr. 2016;28:540–546. doi:10.9755/ejfa.2015-06-447.
- Nguyen MH, Yu VL, Morris AJ, McDermott L, Wagener MW, Harrell L, Snydman DR. Antimicrobial resistance and clinical outcome of Bacteroides bacteremia: findings of a multicenter prospective observational trial. Clin Infect Dis. 2000;30:870–876. doi:10.1086/313805.
- Carolyn Redondo M, Arbo MD, Grindlinger J, Snydman DR. Attributable mortality of bacteremia associated with the Bacteroides fragilis group. Clin Infect Dis. 1995;20:1492–1496. doi:10.1093/clinids/20.6.1492.
- Pellegrini a, Thomas U, Bramaz N, Hunziker P, von Fellenberg R. Isolation and identification of three bactericidal domains in the bovine α-lactalbumin molecule. Bba-gen Subjects. 1999;1426:439–448. doi:10.1016/S0304-4165(98)00165-2.
- Shah NP. Effects of milk-derived bioactives: an overview. Br J Nutr. 2000;84:3–10. doi:10.1017/S000711450000218X.
- Banerjee S, Sindberg G, Wang F, Meng J, Sharma U, Zhang L, Dauer P, Chen C, Dalluge J, Johnson T. Opioid-induced gut microbial disruption and bile dysregulation leads to gut barrier compromise and sustained systemic inflammation. Mucosal Immunol. 2016;9:1418–1428. doi:10.1038/mi.2016.9.
- Gómez-Hurtado I, Santacruz a, Peiró G, Zapater P, Gutiérrez a, Pérez-Mateo M, Sanz Y, Francés R. Gut microbiota dysbiosis is associated with inflammation and bacterial translocation in mice with CCl4-induced fibrosis. PLoS One. 2011;6:e23037. doi:10.1371/journal.pone.0023037.
- Rowin J, Xia Y, Jung B, Sun J. Gut inflammation and dysbiosis in human motor neuron disease. Physiol Rep. 2017;5:e13443. doi:10.14814/phy2.13443.
- Becker a, Hempel G, Grecksch G, Matthies H. Effects of beta-casomorphin derivatives on gastrointestinal transit in mice. Biomed Biochim Acta. 1990;49:1203–1207.
- Defilippi C, Gomez E, Charlin V, Silva C. Inhibition of small intestinal motility by casein: a role of beta casomorphins? Nutrition. 1995;11:751–754.
- Barnett MP, McNabb WC, Roy NC, Woodford KB, Clarke AJ. Dietary A1 β-casein affects gastrointestinal transit time, dipeptidyl peptidase-4 activity, and inflammatory status relative to A2 β-casein in Wistar rats. Int J Food Sci Nutr. 2014;65:720–727. doi:10.3109/09637486.2014.898260.
- Haq MRU, Kapila R, Saliganti V. Consumption of β-casomorphins-7/5 induce inflammatory immune response in mice gut through Th 2 pathway. J Funct Foods. 2014;8:150–160. doi:10.1016/j.jff.2014.03.018.
- Haq MRU, Kapila R, Sharma R, Saliganti V, Kapila S. Comparative evaluation of cow β-casein variants (A1/A2) consumption on Th2-mediated inflammatory response in mouse gut. Eur J Nutr. 2014;53:1039–1049. doi:10.1007/s00394-013-0606-7.
- Ho S, Woodford K, Kukuljan S, Pal S. Comparative effects of A1 versus A2 beta-casein on gastrointestinal measures: a blinded randomised cross-over pilot study. Eur J Clin Nutr. 2014;68:994–1000. doi:10.1038/ejcn.2014.127.
- Jianqin S, Leiming X, Lu X, Yelland GW, Ni J, Clarke AJ. Effects of milk containing only A2 beta casein versus milk containing both A1 and A2 beta casein proteins on gastrointestinal physiology, symptoms of discomfort, and cognitive behavior of people with self-reported intolerance to traditional cows’ milk. Nutr J. 2016;15:35. doi:10.1186/s12937-016-0147-z.