ABSTRACT
Multiple sclerosis (MS) causes long-lasting, multifocal damage to the central nervous system. The complex background of MS is associated with autoimmune inflammation and neurodegeneration processes, and is potentially affected by many contributing factors, including altered composition and function of the gut microbiota. In this review, current experimental and clinical evidence is presented for the characteristics of gut dysbiosis found in MS, as well as for its relevant links with the course of the disease and the dysregulated immune response and metabolic pathways involved in MS pathology. Furthermore, therapeutic implications of these investigations are discussed, with a range of pharmacological, dietary and other interventions targeted at the gut microbiome and thus intended to have beneficial effects on the course of MS.
Introduction
Multiple sclerosis (MS) is one of the most common chronic diseases of the central nervous system (CNS). Disseminated lesions throughout the brain and spinal cord, comprising inflammatory demyelination and axonal loss, cause a range of symptoms of neurological deficit and result in accumulating disability.Citation1 The course of the disease is long-lasting and highly variable, most frequently relapsing-remitting but at some stages also progressive. The core process underlying MS pathology is associated with the dysregulated, autoreactive response of the immune system, targeted at the CNS antigens. A slowly expanding neurodegenerative process accompanies immune-mediated inflammation. However, the etiology of the disease appears to be complex, and some of its aspects still need to be fully elucidated, despite substantial progress in this field.Citation2
In recent years, there has been a consensus about the importance of gene–environment interactions in triggering and modulating the autoimmune response, relevant to the development of MS. Genetic factors are supposed to account for ca. 30% of MS risk. So far, up to 200 genetic variants (mainly associated with the immune system, e.g. HLA-DR and DQ alleles) and their epigenetic modifications have been identified as associated with the risk of MS or modulation of its course. There is also growing evidence of the significant contributing role of environmental factors, linked with genetic predisposition, in determining the onset and progression of MS. These factors include: smoking, exposure to sunlight, level of vitamin D3, stress, obesity, diet components and infections.Citation2–4
According to the hygiene hypothesis, infections in childhood and adolescence enhance later regulatory properties of the immune system; thus, limited exposure to infectious pathogens may facilitate autoimmune diseases.Citation4 Moreover, several mechanisms are suggested as the link between infections and MS onset or its further exacerbations. These include “molecular mimicry”, epitope spread, “bystander activation”, superantigen properties of pathogens, as well as expression of cryptic antigens due to injury of tissues. All these mechanisms may contribute to the activation of autoreactive immunocompetent cells, their proliferation and migration through the blood-brain barrier (BBB), resulting in inflammatory demyelination in the CNS.Citation3 Research focused on viral agents has shown that Epstein-Barr virus (EBV) infection/seroconversion is a main driver for the risk of MS, exposure to HHV6 and HSV-1 moderately increases this risk, while prior infection with CMV seems to have a protective effect.Citation2,Citation3,Citation5 Bacterial agents are suggested to have an impact as potential risk factors for MS mainly through their toxins. In animal models, toxins from Staphylococcus and Clostridium perfringens were reported to stimulate autoreactive T cells, cross the BBB and bind to myelinated neuronal fibers. Conversely, pertussis toxin exerted a protective effect by reducing T cell infiltration, activating microglia and upregulating regulatory cells.Citation6 Helicobacter pylori, which occurs with lower prevalence in MS subjects than in the general population, was also shown to ameliorate experimental inflammatory demyelination.Citation3
Recently, increasing attention has been paid to the role of microorganisms living in the human intestine (the gut microbiota) as well as their genomes, metabolites and the surrounding conditions (defined as the gut microbiome)Citation7 in the background of the CNS diseases.
The gut-brain axis comprises bidirectional communication between the gastrointestinal system and the CNS, including endocrine, metabolic, immune, and neurotransmitters links. The gut microbiome plays a significant role in these interactions, e.g. through vagal stimulation, the release of metabolites into the circulation and stimulating immunocompetent cells within the intestinal wall. There is evidence of the impact of the gut microbiome on the maturation and differentiation of neurons and glial cells, functional integrity of the BBB, as well as maintaining the balance between pro- and anti-inflammatory components of the immune response. Thus, the gut microbiome’s relevance in MS development and dynamics seems particularly appealing.Citation8,Citation9
Some interesting links have been observed between the gut microbiome and genetic or environmental risk factors for MS (). Overall, complex interactions between host and gut microbiota affect composition and function of the latter. Metabolism of bacteria is based on the substrates determined by the host genetics, while host or bacterial gene expression may be mutually regulated by specific miRNAs.Citation10 The role of these interactions in determining susceptibility to CNS autoimmunity was demonstrated in animal models. Evidence was provided for a significant role of the HLA-DQ8 and HLA-DR3 genes in shaping murine gut microbiota as well as their linked contribution to EAE susceptibility or resistance.Citation11 Furthermore, diverse microbial profiles associated with specific genotypes were identified in mice, and the introduction of incompatible bacterial strains (e.g. Lactobacillus reuteri) in a genetically susceptible host was demonstrated to contribute to EAE exacerbation.Citation12
Figure 1. Contribution of genetic and environmental factors to development of MS.
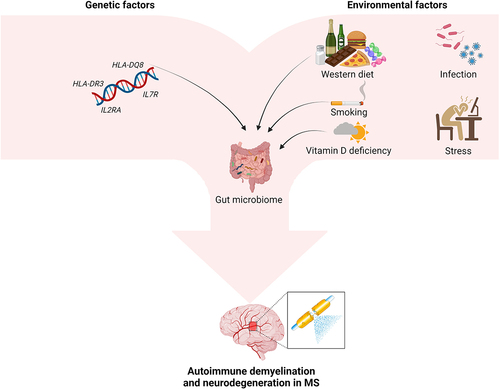
Genome-wide association studies revealed some associations between microbiota composition and variants of the vitamin D receptor (VDR) gene.Citation13 Moreover, the level of vitamin D3 affects intestinal calcium absorption and thus intestinal motility, as well as the integrity of the mucosal intestinal barrier. Thus, vitamin D3 deficiency contributes to gut stasis with a rise in intestinal permeability for bacteria and their products. Smoking, considered as another risk factor for MS (due to the impact on pro-inflammatory cytokines and migration of T cells through the BBB), may modify the content and function of gut microbiota through toxic substances contained in tobacco smoke and/or immune-mediated mechanisms.Citation2,Citation4,Citation14
It is still a matter of debate whether the gut microbiome acts as a triggering and modulating factor for the autoimmune response involved in the background of MS, or whether perhaps the gut dysbiosis develops as a consequence of the disturbed balance between pro- and anti-inflammatory mechanisms and metabolic alterations in the course of disease. However, investigation of the composition and functions of the gut microbiome in people with MS (pwMS) may provide a better insight into the background of MS and dynamics of its clinical course.Citation8,Citation15
Despite recent progress in MS management, there are still some challenges ahead, associated inter alia with individual differences in the course of disease and response to treatment. There is an ongoing search for biomarkers for the disease activity and progression. In the current therapeutic guidelines for MS, a trend for personalized and complex treatment strategies is highlighted, with disease-modifying therapies (DMT) complemented with modification of lifestyle.Citation16,Citation17 In view of that, the gut microbiota profile could be considered as a signature of individual immune or metabolic properties, and thus a promising marker for choice of DMT or supportive therapeutic interventions.
Therefore, in this review, we discuss the current evidence on the role of the gut microbiome in the processes underlying MS pathology, focusing on emerging potential therapeutic implications.
Methods
The online literature search was conducted using PubMed and Scopus databases, covering the publication period from the start of 2010 until 1st March 2023. The following combination of key search terms was applied: “multiple sclerosis”, “gut microbiome/microbiota” and “treatment”. Having excluded conference abstracts and papers written in languages other than English, the reviews and original research studies were screened and analyzed for their relevance to the topic. In addition, the reference lists from the retrieved publications were investigated and further potentially relevant papers were identified. Initially the literature search was performed by the first author, with its results reviewed and further stages of the search verified by the other authors.
Characteristics of the gut microbiome in MS
Composition of the gut microbiota in MS patients
Since 2015 the composition of the gut microbiota in MS has become a subject of considerable interest. One of the earliest studies indicated that epsilon toxin produced by Clostridium perfringens (a gut commensal) can modulate the integrity of the BBB, reach the CNS and act as a potential trigger of the MS-related autoimmune response.Citation18 Several subsequent reports showed that the composition of the main bacterial taxa in the intestine was significantly altered in MS patients compared to healthy subjects (HS) (). The identified changes were heterogenous and differed depending on the studied population and methodology (inter alia, sequencing platform and investigated 16S rRNA gene region). Despite this diversity, a consensus seems to be emerging at the taxonomic level concerning the relative abundance of particular bacterial strains. The most frequently identified depleted bacterial taxa included Bacteroides,Citation19–25 Faecalibacterium,Citation21,Citation22,Citation25–27 PrevotellaCitation23,Citation24,Citation28,Citation29 and Roseburia,Citation21,Citation22,Citation26,Citation30 whereas the enriched ones included AkkermansiaCitation19,Citation28,Citation30–34 and Streptococcus.Citation23,Citation24,Citation30,Citation33,Citation35,Citation36 The composition of gut microbiota differed between the patients with various types of MS.Citation30,Citation32,Citation33,Citation39 Relapsing-remitting type (RRMS) was associated with a decreased amount of Faecalibacterium prausnitzii, Eubacterium rectale and Roseburia,Citation21,Citation32 while primary progressive type (PPMS) was associated with an elevated level of Enterobacteriaceae and Clostridium g24 FCEY and a decreased level of Blautia and Agathobaculum.Citation32 In those with secondary progressive (SPMS) type, a relative increase of Clostridium and Streptococcus strains was observed.Citation33
Table 1. Alterations of the gut microbiota in MS patients without specified treatment.
Further clinical studies were designed to investigate factors contributing to altered composition and function of the gut microbiome in MS.Citation31,Citation40 A study which included twins discordant for the disease indicated interaction of environmental and genetic factors in this field.Citation31 The International MS Microbiome Study Consortium paired MS subjects with household HS (to minimize the effect of dietary habits) and demonstrated the relationships between the gut microbiome alterations and MS risk and stage of disease.Citation40,Citation41 Variability of microbial composition in MS subjects may be further affected by age and gender, dietary components, comorbidities and related therapies (e.g. antibiotics intake).
It is still debatable whether gut dysbiosis can trigger (through the gut-brain axis) the autoimmune response involved in the background of MS, or whether it merely reflects the disturbed balance between pro- and anti-inflammatory components of this autoimmune response. Experimental and clinical studies have been undertaken to elucidate these relationships and reveal their relevant aspects.
The role of gut dysbiosis in MS pathology
Experimental studies
Studies conducted in an animal model of MS – experimental autoimmune encephalitis (EAE) – showed the pattern of alterations in the gut microbiome similar to human studies and related to the course of the disease. Analysis of the intestinal flora at pre-onset, onset, peak and chronic phase of EAE revealed that the abundance of the Lactobacillaceae family was decreased, while other populations such as Clostridiaceae, Ruminococcaceae and Peptostreptococcaceae were expanded in the course of the disease.Citation42 Gandy et al. found diverse microbial populations characteristic for SJL/J mice having relapsing-remitting EAE (RR-EAE) and C57BL/6 mice with chronic progressive EAE (CP-EAE). In RR-EAE mice there was a significant expansion of Bacteroidales (including Bacteroides, Parabacteroides, Prevotella, Rikenellaceae and Odoribacter), compared to CP-EAE. In turn, CP-EAE mice were identified with significantly elevated Akkermansia muciniphila.Citation43 Moles et al. evaluated the relationship between microbiome composition and disease symptoms using EAE and the cuprizone demyelination model (the latter characterized by a progressive course).Citation44 In both models, an increase of Firmicutes and decrease of Bacteroides were observed at the onset of clinical symptoms, accompanied by a remarkable decrease in Actinobacteria, represented mainly by Bifidobacterium. Alterations specific only for the cuprizone model included an apparent rise of the phylum Verrucomicrobiota, exclusively represented by Akkermansia, as well as the Sutterella population, which appeared after cuprizone exposure and during the remyelination process. Furthermore, diversity of microbiota correlated with severity of demyelination and scores for clinical symptoms.
Further experimental studies provided some evidence for a causal link between gut dysbiosis and autoimmune CNS involvement. It was demonstrated that germ-free (GF) mice (lacking the gut microbiota or treated with antibiotics) did not develop EAE or presented with delayed and reduced disease activity.Citation45 In turn, the colonization of GF mice with segmented filamentous bacteria resulted in an increased IL-17 level in the gut, which favored Th17 proliferation and ultimately caused development of EAE.Citation46 In contrast, monocolonization with Bacteroides fragilis prevented EAE in these mice by inducing tolerogenic CD103+ dendritic cells (DCs)Citation47 and enhanced production of intestinal Tregs secreting IL-10.Citation48 This protective effect of B. fragilis was attributed to its structural component, polysaccharide A. Another interesting investigation, which involved testing a single probiotic strain – Lactobacillus helveticusCitation49 – for the EAE effect, also indicated differential modulation of the autoimmune response resulting in EAE attenuation. Examples of other bacteria able to ameliorate EAE include Lactobacillus strains (Lactobacillus crispatus, Lactobacillus rhamnosus, Lactobacillus paracasei, Lactobacillus plantarum and Lactobacillus reuteri)Citation42,Citation50–52 as well as Bifidobacterium strains (Bifidobacterium animalis and Bifidobacterium bifidum). Citation53,Citation54 There are also reports demonstrating that the combination of two probiotic strains – Bifidobacterium animalis with Lactobacillus plantarumCitation55 or Enterococcus faecium with Prevotella histicolaCitation56 – ameliorated neuroinflammation in the EAE model. Surprisingly, it has been found that treatment with a probiotic mixture of Streptococcus thermophilus, Lactobacillus reuteri, Bifidobacterium bifidum, Lactobacillus acidophilus and Lactobacillus casei during induction of EAE delayed disease onset.Citation54
CNS infection caused by Theiler’s encephalomyelitis virus (TMEV) – an experimental model of progressive MS – altered the composition of the gut microbiota of SJL/J mice toward moderate dysbiosis at particular phases of the disease. In the acute phase there was a decrease in the Alloprevotella population, at the presymptomatic stage a decrease of Akkermansia and Anaerotruncus, and at the chronic stage a decrease of Streptococcus. Conversely, Clostridium and Eubacteria were increased throughout the disease. Furthermore, TMEV infection was observed to increase intestinal permeability and CD4+ T cell count in the lamina propria.Citation57 Another study on a murine model of TMEV infection showed its selective impact on gut microbiota, with increased abundance of Marvinbryantia and Coprococcus genera. Links were identified between these bacterial genera and the CNS transcriptome for the T cell receptor, immunoglobulins, major histocompatibility complex (MHC) and complement genes.Citation58
In a cuprizone-induced demyelination model, altered β-diversity of the gut microbiome was found in mice, as well as a positive correlation between relative abundance of some species (including Eisenbergiella and Faecalibaculum) and extent of CNS demyelination and activation of microglia.Citation59 Moreover, subdiaphragmatic vagotomy in cuprizone-treated mice attenuated demyelination and microglial activation in the corpus callosum and also partially restored the abnormal β-diversity of gut microbiota (inter alia with an increase in relative abundance of Lactobacillus and Turicibacter). These findings suggested the involvement of the vagus nerve (as a component of the gut–brain axis) in pathomechanisms of cuprizone-induced CNS demyelination.Citation60
Interesting observations were based on a transfer of intestinal content between human or animal subjects. Transfer of feces obtained from mice with EAE at peak disease activity to naïve mice (before their immunization) suppressed development of the disease in recipients. The feces from EAE animals (as well as untreated pwMS) was shown to be enriched in microRNA-30d, which regulated the expression of lactase essential for growth of Akkermansia muciniphila. Increased abundance of Akkermansia in the gut was associated with stimulation of Tregs and related cytokines, supposedly contributing to amelioration of CNS inflammation.Citation61
Furthermore, administration of Akkermansia isolated from pwMS to animals was shown to ameliorate EAE by reduction of RORγt+ and IL-17 produced by γδ T cells.Citation32 EAE also developed in GF mice after transplantation of gut microbiota obtained from pwMS, in contrast to those who obtained it from HS.Citation19,Citation31 These findings suggest the potential relevance of the microbiota-gut-brain axis in the pathogenesis of MS.
Taken together, the above in vivo data indicate that the gut dysbiosis is functionally linked with a shift from regulatory components of the autoimmune response toward pro-inflammatory ones. These outcomes were believed to be affected by microbial metabolites, mainly SCFA fermentation products. It has been shown that SCFA (e.g. propionate) may directly affect T cell subsets, with decreased differentiation of Th17 cellsCitation62 and increased differentiation of Tregs and their enhanced suppressive capacity.Citation63
Moreover, microbial SCFA was shown to influence the BBB permeabilityCitation64 and the CNS resident cells like microglia.Citation65 GF mice, compared to those with normal gut microbiota, possessed reduced expression of tight junction proteins, which lead to increased BBB integrity. The BBB permeability could be modulated by SCFA-producing bacteria or directly by their metabolites, such as butyrate.Citation64 GF mice also presented abnormal maturation and differentiation of microglial cells; their function could be restored using SCFA metabolites or following colonization with microbiota capable of SCFA production.Citation65
Clinical evidence
The aforementioned findings from experimental studies supported further thorough clinical investigation of the gut microbiome in pwMS, considering the more complex nature of the disease compared to an animal model, with additional confounding factors.
In RRMS patients, an increase in Akkermansia muciniphila, a mucin degrading bacteria, and Acinetobacter calcoaceticus was observed, and they were found to provoke pro-inflammatory activity in vitro.Citation19 Particularly, MS-derived Akkermansia muciniphila enhanced differentiation of human T cells toward Th1 cells,Citation19 while Prevotella (otherwise decreased in MS subjects)Citation66 enhanced cell differentiation toward Th17 expansion. In addition, Jangi et al. reported that increased Akkermansia and Methanobrevibacter positively correlated with the expression of genes for pro-inflammatory T cells and monocytes, both implicated in the pathogenesis of MS.Citation67 Furthermore, elevated antibodies against Acinetobacter in serum of MS patients suggest a possible role of this bacteria in autoimmune cross-reactivity.Citation68 Another example linking microbial alteration with a dysregulated immune response in MS is frequently identified depletion of Bacteroides,Citation19–25 associated with a lower level of lipid 654, a TLR2 ligand, relevant for innate immunity.Citation69 Cekanavicute et al. found that a particular Bacteroides species, Parabacteroides distasonis, can augment the function of Tregs in vitro. Thus, the lower abundance of this species may result in suppressed immunoregulatory activity.Citation34 Furthermore, Bacteroides fragilis was found to modulate maturation of the immune system through bacterial polysaccharide, by correcting deficiencies of T cells and Th1/2 imbalance, and eliciting production of appropriate cytokines.Citation70
Findings from clinical studies also indicated some metabolic alterations potentially related to the gut microbiome. Reduced activity of microbial SCFA producers was found in RRMS patients, as well as a decreased level of their metabolite butyric acid, in contrast to an upregulated level of caproic acid (medium chain FA with pro-inflammatory properties). Absence of the butyrate-producing Fusobacteria or MethanobrevibacterCitation38 was associated with a shorter time to MS relapse.Citation71
Functional differences in gut microbiota were demonstrated for various phenotypes of MS.Citation33 As indicated above, in RRMS patients a decrease in regulatory bacteria, such as Parabacteroides and Prevotella (Bacteroidetes), Adlercreutzia and Collinsella (Actinobacteria) as well as Erysipelotrichaceae (Firmicutes), has been noted.Citation66 Adlercreutzia, Parabacteroides and Prevotella can process phytoestrogens into monomeric compounds, decreasing chemo-attracting proteins-1 and IL-6, thus diminishing oxidative stress and inflammatory cytokine activity.Citation72 In turn, Erysipelotrichaceae play an important role in bile acid metabolism and ensure homeostasis at the mucosal surface.Citation73 In contrast to RRMS subjects, those with SPMS were characterized by elevated levels of oxidative stress markers such as: i) an increase of microbial genes involved in mismatch DNA mutation repair and ii) an increased ratio of cysteine persulfide to cysteine, indicating excessive DNA oxidation.Citation33 In addition, the presence of Clostridium strains in both RRMS and SPMS was associated with higher scores of disability and fatigue.Citation33
Mechanistic concepts of the gut microbiome contribution to MS background
The experimental and clinical evidence revealed the links between gut dysbiosis in MS and the dysregulated immune response, as well as altered metabolic pathways, corresponding to the clinical course of the disease. The key points of this investigation included: i) imbalance between pro-inflammatory and anti-inflammatory/regulatory components of the immune response involved in the MS background and ii) ability of commensal bacteria to promote either the pro- or anti-inflammatory pathway via different mechanisms.Citation74
Impact upon the autoimmune response and disease induction
Among potential mechanisms of the gut microbiome triggering CNS inflammation, the relevant evidence indicates molecular mimicry – cross-reactivity between self-antigens of CNS and microbial peptides. Some similarities were found between myelin basic protein (MBP) and proteins of Bacteroides and Bifidobacterium species. Homologous reactivity of CD4+ T cell clones has also been demonstrated for epitopes of MBP and GDP-L-fucose synthase of Akkermansia and Prevotella. Effects of bacteria from the Erysipelotrichaceae family and L. reuteri on EAE have been associated with myelin oligodendrocyte protein (MOG) molecular mimicry.Citation75,Citation76
Furthermore, bacterial products (e.g. peptidoglycan or N-acetylmuramyl dipeptide) have been found to stimulate receptors on antigen-presenting cells, triggering production of pro-inflammatory mediators and promoting bystander lymphocyte activation.
Other pathogenic mechanisms of the gut microbiome affecting CNS autoreactive inflammation might be associated with its direct impact on immunocompetent cells. Particular microbes (e.g. segmented filamentous bacteria) are able to induce differentiation of Th17 cells in the ileum. Pro-inflammatory properties of these cells may be activated by presence of some dietary compounds (salt or long chain fatty acids). After migration to lymph nodes, Th17 cells can lower the threshold for activation of autoreactive T cells, promoting an autoimmune response.
Interestingly, protective anti-inflammatory effects have been observed for other bacteria (Clostridia, Bacteroides, Prevotella). These effects were suggested to be associated with their particular components, such as polysaccharide A and lipid 654, a TLR2 ligand. The relevant role of these molecules includes an influence on Treg differentiation in the colon, promoting the expansion of FoxP3+ Tregs, correcting Th1/2 imbalance, stimulating production of anti-inflammatory cytokines, and induction of tolerogenic DCs and suppressive macrophages.Citation10,Citation47,Citation48,Citation70,Citation75
Impact upon metabolic pathways and their links with immune and CNS system
The gut microbiome metabolites take part in a range of metabolic pathways relevant for inflammatory and neurodegenerative processes in the CNS.
SCFAs
The SCFAs, by-products of the fermentation of complex and indigestible carbohydrate in the colon, i.e., acetate (C2), propionate (C3) and butyrate (C4) (), are known to be potent immune modulators. Furthermore, SCFAs are able to cross the BBB, which is associated with expression of: i) proton or sodium-dependent monocarboxylate transporters (MCT and SMCT, respectively) and ii) various receptors including extracellular G protein coupled receptors (GPR), namely free fatty acid receptors (FFAR), broadly expressed in various tissues and immune cell types.Citation77 These findings imply the direct influence of SCFAs on the function of CNS resident cells affecting homeostasis (). The main processes triggered by SCFAs included activation of FFAR2 and FFAR3, also known as GPR43 and GPR41.Citation77 The SCFAs activate ERK,Citation78 JNK,Citation62,Citation78 p38-MAPK.Citation62 NRF2Citation81 and NF-κBCitation78 signaling pathways, which modulate the activity of both innate and adaptive immune cells. It has also been observed that SCFA metabolites – acetate, propionate and butyrate – promote IL-10 and FoxP3 peripheral productionCitation88 and inhibit TNF-α and IL-1β in the CNS.Citation90 In addition, after entering the cells, SCFAs can inhibit histone deacetylase (HDACs)Citation80,Citation82,Citation84,Citation86 and directly influence gene expression, causing among other things upregulation of regulatory mediators (IL-10, FoxP3)Citation63,Citation80,Citation87,Citation88 or downregulation of pro-inflammatory mediators (iNOS, IL-6 and IL-12).Citation84
Figure 2. Methabolic pathways of bacterial fermentation resulting in the production of short chain fatty acid (SCFA) components.
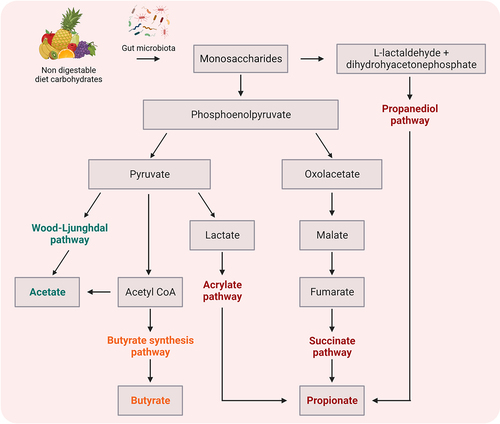
Table 2. Mechanisms of action of bacterial fatty acid metabolites on events related to MS background.
SCFAs were also reported to maintain and increase BBB integrity by increased expression of the tight junction proteins occludin and claudin-5.Citation64 PwMS who had a lower butyric acid (BA)/caproic acid (CA) ratio showed higher intestinal permeability and more Th1 cells. The increase in the BA/CA ratio correlated positively with Treg subsets and negatively with IFN-γ-producing lymphocytes.Citation30
Moreover, acetate-mediated fatty acid synthesis in oligodendrocytes and HDAC-dependent butyrate action are believed to contribute to protection of CNS oligodendroglia, with suppressed demyelinationCitation79 and enhanced remyelination.Citation85
Polyunsaturated fatty acids
Polyunsaturated fatty acids (PUFAs) are characterized by the presence of multiple double bonds within the fatty acid chain, which determines their properties. Particular interest has been attributed to omega-3 PUFA, e.g. α-linolenic acid (ALA), eicosapentaenoic acid (EPA) and docosahexaenoic acids (DHA). Omega-3 PUFA can alter the diversity and abundance of gut microorganisms, particularly influencing beneficial bacteria such as Bifidobacterium and Akkermansia. In addition, they improve the intestinal mucosal barrier function by increasing its thickness and reducing its damage caused by inflammatory and oxidative processes (e.g. LPS, hydrogen peroxide, increased mitochondrial activity).Citation91 More importantly, omega-3 PUFA regulate gut homeostasis and its immunity. They are known to modulate intestinal immunity via the nuclear transcription factor κB (NF-κB) and MAPK signaling pathwaysCitation92 as well as the metabolic pathway of arachidonic acid.Citation93 These acids also possess the ability to control the level of pro-inflammatory (e.g. endotoxins and IL-17) as well as anti-inflammatory (e.g. SCFAs and their salts) mediators.Citation94
Tryptophan metabolites
Tryptophan is an essential amino acid metabolized by the gut microbiota into indole-containing compounds. Particularly, Lactobacillus strains were shown to directly utilize tryptophan as a substrate and metabolize it into indole-3-lactic acid (ILA), indole-3-acetic acid (IAA) and indole-3-aldehyde (IAld) in the gut.Citation95 Moreover, tryptophan itself can be transported from the gut into the circulation and metabolized by the kynurenine and serotonin pathway (). Both tryptophan and its metabolites can act as potent immunomodulators by binding to the aryl hydrocarbon receptor (AhR) (). Different levels of circulating AhR ligands were found in RRMS patients, depending on the disease activity.Citation96
Figure 3. Tryptophan metabolic pathways.
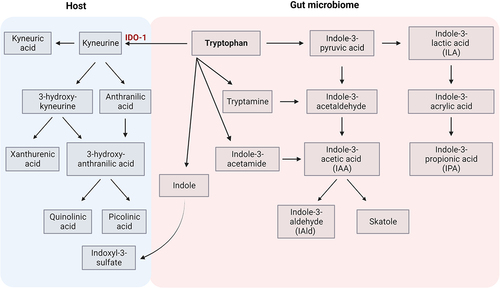
Table 3. Mechanisms of action of tryptophan metabolites on events related to MS background.
Tryptophan metabolites may modulate T cell subsets’ differentiation by either promoting Th1/Th17 cells or generating Tregs.Citation97,Citation107 IAld was found to inhibit Th17 polarization, to reduce production of IL17Citation98, and to induce mucosal IL-22 via AhR.Citation99 A favorable effect of Lactobacilli on EAE outcome was attributed to properties of this metabolite.Citation51,Citation108 Indole and indole-3-propionic acid (IPA) have also been reported to promote tight junction formation, acting directly on epithelial cells in a pregnane X receptor (PXR) dependent manner.Citation100
Metabolic products of the kynurenine pathway can affect brain epithelial cells depending on the condition. Basically, endothelial cells convert tryptophan to kynurenic acid. However, in the presence of pro-inflammatory cytokines, TNF-α and IFN-γ, endothelial cells express indolamine 2,3-dioxygenase (IDO-1), which converts tryptophan to kynurenine, further metabolized by perivascular macrophages and microglia to quinolinic acid.Citation103 Production of quinolinic acid by activated microglia and infiltrating macrophages has been reported to have neuroinflammatory and neurodegenerative effects.Citation105 Increased quinolinic acid/kynurenic acid ratio in patients with progressive types of MS may suggest that quinolinic acid is relevant for the neurodegenerative component of the disease background.Citation104 On the other hand, indole-containing metabolites, namely indole-3-sulfate (I3S), IPA and IAld, limit production of Il-6, TNF-α, CCL2, and iNOS from astrocytes, resulting in disease amelioration.Citation101 In addition, it has been shown that dietary tryptophan metabolites control microglial activation and TGF-α and VEGF-B production, and in consequence modulate astrocyte activities via AhR.Citation102 Amelioration of disease in animal models of MS by synthetic indole derivatives was suggested to be mediated by AhR signaling in astrocytes.Citation106
Apart from these main metabolic pathways involving tryptophan (AhR and PXR ligands), there is also some evidence for relevance of other ones (e.g. tryptophan-derived serotonin)Citation109 in the links between the gut microbiome and MS, which require further investigations.
Polyamines
Polyamines (putrescine, spermidine and spermine), derived from the L-arginine metabolic pathway (), can be produced either by the host or by gut microbiota (i.e. Bacteroides thetaiotaomicron, Fusobacterium varium, Enterococcus, Bifidobacterium).
Figure 4. Polyamine metabolism.
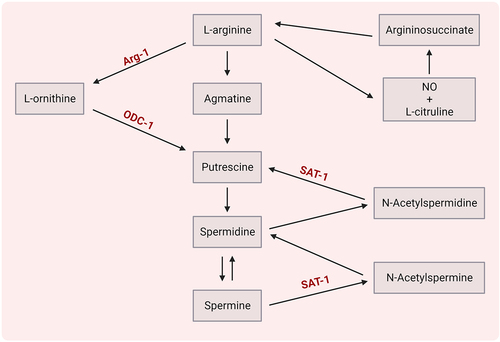
These compounds can diminish neuroinflammation by modulation of T cells or modulation of microglia/macrophages (). Spermidine was found to shift the polarization of Th17 cells toward Tregs in the murine gut in a TGF-β-dependent manner.Citation111 Strikingly, suppression of ornithine decarboxylase 1 (ODC-1) or spermidine/spermine N1 acetyltransferase 1 (SAT-1) – enzymes involved in polyamine metabolism – can specifically limit Th17 function in a putrescine-dependent mode.Citation110
Table 4. Mechanisms of action of polyamine metabolites on events related to MS background.
Following treatment with spermidine, amelioration of EAE was observed, associated with decreased secretion of pro-inflammatory cytokines IL-1β and IL-12, lowered levels of co-stimulatory molecules CD80 and CD86 by inhibition of the NF-κB pathway along with upregulation of expression of arginase 1 (Arg-1) in macrophagesCitation112, both causing a shift to M2 phenotype. Spermine and spermidine were also reported to reduce immune cell infiltration into the CNS, as a result of decreased release of astrocyte-derived chemokines, such as MIP-1a, MCP-1, and RANTESCitation113 or direct inhibition of LFA-1 on T cells.Citation114
Polyphenols
Polyphenols can be classified into flavonoids (e.g. apigenin, epigallocatechin-3-gallate, hesperidin A) and nonflavonoids (e.g. resveratrol). They exert a protective effect on neurons by acting against oxidative stress and suppressing pro-inflammatory NF-κB pathway. The gut microbiota contributes to the biotransformation of polyphenols into their bioavailable forms and active metabolites, relevant for the synthesis of neurotransmitters.
Ellagic acid, another polyphenol compound, is metabolized in the gut by Gordonibacter urolithinfaciens and Gordonibacter pamelaeae and generates urolithins. Studies have shown that urolithins exert effects on T cells, DCs, microglia, oligodendrocytes and neurons (). Interestingly, ellagic acid, a urolithin A precursor, has been shown to protect against myelin-associated sphingolipid loss by affecting ceramide synthesis.Citation120 In addition, Zhang et al. reported that T cell activation and proliferation can be suppressed by urolithin A through interfering with calcium machinery in a miR-10a-5p dependent manner.Citation115 In line with this, reduced infiltration of Th1/Th17 cells and monocytes to the CNS by targeting AhR was observed.Citation116 The impact of urolithin A administration also included reduced levels of co-stimulatory molecules CD80, CD86 and MHC-II on DCs, as well as a lower proportion of M1 pro-inflammatory type of microglia.Citation116
Table 5. Mechanisms of action of urolithin metabolites on events related to MS background.
Moreover, urolithin A was shown to decrease the epithelial intestinal permeability and attenuate inflammation in an Ahr-Nrf2-dependent fashion through modulation of tight junction proteins.Citation117 The neuroprotective effect of urolithins was additionally associated with AMPK, MAPK, JNK, ERK signaling pathways.Citation118,Citation119,Citation121
Gut bacteria and their products can spread more easily and become exposed to the immune system due to disruption of the intestinal barrier (“leaky gut” phenomenon). This may be associated with loss of tolerance to bacterial antigens and triggering a local immune response as well as generalized autoimmune activity. Pro-inflammatory cytokines (e.g. IFN-γ and IL-17) may affect function of the intestinal tight junctions and contribute to disruption of the gut barrier. As stated above, intestinal integrity may also be compromised by metabolic alterations (e.g. decreased level of PUFA or increased level of urolithin). Overall, “leaky gut” may result from intestinal dysbiosis but also enhance its consequences, which highlights the complexity and reciprocal character of the links between the gut microbiome and inflammatory processes.Citation8,Citation75,Citation76
Impact of disease-modifying treatment on the gut microbiome in MS
According to current therapeutic standards, immediately after establishing a definite diagnosis of MS, DMT should be initiated, aimed at gaining control over MS activity and sustaining the stable condition of the patients. Due to significant progress in the knowledge about MS background, currently several DMTs are available, differing in efficacy and immunomodulatory/immunosuppressive properties and thus enabling individualized therapeutic strategies. However, DMTs are mainly appropriate for RRMS and targeted at inflammatory processes, while treatment of the progressive phase of MS, including neuroprotective and remyelinating effects, still remains a challenge. Therefore, there is an ongoing investigation in this field, in search for supportive or novel therapeutic options.
In the already mentioned large study comprising MS patients paired with household HSCitation41, the composition of the gut microbiome in pwMS differed between the untreated ones and those receiving DMT. Some of the alterations in bacterial taxa found in the untreated MS subgroup in comparison with HS (including Parabacteroides and Akkermansia species) were not replicated in the subgroup treated with DMT. Furthermore, the use of DMTs was associated with changes in microbial species which did not differentiate untreated MS subjects from controls (e.g. reduction in Bacteroides, Clostridium, Roseburia, Prevotella and Blautia species and increase in Phascolarctobacterium and Eubacterium).
The effects of particular DMTs on the gut microbiome have been studied before but usually in small and/or heterogeneous groups (). Diversity in DMT mode of action and way of administration (injectable or oral) must be considered.
Table 6. Modulation of the gut microbiota in MS patients after specified disease-modifying treatment.
Treatment with IFN-β was associated with lower microbial richness, especially decreased presence of Ruminococcus sp., Clostridium sp., F. prausnitzii, Roseburia, but with a concomitant increase in Parabacteroides distasonis and Bacteroides uniformis.Citation39,Citation41 Increased abundance of Prevotella (comparable to HS) has also been occasionally noted. Interestingly, administration of Prevotella histicola to transgenic mice suppressed EAE as effectively as IFN-β, while the combination of the probiotic and the drug did not further increase the effectiveness of treatment.Citation122,Citation127 A similar effect on abundance of Prevotella was observed in MS patients treated with glatiramer acetate (GA).Citation127 In the experimental model, administration of GA to the EAE mice was associated with an increase in gut Prevotella, and combined treatment with the probiotic and GA attenuated the disease more than GA alone.Citation25 In clinical studies, treatment with GA was linked with decreases in Sutterella and two Clostridial families – Lachnospiraceae and VeillonellaceaeCitation123 – as well as increases in atypical forms of E. coli, Enterobacter, Proteus, and Parvimonas micra.Citation124
Similarly to the GA effect, decreased abundance of Lachnospiraceae and Veillonellaceae was observed during treatment with dimethyl fumarate (DMF), additionally accompanied by a decrease in Firmicutes and Fusobacteria and an increase in Bacteroidetes.Citation123 DMF was also found to specifically reduce Bacteroides stercoris, Clostridium, and Eubacterium species,Citation41 and tended to normalize the low abundance of Faecalibacterium levelCitation124 and short-term depletion of Bifidobacterium,Citation27 though these effects were not consistent throughout the studies. Furthermore, DMF and agents with similar chemical structure (α, β unsaturated carbonyls) were found to inhibit the in vitro growth of Clostridium perfringens.Citation128 Fingolimod and its homolog sphingosine were also found to be potent inhibitors of C. perfringens.Citation128 Other effects of fingolimod on the gut microbiome included increases in atypical forms of E. coli, Enterobacter, Proteus and Parvimonas micra,Citation124 as well as reductions in Bacteroides finegoldii, Roseburia faecis and Blautia species.Citation41
There is little evidence of other DMTs’ impact on microbial composition. Treatment with natalizumab was associated with reductions in Bacteroides uniformis, Prevotella species and Bifidobacterium longum and an increase in Phascolarctobacterium sp., while decreases of Bacteroides finegoldii and Blautia sp. were observed in patients treated with anti-CD20 antibodies.Citation41 The effects of alemtuzumab were observed only in the monkey model of EAE, with profound alterations in Lactobacillales, Enterobacterales, Clostridiales, Prevotella and Faecalibacterium abundance.Citation129
Despite some diversity in the reported effects of DMT on particular bacterial taxa, their overall impact is associated with reshaping composition of the gut microbiota in favor of anti-inflammatory strains. It was suggested that DMTs exert their immunomodulatory/immunosuppressive effects within the intestinal environment. IFN-β and teriflunomide were observed to mediate local proliferation of Tregs. Fingolimod was demonstrated to regulate migration of lymphoid cells from intestinal lamina propria and regulate maturation of plasmablasts in Peyer’s patches. By blocking migration of T cells, natalizumab was suggested to reduce their exposure to microbial antigens and subsequent activation and expansion. Some of the DMTs (DMF, GA and alemtuzumab) have also been found to stabilize the intestinal barrier and promote tissue integrity.Citation15,Citation123
Other mechanisms explaining DMT effects on the gut microbiota seemed to include shared metabolic pathways. Pathways of bacterial metabolism of retinol and methane were identified as targets of DMF and GA.Citation123 As fumarates are degraded in the citrate cycle, an increase in late citrate cycle intermediates was observed during treatment with DMF, both in the patients’ serum and in the gut microbes’ metabolism.Citation130 The inhibitory effect of DMF on Clostridium perfringens growth was neutralized by glutathione (having anti-oxidative properties), which suggests modulation of oxidative stress as another potential link between DMT and bacteria.Citation128 Findings from a large panel of metabolomic analysis in serum and stools of MS patientsCitation41 demonstrated that pathways related to synthesis of lysine, L-ornithine, sugar nucleotides and unsaturated fatty acids were specifically modulated by particular DMTs. The most remarkable alterations were induced by fingolimod and IFN-β. Depletion of F. prausnitzii, a bacterium producing SCFA, and microbial pathways leading to pyruvate production was suggested to account for the low level of pyruvate, acetate and propionate in feces and serum from MS patients receiving these therapies. Increased absorption of propionate derived from bacteria, via upregulation of the SCFA transporter MCT1, was also hypothesized as a contributing mechanism.Citation41
An interesting thread of investigation focused on the relationships between alterations in gut microbiota and side effects of DMT. With regard to DMF, one of the pilot studiesCitation27 failed to link microbial composition with gastrointestinal complaints, common in the early phase of treatment. Interestingly, in another study,Citation130 it was found that a particular baseline microbiome signature (presence of A. muciniphila and absence of P. copri) could predict occurrence of lymphopenia, a relevant side effect of DMF.
The findings from studies on DMT’s impact on the gut microbiome encouraged further investigation into the therapeutic interventions modifying microbial composition, which would complement treatment with DMT (optimizing response to treatment, compromising their side effects) or affect other disease outcomes, not addressed so far.
Pharmacological interventions targeting the gut microbiome in MS
Probiotics
Probiotics are live microorganisms which confer a health benefit on the host, when administered in adequate amounts. They can interact with the gut microbiome and have an impact on the immunological system and the CNS function (e.g. through neurotrophic factors and neurotransmitters activity).Citation131 Thus investigation of the role of probiotic supplementation affecting the course of MS has been undertaken.
Studies on animal models demonstrated some positive effects of Lactobacillus, Escherichia coli and Prevotella strains (administered orally or intraperitoneally), which prevented development of EAE or ameliorated its course.Citation132
Marmoset twins were divided into two groups and fed either a yogurt-based (YBD) or water-based (WBD) diet before being immunized. The twins on YBD had less demyelination and a reduced pro-inflammatory response from T cells, B cells and cytokines. Some of the marmosets on YBD did not display any symptoms of EAE. The composition of the gut microbiome was only altered in these marmosets after they were immunized, likely due to the interplay between their diet and immune system responses.Citation133
Administration of a probiotic cocktail to mice during chronic phase of TMEV infection reduced severity of the disease and emerging motor disability, as well as affecting composition of the gut microbiota, with increased abundance of Bacteroidetes, Actinobacteria, Tenericutes and TM7 taxa. Related findings included reduced gliosis, infiltration of leukocytes and expression of IL-1β and IL-6 in the CNS and increased levels of butyrate and acetate in plasma.Citation134
Probiotics were reported to promote integrity of the intestinal barrier and have an immunomodulatory effect (increasing the level of regulatory and anti-inflammatory cell subsets and their products and suppressing pro-inflammatory ones).Citation135 As discussed before, bacterial metabolites (e.g. SCFAs) might also contribute to the reduction of regional and systemic immune-mediated responses.Citation136 However, some contradictory findings were also reported, with Lactobacillus reuteri strains contributing to exacerbation of EAE, probably as a result of interaction with other bacteria and activation of molecular mimicry.Citation132
There is scarce evidence for beneficial effect of probiotics in pwMS. The results of a few clinical trials showed that administration of probiotics (e.g. capsules containing Lactobacillus and Bifidobacterium strains) was associated with improvement in measures of disability, depression and general health, as well as reduced expression of pro-inflammatory cytokines, and favorable effects on markers of insulin resistance, profile of lipids and NO metabolites.Citation137–139
The study of Tankou et al.Citation140 focused particularly on probiotics’ impact on the gut microbiome. Following administration of a probiotic mix (Lactobacillus, Bifidobacterium and Streptococcus) for two months, the composition of microbiota changed in MS patients and HS with an increase in the relative abundance of mentioned species and a decrease in α-diversity. Reduced expression of CD14 and CD80 on peripheral monocytes was also observed. Microbial and immunological alterations ceased after withdrawal of probiotics. Due to the relatively small sample size, short duration and a number of confounding factors (dietary habits, DMT used), the relevance of findings from these trials is limited and further studies seem to be necessary.Citation8
Antibiotics
Bactericidal or bacteriostatic effects of antibiotics are bound to influence the gut microbiome; therefore antibiotics have been considered as possibly beneficial interventions in MS.
In several studies on experimental models of CNS autoimmune inflammation, oral administration of broad spectrum antibiotics before immunization prevented or ameliorated the disease.Citation141,Citation142
Gut dysbiosis was found in a non-obese diabetic murine model of EAE, resembling SPMS. Diminished disease severity and mortality in mice treated with a mixture of broad-spectrum antibiotics suggested reciprocal effects between CNS inflammatory demyelination and composition of the gut microbiome.Citation45
Modifications of gut microbiota in EAE mice (decreased phylogenetic diversity and richness, lowered Firmicutes/Bacteroidetes ratio) were associated with an increased Treg responseCitation143 and altered function of iNKT cells.Citation144 Following antibiotic administration, suppression of molecules produced by L. reuteri and Erysipelotrichaceae was also observed and a subsequent decrease in accumulation of MOG specific Th17 cells.Citation145 The oral administration of antibiotics to mice infected with TMEV induced profound but temporary dysbiosis and modified immune-mediated responses, with lower levels of CD4+ and CD8+T cells in the CNS and in peripheral lymph nodes. Moreover, increased mortality in the course of TMEV infection was observed after cessation of antibiotics.Citation57
However, the impact of antibiotics was limited when they were used during already established autoimmune inflammation, and so was their effect on oligodendrocyte progenitor cell differentiation and remyelination.Citation146 In contrast to oral treatment, intraperitoneal administration of ampicillin caused worsening of EAE; a concomitant decrease in bacteria able to transform tryptophan into AhR agonists indicated a potential pathomechanism.Citation101
These findings were not translated into clinical investigations. There were inconsistent results from the studies analyzing the relationships between risk of MS and use of antibiotics due to infections.Citation147,Citation148 Two small trials showed a positive effect of doxycycline (combined with IFN-β) or minocycline on clinical and radiological indices of MS activity, but the composition of gut microbiota was not taken into account.Citation149,Citation150 Despite some promising results, adverse effects of long-term treatment with antibiotics (including growth of opportunistic and resistant pathogens) are a significant barrier to use of these interventions in pwMS.
Dietary interventions
Despite established the substantial role of pharmacological treatment (mainly DMT) in management of MS, there is still ongoing interest in the supportive role of dietary interventions and their ability to influence MS-related outcomes. Particular components of diet or dietary regimens were investigated in view of their impact on the course of disease (frequency and severity of relapses), its particular symptoms (e.g. pain or fatigue), but also general health issues and quality of life. Due to diversity in design of the trials and investigated variables, consistent and conclusive evidence of the benefit from dietary intervention in pwMS is still lacking.Citation151
A significant thread of investigation of dietary interventions in MS is focused on their interaction with the gut microbiome through multiple pathways. Particular diet compounds, as metabolic substrates, may directly support the growth of some bacterial strains and inhibit others, thus shaping the microbial composition. Indirect effects include the impact of diet on host-microbiota immune interactions, e.g. the immune sampling within intestinal Peyer’s patches. Furthermore, diet may affect integrity and function of the intestinal barrier. Considering these links and potential to target the gut microbiome with various dietary interventions, their putative role in a complex therapeutic approach to MS has been investigated ().Citation152,Citation153
Figure 5. Effects of dietary intervention upon the gut dysbiosis and processes involved in MS pathology.
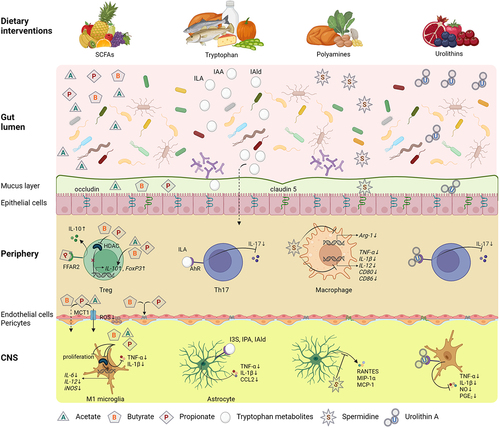
Dietary components
Vitamin D
A low level of vitamin D is considered as a risk factor for MS development and activity, with moderate strength of evidence from randomized trials.Citation2,Citation154 Some beneficial effects of vitamin D on inflammatory markers and clinical and/or radiological measures of MS activity have been reported.Citation155,Citation156 However, the concomitant effects of DMT, as well as interactions between sun exposure, geographical distribution and dietary intake of vitamin D, must be considered.Citation2
With regard to links with the gut microbiome, it is known that deficiency of vitamin D3 affects intestinal calcium absorption, disturbs gut homeostasis and increases intestinal permeability for bacteria and their products, which results in a concomitant rise in bacterial toxins.Citation14 Investigation of the gut microbiome in HS with vitamin D deficiency showed only temporary changes in the Firmicutes/Bacteroidetes ratio during the restoration of vitamin level, while microbial α-diversity and β-diversity did not change throughout the study.Citation157 Following vitamin D supplementation in overweight/obese healthy adults, higher abundance of Lachnospira and lower abundance of Blautia were observed.Citation158 A study evaluating the effect of vitamin D supplementation on the bacterial community in MS female patients (untreated or receiving GA) showed an increase in Faecalibacterium, Akkermansia and Coprococcus genera in the untreated subgroup.Citation25
Prebiotics
Prebiotics – the substrates used by intestinal bacteria – include fructans, galactooligosaccharides and so-called resistant starch. Indigestible for the host, they can be degraded by bacterial strains to products which feed other bacteria or enter further metabolic pathways, e.g. folates, indoles, secondary bile acids, trimethylamine-N-oxide (TMAO), lactate, succinate and SCFA. Administration of prebiotics may modulate the composition of the gut microbiome, supporting an increase in the number of Bifidobacteria and other SCFA producers. Furthermore, prebiotics can interact with the receptors of immune cells and induce cytokine expression.Citation159
In an animal model, administration of dietary non-fermentable fiber, which prevents the development of EAE, was shown both to affect the composition of gut microbiota (overall decrease in diversity, with increased abundance of Ruminococcaceae, Helicobacteraceae and Enterococcaceae and reduced abundance of Sutterellaceae, Lactobacillaceae and Coriobacteriaceae) and to alter the metabolic profile, with an increase in long-chain fatty acids, reportedly promoting suppressive Th2 responses.Citation160 In a clinical study, an association was detected between the dietary fiber intake in MS subjects and indices of systemic inflammation, anthropometric measures and disability level.Citation161
SCFAs
As discussed earlier, a reduced level of SCFAs in MS subjects, possibly associated with alterations in the gut microbiome, might indicate beneficial effects of SCFA supplementation. Duscha et al.Citation63 investigated this concept in a large cohort of MS subjects (including different disease types and DMT used) and noted initial depletion of Butyricimonas (SCFA producers) and enrichment of Flavonifractor, Escherichia/Shigella and Collinsella (promoted by low-SCFA conditions), accompanied by reduced amounts of propionic acid in the serum and stool of the patients (especially in treatment-naïve ones). Oral supplementation with propionic acid, believed to restore gut eubiosis, was found to cause induction of Tregs’ suppressive function and decrease Th1/Th17. Furthermore, it improved clinical measures of the disease, including the relapse rate and progression of disability.
Animal model studies showed that administration of SCFAs, specifically acetate, ameliorated the severity of EAE in an IL-10-dependent fashion.Citation88 Similar findings indicated that colonization of microbiota derived from MS patients resulted in upregulated gene expression related with Tregs, but only when it was preceded by treatment with propionate.Citation62 Furthermore, reduced CNS inflammation and demyelination were observed after preventive treatment with butyrateCitation162 and propionate.Citation62 Intriguingly, butyrate administration after disease onset had little impact on disease course,Citation162 while propionate treatment in the same fashion resulted in recovery of axonal density, even though in the preventive approach butyrate reduced demyelination and immune cell infiltration.Citation62 Thus immunomodulatory properties of SCFAs and their link with the gut microbiome may indicate a therapeutic potential for MS.
PUFAs
Polyunsaturated fatty acids (particularly omega-3 PUFA) are contained in plant or marine derived food products but also widely available as supplements of diet.
Studies on experimental models of MS have indicated a beneficial effect of PUFA exerted by their immunomodulatory features, inhibiting peripheral and CNS T cells’ activity. They were shown to prevent demyelinationCitation163 as well as promote neuroprotection and remyelination,Citation164 which corresponded with amelioration of the disease and delay in its onset.Citation165 Conjugated linolenic acid (CLA) supplementation caused an increase in myeloid-derived suppressor cells in the intestinal lamina propria, which was associated with suppression of inflammation. Principal component analysis and relative abundance of types of the gut microbiome revealed profound differences, e.g. increases in Porphyromonadaceae, Lachnospiraceae, Bacteroides, Lactobacillus and Akkermansia in affected animals. However, antibiotic treatment did not abrogate beneficial effects of CLA on the course of EAE, which challenges the concept of the gut microbiome mediating this impact.Citation165
Clinical studies concerning effects of PUFA on MS outcomes have not been as conclusive as those on animal models and have produced conflicting results.Citation166,Citation167 The study by Fleck et al. showed that CLA intake in RRMS patients on DMT significantly improved the anti-inflammatory profiles and functional signatures of circulating myeloid cells. However, the composition of the human gut microbiome was not analyzed.Citation165
Polyphenols
Polyphenols (together with other plant-derived compounds) may affect the composition of gut microbiota. The studies on HS revealed that polyphenols promote growth of Clostridia, Bifidobacteria and Lactobacilli and reduce the abundance of pathogenic strains of Clostridium and Bacteroides.Citation168
So far, the effects of polyphenols have been examined in the experimental model of EAE, with partly contradictory results. Flavonoids have been reported to lower levels of pro-inflammatory cytokines and to alleviate symptoms of the disease,Citation169–171 but also to delay recovery from its acute phase.Citation172 Resveratrol was associated with exacerbation of EAE,Citation173 yet supported remyelination in another preclinical MS model.Citation174 There is scarce evidence from clinical studies of beneficial effects of polyphenols in MS. Treatment with nanocurcumin was reported to decrease the expression of pro-inflammatory cytokines and Th17 related parameters, increase Treg activity and restore the expression profile of miRNA, as well as to improve the level of disability and quality of life in RRMS subjects.Citation175 However, the direct impact of polyphenols on the gut microbiome and their emerging therapeutic potential in MS need further investigations.
Sodium chloride
Increased NaCl intake, typical for the “western diet” (WD), affects local and systemic tissue inflammation and may alter the composition and function of the intestinal microbiome. Research has shown that a high concentration of NaCl in the extracellular environment leads to increased amounts of Lachnospiraceae, Ruminococcus and Prevotella spp. as well as decreased numbers of Lactobacillus in the gut of EAE mice.Citation176,Citation177 It has also been demonstrated that an increased NaCl level results in dysregulation of immune homeostasis, an example of which is preferred activation of pro-inflammatory M1 macrophages and Th17 cells and suppressed induction of anti-inflammatory M2 macrophages and Tregs, specifically in the gut lamina propria.Citation178 The proposed scenario in this setting is as follows: depletion of Lactobacillus impedes the integrity of the intestinal barrier, prevents production of anti-inflammatory butyrate and coincides with increased Th17 and decreased Treg activity, most likely mediated by decreased production of indole-3-lactic acid, a tryptophan metabolite.Citation179 These processes ultimately lead to exacerbation of EAE, which can be reversed or ameliorated following administration of Lactobacillus.Citation98
Clinical studies on NaCl relevance for MS are quite limited and their results have been inconsistent. Farez et al. reported that a diet enriched in NaCl was related to increased numbers of demyelinating lesions in MRI and a higher relapse rate.Citation180 However, other reports did not reveal an effect of high NaCl intake on the risk of MS development or time to subsequent relapse.Citation181–183 These studies did not investigate the impact of NaCl dietary intake in pwMS on gut dysbiosis.
Dietary regimens
Intermittent fasting and ketogenic diet
Chronic or intermittent restriction in food intake (with or without malnutrition) and a ketogenic diet, which mimics fasting conditions, are associated with the formation of ketone bodies, which are an alternative energy source. These conditions affect signaling pathways involved in inflammatory processes and oxidative stress (e.g. FoxO transcription factors, sirtuins, antioxidant enzymes) and stimulate expression of neurotrophic factors. Dietary restriction modifies the gut microbiome by inducing enrichment in anti-inflammatory strains and decreases in pro-inflammatory bacterial products. The impact of these dietary interventions on the CNS is further mediated by activation of the endocrine and autonomic function.Citation184,Citation185
The beneficial effects of fasting and a ketogenic diet have been demonstrated in several studies on a murine EAE model.Citation186–188 These dietary interventions delayed the disease onset and attenuated or even reversed its motor and cognitive symptoms. Clinical improvement was accompanied by increased levels of corticosterone, adiponectin and immunoregulatory markers, while pro-inflammatory cytokines and cell subsets, as well as the production of reactive oxygen species, were suppressed. Furthermore, pathologic findings included a decrease in inflammatory infiltration and demyelination in the spinal cord, with the promotion of remyelination and regeneration of oligodendrocyte precursors.
In the study by Cignarella et al.,Citation189 fasting was found to result in enrichment of the Bacteroidaceae, Prevotellaceae and Lactobacillaceae families in the murine intestine, with enhanced microbial antioxidant metabolic pathways. Interestingly, FMT from mice on intermittent fasting improved the course of EAE in the mice on a normal diet, which highlights the role of the gut microbiome in the impact of fasting on the CNS autoimmune condition.
Although the effects of various fasting regimens on outcomes of MS have been investigated, few of these studies considered their link with gut microbiota.
Cignarella et al., based on their findings from an animal model, undertook a pilot clinical trial with pwMS during a relapse, who were treated with corticosteroids and underwent intermittent fasting versus an optional diet. The abundance of Faecalibacterium, Lachnospiracea incertae sedis and Blautia in gut microbiota showed an increasing trend after intermittent fasting, and the level of Faecalibacterium correlated with a concomitant decrease in leptin.Citation189
Swidsinski et al. analyzed the composition of the gut microbiome in MS patients during 6 months of a ketogenic diet. The total concentrations and diversity of bacterial groups were reduced at baseline. During the dietary intervention, these indices further decreased, but they returned to baseline values after 12 weeks and exceeded them significantly by weeks 23–24, approaching the values in HS. These fluctuations were especially relevant for Akkermansia strains.Citation22
An ongoing clinical trial investigating clinical, immunological and metabolic outcomes of MS patients using fasting or a ketogenic diet is expected to provide further evidence of the links between the gut microbiome and these dietary interventions in MS.Citation190
Other types of diet
In a longitudinal study of untreated RRMS subjects, Cantoni et al.Citation191 analyzed relationships between the gut microbiome, immune and metabolic parameters, diet and clinical outcomes of the disease. Although alterations in the gut microbiome and corresponding immune dysregulation were found in MS subjects in comparison with HS, they were not affected by the overall composition of dietary intake. However, a specific link was identified between the consumption of meat with abundance of meat-associated blood metabolites and a decreased amount of Bacteroides thetaiotaomicron (a polysaccharide-digesting bacterium), as well as an increase in Th17 cells.
Saresella et al. analyzed gut microbiota composition and immunological profiles in two groups of MS patients, on a high-vegetable/low-protein (HV/LP) diet or on WD. The results showed that Lachnospiraceae family members were significantly more abundant while the number of IL-17 producing T cells was lower in the HV/LP group compared to the WD group. The HV/LP diet also impacted clinical measures: relapse rate and level of disability.Citation192
Among other diet regimens considered as potentially beneficial in MS, the modified Paleolithic,Citation193 elimination Wahls/SwankCitation194 and low-fat plant-based dietCitation195 have been tested in randomized clinical trials. The results, though inconsistent, showed some improvement in measures of manual dexterity, fatigue and quality of life. However, no effects of dietary interventions on the gut microbiome were evaluated in these studies.
Faecal microbiota transplantation
FMT is an infusion of crafted stool samples from a healthy donor into the gastrointestinal tract of the recipient, in order to restore microbial balance (eubiosis). FMT has primarily been used in the treatment of Clostridium difficile infection as well as other inflammatory or functional disorders of the gastrointestinal tract.Citation196,Citation197 Furthermore, potential FMT effects have also been investigated in systemic autoimmune diseases and disorders with primary or secondary CNS involvement.Citation198,Citation199
Regarding experimental studies on MS, FMT from healthy to immunized mice resulted in altered composition of their gut bacterial taxa and was associated with milder severity of the EAE symptoms, reduced expression of markers of neurodegeneration and diminished pathological symptoms (demyelination and axonal loss).Citation200 An interesting study by Berer et al. conducted colonization of SJL mice (an animal model of spontaneous RRMS) with gut microbiota from human twins discordant for diagnosis of MS.Citation31 Worsening of disease was observed only after application of stools derived from MS-affected individuals, accompanied by abundance of Sutterella strains and an enhanced immunoregulatory profile.
Investigation of the therapeutic potential of FMT in MS was encouraged by case reports, describing MS patients who were treated with this method due to severe gastrointestinal problems.Citation201 Following FMT, they experienced a long-term (up to several years) stabilization of the disease course, with minimal improvement in disability and functional scores. A more recent single-subject studyCitation202, based on a year-long follow-up of an MS patient after double FMT, provided evidence for possible mechanisms of the beneficial effect of FMT. Improvement in measures of gait was accompanied by altered microbial parameters (abundance of Faecalibacterium prausnitzii, increased ratios of Firmicutes-to-Bacteroidetes and Prevotellaceae-to-Bacteroidaceae), increased concentrations of acetate, propionate and butyrate in successive stool samples, and enhancement of SCFA genomic pathways, which correlated with the serum level of brain derived neurotrophic factor.
A pilot randomized controlled trial was performed on a small group of RRMS patients, who received monthly FMTs as an early or late intervention for up to 6 months.Citation203 Donor-specific alterations in gut microbiome composition were found after FMT, although high intraindividual variability prevented significance of modifications in α- and β-diversity of species. Elevated intestinal permeability in some of the subjects was normalized following a series of FMT. No significant changes in serum levels of pro-inflammatory and immunoregulatory biomarkers were observed throughout the study. However, the trial was prematurely stopped for non-medical reasons, so not all the planned outcomes were achieved.
Further studies (including ongoing phase 1 clinical trials) are expected to provide more data about the effects, tolerance and optimal procedure of FMT, which would further support implementation of this method in MS treatment.
Other interventions
According to some reports, parasite infections could ameliorate clinical and radiological activity in MS. This effect (reversed by anti-helminth drugs) was attributed to induction of Tregs and secretion of immunosuppressive cytokines.Citation204,Citation205 Attempts have been made to evaluate the impact of intentional human hookworm infection on gut dysbiosis in MS, considering possible interactions between these microorganisms. Jenkins et al.Citation206 observed significantly increased α-diversity in fecal samples of infected pwMS compared to the placebo group and differences in the abundances of a few bacterial taxa (e.g. Parabacteroides) which probably possess immunomodulatory functions. In the placebo group, greater abundance of the Lachnospiraceae family was found, considered to be associated with a shift toward a pro-inflammatory microbial profile and emerging disease progression. This observation seems contradictory to the previous findings because Lachnospiraceae members are SCFA producers.
Another interesting attempt to target the gut microbiome in pwMS was associated with physical activity. Barone et al.Citation207 evaluated the influence of a multidimensional rehabilitation programme on various MS outcomes. The intervention resulted in improved gait efficiency and reduced fatigue, accompanied by a decrease in pro-inflammatory immune markers (CD4+/IFN-γ+Th1, CD4+/ROR-γ+ and CD4+/IL-17+ Th17) and serum concentration of lipopolysaccharide. In addition, significant changes were observed in the composition of gut microbiota. At baseline, pwMS presented with depletion in several bacterial families (Lachnospiraceae and Ruminococcaceae) and enrichment in others (Coriobacteriaceae, Veillonellaceae, Prevotellaceae, Enterobacteriaceae). Concerning their functions, there was a decrease in bacteria producing SCFA (Roseburia, Coprococcus and Blautia) and an increase in those exhibiting pro-inflammatory activity (Collinsella and Prevotella). After the rehabilitation programme, specific alterations of the gut microbiome included relative abundance of Actinobacteria (similar to HS), sustained predomination of Bacteroidetes and Proteobacteria over Firmicutes, as well as lowered levels (below reference values for HS) of Ruminococcus and Dorea (SCFA producers, pro-inflammatory profile). Furthermore, the decrease of Collinsella positively correlated with CD4+/ROR-γ+ Th17 levels, suggesting a link between this bacterial genus and the pro-inflammatory component of the autoimmune response.
Conclusions
Altered composition and function of the gut microbiota, demonstrated in pwMS, are suggested to play a significant role in the complex background of the disease. Experimental and clinical studies have revealed the links between gut dysbiosis and a dysregulated immune response (mainly predominance of its pro-inflammatory components over regulatory ones) as well as bacterial metabolic pathways. Analysis of these host-bacteria interactions may provide a better insight into the processes contributing to MS development and affecting its course. Furthermore, investigation of gut dysbiosis in MS may enable clarification of currently known therapeutic targets and hopefully reveal potential new ones. Besides DMT, other interventions, targeted at gut microbiota, apparently have some potential to affect the activity and the course of the disease (). Due to problems with the translation of experimental findings into clinical models, further investigation and randomized trials are necessary to verify the beneficial effects of these interventions in MS.
Table 7. Experimental and clinical studies on gut commensal-based therapies and dietary interventions affecting gut microbiome in MS.
Abbreviations
AhR | = | aryl hydrocarbon receptor |
Akt | = | serine/threonine protein kinase |
AMPK | = | adenosine monophosphate-activated protein kinase |
APC | = | antigen presenting cell |
Arg-1 | = | arginase 1 |
BACE1 | = | β-site amyloid precursor protein cleaving enzyme 1 |
BBB | = | blood-brain barrier |
BDNF | = | brain derived neurotrophic factor |
CCL2 | = | chemokine (C-C motif) ligand 2 |
CD | = | Crohn’s disease |
CNS | = | central nervous system |
cPLA2 | = | cytosolic phospholipase A2 |
CSF | = | cerebrospinal fluid |
DC | = | dendritic cell |
DMF | = | dimethyl fumarate |
DMT | = | disease modifying therapy |
EAE | = | experimental autoimmune encephalomyelitis |
EDSS | = | expanded disability status scale |
ERK | = | extracellular signal-regulated kinase |
FFAR | = | free fatty acid receptor |
FMT | = | fecal microbiota transplantation |
FoxP3 | = | forkhead box P3 |
FTY720 | = | fingolimod |
GA | = | glatiramer acetate |
GF | = | germ-free |
GPR | = | G protein coupled receptor |
HDAC | = | histone deacetylase |
HLA | = | human leukocyte antigen |
HS | = | healthy subjects |
I3C | = | indole-3-carbinol |
I3S | = | indole-3-sulfate |
IAA | = | indole-3-acetic acid |
IAld | = | indole-3-aldehyde |
IDO | = | 1-indolamine 2,3-dioxygenase |
IER | = | intermittent energy restriction |
IL | = | interleukin |
ILA | = | indole-3-lactic acid |
iNKT | = | invariant natural killer T cells |
iNOS | = | induced nitric oxide synthase |
IPA | = | indole-3-propionic acid |
JNK | = | Jun N-terminal kinase |
LCFAs | = | long chain fatty acids |
LFA-1 | = | lymphocyte function associated antigen 1 |
MAPK | = | mitogen-activated protein kinase |
MBP | = | myelin basic protein |
MCP-1 | = | monocyte chemoattractant protein-1 |
MCT1 | = | proton-dependent monocarboxylate transporter 1 |
MHC-II | = | major histocompatibility complex class II |
MIP-1α | = | macrophage inflammatory protein-1α |
MOG | = | myelin oligodendrocyte protein |
MS | = | multiple sclerosis |
mTOR | = | mammalian target of rapamycin |
NFE2L2 | = | nuclear factor erythroid‐derived 2‐like 2 |
NFκB | = | nuclear factor kappa light chain enhancer of activated B cells |
NMOSD | = | neuromyelitis optica spectrum disorder |
NO | = | nitric oxide |
Nrf2 | = | nuclear factor erythroid 2-related factor 2 |
NZ | = | natulizumab |
ODC-1 | = | ornithine decarboxylase 1 |
Orai1 | = | calcium release-activated calcium modulator 1 |
OSE | = | spontaneous opticospinal encephalomyelitis |
OUT | = | operational taxonomic unit |
PGE2 | = | prostaglandin E2 |
PI3K | = | phosphoinositide 3-kinase |
PPMS | = | primary progressive multiple sclerosis |
PUFAs | = | polyunsaturated fatty acids |
pwMS | = | people with multiple sclerosis |
PXR | = | pregnane X receptor |
RA | = | rheumatoid arthritis |
RANTES | = | regulated upon activation, normal T cell expressed and secreted |
(Rh) MOG | = | recombinant human myelin, oligodendrocyte glycoprotein |
ROS | = | reactive oxygen species |
RRMS | = | relapsing-remitting multiple sclerosis |
S1P | = | sphingosine 1-phosphate |
SAT-1 | = | spermidine/spermine N1 acetyltransferase 1 |
SCFAs | = | short chain fatty acids |
SMCT1 | = | sodium-coupled monocarboxylate transporter 1 |
SPMS | = | secondary progressive multiple sclerosis |
STIM | = | stromal cell interaction molecule |
Th | = | T helper |
TLR | = | toll-like receptor |
TMEV-IDD | = | Theiler’s murine encephalomyelitis virus (TMEV)-induced demyelinating disease |
TNF-α | = | tumor necrosis factor alpha |
Treg | = | regulatory T cell |
UC | = | ulcerative colitis |
VDR | = | Vitamin D Receptor |
VEGF-B | = | vascular endothelial growth factor B |
WD | = | western diet |
Acknowledgments
The authors greatly acknowledge the encouragement and support from Professor Sabina Gorska, Head of Laboratory of Microbiome Immunobiology, Ludwik Hirszfeld Institute of Immunology & Experimental Therapy, Polish Academy of Sciences, Wroclaw, Poland. All Figures were created using Biorender.com.
Disclosure statement
No potential conflict of interest was reported by the authors.
Data availability statement
Data sharing is not applicable to this article as no new data were created or analyzed in this study.
Correction Statement
This article has been corrected with minor changes. These changes do not impact the academic content of the article.
References
- Filippi M, Bar-Or A, Piehl F, Preziosa P, Solari A, Vukusic S, Rocca MA. Multiple sclerosis. Nat Rev Dis Primers. 2018;4(1):1–37. doi:10.1038/s41572-018-0041-4.
- Waubant E, Lucas R, Mowry E, Graves J, Olsson T, Alfredsson L, Langer‐Gould A. Environmental and genetic risk factors for MS: an integrated review. Ann Clin Transl Neurol. 2019;6(9):1905–1922. doi:10.1002/acn3.50862.
- Marrodan M, Alessandro L, Farez MF, Correale J. The role of infections in multiple sclerosis. Mult Scler. 2019;25(7):891–901. doi:10.1177/1352458518823940.
- Tarlinton RE, Khaibullin T, Granatov E, Martynova E, Rizvanov A, Khaiboullina S. The interaction between viral and environmental risk factors in the pathogenesis of multiple sclerosis. IJMS. 2019;20(2):303. doi:10.3390/ijms20020303.
- Bjornevik K, Cortese M, Healy BC, Kuhle J, Mina MJ, Leng Y, Elledge SJ, Niebuhr DW, Scher AI, Munger KL, et al. Longitudinal analysis reveals high prevalence of Epstein-Barr virus associated with multiple sclerosis. Sci. 2022;375(6578):296–301. doi:10.1126/science.abj8222.
- Yin JX, Tang Z, Gan Y, Li L, Shi F, Coons S, Shi J. Pertussis toxin modulates microglia and T cell profile to protect experimental autoimmune encephalomyelitis. Neuropharmacology. 2014;81:1–5. doi:10.1016/j.neuropharm.2014.01.027.
- Marchesi JR, Ravel J. The vocabulary of microbiome research: a proposal. Microbiome. 2015;3(1):31. doi:10.1186/s40168-015-0094-5.
- Ghezzi L, Cantoni C, Pinget GV, Zhou Y, Piccio L. Targeting the gut to treat multiple sclerosis. J Clin Invest. 2021;131(13). doi:10.1172/JCI143774.
- Shahi SK, Freedman SN, Mangalam AK. Gut microbiome in multiple sclerosis: the players involved and the roles they play. Gut Microbes. 2017;8(6):607–615. doi:10.1080/19490976.2017.1349041.
- Maglione A, Zuccala M, Tosi M, Clerico M, Rolla S. Host genetics and gut microbiome: perspectives for multiple sclerosis. Genes (Basel). 2021;12(8):1181. doi:10.3390/genes12081181.
- Shahi SK, Ali S, Jaime CM, Guseva NV, Mangalam AK. HLA class II polymorphisms modulate gut microbiota and experimental autoimmune encephalomyelitis phenotype. Immunohorizons. 2021;5(8):627–646. doi:10.4049/immunohorizons.2100024.
- Montgomery TL, Künstner A, Kennedy JJ, Fang Q, Asarian L, Culp-Hill R, D’Alessandro A, Teuscher C, Busch H, Krementsov DN, et al. Interactions between host genetics and gut microbiota determine susceptibility to CNS autoimmunity. Proc Natl Acad Sci U S A. 2020;117(44):27516–27527. doi:10.1073/pnas.2002817117.
- Wang J, Thingholm LB, Skiecevičienė J, Rausch P, Kummen M, Hov JR, Degenhardt F, Heinsen F-A, Rühlemann MC, Szymczak S, et al. Genome-wide association analysis identifies variation in vitamin D receptor and other host factors influencing the gut microbiota. Nat Genet. 2016;48(11):1396–1406. doi:10.1038/ng.3695.
- Ghareghani M, Reiter RJ, Zibara K, Farhadi NL, Vitamin D. Melatonin, and gut microbiota act in concert to initiate multiple sclerosis: a New mechanistic pathway. Front Immunol. 2018;9:2484. doi:10.3389/fimmu.2018.02484.
- Boziki MK, Kesidou E, Theotokis P, Mentis AFA, Karafoulidou E, Melnikov M, Sviridova A, Rogovski V, Boyko A, Grigoriadis N, et al. Microbiome in multiple sclerosis; where are we, what we know and do not know. Brain Sci. 2020;10(4):234. doi:10.3390/brainsci10040234.
- Inojosa H, Proschmann U, Akgun K, Ziemssen T. The need for a strategic therapeutic approach: multiple sclerosis in check. Ther Adv Chronic Dis. 2022;13:20406223211063032. doi:10.1177/20406223211063032.
- Weld-Blundell IV, Grech L, Learmonth YC, Marck CH. Lifestyle and complementary therapies in multiple sclerosis guidelines: systematic review. Acta Neurol Scand. 2022;145(4):379–392. doi:10.1111/ane.13574.
- Rumah KR, Linden J, Fischetti VA, Vartanian T, Esteban FJ. Isolation of Clostridium perfringens type B in an individual at first clinical presentation of multiple sclerosis provides clues for environmental triggers of the disease. PloS One. 2013;8(10):e76359. doi:10.1371/journal.pone.0076359.
- Cekanaviciute E, Yoo BB, Runia TF, Debelius JW, Singh S, Nelson CA, Kanner R, Bencosme Y, Lee YK, Hauser SL, et al. Gut bacteria from multiple sclerosis patients modulate human T cells and exacerbate symptoms in mouse models. Proc Natl Acad Sci U S A. 2017;114(40):10713–10718. doi:10.1073/pnas.1711235114.
- Baum K, Rejmus R, Dorffel Y. Commensal gut flora in MS: spatial organization and composition. Mult Scler. 2015;21:458–159.
- Levi I, Gurevich M, Perlman G, Magalashvili D, Menascu S, Bar N, Godneva A, Zahavi L, Chermon D, Kosower N, et al. Potential role of indolelactate and butyrate in multiple sclerosis revealed by integrated microbiome-metabolome analysis. Cell Rep Med. 2021;2(4):100246. doi:10.1016/j.xcrm.2021.100246.
- Swidsinski A, Dörffel Y, Loening-Baucke V, Gille C, Göktas Ö, Reißhauer A, Neuhaus J, Weylandt K-H, Guschin A, Bock M, et al. Reduced Mass and diversity of the colonic microbiome in patients with multiple sclerosis and their improvement with Ketogenic Diet. Front Microbiol. 2017;8:1141. doi:10.3389/fmicb.2017.01141.
- Cosorich I, Dalla-Costa G, Sorini C, Ferrarese R, Messina MJ, Dolpady J, Radice E, Mariani A, Testoni PA, Canducci F, et al. High frequency of intestinal T H 17 cells correlates with microbiota alterations and disease activity in multiple sclerosis. Sci Adv. 2017;3(7):e1700492. doi:10.1126/sciadv.1700492.
- Miyake S, Kim S, Suda W, Oshima K, Nakamura M, Matsuoka T, Chihara N, Tomita A, Sato W, Kim S-W, et al. Dysbiosis in the gut microbiota of patients with multiple sclerosis, with a striking depletion of species belonging to Clostridia XIVa and IV clusters. PloS One. 2015;10(9):e0137429. doi:10.1371/journal.pone.0137429.
- Cantarel BL, Waubant E, Chehoud C, Kuczynski J, DeSantis TZ, Warrington J, Venkatesan A, Fraser CM, Mowry EM. Gut microbiota in multiple sclerosis: possible influence of immunomodulators. Journal Of Investigative Medicine. 2015;63(5):729–734. doi:10.1097/JIM.0000000000000192.
- Ling Z, Cheng Y, Yan X, Shao L, Liu X, Zhou D, Zhang L, Yu K, Zhao L, et al. Alterations of the fecal microbiota in Chinese patients with multiple sclerosis. Front Immunol. 2020;11:590783. doi:10.3389/fimmu.2020.590783.
- Storm-Larsen C, Myhr K-M, Farbu E, Midgard R, Nyquist K, Broch L, Berg-Hansen P, Buness A, Holm K, Ueland T, et al. Gut microbiota composition during a 12-week intervention with delayed-release dimethyl fumarate in multiple sclerosis – a pilot trial. Mult Scler J Exp Transl Clin. 2019;5(4):2055217319888767. doi:10.1177/2055217319888767.
- Ventura RE, Iizumi T, Battaglia T, Liu M, Perez-Perez GI, Herbert J, Blaser MJ. Gut microbiome of treatment-naïve MS patients of different ethnicities early in disease course. Sci Rep. 2019;9(1):16396. doi:10.1038/s41598-019-52894-z.
- Oezguen N, Yalcinkaya N, Kücükali CI, Dahdouli M, Hollister EB, Luna RA, Türkoglu R, Kürtüncü M, Eraksoy M, Savidge TC, et al. Microbiota stratification identifies disease-specific alterations in neuro-Behçet’s disease and multiple sclerosis. Clin Exp Rheumatol. 2019;37 Suppl 121(6):58–66.
- Saresella M, Marventano I, Barone M, La Rosa F, Piancone F, Mendozzi L, d’Arma A, Rossi V, Pugnetti L, Roda G, et al. Alterations in circulating fatty acid are associated with gut microbiota dysbiosis and inflammation in multiple sclerosis. Front Immunol. 2020;11:1390. doi:10.3389/fimmu.2020.01390.
- Berer K, Gerdes LA, Cekanaviciute E, Jia X, Xiao L, Xia Z, Liu C, Klotz L, Stauffer U, Baranzini SE, et al. Gut microbiota from multiple sclerosis patients enables spontaneous autoimmune encephalomyelitis in mice. Proc Natl Acad Sci U S A. 2017;114(40):10719–10724. doi:10.1073/pnas.1711233114.
- Cox LM, Maghzi AH, Liu S, Tankou SK, Dhang FH, Willocq V, Song A, Wasén C, Tauhid S, Chu R, et al. Gut microbiome in progressive multiple sclerosis. Ann Neurol. 2021;89(6):1195–1211. doi:10.1002/ana.26084.
- Takewaki D, Suda W, Sato W, Takayasu L, Kumar N, Kimura K, Kaga N, Mizuno T, Miyake S, Hattori M, et al. Alterations of the gut ecological and functional microenvironment in different stages of multiple sclerosis. Proc Natl Acad Sci U S A. 2020;117(36):22402–22412. doi:10.1073/pnas.2011703117.
- Cekanaviciute E, Debelius, JW, Singh S, Runia T, Nelson C, Yoo B, Kanner R, Crabtree-Hartman E, Mazmanian S, Knight R et al. Gut dysbiosis is a feature of MS and it is characterized by bacteria able to regulate lymphocyte differentiation in vitro. Mult Scler. 2016;22(S3):58–59.
- Zeng Q, Gong J, Liu X, Chen C, Sun X, Li H, Zhou Y, Cui C, Wang Y, Yang Y, et al. Gut dysbiosis and lack of short chain fatty acids in a Chinese cohort of patients with multiple sclerosis. Neurochem Int. 2019;129:104468. doi:10.1016/j.neuint.2019.104468.
- Forbes JD, Chen C-Y, Knox NC, Marrie R-A, El-Gabalawy H, de Kievit T, Alfa M, Bernstein CN, Van Domselaar G. A comparative study of the gut microbiota in immune-mediated inflammatory diseases—does a common dysbiosis exist? Microbiome. 2018;6(1):221. doi:10.1186/s40168-018-0603-4.
- Kozhieva M, Naumova N, Alikina T, Boyko A, Vlassov V, Kabilov MR. Primary progressive multiple sclerosis in a Russian cohort: relationship with gut bacterial diversity. BMC Microbiol. 2019;19(1):309. doi:10.1186/s12866-019-1685-2.
- Choileain SN, Kleinewietfeld M, Raddassi K, Hafler DA, Ruff WE, Longbrake EE. CXCR3+ T cells in multiple sclerosis correlate with reduced diversity of the gut microbiome. J Transl Autoimmunity. 2020;3:100032. doi:10.1016/j.jtauto.2019.100032.
- Reynders T, Devolder L, Valles‐Colomer M, Van Remoortel A, Joossens M, De Keyser J, Nagels G, D’hooghe M, Raes J. Gut microbiome variation is associated to multiple sclerosis phenotypic subtypes. Ann Clin Transl Neurol. 2020;7(4):406–419. doi:10.1002/acn3.51004.
- Zhou X, Singh S, Baumann R, Barba P, Landefeld J, Casaccia P, Sand IK, Xia Z, Weiner H, Chitnis T, et al. Household paired design reduces variance and increases power in multi-city gut microbiome study in multiple sclerosis. Mult Scler. 2021;27(3):366–379. doi:10.1177/1352458520924594.
- Zhou X, Baumann R, Gao X, Mendoza M, Singh S, Katz Sand I, Xia Z, Cox LM, Chitnis T, Yoon H. iMSMS & consortium. Gut microbiome of multiple sclerosis patients and paired household healthy controls reveal associations with disease risk and course. Cell. 2022;185(19):3467–3486.e16. doi:10.1016/j.cell.2022.08.021.
- Johanson DM 2nd, Goertz JE, Marin IA, Costello J, Overall CC, Gaultier A. Experimental autoimmune encephalomyelitis is associated with changes of the microbiota composition in the gastrointestinal tract. Science Reports. 2020;10(1):15183. doi:10.1038/s41598-020-72197-y.
- Gandy KAO, Zhang J, Nagarkatti P, Nagarkatti M. The role of gut microbiota in shaping the relapse-remitting and chronic-progressive forms of multiple sclerosis in mouse models. Sci Rep. 2019;9(1):6923. doi:10.1038/s41598-019-43356-7.
- Moles L, Egimendia A, Osorio-Querejeta I, Iparraguirre L, Alberro A, Suárez J, Sepúlveda L, Castillo-Triviño T, Muñoz-Culla M, Ramos-Cabrer P, et al. Gut microbiota changes in experimental autoimmune encephalomyelitis and cuprizone mice models. ACS Chem Neurosci. 2021;12(5):893–905. doi:10.1021/acschemneuro.0c00695.
- Colpitts SL, Kasper EJ, Keever A, Liljenberg C, Kirby T, Magori K, Kasper LH, Ochoa-Repáraz J. A bidirectional association between the gut microbiota and CNS disease in a biphasic murine model of multiple sclerosis. Gut Microbes. 2017;8(6):561–573. doi:10.1080/19490976.2017.1353843.
- Lee YK, Menezes JS, Umesaki Y, Mazmanian SK. Proinflammatory T-cell responses to gut microbiota promote experimental autoimmune encephalomyelitis. Proc Natl Acad Sci USA. 2011;Suppl 108(supplement_1):4615–4622. doi:10.1073/pnas.1000082107.
- Ochoa-Reparaz J, Mielcarz DW, Ditrio LE, Burroughs AR, Begum-Haque S, Dasgupta S, Kasper DL, Kasper LH. Central nervous system demyelinating disease protection by the human commensal Bacteroides fragilis depends on polysaccharide a expression. The Journal Of Immunology. 2010;185(7):4101–4108. doi:10.4049/jimmunol.1001443.
- Telesford KM, Yan W, Ochoa-Reparaz J, Pant A, Kircher C, Christy MA, Begum-Haque S, Kasper DL, Kasper LH. A commensal symbiotic factor derived from Bacteroides fragilis promotes human CD39 + Foxp3 + T cells and T reg function. Gut Microbes. 2015;6(4):234–242. doi:10.1080/19490976.2015.1056973.
- Yamashita M, Ukibe K, Matsubara Y, Hosoya T, Sakai F, Kon S, Arima Y, Murakami M, Nakagawa H, Miyazaki T, et al. Lactobacillus helveticus SBT2171 attenuates experimental autoimmune encephalomyelitis in mice. Front Microbiol. 2017;8:2596. doi:10.3389/fmicb.2017.02596.
- Lavasani S, Dzhambazov B, Nouri M, Fåk F, Buske S, Molin G, Thorlacius H, Alenfall J, Jeppsson B, Weström B, et al. A novel probiotic mixture exerts a therapeutic effect on experimental autoimmune encephalomyelitis mediated by IL-10 producing regulatory T cells. PloS One. 2010;5(2):e9009. doi:10.1371/journal.pone.0009009.
- He B, Hoang TK, Tian X, Taylor CM, Blanchard E, Luo M, Bhattacharjee MB, Freeborn J, Park S, Couturier J, et al. Lactobacillus reuteri reduces the severity of experimental autoimmune encephalomyelitis in mice by modulating gut microbiota. Front Immunol. 2019;10:385. doi:10.3389/fimmu.2019.00385.
- Consonni A, Cordiglieri C, Rinaldi E, Marolda R, Ravanelli I, Guidesi E, Elli M, Mantegazza R, Baggi F. Administration of bifidobacterium and lactobacillus strains modulates experimental myasthenia gravis and experimental encephalomyelitis in Lewis rats. Oncotarget. 2018;9(32):22269–22287. doi:10.18632/oncotarget.25170.
- Ezendam J, de Klerk A, Gremmer ER, van Loveren H. Effects of Bifidobacterium animalis administered during lactation on allergic and autoimmune responses in rodents. Clin Exp Immunol. 2008;154(3):424–431. doi:10.1111/j.1365-2249.2008.03788.x.
- Kwon HK, Kim G-C, Kim Y, Hwang W, Jash A, Sahoo A, Kim J-E, Nam JH, Im S-H. Amelioration of experimental autoimmune encephalomyelitis by probiotic mixture is mediated by a shift in T helper cell immune response. Clinical Immunology. 2013;146(3):217–227. doi:10.1016/j.clim.2013.01.001.
- Salehipour Z, Haghmorad D, Sankian M, Rastin M, Nosratabadi R, Soltan Dallal MM, Tabasi N, Khazaee M, Nasiraii LR, Mahmoudi M, et al. Bifidobacterium animalis in combination with human origin of Lactobacillus plantarum ameliorate neuroinflammation in experimental model of multiple sclerosis by altering CD4+ T cell subset balance. Biomed Pharmacother. 2017;95:1535–1548. doi:10.1016/j.biopha.2017.08.117.
- Mangalam A, Shahi SK, Luckey D, Karau M, Marietta E, Luo N, Choung RS, Ju J, Sompallae R, Gibson-Corley K, et al. Human gut-derived commensal bacteria suppress CNS inflammatory and demyelinating disease. Cell Rep. 2017;20(6):1269–1277. doi:10.1016/j.celrep.2017.07.031.
- Carrillo-Salinas FJ, Mestre L, Mecha M, Feliú A, Del Campo R, Villarrubia N, Espejo C, Montalbán X, Álvarez-Cermeño JC, Villar LM, et al. Gut dysbiosis and neuroimmune responses to brain infection with Theiler’s murine encephalomyelitis virus. Sci Rep. 2017;7(1):44377. doi:10.1038/srep44377.
- Omura S, Sato F, Park A-M, Fujita M, Khadka S, Nakamura Y, Katsuki A, Nishio K, Gavins FNE, Tsunoda I, et al. Bioinformatics analysis of gut microbiota and CNS transcriptome in virus-induced acute myelitis and chronic inflammatory demyelination; potential association of Distinct bacteria with CNS IgA upregulation. Front Immunol. 2020;11:1138. doi:10.3389/fimmu.2020.01138.
- Wang X, Chang L, Wan X, Tan Y, Qu Y, Shan J, Yang Y, Ma L, Hashimoto K. (R)-ketamine ameliorates demyelination and facilitates remyelination in cuprizone-treated mice: a role of gut–microbiota–brain axis. Neurobiology Of Disease. 2022;165:105635. doi:10.1016/j.nbd.2022.105635.
- Wang X, Eguchi A, Yang Y, Chang L, Wan X, Shan J, Qu Y, Ma L, Mori C, Yang J, et al. Key role of the gut–microbiota–brain axis via the subdiaphragmatic vagus nerve in demyelination of the cuprizone-treated mouse brain. Neurobiol Dis. 2023;176:105951. doi:10.1016/j.nbd.2022.105951.
- Liu S, Rezende RM, Moreira TG, Tankou SK, Cox LM, Wu M, Song A, Dhang FH, Wei Z, Costamagna G, et al. Oral administration of miR-30d from feces of MS patients suppresses MS-like symptoms in mice by expanding Akkermansia muciniphila. Cell Host & Microbe. 2019;26(6):779–794.e8. doi:10.1016/j.chom.2019.10.008.
- Haghikia A, Jörg S, Duscha A, Berg J, Manzel A, Waschbisch A, Hammer A, Lee D-H, May C, Wilck N, et al. Dietary fatty acids directly impact central nervous system autoimmunity via the small intestine. Immunity. 2015;43(4):817–829. doi:10.1016/j.immuni.2015.09.007.
- Duscha A, Gisevius B, Hirschberg S, Yissachar N, Stangl GI, Dawin E, Bader V, Haase S, Kaisler J, David C, et al. Propionic acid shapes the multiple sclerosis disease course by an immunomodulatory mechanism. Cell. 2020;180(6):1067–1080.e16. doi:10.1016/j.cell.2020.02.035.
- Braniste V, Al-Asmakh M, Kowal C, Anuar F, Abbaspour A, Tóth M, Korecka A, Bakocevic N, Ng LG, Kundu P, et al. The gut microbiota influences blood-brain barrier permeability in mice. Sci Transl Med. 2014;6(263):263ra158. doi:10.1126/scitranslmed.3009759.
- Mossad O, Erny D. The microbiota–microglia axis in central nervous system disorders. Brain Pathology. 2020;30(6):1159–1177. doi:10.1111/bpa.12908.
- Chen J, Chia N, Kalari KR, Yao JZ, Novotna M, Paz Soldan MM, Luckey DH, Marietta EV, Jeraldo PR, Chen X, et al. Multiple sclerosis patients have a distinct gut microbiota compared to healthy controls. Sci Rep. 2016;6(1):28484. doi:10.1038/srep28484.
- Jangi S, Gandhi R, Cox LM, Li N, von Glehn F, Yan R, Patel B, Mazzola MA, Liu S, Glanz BL, et al. Alterations of the human gut microbiome in multiple sclerosis. Nat Commun. 2016;7(1):12015. doi:10.1038/ncomms12015.
- Hughes LE, Bonell S, Natt RS, Wilson C, Tiwana H, Ebringer A, Cunningham P, Chamoun V, Thompson EJ, Croker J, et al. Antibody responses to Acinetobacter spp. And Pseudomonas aeruginosa in multiple sclerosis: prospects for diagnosis using the myelin-acinetobacter-neurofilament antibody index. Clin Diagn Lab Immunol. 2001;8(6):1181–1188. doi:10.1128/CDLI.8.6.1181-1188.2001.
- Farrokhi V, Nemati R, Nichols FC, Yao X, Anstadt E, Fujiwara M, Grady J, Wakefield D, Castro W, Donaldson J, et al. Bacterial lipodipeptide, lipid 654, is a microbiome-associated biomarker for multiple sclerosis. Clin & Trans Imm. 2013;2(11):e8. doi:10.1038/cti.2013.11.
- Mazmanian SK, Liu CH, Tzianabos AO, Kasper DL. An immunomodulatory molecule of symbiotic bacteria directs maturation of the host immune system. Cell. 2005;122(1):107–118. doi:10.1016/j.cell.2005.05.007.
- Tremlett H, Fadrosh DW, Faruqi AA, Hart J, Roalstad S, Graves J, Lynch S, Waubant E, Aaen G, Belman A, et al. Gut microbiota composition and relapse risk in pediatric MS: a pilot study. J Neurol Sci. 2016;363:153–157. doi:10.1016/j.jns.2016.02.042.
- Cady N, Peterson SR, Freedman SN, Mangalam AK. Beyond metabolism: the complex interplay between dietary phytoestrogens, gut bacteria, and cells of nervous and immune systems. Front Neurol. 2020;11:150. doi:10.3389/fneur.2020.00150.
- Freedman SN, Shahi SK, Mangalam AK. The “gut feeling”: breaking down the role of gut microbiome in multiple sclerosis. Neurotherapeutics. 2018;15(1):109–125. doi:10.1007/s13311-017-0588-x.
- Cocchi M, Mondo E, Romeo M, Traina G. The inflammatory conspiracy in multiple sclerosis: a crossroads of clues and insights through mast cells, platelets, inflammation, gut microbiota, mood disorders and stem cells. Int J Mol Sci. 2022;23(6):3253. doi:10.3390/ijms23063253.
- Garabatos N, Santamaria P. Gut microbial antigenic mimicry in autoimmunity. Front Immunol. 2022;13:873607. doi:10.3389/fimmu.2022.873607.
- Elsayed NS, Aston P, Bayanagari VR, Shukla SK. The gut microbiome molecular mimicry piece in the multiple sclerosis puzzle. Front Immunol. 2022;13:972160. doi:10.3389/fimmu.2022.972160.
- Brown AJ, Goldsworthy SM, Barnes AA, Eilert MM, Tcheang L, Daniels D, Muir AI, Wigglesworth MJ, Kinghorn I, Fraser NJ, et al. The Orphan G protein-coupled receptors GPR41 and GPR43 are activated by propionate and other short chain carboxylic acids. J Biol Chem. 2003;278(13):11312–11319. doi:10.1074/jbc.M211609200.
- Liu J, Li H, Gong T, Chen W, Mao S, Kong Y, Yu J, Sun J. Anti-neuroinflammatory effect of short-chain fatty acid acetate against Alzheimer’s disease via upregulating GPR41 and inhibiting ERK/JNK/NF-κB. J Agric Food Chem. 2020;68(27):7152–7161. doi:10.1021/acs.jafc.0c02807.
- Chevalier AC, Rosenberger TA. Increasing acetyl-CoA metabolism attenuates injury and alters spinal cord lipid content in mice subjected to experimental autoimmune encephalomyelitis. J Neurochem. 2017;141(5):721–737. doi:10.1111/jnc.14032.
- Smith PM, Howitt MR, Panikov N, Michaud M, Gallini CA, Bohlooly-Y M, Glickman JN, Garrett WS. The microbial metabolites, short-chain fatty acids, regulate colonic T reg cell homeostasis. Sci. 2013;341(6145):569–573. doi:10.1126/science.1241165.
- Hoyles L, Snelling T, Umlai U-K, Nicholson JK, Carding SR, Glen RC, McArthur S. Microbiome–host systems interactions: protective effects of propionate upon the blood–brain barrier. Microbiome. 2018;6(1). doi:10.1186/s40168-018-0439-y.
- Furusawa Y, Obata Y, Fukuda S, Endo TA, Nakato G, Takahashi D, Nakanishi Y, Uetake C, Kato K, Kato T, et al. Commensal microbe-derived butyrate induces the differentiation of colonic regulatory T cells. Nature. 2013;504(7480):446–450. doi:10.1038/nature12721.
- Kespohl M, Vachharajani N, Luu M, Harb H, Pautz S, Wolff S, Sillner N, Walker A, Schmitt-Kopplin P, Boettger T, et al. The microbial metabolite butyrate induces expression of Th1-associated factors in CD4(+) T cells. Front Immunol. 2017;8:1036. doi:10.3389/fimmu.2017.01036.
- Chang PV, Hao L, Offermanns S, Medzhitov R. The microbial metabolite butyrate regulates intestinal macrophage function via histone deacetylase inhibition. Proc Natl Acad Sci U S A. 2014;111(6):2247–2252. doi:10.1073/pnas.1322269111.
- Chen T, Noto D, Hoshino Y, Mizuno M, Miyake S. Butyrate suppresses demyelination and enhances remyelination. J Neuroinflammation. 2019;16(1):165. doi:10.1186/s12974-019-1552-y.
- Arpaia N, Campbell C, Fan X, Dikiy S, van der Veeken J, deRoos P, Liu H, Cross JR, Pfeffer K, Coffer PJ, et al. Metabolites produced by commensal bacteria promote peripheral regulatory T-cell generation. Nature. 2013;504(7480):451–455. doi:10.1038/nature12726.
- Luu M, Pautz S, Kohl V, Singh R, Romero R, Lucas S, Hofmann J, Raifer H, Vachharajani N, Carrascosa LC, et al. The short-chain fatty acid pentanoate suppresses autoimmunity by modulating the metabolic-epigenetic crosstalk in lymphocytes. Nat Commun. 2019;10(1):760. doi:10.1038/s41467-019-08711-2.
- Park J, Wang Q, Wu Q, Mao-Draayer Y, Kim CH. Bidirectional regulatory potentials of short-chain fatty acids and their G-protein-coupled receptors in autoimmune neuroinflammation. Sci Rep. 2019;9(1):8837. doi:10.1038/s41598-019-45311-y.
- Erny D, Hrabě de Angelis AL, Jaitin D, Wieghofer P, Staszewski O, David E, Keren-Shaul H, Mahlakoiv T, Jakobshagen K, Buch T, et al. Host microbiota constantly control maturation and function of microglia in the CNS. Nat Neurosci. 2015;18(7):965–977. doi:10.1038/nn.4030.
- Wenzel TJ, Gates EJ, Ranger AL, Klegeris A. Short-chain fatty acids (SCFAs) alone or in combination regulate select immune functions of microglia-like cells. Mol Cell Neurosci. 2020;105:103493. doi:10.1016/j.mcn.2020.103493.
- Sundaram TS, Giromini C, Rebucci R, Baldi A. Omega-3 Polyunsaturated fatty acids counteract inflammatory and oxidative damage of non-transformed porcine enterocytes. Anim (Basel). 2020;10(6):956. doi:10.3390/ani10060956.
- Denys A, Hichami A, Khan NA. N-3 PUFAs modulate T-cell activation via protein kinase C-α and -ε and the NF-κB signaling pathway. J Lipid Res. 2005;46(4):752–758. doi:10.1194/jlr.M400444-JLR200.
- Djuricic I, Calder PC. Beneficial outcomes of omega-6 and omega-3 Polyunsaturated fatty acids on human health: an update for 2021. Nutrients. 2021;13(7):2421. doi:10.3390/nu13072421.
- Fu Y, Wang Y, Gao H, Li D, Jiang R, Ge L, Tong C, Xu K. Associations among dietary omega-3 Polyunsaturated fatty acids, the gut microbiota, and intestinal immunity. Mediators Of Inflammation. 2021;2021:1–11. doi:10.1155/2021/8879227.
- Montgomery TL, Eckstrom K, Lile KH, Caldwell S, Heney ER, Lahue KG, D’Alessandro A, Wargo MJ, Krementsov DN. Lactobacillus reuteri tryptophan metabolism promotes host susceptibility to CNS autoimmunity. Microbiome. 2022;10(1):198. doi:10.1186/s40168-022-01408-7.
- Rothhammer V, Borucki DM, Garcia Sanchez MI, Mazzola MA, Hemond CC, Regev K, Paul A, Kivisäkk P, Bakshi R, Izquierdo G, et al. Dynamic regulation of serum aryl hydrocarbon receptor agonists in MS. Neurol Neuroimmunol Neuroinflamm. 2017;4(4):e359. doi:10.1212/NXI.0000000000000359.
- Sonner JK, Keil M, Falk-Paulsen M, Mishra N, Rehman A, Kramer M, Deumelandt K, Röwe J, Sanghvi K, Wolf L, et al. Dietary tryptophan links encephalogenicity of autoreactive T cells with gut microbial ecology. Nat Commun. 2019;10(1):4877. doi:10.1038/s41467-019-12776-4.
- Wilck N, Matus MG, Kearney SM, Olesen SW, Forslund K, Bartolomaeus H, Haase S, Mähler A, Balogh A, Markó L, et al. Salt-responsive gut commensal modulates T(H)17 axis and disease. Nature. 2017;551(7682):585–589. doi:10.1038/nature24628.
- Zelante T, Iannitti R, Cunha C, De Luca A, Giovannini G, Pieraccini G, Zecchi R, D’Angelo C, Massi-Benedetti C, Fallarino F, et al. Tryptophan catabolites from microbiota engage aryl hydrocarbon receptor and balance mucosal reactivity via interleukin-22. Immunity. 2013;39(2):372–385. doi:10.1016/j.immuni.2013.08.003.
- Venkatesh M, Mukherjee S, Wang H, Li H, Sun K, Benechet A, Qiu Z, Maher L, Redinbo M, Phillips R, et al. Symbiotic bacterial metabolites regulate gastrointestinal barrier function via the xenobiotic sensor PXR and toll-like receptor 4. Immunity. 2014;41(2):296–310. doi:10.1016/j.immuni.2014.06.014.
- Rothhammer V, Mascanfroni ID, Bunse L, Takenaka MC, Kenison JE, Mayo L, Chao C-C, Patel B, Yan R, Blain M, et al. Type I interferons and microbial metabolites of tryptophan modulate astrocyte activity and central nervous system inflammation via the aryl hydrocarbon receptor. Nat Med. 2016;22(6):586–597. doi:10.1038/nm.4106.
- Rothhammer V, Borucki DM, Tjon EC, Takenaka MC, Chao C-C, Ardura-Fabregat A, de Lima KA, Gutiérrez-Vázquez C, Hewson P, Staszewski O, et al. Microglial control of astrocytes in response to microbial metabolites. Nature. 2018;557(7707):724–728. doi:10.1038/s41586-018-0119-x.
- Owe-Young R, Webster NL, Mukhtar M, Pomerantz RJ, Smythe G, Walker D, Armati PJ, Crowe SM, Brew BJ. Kynurenine pathway metabolism in human blood–brain–barrier cells: implications for immune tolerance & neurotoxicity. J Neurochem. 2008;105(4):1346–1357. doi:10.1111/j.1471-4159.2008.05241.x.
- Lim CK, Bilgin A, Lovejoy DB, Tan V, Bustamante S, Taylor BV, Bessede A, Brew BJ, Guillemin GJ. Kynurenine pathway metabolomics predicts and provides mechanistic insight into multiple sclerosis progression. Sci Rep-Uk. 2017;7(1). doi:10.1038/srep41473.
- Guillemin GJ, Smythe G, Takikawa O, Brew BJ. Expression of indoleamine 2,3-dioxygenase and production of quinolinic acid by human microglia, astrocytes, and neurons. Glia. 2005;49(1):15–23. doi:10.1002/glia.20090.
- Rothhammer V, Kenison JE, Li Z, Tjon E, Takenaka MC, Chao C-C, Alves de Lima K, Borucki DM, Kaye J, Quintana FJ, et al. Aryl hydrocarbon receptor activation in astrocytes by laquinimod ameliorates autoimmune inflammation in the CNS. Neurol Neuroimmunol Neuroinflamm. 2021;8(2):e946. doi:10.1212/NXI.0000000000000946.
- Haase S, Haghikia A, Wilck N, Muller DN, Linker RA. Impacts of microbiome metabolites on immune regulation and autoimmunity. Immunology. 2018;154(2):230–238. doi:10.1111/imm.12933.
- Calvo-Barreiro L, Eixarch H, Ponce-Alonso M, Castillo M, Lebrón-Galán R, Mestre L, Guaza C, Clemente D, Del Campo R, Montalban X, et al. A commercial probiotic induces tolerogenic and reduces pathogenic responses in experimental autoimmune encephalomyelitis. Cells. 2020;9(4):906. doi:10.3390/cells9040906.
- San Hernandez AM, Singh C, Valero DJ, Nisar J, Trujillo Ramirez JI, Kothari KK, Isola S, Gordon DK. Multiple sclerosis and serotonin: potential therapeutic applications. Cureus. 2020;12:e11293. doi:10.7759/cureus.11293.
- Wagner A, Wang C, Fessler J, DeTomaso D, Avila-Pacheco J, Kaminski J, Zaghouani S, Christian E, Thakore P, Schellhaass B, et al. Metabolic modeling of single Th17 cells reveals regulators of autoimmunity. Cell. 2021;184(16):4168–4185.e21. doi:10.1016/j.cell.2021.05.045.
- Carriche GM, Almeida L, Stüve P, Velasquez L, Dhillon-LaBrooy A, Roy U, Lindenberg M, Strowig T, Plaza-Sirvent C, Schmitz I, et al. Regulating T-cell differentiation through the polyamine spermidine. J Allergy Clin Immun. 2021;147(1):335–348.e11. doi:10.1016/j.jaci.2020.04.037.
- Yang Q, Zheng C, Cao J, Cao G, Shou P, Lin L, Velletri T, Jiang M, Chen Q, Han Y, et al. Spermidine alleviates experimental autoimmune encephalomyelitis through inducing inhibitory macrophages. Cell Death Differ. 2016;23(11):1850–1861. doi:10.1038/cdd.2016.71.
- Guo X, Harada C, Namekata K, Kimura A, Mitamura Y, Yoshida H, Matsumoto Y, Harada T. Spermidine alleviates severity of murine experimental autoimmune encephalomyelitis. Invest Ophthalmol Vis Sci. 2011;52(5):2696–2703. doi:10.1167/iovs.10-6015.
- Soda K, Kano Y, Nakamura T, Kasono K, Kawakami M, Konishi F. Spermine, a natural polyamine, suppresses LFA-1 expression on human lymphocyte. The Journal Of Immunology. 2005;175(1):237–245. doi:10.4049/jimmunol.175.1.237.
- Zhang S, Al-Maghout T, Cao H, Pelzl L, Salker MS, Veldhoen M, Cheng A, Lang F, Singh Y. Gut bacterial metabolite Urolithin a (UA) mitigates Ca(2+) entry in T cells by regulating miR-10a-5p. Front Immunol. 2019;10:1737. doi:10.3389/fimmu.2019.01737.
- Shen PX, Li X, Deng S-Y, Zhao L, Zhang Y-Y, Deng X, Han B, Yu J, Li Y, Wang Z-Z, et al. Urolithin a ameliorates experimental autoimmune encephalomyelitis by targeting aryl hydrocarbon receptor. EBioMedicine. 2021;64:103227. doi:10.1016/j.ebiom.2021.103227.
- Singh R, Chandrashekharappa S, Bodduluri SR, Baby BV, Hegde B, Kotla NG, Hiwale AA, Saiyed T, Patel P, Vijay-Kumar M, et al. Enhancement of the gut barrier integrity by a microbial metabolite through the Nrf2 pathway. Nat Commun. 2019;10(1). doi:10.1038/s41467-018-07859-7.
- Gong Z, Huang J, Xu B, Ou Z, Zhang L, Lin X, Ye X, Kong X, Long D, Sun X, et al. Urolithin a attenuates memory impairment and neuroinflammation in APP/PS1 mice. J Neuroinflammation. 2019;16(1):62. doi:10.1186/s12974-019-1450-3.
- DaSilva NA, Nahar PP, Ma H, Eid A, Wei Z, Meschwitz S, Zawia NH, Slitt AL, Seeram NP. Pomegranate ellagitannin-gut microbial-derived metabolites, urolithins, inhibit neuroinflammation in vitro. Nutritional Neuroscience. 2019;22(3):185–195. doi:10.1080/1028415X.2017.1360558.
- Busto R, Serna J, Perianes-Cachero A, Quintana-Portillo R, García-Seisdedos D, Canfrán-Duque A, Paino CL, Lerma M, Casado ME, Martín-Hidalgo A, et al. Ellagic acid protects from myelin-associated sphingolipid loss in experimental autoimmune encephalomyelitis. Biochim Biophys Acta Mol Cell Biol Lipids. 2018;1863(9):958–967. doi:10.1016/j.bbalip.2018.05.009.
- Chen P, Chen FC, Lei JX, Wang GH, Zhou BH. The gut microbiota metabolite Urolithin B improves cognitive deficits by Inhibiting Cyt C-Mediated apoptosis and promoting the survival of neurons through the PI3K pathway in aging mice. Front Pharmacol. 2021;12:768097. doi:10.3389/fphar.2021.768097.
- Castillo-Alvarez F, Perez-Matute P, Oteo JA, Marzo-Sola ME. The influence of interferon β-1b on gut microbiota composition in patients with multiple sclerosis. Neurología (English Ed). 2021;36(7):495–503. doi:10.1016/j.nrleng.2020.05.006.
- Katz Sand I, Zhu Y, Ntranos A, Clemente JC, Cekanaviciute E, Brandstadter R, Crabtree-Hartman E, Singh S, Bencosme Y, Debelius J, et al. Disease-modifying therapies alter gut microbial composition in MS. Neurol Neuroimmunol Neuroinflamm. 2019;6(1):e517. doi:10.1212/NXI.0000000000000517.
- Abdurasulova IN, Tarasova EA, Nikiforova IG, Il’ves AG, Ivashkova EV, Matsulevich AV, Tatarinov AE, Shangina LV, Ermolenko EI, Klimenko VM, et al. The intestinal microbiota composition in patients with multiple sclerosis receiving different disease-modifying therapies DMT. Zh Nevrol Psikhiatr Im S S Korsakova. 2018;118(8):62–69. doi:10.17116/jnevro201811808262.
- Tremlett H, Fadrosh DW, Faruqi AA, Zhu F, Hart J, Roalstad S, Graves J, Lynch S, Waubant E. Gut microbiota in early pediatric multiple sclerosis: a case−control study. Euro J Of Neurology. 2016;23(8):1308–1321. doi:10.1111/ene.13026.
- Tremlett H, Fadrosh DW, Faruqi AA, Hart J, Roalstad S, Graves J, Spencer CM, Lynch SV, Zamvil SS, Waubant E, et al. Associations between the gut microbiota and host immune markers in pediatric multiple sclerosis and controls. BMC Neurol. 2016;16(1):182. doi:10.1186/s12883-016-0703-3.
- Shahi SK, Freedman SN, Murra AC, Zarei K, Sompallae R, Gibson-Corley KN, Karandikar NJ, Murray JA, Mangalam AK. Prevotella histicola, a human gut commensal, is as potent as COPAXONE® in an animal model of multiple sclerosis. Front Immunol. 2019;10:462. doi:10.3389/fimmu.2019.00462.
- Rumah KR, Vartanian TK, Fischetti VA. Oral multiple sclerosis drugs inhibit the in vitro growth of epsilon toxin producing gut bacterium, Clostridium perfringens. Front Cell Infect Microbiol. 2017;7:11. doi:10.3389/fcimb.2017.00011.
- Li QR, Wang CY, Tang C, He Q, Li N, Li JS. Reciprocal interaction between intestinal microbiota and mucosal lymphocyte in cynomolgus monkeys after alemtuzumab treatment. Am J Transplant. 2013;13(4):899–910. doi:10.1111/ajt.12148.
- Diebold M, Meola M, Purushothaman S, Siewert LK, Pössnecker E, Roloff T, Lindberg RL, Kuhle J, Kappos L, Derfuss T, et al. Gut microbiota composition as a candidate risk factor for dimethyl fumarate-induced lymphopenia in multiple sclerosis. Gut Microbes. 2022;14(1):2147055. doi:10.1080/19490976.2022.2147055.
- Dehghani F, Abdollahi S, Shidfar F, Clark CCT, Soltani S. Probiotics supplementation and brain-derived neurotrophic factor (BDNF): a systematic review and meta-analysis of randomized controlled trials. Nutr Neurosci. 2022;1–11. doi:10.1080/1028415X.2022.2110664.
- Valizadeh S, Majdi Seghinsara A, Maleki Chollou K, Bahadori A, Abbaszadeh S, Taghdir M, Behniafar H, Riahi SM. The efficacy of probiotics in experimental autoimmune encephalomyelitis (an animal model for MS): a systematic review and meta-analysis. Lett Appl Microbiol. 2021;73(4):408–417. doi:10.1111/lam.13543.
- Kap YS, Bus-Spoor C, van Driel N, Dubbelaar ML, Grit C, Kooistra SM, Fagrouch ZC, Verschoor EJ, Bauer J, Eggen BJL, et al. Targeted diet modification reduces multiple sclerosis–like disease in adult marmoset monkeys from an outbred colony. J Immunol. 2018;201(11):3229–3243. doi:10.4049/jimmunol.1800822.
- Mestre L, Carrillo-Salinas FJ, Feliú A, Mecha M, Alonso G, Espejo C, Calvo-Barreiro L, Luque-García JL, Estevez H, Villar LM, et al. How oral probiotics affect the severity of an experimental model of progressive multiple sclerosis? Bringing commensal bacteria into the neurodegenerative process. Gut Microbes. 2020;12(1):1813532. doi:10.1080/19490976.2020.1813532.
- Delgado S, Sanchez B, Margolles A, Ruas-Madiedo P, Ruiz L. Molecules produced by probiotics and intestinal microorganisms with immunomodulatory activity. Nutrients. 2020;12(2):391. doi:10.3390/nu12020391.
- Moravejolahkami AR, Paknahad Z, Chitsaz A. Dietary intake of energy and fiber in MS patients; an approach to prebiotics role. Nutr Food Sci. 2019;49(6):1039–1050. doi:10.1108/NFS-01-2019-0001.
- Mirashrafi S, Hejazi Taghanaki SZ, Sarlak F, Moravejolahkami AR, Hojjati Kermani MA, Haratian M. Effect of probiotics supplementation on disease progression, depression, general health, and anthropometric measurements in relapsing-remitting multiple sclerosis patients: a systematic review and meta-analysis of clinical trials. Int J Clin Pract. 2021;75(11):e14724. doi:10.1111/ijcp.14724.
- Jiang J, Chu C, Wu C, Wang C, Zhang C, Li T, Zhai Q, Yu L, Tian F, Chen W, et al. Efficacy of probiotics in multiple sclerosis: a systematic review of preclinical trials and meta-analysis of randomized controlled trials. Food Funct. 2021;12(6):2354–2377. doi:10.1039/D0FO03203D.
- Kouchaki E, Tamtaji OR, Salami M, Bahmani F, Daneshvar Kakhaki R, Akbari E, Tajabadi-Ebrahimi M, Jafari P, Asemi Z. Clinical and metabolic response to probiotic supplementation in patients with multiple sclerosis: a randomized, double-blind, placebo-controlled trial. Clin Nutr. 2017;36(5):1245–1249. doi:10.1016/j.clnu.2016.08.015.
- Tankou SK, Regev K, Healy BC, Tjon E, Laghi L, Cox LM, Kivisäkk P, Pierre IV, Hrishikesh L, Gandhi R, et al. A probiotic modulates the microbiome and immunity in multiple sclerosis. Ann Neurol. 2018;83(6):1147–1161. doi:10.1002/ana.25244.
- Zeraati M, Enayati M, Kafami L, Shahidi SH, Salari AA. Gut microbiota depletion from early adolescence alters adult immunological and neurobehavioral responses in a mouse model of multiple sclerosis. Neuropharmacology. 2019;157:107685. doi:10.1016/j.neuropharm.2019.107685.
- Bianchimano P, Britton GJ, Wallach DS, Smith EM, Cox LM, Liu S, Iwanowski K, Weiner HL, Faith JJ, Clemente JC, et al. Mining the microbiota to identify gut commensals modulating neuroinflammation in a mouse model of multiple sclerosis. Microbiome. 2022;10(1):174. doi:10.1186/s40168-022-01364-2.
- Chen H, Ma X, Liu Y, Ma L, Chen Z, Lin X, Si L, Ma X, Chen X. Gut microbiota interventions with Clostridium butyricum and norfloxacin modulate immune response in experimental autoimmune encephalomyelitis mice. Front Immunol. 2019;10:1662. doi:10.3389/fimmu.2019.01662.
- Yokote H, Miyake S, Croxford JL, Oki S, Mizusawa H, Yamamura T. NKT cell-dependent amelioration of a mouse model of multiple sclerosis by altering gut flora. The American Journal Of Pathology. 2008;173(6):1714–1723. doi:10.2353/ajpath.2008.080622.
- Miyauchi E, Kim S-W, Suda W, Kawasumi M, Onawa S, Taguchi-Atarashi N, Morita H, Taylor TD, Hattori M, Ohno H, et al. Gut microorganisms act together to exacerbate inflammation in spinal cords. Nature. 2020;585(7823):102–106. doi:10.1038/s41586-020-2634-9.
- McMurran CE, Guzman de la Fuente A, Penalva R, Ben Menachem-Zidon O, Dombrowski Y, Falconer J, Gonzalez GA, Zhao C, Krause FN, Young AMH, et al. The microbiota regulates murine inflammatory responses to toxin-induced CNS demyelination but has minimal impact on remyelination. Proc Natl Acad Sci U S A. 2019;116(50):25311–25321. doi:10.1073/pnas.1905787116.
- Suleiman L, Waubant E, Aaen G, Belman A, Benson L, Candee M, Chitnis T, Gorman M, Goyal M, Greenberg B, et al. Early infectious exposures are not associated with increased risk of pediatric-onset multiple sclerosis. Mult Scler Relat Disord. 2018;22:103–107. doi:10.1016/j.msard.2018.03.015.
- Baldin E, Zenesini C, Antonazzo IC, Bartolomei I, Caniatti L, Costa M, Curti E, Ferraro D, Foschi M, Granella F, et al. Antibiotic use and risk of multiple sclerosis: a nested case-control study in Emilia-Romagna region, Italy. Neuroepidemiology. 2021;55(3):224–231. doi:10.1159/000515682.
- Metz LM, Li D, Traboulsee A, Myles ML, Duquette P, Godin J, Constantin M, Yong VW. Glatiramer acetate in combination with minocycline in patients with relapsing—remitting multiple sclerosis: results of a Canadian, multicenter, double-blind, placebo-controlled trial. Mult Scler. 2009;15(10):1183–1194. doi:10.1177/1352458509106779.
- Sorensen PS, Sellebjerg F, Lycke J, Färkkilä M, Créange A, Lund CG, Schluep M, Frederiksen JL, Stenager E, Pfleger C, et al. Minocycline added to subcutaneous interferon β-1a in multiple sclerosis: randomized RECYCLINE study. Eur J Neurol. 2016;23(5):861–870. doi:10.1111/ene.12953.
- Parks NE, Jackson-Tarlton CS, Vacchi L, Merdad R, Johnston BC. Dietary interventions for multiple sclerosis-related outcomes. Cochrane Database Syst Rev. 2020;2020(5):CD004192. doi:10.1002/14651858.CD004192.pub4.
- Sanchez JMS, DePaula-Silva AB, Libbey JE, Fujinami RS. Role of diet in regulating the gut microbiota and multiple sclerosis. Clin Immunol. 2022;235:108379. doi:10.1016/j.clim.2020.108379.
- Valburg C, Sonti A, Stern JN, Najjar S, Harel A. Dietary factors in experimental autoimmune encephalomyelitis and multiple sclerosis: a comprehensive review. Mult Scler. 2021;27(4):494–502. doi:10.1177/1352458520923955.
- Bhargava P, Cassard S, Steele SU, Azevedo C, Pelletier D, Sugar EA, Waubant E, Mowry EM. The vitamin D to ameliorate multiple sclerosis (VIDAMS) trial: study design for a multicenter, randomized, double-blind controlled trial of vitamin D in multiple sclerosis. Contemporary Clinical Trials. 2014;39(2):288–293. doi:10.1016/j.cct.2014.10.004.
- Rosjo E, Steffensen LH, Jørgensen L, Lindstrøm JC, Šaltytė Benth J, Michelsen AE, Aukrust P, Ueland T, Kampman MT, Torkildsen Ø, et al. Vitamin D supplementation and systemic inflammation in relapsing-remitting multiple sclerosis. J Neurol. 2015;262(12):2713–2721. doi:10.1007/s00415-015-7902-5.
- Fitzgerald KC, Munger KL, Köchert K, Arnason BGW, Comi G, Cook S, Goodin DS, Filippi M, Hartung H-P, Jeffery DR, et al. Association of vitamin D levels with multiple sclerosis activity and progression in patients receiving interferon beta-1b. JAMA Neurol. 2015;72(12):1458–1465. doi:10.1001/jamaneurol.2015.2742.
- Shieh A, Lee, SM, Lagishetty V, Gottleib C, Jacobs, JP, Adams, JS. Pilot trial of vitamin D3 and calcifediol in healthy vitamin D deficient adults: does it change the fecal microbiome? J Clin Endocrinol Metab. 2021;106(12):3464–3476. doi:10.1210/clinem/dgab573.
- Naderpoor N, Mousa A, Fernanda Gomez Arango L, Barrett HL, Dekker Nitert M, de Courten B. Effect of vitamin D supplementation on faecal microbiota: a randomised clinical trial. Nutrients. 2019;11(12):2888. doi:10.3390/nu11122888.
- Enam F, Mansell TJ. Prebiotics: tools to manipulate the gut microbiome and metabolome. J Ind Microbiol Biotechnol. 2019;46(9–10):1445–1459. doi:10.1007/s10295-019-02203-4.
- Berer K, Martínez I, Walker A, Kunkel B, Schmitt-Kopplin P, Walter J, Krishnamoorthy G. Dietary non-fermentable fiber prevents autoimmune neurological disease by changing gut metabolic and immune status. Sci Rep. 2018;8(1):10431. doi:10.1038/s41598-018-28839-3.
- Moravejolahkami AR, Paknahad Z, Chitsaz A. Dietary intake of energy and fiber in MS patients; an approach to prebiotics role. Nutr Food Sci. 2019;49(6):1039–1050. doi:10.1108/NFS-01-2019-0001.
- Calvo-Barreiro L, Eixarch H, Cornejo T, Costa C, Castillo M, Mestre L, Guaza C, Martínez-Cuesta MDC, Tanoue T, Honda K, et al. Selected Clostridia strains from the human microbiota and their metabolite, butyrate, improve experimental autoimmune encephalomyelitis. Neurotherapeutics. 2021;18(2):920–937. doi:10.1007/s13311-021-01016-7.
- Di Biase A, Salvati S, Di Benedetto R, Attorri L, Martinelli A, Malchiodi F. Eicosapentaenoic acid pre-treatment reduces biochemical changes induced in total brain and myelin of weanling wistar rats by cuprizone feeding. Prostaglandins, Leukotrienes And Essential Fatty Acids. 2014;90(4):99–104. doi:10.1016/j.plefa.2013.11.004.
- Torkildsen Ø, Brunborg LA, Thorsen F, Mørk SJ, Stangel M, Myhr K-M, Bø L. Effects of dietary intervention on MRI activity, de- and remyelination in the cuprizone model for demyelination. Exp Neurol. 2009;215(1):160–166. doi:10.1016/j.expneurol.2008.09.026.
- Fleck AK, Hucke S, Teipel F, Eschborn M, Janoschka C, Liebmann M, Wami H, Korn L, Pickert G, Hartwig M, et al. Dietary conjugated linoleic acid links reduced intestinal inflammation to amelioration of CNS autoimmunity. Brain. 2021;144(4):1152–1166. doi:10.1093/brain/awab040.
- Dong TA, Sandesara PB, Dhindsa DS, Mehta A, Arneson LC, Dollar AL, Taub PR, Sperling LS. Intermittent fasting: a heart healthy dietary pattern? The American Journal Of Medicine. 2020;133(8):901–907. doi:10.1016/j.amjmed.2020.03.030.
- Catenacci VA, Pan Z, Ostendorf D, Brannon S, Gozansky WS, Mattson MP, Martin B, MacLean PS, Melanson EL, Troy Donahoo W, et al. A randomized pilot study comparing zero-calorie alternate-day fasting to daily caloric restriction in adults with obesity. Obesity (Silver Spring). 2016;24(9):1874–1883. doi:10.1002/oby.21581.
- Duenas M, Muñoz-González I, Cueva C, Jiménez-Girón A, Sánchez-Patán F, Santos-Buelga C, Moreno-Arribas MV, Bartolomé B. A survey of modulation of gut microbiota by dietary polyphenols. BioMed Research International. 2015;2015:1–15. doi:10.1155/2015/850902.
- Ginwala R, McTish E, Raman C, Singh N, Nagarkatti M, Nagarkatti P, Sagar D, Jain P, Khan ZK. Apigenin, a natural flavonoid, attenuates EAE severity through the modulation of dendritic cell and other immune cell functions. J Neuroimmune Pharmacol. 2016;11(1):36–47. doi:10.1007/s11481-015-9617-x.
- Aktas O, Prozorovski T, Smorodchenko A, Savaskan NE, Lauster R, Kloetzel P-M, Infante-Duarte C, Brocke S, Zipp F. Green Tea Epigallocatechin-3-Gallate Mediates T Cellular NF-κB inhibition and exerts Neuroprotection in autoimmune encephalomyelitis. J Immunol. 2004;173(9):5794–5800. doi:10.4049/jimmunol.173.9.5794.
- Haghmorad D, Mahmoudi, MB, Salehipour Z, Jalayer Z, Rastin M, Kokhaei P, Mahmoudi M. Hesperidin ameliorates immunological outcome and reduces neuroinflammation in the mouse model of multiple sclerosis. J Neuroimmunol. 2017;302:23–33. doi:10.1016/j.jneuroim.2016.11.009.
- Verbeek R, van Tol EA, van Noort JM. Oral flavonoids delay recovery from experimental autoimmune encephalomyelitis in SJL mice. Biochem Pharmacol. 2005;70(2):220–228. doi:10.1016/j.bcp.2005.04.041.
- Sato F, Martinez NE, Shahid M, Rose JW, Carlson NG, Tsunoda I. Resveratrol exacerbates both autoimmune and viral models of multiple sclerosis. The American Journal Of Pathology. 2013;183(5):1390–1396. doi:10.1016/j.ajpath.2013.07.006.
- Ghaiad HR, Nooh MM, El-Sawalhi MM, Shaheen AA. Resveratrol promotes remyelination in cuprizone model of multiple sclerosis: biochemical and histological study. Mol Neurobiol. 2017;54(5):3219–3229. doi:10.1007/s12035-016-9891-5.
- Dolati S, Babaloo Z, Ayromlou H, Ahmadi M, Rikhtegar R, Rostamzadeh D, Roshangar L, Nouri M, Mehdizadeh A, Younesi V, et al. Nanocurcumin improves regulatory T-cell frequency and function in patients with multiple sclerosis. J Neuroimmunol. 2019;327:15–21. doi:10.1016/j.jneuroim.2019.01.007.
- Wang C, Huang Z, Yu K, Ding R, Ye K, Dai C, Xu X, Zhou G, Li C. High-salt diet has a certain impact on protein Digestion and gut microbiota: a sequencing and proteome combined study. Front Microbiol. 2017;8:1838. doi:10.3389/fmicb.2017.01838.
- Ferguson JF, Aden LA, Barbaro NR, Van Beusecum JP, Xiao L, Simons AJ, Warden C, Pasic L, Himmel LE, Washington MK, et al. High dietary salt–induced DC activation underlies microbial dysbiosis-associated hypertension. JCI Insight. 2019;5(13). doi:10.1172/jci.insight.126241.
- Hernandez AL, Kitz A, Wu C, Lowther DE, Rodriguez DM, Vudattu N, Deng S, Herold KC, Kuchroo VK, Kleinewietfeld M, et al. Sodium chloride inhibits the suppressive function of FOXP3+ regulatory T cells. J Clin Invest. 2015;125(11):4212–4222. doi:10.1172/JCI81151.
- Haase S, Wilck N, Haghikia A, Gold R, Mueller DN, Linker RA. The role of the gut microbiota and microbial metabolites in neuroinflammation. Eur J Immunol. 2020;50(12):1863–1870. doi:10.1002/eji.201847807.
- Farez MF, Fiol MP, Gaitan MI, Quintana FJ, Correale J. Sodium intake is associated with increased disease activity in multiple sclerosis. J Neurol Neurosurg Psychiatry. 2015;86(1):26–31. doi:10.1136/jnnp-2014-307928.
- Fitzgerald KC, Munger KL, Hartung H-P, Freedman MS, Montalbán X, Edan G, Wicklein E-M, Radue E-W, Kappos L, Pohl C, et al. Sodium intake and multiple sclerosis activity and progression in BENEFIT. Ann Neurol. 2017;82(1):20–29. doi:10.1002/ana.24965.
- McDonald J, Graves J, Waldman A, Lotze T, Schreiner T, Belman A, Greenberg B, Weinstock-Guttman B, Aaen G, Tillema J-M, et al. A case-control study of dietary salt intake in pediatric-onset multiple sclerosis. Mult Scler Relat Disord. 2016;6:87–92. doi:10.1016/j.msard.2016.02.011.
- Nourbakhsh B, Graves J, Casper TC, Lulu S, Waldman A, Belman A, Greenberg B, Weinstock-Guttman B, Aaen G, Tillema J-M, et al. Dietary salt intake and time to relapse in paediatric multiple sclerosis. J Neurol Neurosurg Psychiatry. 2016;87(12):1350–1353. doi:10.1136/jnnp-2016-313410.
- Phillips MCL. Fasting as a Therapy in neurological disease. Nutrients. 2019;11(10):2501. doi:10.3390/nu11102501.
- Storoni M, Plant GT. The therapeutic potential of the ketogenic diet in treating progressive multiple sclerosis. Mult Scler Int. 2015;2015:681289. doi:10.1155/2015/681289.
- Choi IY, Piccio L, Childress P, Bollman B, Ghosh A, Brandhorst S, Suarez J, Michalsen A, Cross A, Morgan T, et al. A diet mimicking fasting promotes regeneration and reduces autoimmunity and multiple sclerosis symptoms. Cell Rep. 2016;15(10):2136–2146. doi:10.1016/j.celrep.2016.05.009.
- Razeghi Jahromi S, Ghaemi A, Alizadeh A, Sabetghadam F, Moradi Tabriz H, Togha M. Effects of intermittent fasting on experimental Autoimune encephalomyelitis in C57BL/6 mice. Iran J Allergy Asthma Immunol. 2016;15:212–219.
- Bai M, Wang Y, Han R, Xu L, Huang M, Zhao J, Lin Y, Song S, Chen Y. Intermittent caloric restriction with a modified fasting-mimicking diet ameliorates autoimmunity and promotes recovery in a mouse model of multiple sclerosis. J Nutr Biochem. 2021;87:108493. doi:10.1016/j.jnutbio.2020.108493.
- Cignarella F, Cantoni C, Ghezzi L, Salter A, Dorsett Y, Chen L, Phillips D, Weinstock GM, Fontana L, Cross AH, et al. Intermittent fasting confers protection in CNS autoimmunity by altering the gut microbiota. Cell Metab. 2018;27(6):1222–1235.e6. doi:10.1016/j.cmet.2018.05.006.
- Bahr LS, Bock M, Liebscher D, Bellmann-Strobl J, Franz L, Prüß A, Schumann D, Piper SK, Kessler CS, Steckhan N, et al. Ketogenic diet and fasting diet as nutritional approaches in multiple sclerosis (NAMS): protocol of a randomized controlled study. Trials. 2020;21(1). doi:10.1186/s13063-019-3928-9.
- Cantoni C, Lin Q, Dorsett Y, Ghezzi L, Liu Z, Pan Y, Chen K, Han Y, Li Z, Xiao H, et al. Alterations of host-gut microbiome interactions in multiple sclerosis. EBioMedicine. 2022;76:103798. doi:10.1016/j.ebiom.2021.103798.
- Saresella M, Mendozzi L, Rossi V, Mazzali F, Piancone F, LaRosa F, Marventano I, Caputo D, Felis GE, Clerici M, et al. Immunological and clinical effect of diet modulation of the gut microbiome in multiple sclerosis patients: a pilot study. Front Immunol. 2017;8:1391. doi:10.3389/fimmu.2017.01391.
- Wahls TL, Titcomb TJ, Bisht B, Eyck PT, Rubenstein LM, Carr LJ, Darling WG, Hoth KF, Kamholz J, Snetselaar LG, et al. Impact of the Swank and Wahls elimination dietary interventions on fatigue and quality of life in relapsing-remitting multiple sclerosis: the WAVES randomized parallel-arm clinical trial. Mult Scler J Exp Transl Clin. 2021;7(3):20552173211035399. doi:10.1177/20552173211035399.
- Irish AK, Erickson CM, Wahls TL, Snetselaar LG, Darling WG. Randomized control trial evaluation of a modified Paleolithic dietary intervention in the treatment of relapsing-remitting multiple sclerosis: a pilot study. Degener Neurol Neuromuscul Dis. 2017;7:1–18. doi:10.2147/DNND.S116949.
- Yadav V, Marracci G, Kim E, Spain R, Cameron M, Overs S, Riddehough A, Li DKB, McDougall J, Lovera J, et al. Low-fat, plant-based diet in multiple sclerosis: a randomized controlled trial. Mult Scler Relat Disord. 2016;9:80–90. doi:10.1016/j.msard.2016.07.001.
- Halkjaer SI, Boolsen AW, Gunther S, Christensen AH, Petersen AM. Can fecal microbiota transplantation cure irritable bowel syndrome? World J Gastroenterol. 2017;23(22):4112–4120. doi:10.3748/wjg.v23.i22.4112.
- Paramsothy S, Kamm MA, Kaakoush NO, Walsh AJ, van den Bogaerde J, Samuel D, Leong RWL, Connor S, Ng W, Paramsothy R, et al. Multidonor intensive faecal microbiota transplantation for active ulcerative colitis: a randomised placebo-controlled trial. Lancet. 2017;389(10075):1218–1228. doi:10.1016/S0140-6736(17)30182-4.
- Xue LJ, Yang X-Z, Tong Q, Shen P, Ma S-J, Wu S-N, Zheng J-L, Wang H-G. Fecal microbiota transplantation therapy for Parkinson’s disease: a preliminary study. Med. 2020;99(35):e22035. doi:10.1097/MD.0000000000022035.
- Mao D, Jiang Q, Sun Y, Mao Y, Guo L, Zhang Y, Man M, Ouyang G, Sheng L. Treatment of intestinal graft-versus-host disease with unrelated donor fecal microbiota transplantation capsules: a case report. Med. 2020;99(38):e22129. doi:10.1097/MD.0000000000022129.
- Li K, Wei S, Hu L, Yin X, Mai Y, Jiang C, Peng X, Cao X, Huang Z, Zhou H, et al. Protection of fecal microbiota transplantation in a mouse model of multiple sclerosis. Mediators Of Inflammation. 2020;2020:1–13. doi:10.1155/2020/2058272.
- Makkawi S, Camara-Lemarroy C, Metz L. Fecal microbiota transplantation associated with 10 years of stability in a patient with SPMS. Neurol Neuroimmunol Neuroinflamm. 2018;5(4):e459. doi:10.1212/NXI.0000000000000459.
- Engen PA, Zaferiou A, Rasmussen H, Naqib A, Green SJ, Fogg LF, Forsyth CB, Raeisi S, Hamaker B, Keshavarzian A, et al. Single-arm, non-randomized, time series, single-subject study of fecal microbiota transplantation in multiple sclerosis. Front Neurol. 2020;11:978. doi:10.3389/fneur.2020.00978.
- Al KF, Craven LJ, Gibbons S, Parvathy SN, Wing AC, Graf C, Parham KA, Kerfoot SM, Wilcox H, Burton JP, et al. Fecal microbiota transplantation is safe and tolerable in patients with multiple sclerosis: a pilot randomized controlled trial. Mult Scler J Exp Transl Clin. 2022;8(2):20552173221086662. doi:10.1177/20552173221086662.
- Tanasescu R, Tench CR, Constantinescu CS, Telford G, Singh S, Frakich N, Onion D, Auer DP, Gran B, Evangelou N, et al. Hookworm treatment for relapsing multiple sclerosis: a randomized Double-blinded placebo-controlled trial. JAMA Neurol. 2020;77(9):1089–1098. doi:10.1001/jamaneurol.2020.1118.
- Correale J, Farez MF. The impact of parasite infections on the course of multiple sclerosis. J Neuroimmunol. 2011;233(1–2):6–11. doi:10.1016/j.jneuroim.2011.01.002.
- Jenkins TP, Pritchard DI, Tanasescu R, Telford G, Papaiakovou M, Scotti R, Cortés A, Constantinescu CS, Cantacessi C. Experimental infection with the hookworm, necator americanus, is associated with stable gut microbial diversity in human volunteers with relapsing multiple sclerosis. BMC Biol. 2021;19(1):74. doi:10.1186/s12915-021-01003-6.
- Barone M, Mendozzi L, D’Amico F, Saresella M, Rampelli S, Piancone F, La Rosa F, Marventano I, Clerici M, d’Arma A, et al. Influence of a high-impact multidimensional rehabilitation program on the gut microbiota of patients with multiple sclerosis. Int J Mol Sci. 2021;22(13):7173. doi:10.3390/ijms22137173.