ABSTRACT
Antibodies that block PD-L1/PD-1 immune checkpoints restore the activity of functionally-impaired antitumor T cells. These antibodies show unprecedented clinical benefit in various advanced cancers, particularly in melanoma. However, only a subset of cancer patients responds to current PD-L1/PD-1-blocking strategies, highlighting the need for further advancements in PD-L1/PD-1-based immunotherapy. Here, we report on a novel approach designed to combine PD-L1 checkpoint inhibition with the tumor-selective induction of apoptosis by TNF-related Apoptosis Inducing Ligand (TRAIL). In brief, a new bi-functional fusion protein, designated anti-PD-L1:TRAIL, was constructed comprising a PD-L1-blocking antibody fragment genetically fused to the extracellular domain of the pro-apoptotic tumoricidal protein TRAIL. Treatment of PD-L1-expressing cancer cells with anti-PD-L1:TRAIL induced PD-L1-directed TRAIL-mediated cancer cell death. Treatment of T cells with anti-PD-L1:TRAIL augmented T cell activation, as evidenced by increased proliferation, secretion of IFNγ and enhanced killing of cancer cell lines and primary patient-derived cancer cells in mixed T cell/cancer cell culture experiments. Of note, elevated levels of IFNγ further upregulated PD-L1 on cancer cells and simultaneously sensitized cancer cells to TRAIL-mediated apoptosis by anti-PD-L1:TRAIL. Additionally, anti-PD-L1:TRAIL converted immunosuppressive PD-L1-expressing myeloid cells into pro-apoptotic effector cells that triggered TRAIL-mediated cancer cell death. In conclusion, combining PD-L1 checkpoint inhibition with TRAIL-mediated induction of apoptosis using anti-PD-L1:TRAIL yields promising multi-fold and mutually reinforcing anticancer activity that may be exploited to enhance the efficacy of therapeutic PD-L1/PD-1 checkpoint inhibition.
Introduction
Programmed Death Ligand 1 (PD-L1) and its cognate receptor PD-1 represent an immune checkpoint of great interest for cancer immunotherapy. Antibodies that block PD-L1/PD-1 interaction restore the anticancer activity of functionally impaired tumor-infiltrating lymphocytes (TILs), specifically cytotoxic T cells. Treatment with these antibodies has transformed the landscape of cancer immunotherapy, yielding long-term remission and cure in a subset of advanced stage melanoma patients.Citation1,2
The PD-L1/PD-1 immune checkpoint normally ensures timely shut-down of immune responses to prevent collateral damage or autoimmunity (reviewed inCitation3). In brief, PD-L1 expression is upregulated on antigen-presenting cells during inflammation by locally produced IFNγ and is expressed by myeloid suppressor cells.Citation4-6 Simultaneously, expression of PD-1 increases on activated T cells,Citation7 which upon interaction with PD-L1 dampens the cytolytic activity of T cells.Citation4 Interestingly, activated T cells not only express PD-1, but upon activation also upregulate PD-L1.Citation8,9 Antibody-mediated cross-linking of PD-L1 on T cells triggers co-stimulatory signaling and ultimately leads to induction of apoptosis,Citation10 indicative of an immunoregulatory role for PD-L1 on T cells.
Various cancer types upregulate PD-L1 expression either constitutively via oncogenic signaling pathways or in response to IFNγ produced in the tumor environment.Citation11,12 Consequently, antitumor T cells are inhibited via PD-L1/PD-1 interaction, which allows cancer cells to evade the immune system even in highly immunogenic malignancies such as melanoma.Citation12,13 Hence, the expression of PD-L1 on cancer cells often correlates with unfavorable prognosis.Citation14,15 Although PD-1 and PD-L1-blocking antibodies have triggered breakthrough curative anticancer immunity, most notably in advanced melanoma,Citation1,2 the benefit of these antibodies is still restricted to a minority of cancer patients.
To expand the clinical effects of immune checkpoint therapy, various combinatorial approaches have been attempted in order to identify opportunities for synergistic activity. For instance, treatment with PD-1-blocking antibody nivolumab was combined with CTLA-4 antibody ipilimumab, which significantly enhanced response rates in melanoma patients.Citation16 Similarly, combination of PD-1/PD-L1 blockade with induction of cytotoxic cancer cell death by radiotherapy proved more effective and enhanced activation of anticancer immunity.Citation17,18 These selected examples highlight that more efficacious PD-L1/PD-1-targeted therapy can be achieved by rational design of combinatorial therapeutic approaches.
In this respect, we and others have previously reported on a class of bi-functional fusion proteins that comprise an scFv antibody fragment genetically fused to the tumoricidal protein tumor necrosis factor (TNF)-related apoptosis inducing ligand (TRAIL) (reviewed inCitation19). TRAIL is a homotrimeric death-inducing ligand of the TNF superfamily with well-documented tumor-selective pro-apoptotic activity that has been proven safe in clinical trials (reviewed inCitation20). Antibody fragment-mediated delivery of soluble TRAIL optimizes its target cell-selective accretion and, moreover, triggers enhanced TRAIL-receptor-mediated apoptosis in targeted cancer cells.Citation21,22 Importantly, use of an scFv antibody fragment with antagonistic activity equips the scFv:TRAIL fusion protein with additional tumoricidal activity, e.g., by inhibition of EGFR-mitogenic signalingCitation23 or by blocking tumor-expressed CD47 and thereby augmenting neutrophil-mediated phagocytosis of cancer cells.Citation24 Furthermore, scFv-mediated display of TRAIL on the surface of T cells or granulocytes augments the cytolytic activity of these immune effector cells.Citation25,26
Based on this bi-functional TRAIL-based fusion protein format, we constructed and pre-clinically evaluated an anti-PD-L1:TRAIL fusion protein comprised of a PD-L1-blocking antibody fragment genetically fused to human soluble TRAIL. This anti-PD-L1:TRAIL fusion protein was designed to combine PD-L1 checkpoint inhibition with simultaneous TRAIL-mediated activation of cancer cell death.
Materials & methods
Antibodies, reagents, inhibitors
The following antibodies were used in this study; anti-CD279-PE (PD-1, clone MIH4, eBioscience), anti-CD274-APC (PD-L1, clone 29E.2A3, BioLegend), anti-CD83-PE (clone HB15e, eBioscience), anti-CD206-PE (clone 19.2, eBioscience), anti-CD3-PerCP-Cyanine5.5 (clone OKT-3, eBioscience), anti-TRAIL/TNFSF10-PE (clone 75402, R&D systems), NG2-FITC (anti-MCSP, clone LHM2, Santa Cruz Biotechnology), polyclonal Goat-anti-Human-PE (SouthernBiotech), Goat-anti-Mouse IgG (H+L) Secondary Antibody (Alexa Fluor 488 conjugate, Thermo Scientific) and anti-CD4-APC (clone MEM-241), anti-CD8-FITC (clone HIT8a), anti-CD56-PE (clone B-A19), anti-CD14-FITC (clone MEM-15), anti-CD11b-FITC (clone MEM-174), anti-HLA-DR-PE (clone MEM-12), anti-CD86-FITC (clone BU63), Mouse IgG2b-APC, Annexin-V-FITC (all Immunotools). Recombinant human IFNγ, GM-CSF, M-CSF, IL-4, IL-10, TGF-β1, anti-CD3 (clone UCHT-1), and anti-IFNγ (clone B27) were purchased from ImmunoTools. LPS (Lipopolysaccharides from E. coli 0111:B4) was purchased from Sigma-Aldrich. Recombinant human PD-1:Fc was purchased from R&D systems. Pan-caspase inhibitor z-VAD-fmk, TRAILR1 (clone DJR1), and TRAILR2 (clone DJR2-4) antibodies were purchased from Enzo Life Sciences. TRAIL-neutralizing mAb 2E5 was purchased from Life Technologies. Recombinant CMV protein pp65 was purchased from Miltenyi Biotec. A PD-L1 neutralizing murine antibody was purchased from BPS Bioscience.
Cell lines
DLD-1, HCT-116, SK-MEL-28, A2058 and CHO-K1, NCI-H1975, ES-2, MDA-MB-231 were obtained from the American Type Culture Collection (ATCC). TRAIL-resistant cell line HCT-116.cFLIPs was kindly provided by Prof. dr. Harald Wajant (University of Würzburg, Würzburg, Germany). All cell lines were cultured in RPMI-1640 or DMEM (Lonza) supplemented with 10% fetal calf serum (FCS, Thermo Scientific). DLD-1.PD-L1 cells were generated by transfection of parental DLD-1 cells with eukaryotic expression plasmid pCMV6-PD-L1 using Fugene-HD (Promega). Stable transfectants were generated using Hygromycin B selection (Life technologies). All cells were cultured at 37°C, in a humidified 5% CO2 atmosphere. Cell numbers were quantified using a cell counter (Sysmex). For experiments, tumor cells were cultured in 48-wells plates at a density of 2 × 104 cells/well. For upregulation of PD-L1, cells were pre-treated for 24 h with 20 ng/mL IFNγ. PD-L1 expression was analyzed with an Accuri C6 flow cytometer (BD Biosciences) using PD-L1-APC antibody or appropriate isotype control. Relative PD-L1 expression levels are listed in Table S1. TRAIL receptor expression was determined by flow cytometry using TRAILR1 and TRAILR2 antibodies with secondary Goat-anti-Mouse-488 conjugate staining. Relative TRAIL receptor expression levels are listed in Table S2.
Primary patient-derived melanoma cells and tumor-infiltrating lymphocytes
Fresh melanoma and appendix carcinoma tissue was collected during surgical resection after informed consent (local approval nr. METc2012/330). Tissue was minced and cultured in RPMI 1640 with 10% FCS. Adherent cell phenotype was analyzed by flow cytometry using fluorescently labeled CD14, PD-L1, and MCSP antibodies. Primary patient-derived melanoma cells used in this study were CD14 negative and MCSP positive and were used before passage 4. For generation of TILs, minced tissue fragments were cultured in RPMI 1640 with 10% FCS supplemented with 50 IU/mL IL-2 (Proleukin, Novartis). TIL phenotype was analyzed by flow cytometry for CD3, CD4, CD8, and CD56.
Production of TRAIL fusion proteins
Anti-PD-L1:TRAIL was constructed by insertion of an anti-PD-L1 mAb 3G10-derived scFv into Sfi1 and Not1 restriction sites into the previously described plasmid pEE14-scFv:TRAIL.Citation27 Briefly, CHO-K1 cells were transfected with eukaryotic expression plasmid pEE14scFv:sTRAIL using the Fugene-HD reagent (Promega) and stable transfectants were generated by the glutamine synthetase selection method. Stable transfectants were cultured at 37°C in serum-free CHO-S SFM II suspension medium (Gibco, Life Technologies) for up to 7 d after which supernatant was harvested (1,500 g, 10 min) and stored at −20°C until further use. Fusion protein concentration in culture supernatant was determined by TRAIL ELISA (Abcam). Anti-EpCAM:TRAIL and anti-MCSP:TRAIL were described before.Citation22,27
PD-L1-specific binding of anti-PD-L1:TRAIL
Tumor cells were incubated with anti-PD-L1:TRAIL (1 µg/mL) for 1 h at 4˚C, washed twice with PBS (1,000 g, 5 min), stained with anti-TRAIL-PE for 30 min at 4˚C, washed twice with PBS, and analyzed for binding by flow cytometry. Where indicated tumor cells were pre-incubated with excess (10 µg/mL) PD-L1 blocking mAb.
PD-1/PD-L1 blocking by anti-PD-L1:TRAIL
DLD-1 and DLD-1.PD-L1 cells were pre-incubated with indicated concentrations of anti-PD-L1:TRAIL for 1 h at 0°C, after which cells were washed twice (1000 g, 5 min) and incubated with 4 μg/mL PD-1:Fc for 1 h at 0°C. Subsequently, cells were washed twice (1,000 g, 5 min) and stained with Goat-anti-Human-PE for 30 min at 0˚C and washed twice (1000 g, 5 min). PD-1:Fc binding was evaluated by flow cytometry.
Apoptosis assay
Tumor cells were treated with anti-PD-L1:TRAIL or anti-EpCAM:TRAIL and, where indicated, in the presence of PD-L1 blocking mAb (10 µg/mL), pan-caspase inhibitor z-VAD-FMK (10 µM) or TRAIL-neutralizing mAb 2E5 (1 µg/mL). After 18 h, apoptosis was assessed by flow cytometry using Annexin-V staining according to manufacturer's protocol (Immunotools). Where indicated, cells were co-treated with 1 μg/mL cycloheximide (CHX, Sigma-Aldrich).
Spheroid assay
DLD-1, DLD-1.PD-L1, or NCI-H1975 cells (1–5 × 103 cells/well) were seeded in low adherence 96-well plates (Costar) in DMEM supplemented with MITO+ Serum Extender (Corning). Cells were treated with anti-PD-L1:TRAIL, anti-EpCAM:TRAIL, or anti-MCSP:TRAIL as indicated. After 72 h, cell viability was determined using MTS (CellTiter 96 AQueous One Solution Cell Proliferation, Promega) at 490 nM using a Victor V3 multi-label plate counter (Perkin Elmer). Absorbance of the maximum death control (treatment with 70% ethanol for 15 min) was subtracted from all values, after which cell viability was calculated as percentage of medium control. Light microscopy images were acquired at 10× magnification using the EVOS XL core cell imaging system (Life Technologies) and colony number was counted manually in three separate fields-of-view per condition in triplicates. Pre-formed spheroids of DLD-1 and DLD-1.PD-L1 cells were generated by 96 h culture in low adherence flasks (Costar), after which spheroids were transferred to low adherence plates for experiments.
PBMC stimulation assays
Peripheral blood mononuclear cells (PBMCs) were obtained from venous blood of healthy volunteers after informed written consent using standard density gradient centrifugation (Lymphoprep). PBMCs (1.25 × 105/well) were cultured in a 48-well plate in the presence of 0.5 μg/mL agonistic CD3 mAb (UCHT-1) and indicated concentrations anti-PD-L1:TRAIL, anti-EpCAM:TRAIL, or PD-L1 mAb. After 72 h, total cell number was quantified using an automated cell counter (Sysmex) and culture supernatants were stored at −20°C. IFNγ levels in culture supernatant were determined by IFNγ ELISA (eBioscience). Where indicated, freshly isolated PBMCs were labeled with carboxyfluorescein succinimidyl ester (CFSE) (CellTrace CFSE Cell Proliferation Kit, Invitrogen), and after 72 h of respective treatment the cell proliferation was analyzed by flow cytometry within the live PBMCs (defined by FSC/SSC gating).
For CMV specific responses, freshly isolated PBMCs from CMV negative and positive donors were cultured in 96-well plates (1.5 × 105/well) in the presence of CMV pp65 according to manufacturer's instructions (Miltenyi Biotec). After 96 h, culture supernatants were stored at −20°C and secreted IFNγ was determined by IFNγ ELISA.
Antitumor reactivity assay
Tumor cells were labeled with DiD (Vybrant Cell-Labeling Solution, LifeTechnologies). Subsequently, 2 × 104 tumor cells were co-cultured with freshly isolated PBMCs or CD3+ T cells (98% purity, using the human Pan T Cell Isolation Kit (Miltenyi Biotec)) in the presence of 0.5 μg/mL agonistic CD3 mAb (UCHT-1) or CD3/CD28 beads (Dynabeads Human T-Activator CD3/CD28, Thermo Fischer) at a bead-to-cell ratio of 1:10, respectively. Mixed cultures were further treated with 0.5 μg/mL anti-PD-L1:TRAIL, anti-EpCAM:TRAIL or PD-L1 mAb. After 48 h, loss of mitochondrial membrane potential (δψ) in DiD labeled tumor cells was analyzed by DiOC6 staining (Eugene) as previously described.Citation27 After harvesting, PBMCs were stained with fluorescent CD4 and CD8 antibodies whereupon the number of CD4+ and CD8+ T cells within the PBMC gate was analyzed by flow cytometry.
Alternatively, 5 × 104 DLD-1 cells were pre-seeded 24 h before addition of freshly isolated PBMCs. Tumor cells were mixed with PBMCs at indicated E:T ratio's and co-treated with 50 ng/mL BIS-1 (anti-EpCAM:anti-CD3 bispecific antibody previously described inCitation28 and 0.5 μg/mL anti-PD-L1:TRAIL or anti-MCSP:TRAIL. After 24 h, non-adherent cells were carefully washed away and cell viability was determined using MTS as described above.
Patient-derived melanoma and appendix carcinoma cells were co-cultured with autologous TILs in E:T ratio of 2:1. After 48 h, apoptosis was assessed by Annexin-V staining and IFNγ levels in culture supernatant were determined by ELISA.
Isolation and generation of myeloid-derived cell types
Monocytes: PBMCs were subjected to magnetic-activated cell sorting (MACS) with anti-CD14-beads and MS columns (Miltenyi Biotec). Macrophages: monocytes (1 × 106/mL) were treated with 50 ng/mL M-CSF for 6 d, yielding M0 macrophages. M0 macrophages were subsequently stimulated with 50 ng/mL LPS and 20 ng/mL IFNγ to generate M1 macrophages or 20 ng/mL IL-4, IL-10, and TGF-β1 to generate M2 macrophages. Macrophage phenotype was confirmed by flow cytometric analysis of CD14, CD206, and PD-L1, as described before.Citation29 Dendritic cells: immature DCs (iDCs) were generated by treatment of monocytes (3 × 106/mL) with 500 U/mL GM-CSF and 1000 U/mL IL-4 for 7 d. Mature DCs (mDCs) were generated by treatment of iDCs with 1 μg/mL LPS for 3 d. DC phenotype was confirmed by flow cytometric analysis of PD-L1, CD83, CD14, HLA-DR, and CD86.
For mixed culture experiments, myeloid cell types were mixed with 2.5 × 103 DLD-1 cells (at E:T ratio 4:1) with the indicated concentrations of anti-PD-L1:TRAIL in presence or absence of PD-L1 mAb. After 18 h, DLD-1 cells were analyzed for apoptosis by flow cytometric analysis of Annexin-V staining. DLD-1 cells were separately analyzed by excluding CD14+ (monocytes and macrophages) or CD11b+ (DCs) cells.
Statistical analysis
Statistical analysis was performed by two-way ANOVA followed by Tukey–Kramer post-test, one-way ANOVA followed by Tukey–Kramer post-test, Wilcoxon matched pairs test or two-sided unpaired Student t test as indicated using Prism software. p < 0.05 was defined as a statistically significant difference. Where indicated *p < 0.05; **p < 0.01; ***p < 0.001.
Results
anti-PD-L1:TRAIL induces PD-L1-restricted TRAIL-mediated apoptosis in cancer cells
The anti-PD-L1:TRAIL fusion protein was designed to bind to PD-L1 on cancer cells and subsequently trigger TRAIL-mediated apoptosis by activating agonistic TRAIL-receptors. In line with this, anti-PD-L1:TRAIL strongly and dose-dependently bound to carcinoma cell line DLD-1.PD-L1 that ectopically overexpress PD-L1 (). In contrast, anti-PD-L1:TRAIL did not bind to parental DLD-1 cells ( and Fig. S1A). Binding of anti-PD-L1:TRAIL to DLD-1.PD-L1 was abrogated by co-incubation with molar excess of epitope-competing anti-PD-L1 monoclonal antibody (). Treatment of DLD-1.PD-L1 with anti-PD-L1:TRAIL triggered dose-dependent TRAIL-mediated apoptosis, whereas similar treatment of DLD-1 cells did not trigger apoptosis (). Thus, anti-PD-L1:TRAIL triggers cell death specifically after binding to cell surface-expressed PD-L1. Of note, treatment of DLD1.PD-L1 with PD-L1-blocking antibody alone and anti-EpCAM:TRAIL alone induced ∼20% and 45% apoptosis, respectively (). Combined treatment with PD-L1-blocking antibody and anti-EpCAM:TRAIL additively enhanced apoptosis to ∼65% (). However, treatment with anti-PD-L1:TRAIL at the same concentration induced up to 90% apoptosis (). The apoptotic activity of anti-PD-L1:TRAIL was abrogated when cells were treated in the presence of excess epitope-competing PD-L1 monoclonal antibody (). Further, apoptotic activity was TRAIL-mediated since TRAIL-neutralizing monoclonal antibody or total caspase-inhibitor z-VAD-fmk-blocked apoptosis induction in DLD-1.PD-L1 (). Of note, DLD-1 and DLD.PD-L1 are equally sensitive to TRAIL-mediated apoptosis since control EpCAM-targeted anti-EpCAM:TRAIL, that binds equally well to both cell lines (data not shown), induced apoptosis to a similar extent in both cell lines (). In contrast, a control non-targeted fusion protein, anti-MCSP:TRAIL, only minimally induces apoptosis in either cell line (). In a small panel of cancer cell lines that naturally express PD-L1 (Table S1), treatment with anti-PD-L1:TRAIL also induced apoptosis (). Anti-PD-L1:TRAIL treatment further abrogated spheroid formation in DLD-1.PD-L1, but not DLD-1 cells (), and strongly reduced viability of established spheroids (). In spheroid forming assays, anti-PD-L1:TRAIL also significantly reduced cell viability of NCI-H1975 cells that endogenously express PD-L1 (Figs. S1B and S1C). Thus, apoptotic activity of anti-PD-L1:TRAIL is dependent on PD-L1 specific binding to target cells and subsequent induction of TRAIL-mediated apoptosis.
Figure 1. anti-PD-L1:TRAIL induces PD-L1-restricted TRAIL-mediated apoptosis in cancer cells. (A) Binding of anti-PD-L1:TRAIL to DLD-1.PD-L1 cells in the presence or absence of excess PD-L1 blocking antibody (10 µg/mL) was analyzed by flow cytometry. (B) DLD-1.PD-L1 or DLD-1 cells were incubated with an increasing dose of anti-PD-L1:TRAIL and binding was assessed by flow cytometry. (C) DLD-1.PD-L1 or DLD-1 cells were treated with an increasing dose of anti-PD-L1:TRAIL for 18 h, after which apoptosis was measured by flow cytometry using Annexin-V staining. (D) DLD-1.PD-L1 or DLD-1 cells were treated with anti-PD-L1:TRAIL (250 ng/mL), anti-EpCAM:TRAIL (250 ng/mL) or PD-L1 antibody (1 µg/mL). Apoptosis was assessed by Annexin-V staining after 18 h. (E) DLD-1.PD-L1 or DLD-1 cells were treated with anti-PD-L1:TRAIL (250 ng/mL) in the presence or absence of PD-L1 blocking antibody (10 µg/mL), TRAIL-neutralizing mAb (1 µg/mL), or total caspase inhibitor z-VAD-fmk (10 µM). DLD-1.PD-L1 or DLD-1 cells were also treated with anti-MCSP:TRAIL(250 ng/mL). Apoptosis was assessed by Annexin-V staining after 18 h. (F) PD-L1-expressing cell lines were co-treated with cycloheximine (CHX, 1 µg/mL) and anti-PD-L1:TRAIL (1 µg/mL). Apoptosis was determined by Annexin-V staining after 18 h. (G) Representative light microscopy images of spheroid size of DLD-1.PD-L1 cells or DLD-1 cells in medium control versus anti-PD-L1:TRAIL-treated conditions after 72 h. (H) Spheroid formation of DLD-1.PD-L1 or DLD-1 cells was assessed in the presence or absence of 100 ng/mL anti-PD-L1:TRAIL, anti-MCSP:TRAIL, or anti-EpCAM:TRAIL. Number of spheroid colonies was determined after 72 h by counting three fields-of-view per condition in triplicates. (I) Established spheroids of DLD-1.PD-L1 cells or DLD-1 cells were treated with 500 ng/mL anti-PD-L1:TRAIL, anti-MCSP:TRAIL or anti-EpCAM:TRAIL. Cell viability was determined by MTS after 72 h. All graphs represent mean±SD. Statistical analysis was performed using two-way ANOVA (*p < 0.05, **p < 0.01, ***p < 0.001, n.s. not significant).
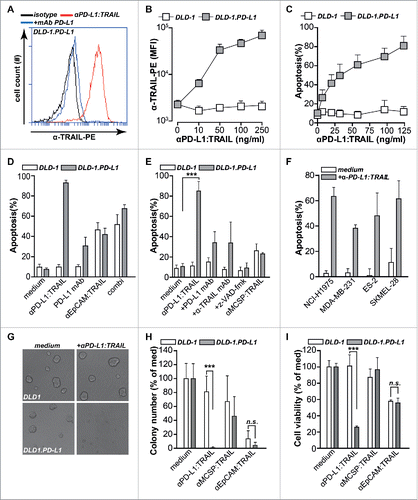
anti-PD-L1:TRAIL blocks PD-1/PD-L1 interaction and enhances T cell activation
Since TRAIL is a naturally occurring homotrimer the anti-PD-L1:TRAIL fusion protein contains three PD-L1-blocking scFvs, which should allow for effective inhibition of PD-L1/PD-1 interaction. Indeed, anti-PD-L1:TRAIL dose-dependently inhibited binding of recombinant PD-1:Fc to DLD-1.PD-L1 cells, whereas similar treatment with anti-EpCAM:TRAIL did not affect PD-1:Fc binding (). In line with the PD-L1/PD-1 blocking effect of anti-PD-L1:TRAIL, treatment of PBMCs with anti-PD-L1:TRAIL and an agonistic CD3 mAb increased proliferation and cell number 2-fold compared to treatment with the agonistic CD3 mAb alone (). Furthermore, anti-PD-L1:TRAIL dose-dependently increased secretion of IFNγ (), to a level similar to that induced by a monoclonal antagonistic anti-PD-L1 antibody (). This T cell stimulatory effect of anti-PD-L1:TRAIL was also detected in mixed cultures of PBMCs with DLD-1 cells (Fig. S1D). Importantly, co-treatment with anti-EpCAM:TRAIL did not increase T cell proliferation or T cell number compared to CD3 activation alone (). To subsequently investigate pro-inflammatory activity of anti-PD-L1:TRAIL in an antigen-specific reaction, a model system using Cytomegalovirus (CMV)-specific T cells was used. To this end, PBMCs from CMVpos and CMVneg donors were loaded with CMV protein pp65 in combination with anti-PD-L1:TRAIL or anti-EpCAM:TRAIL. Loading of PBMCs with pp65 in the presence of anti-PD-L1:TRAIL significantly increased IFNγ secretion by CMVpos donor cells (), whereas no effect on IFNγ secretion was detected in T cells from CMVneg donors. Importantly, anti-EpCAM:TRAIL only minimally induces IFNγ secretion (Fig. S1E). Taken together, anti-PD-L1:TRAIL potentiates T cell proliferation and IFNγ production via blockade of PD-1/PD-L1 interaction.
Figure 2. anti-PD-L1:TRAIL blocks the PD-1/PD-L1 interaction and enhances T cell activation. (A) Binding of PD-1:Fc (4 µg/mL) to DLD-1.PD-L1 cells in the presence of an increasing dose of anti-PD:L1:TRAIL or anti-EpCAM-TRAIL was analyzed by flow cytometry. (B) Representative histograms of CFSE-labeled PBMCs co-treated with agonistic CD3 mAb (0.5 µg/mL) and 500 ng/mL anti-PD-L1:TRAIL or anti-EpCAM:TRAIL. After 72 h, cell proliferation was analyzed by flow cytometry. (C) PBMCs were treated with 500 ng/mL anti-PD-L1:TRAIL or anti-EpCAM:TRAIL in the presence of agonistic CD3 mAb (0.5 μg/mL). After 72 h, cell number was quantified using an automated cell counter. (D) PBMCs were co-treated with agonistic CD3 mAb (0.5 μg/mL) and an increasing dose of anti-PD-L1:TRAIL or anti-EpCAM:TRAIL. After 72 h, IFNγ levels in culture supernatant were determined by ELISA. (E) PBMCs were co-treated with agonistic CD3 mAb (0.5 μg/mL) and 500 ng/mL anti-PDL1:TRAIL, anti-EPCAM:TRAIL or mAb PD-L1 for 72 h. IFNγ levels in culture supernatant were determined by ELISA. (F) PBMCs from CMV-positive or CMV-negative donors and were treated with 500 ng/mL anti-PD-L1:TRAIL in the presence of CMV protein pp65 for 96 h. IFNγ levels in culture supernatant were determined by ELISA. All graphs represent mean±SD. Statistical analysis was performed using unpaired two-sided Student t test (C), two-way ANOVA (D) or Wilcoxon matched pairs test (F) (*p < 0.05, **p < 0.01, ***p < 0.001, n.s. not significant).
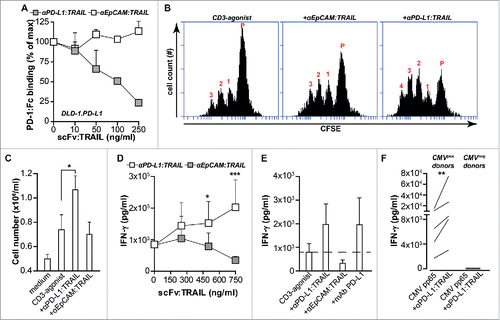
PD-L1:TRAIL enhances anticancer T cell activity
Next, we analyzed whether enhanced T cell activation by anti-PD-L1:TRAIL could augment anticancer T cell activity. In mixed cultures of A2058 melanoma cells and PBMCs, treatment with CD3 agonist at sub-optimal doses triggered apoptosis in ∼30% of cancer cells, whereas treatment with anti-PD-L1:TRAIL alone did not induce apoptosis (). However, combination treatment with CD3 agonist and anti-PD-L1:TRAIL synergistically enhanced apoptosis in A2058 to over 60% (). Further, co-treatment with anti-PD-L1:TRAIL also significantly increases IFNγ secretion (Fig. S1F). Correspondingly, in these mixed culture experiments the expression of PD-L1 on A2058 increased 3-fold (Fig. S1G), whereas PD-1 expression on T cells increased 6-fold (Fig. S1H). Importantly, treatment of activated T cells with PD-L1:TRAIL did not induce apoptosis in T cells (Fig. S1I). Anti-PD-L1:TRAIL also significantly increased production of IFNγ in mixed culture experiments with primary melanoma patient-derived TILs and autologous melanoma cells (), to a level similar to that induced by anti-PD-L1-blocking antibody. PD-L1:TRAIL treatment of autologous primary cancer/TIL mixed culture experiments also increased apoptotic cell death in autologous cancer cells, both in melanoma and appendix carcinoma cells (). The enhanced cytotoxicity of PBMCs upon CD3 agonist and anti-PD-L1:TRAIL treatment was blocked to medium levels by co-incubation with TRAIL-neutralizing antibody (2E5) () and was, therefore, dependent on TRAIL/TRAIL-receptor interaction. Correspondingly, anti-PD-L1:TRAIL induced apoptosis in mixed cultures of HCT-116 and PBMCs, but not in TRAIL resistant HCT-116.cFLIPs (Fig. S1J), demonstrating that cell death upon anti-PD-L1:TRAIL treatment is TRAIL-mediated.
Figure 3. PD-L1:TRAIL enhances anticancer T cell activity. (A) DiD-labeled A2058 cells were co-cultured with PBMCs at E:T ratio 5:1. Where indicated, cells were co-treated with 500 ng/mL anti-PD-L1:TRAIL or anti-EpCAM:TRAIL, in the presence or absence of agonistic CD3 mAb (0.5 μg/mL). After 48 h, apoptosis in DiD-positive cells was determined by flow cytometry using DiOC6 staining (B) Autologous primary patient-derived melanoma cells and TILs were co-cultured at E:T ratio 2:1 and treated with 1 μg/mL anti-PD-L1:TRAIL, anti-EpCAM:TRAIL or 4 μg/mL mAb PD-L1 for 48 h. IFNγ levels in culture supernatant were determined by ELISA. (C) Primary patient-derived melanoma and appendix carcinoma cells were co-cultured with autologous TILs at E:T ratio 2:1 and treated with 1 μg/mL anti-PD-L1:TRAIL for 48 h, after which apoptosis was assessed by Annexin-V staining. (D) DiD-labeled A2058 cells were co-cultured with PBMCs at E:T ratio 5:1 in the presence of agonistic CD3 mAb (0.5 μg/mL). Cells were co-treated with 500 ng/mL anti-PD-L1:TRAIL or anti-EpCAM:TRAIL, where indicated cells were co-treated with TRAIL-neutralizing antibody (1 ug/mL). After 48 h, apoptosis in DiD-positive cells was determined by flow cytometry using DiOC6 staining. (E) In mixed cultures of PBMCs and A2058 as described in D, the PBMC population was stained with fluorescent CD4 and CD8 antibodies whereupon the number of CD4+ and CD8+ T cells within the PBMC gate was analyzed by flow cytometry. (F) DiD-labeled A2058 cells were co-cultured with isolated CD3+ T-cells at E:T ratio 5:1 in the presence of CD3/CD28 beads at a bead-to-cell ratio of 1:10. Cells were co-treated with 500 ng/mL anti-PD-L1:TRAIL or anti-EpCAM:TRAIL and after 48 h, apoptosis in DiD-positive cells was determined by flow cytometry using DiOC6 staining. (G) IFNγ levels in culture supernatant of F were determined by ELISA. (H) DLD-1 cells were pre-seeded 24 h before PBMCs were added at indicated E:T ratio's in the presence of anti-EpCAM:anti-CD3 (50 ng/mL) with or without 500 ng/mL anti-PD-L1:TRAIL or anti-MCSP-TRAIL. Cell viability was determined by MTS after 24 h. (I) IFNγ levels in culture supernatant of H were determined by ELISA. All graphs represent mean ± SD. Statistical analysis was performed using two-way ANOVA (A), one-way ANOVA (D) or unpaired two-sided Student t test (B, F) (*p < 0.05, **p < 0.01, ***p < 0.001, n.s. not significant).
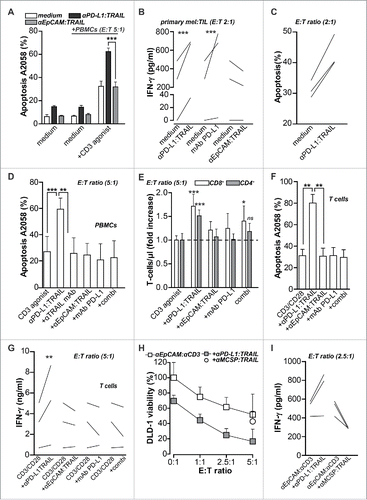
Of note, treatment with anti-EpCAM:TRAIL, PD-L1-blocking antibody or a combination did not significantly enhance the cytotoxic activity of PBMCs toward A2058 cells () nor did it increase the number of T cells (). In these mixed cultures, the number of T-cells did significantly increase upon anti-PD-L1:TRAIL treatment (). Furthermore, in mixed cultures of A2058 and isolated CD3+ T-cells, only anti-PD-L1:TRAIL treatment synergistically enhances apoptosis in A2058 to >80% () and significantly increases IFNγ secretion (). Thus, anti-PD-L1:TRAIL augments cytolytic activity of T cells in mixed culture experiments with T cells and tumor cells.
To mimic antigen-specific T cell activation, DLD-1 cancer cells were treated with T cell retargeting bispecific antibody (bsAb) anti-EpCAM:anti-CD3.Citation28 This bsAb retargets T cells to EpCAM-positive cancer cells and, in mixed cultures of DLD-1 and PBMCs, triggered cell death in an E:T ratio-dependent manner (). Importantly, co-treatment with anti-EpCAM:anti-CD3 and anti-PD-L1:TRAIL significantly increased apoptotic elimination of DLD-1 cells leading to ∼80% loss in cell viability after 24 h of treatment at an E:T ratio of 5:1. (). In contrast, control fusion protein anti-MCSP:TRAIL did not potentiate the cytotoxic effect of anti-EpCAM:anti-CD3 retargeted T cells (). In these mixed cultures, IFNγ production increased upon co-treatment with anti-EpCAM:anti-CD3 and anti-PD-L1:TRAIL when compared to co-treatment with anti-MCSP:TRAIL ().
IFNγ enhances PD-L1 expression and sensitizes cancer cells to TRAIL-mediated apoptosis
IFNγ upregulates PD-L1 expression on cancer cells,Citation11 a finding confirmed here in a panel of seven cancer cell lines and nine primary patient-derived melanoma cell cultures (). Since pro-apoptotic activity of anti-PD-L1:TRAIL is dependent on PD-L1 specific binding to cells, this upregulation of PD-L1 may sensitize cancer cells to anti-PD-L1:TRAIL-mediated killing. Further, IFNγ is known to sensitize cancer cells to TRAIL-mediated apoptosis, e.g., through downregulation of c-FLIP.Citation30,31 Correspondingly, apoptotic activity of control fusion protein anti-EpCAM:TRAIL on DLD-1 cells was increased by IFNγ pre-treatment (). This effect was due to TRAIL sensitization and not due to EpCAM upregulation as IFNγ does not affect EpCAM expression.Citation32 Thus, IFNγ both upregulates PD-L1 and sensitizes cancer cells to TRAIL, which may augment anti-PD-L1:TRAIL activity. In line with the above, pre-treatment of A2058 cells with IFNγ dose-dependently sensitized A2058 cells to anti-PD-L1:TRAIL-mediated apoptosis (), as well as a further panel of five cancer cell lines () and 11 primary melanoma cell cultures (). This apoptotic activity of anti-PD-L1:TRAIL was still abrogated by co-treatment with excess PD-L1 blocking antibody (). Additionally, co-treatment with IFNγ neutralizing antibody inhibited IFNγ-mediated PD-L1 upregulation on DLD-1 cells () and abrogated anti-PD-L1:TRAIL-mediated apoptosis (). Thus, the apoptotic activity of anti-PD-L1:TRAIL is enhanced by IFNγ, likely due to both upregulation of PD-L1 expression on cancer cells and simultaneous sensitization of cancer cells to TRAIL-mediated apoptotic signaling.
Figure 4. IFNγ upregulates PD-L1 expression and sensitizes cancer cells to TRAIL-mediated apoptosis. (A) Primary patient-derived melanoma cells were treated with or without 20 ng/mL IFNγ for 24 h after which PD-L1 expression was analyzed by flow cytometry. (B) Seven cancer cell lines and nine primary patient-derived melanoma cell cultures were treated with or without 20 ng/mL IFNγ for 24 h after which PD-L1 expression was analyzed by flow cytometry. Fold increase was calculated compared to non-treated cells. (C) IFNγ pre-treated or non-treated DLD-1 cells were incubated with an increasing dose of anti-EpCAM:TRAIL for 18 h, after which apoptosis was assessed by flow cytometry using Annexin-V staining. (D) IFNγ pre-treated or non-treated A2058 cells were incubated with an increasing dose of anti-PD-L1:TRAIL. Apoptosis was assessed by Annexin-V staining after 18 h. (E) IFNγ pre-treated or non-treated A2058 cells were incubated with 500 ng/mL anti-PD-L1:TRAIL in the presence or absence of PD-L1 blocking mAb (10 µg/mL). Apoptosis was determined by Annexin-V staining after 18 h. (F) A small panel of cancer cell lines were pre-treated with or without IFNγ (20 ng/mL), followed by treatment of anti-PD-L1:TRAIL (500 ng/mL) for additional 18 h. Apoptosis was determined by Annexin-V staining. (G) IFNγ pre-treated primary patient-derived melanoma cultures were treated with 1 μg/mL anti-PD-L1:TRAIL or anti-EpCAM:TRAIL for 48 h. Apoptosis was determined using Annexin-V. (H) DLD-1 cells were treated with or without 20 ng/mL IFNγ in the presence or absence of 8 µg/mL IFN-y neutralizing mAb. After 24 h, PD-L1 expression was analyzed by flow cytometry. (I) DLD-1 cells were pre-treated with or without 20 ng/mL IFNγ in the presence or absence of 8 µg/mL IFNγ neutralizing mAb. After 24 h, cells were treated with anti-PD-L1:TRAIL (250 ng/mL) in the presence or absence of PD-L1 blocking mAb (10 µg/mL), anti-EpCAM:TRAIL (250 ng/mL) or mAb PD-L1 (1 μg/mL). All graphs represent mean±SD. Statistical analysis was performed using two-way ANOVA (F) or Wilcoxon matched pairs test (G) (*p < 0.05, **p < 0.01, ***p < 0.001, n.s. not significant).
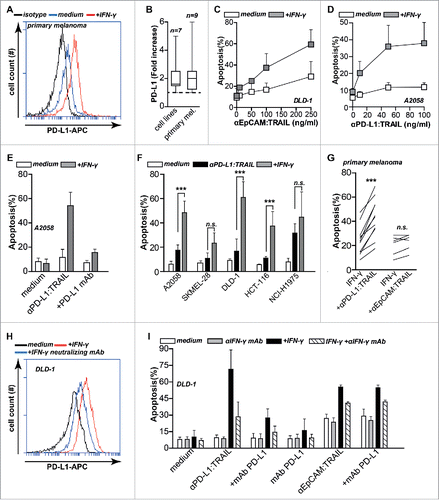
Anti-PD-L1:TRAIL converts PD-L1-expressing myeloid cells into pro-apoptotic tumoricidal effector cells
Within the tumor micro-environment, various types of infiltrated myeloid cells, such as M2 macrophages and DCs, are known to express PD-L1 and to suppress antitumor immunity.Citation33,34 On these cells, PD-L1 expression is further elevated by tumor localized IFNγ.Citation4,5 Previously, we demonstrated that direct arming of myeloid effector cells using a TRAIL fusion protein that binds to CLL-1 on granulocytes, enhanced the pro-apoptotic activity of such myeloid cells.Citation26 To assess whether potentiation of myeloid effector cell activity might also contribute to anti-PD-L1:TRAIL activity, we generated various myeloid effector cell populations, i.e., monocytes, M0, M1, and M2 macrophages, iDCs and mDCs. All of these effector cells expressed PD-L1 to a varying degree, with monocytes having lowest and mDCs having the highest expression (). Further, M1 macrophages had higher PD-L1 expression compared to the immunosuppressive M2 macrophages, a finding in line with an earlier report.Citation29 PD-L1 expression was upregulated by IFNγ pre-treatment, as illustrated for monocytes (). In subsequent mixed culture experiments of monocytes and DLD-1 target cells, such IFNγ pre-treated monocytes alone did not significantly induce apoptosis in DLD1 cells (). However, treatment with anti-PD-L1:TRAIL dose-dependently increased apoptosis in DLD-1 cells (). This increase was blocked by co-treatment with molar excess of PD-L1 blocking mAb (). Similarly, M0, M1, or M2 macrophages and immature or mature DCs alone minimally induced apoptosis of DLD-1 cells in mixed culture experiments (). However, addition of anti-PD-L1:TRAIL to these mixed cultured significantly triggered apoptosis in DLD-1 cells (), with e.g., an >50% increase in apoptosis in DLD-1 cells in mDC mixed culture experiments (). In all mixed culture experiments, PD-L1-blocking mAb strongly inhibited the apoptotic activity of anti-PD-L1:TRAIL (). Of note, background apoptosis in DLD-1 cells was reduced from ∼20% to ∼10% in the presence of M2 macrophages, a finding in line with their pro-tumorigenic role (). Further, although M1 macrophages express higher levels of PD-L1 () and were intrinsically more cytolytic (), the potentiating effect of anti-PD-L1:TRAIL was most pronounced for M2 macrophages, with a 4-fold increase in apoptosis with M2 vs. a 3-fold increase with M1 macrophages (Fig. S1K). Taken together, these data demonstrate that anti-PD-L1:TRAIL binds to PD-L1 on myeloid cells, whereupon these normally immunosuppressive cells trigger TRAIL-mediated apoptotic cell death in cancer cells.
Figure 5. Anti-PD-L1:TRAIL converts PD-L1-expressing myeloid cells into pro-apoptotic tumoricidal effector cells. (A) PD-L1 expression levels of monocytes, M0, M1, M2 macrophages, immature and mature DCs were determined by flow cytometry. Isotype control MFI was subtracted from original MFI. (B) Monocytes were treated with or without 20 ng/mL IFNγ for 24 h after which PD-L1 expression was analyzed by flow cytometry. (C) Monocytes were pre-treated with or without 20 ng/mL IFNγ for 24 h, washed twice with PBS after which DLD-1 cells were added at E:T ratio 4:1 in the presence of an increasing dose of anti-PD-L1:TRAIL. After 18 h, apoptosis was assessed by Annexin-V staining. (D) As in C with 500 ng/mL anti-PD-L1:TRAIL with or without PD-L1 blocking mAb (10 µg/mL). (E) M0, M1 or M2 macrophages were co-cultured with DLD-1 cells at E:T ratio 4:1 in the presence of 500 ng/mL anti-PD-L1:TRAIL with or without PD-L1-blocking mAb (10 µg/mL). After 18 h, apoptosis was assessed by Annexin-V staining. (F) Immature or mature DCs were co-cultured with DLD-1 cells at E:T ratio 4:1 in the presence of 500 ng/mL anti-PD-L1:TRAIL with or without PD-L1-blocking mAb (10 µg/mL). After 18 h, apoptosis was assessed by Annexin-V staining. All graphs represent mean ± SD. Statistical analysis was performed using two-way ANOVA (*p < 0.05, **p < 0.01, ***p < 0.001, n.s. not significant).
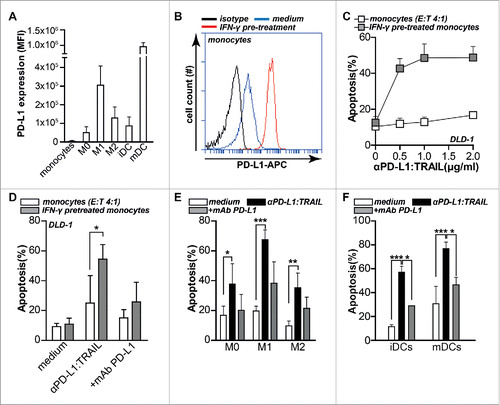
Discussion
Here, we describe a new PD-L1/PD-1 checkpoint inhibitor approach that combines PD-L1 checkpoint blockade with targeted delivery of the pro-apoptotic tumoricidal protein TRAIL. Fusion protein anti-PD-L1:TRAIL has a multi-fold therapeutic effect, depicted in , comprised of (1) induction of TRAIL-mediated cancer cell death after binding to tumor-expressed PD-L1, (2) reactivation of antitumor T-cells by blocking of PD-L1/PD-1 interaction, (3) converting suppressive monocytes/macrophages/DCs into pro-apoptotic effector cells that trigger TRAIL-mediated cancer cell death, and (4) enhancement of IFNγ production by immune effector cells, leading to simultaneous PD-L1 upregulation and sensitization of cancer cells to TRAIL.
Figure 6. Proposed mechanism of action for anti-PD-L1:TRAIL. anti-PD-L1:TRAIL induces TRAIL-mediated cancer cell death after binding to tumor-expressed PD-L1 (1) or after binding to PD-L1 on myeloid effector cells (2), restores proliferation and antitumor activity of T cells by blocking PD-L1/PD-1 interaction (3) and enhances IFNγ production of T cells, leading to simultaneous PD-L1 upregulation and sensitization of cancer cells to TRAIL (4).
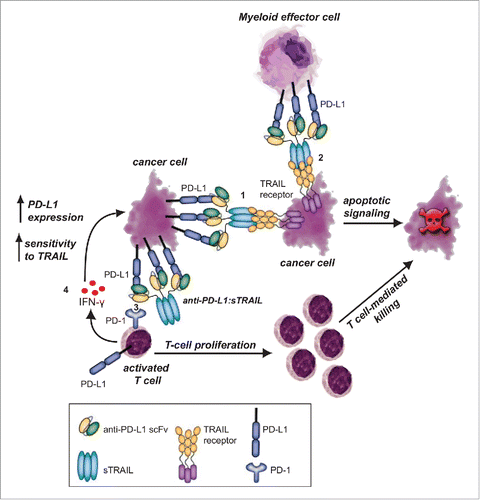
Blockade of the PD-L1/PD-1 axis by anti-PD-L1:TRAIL enhanced T cell activation, proliferation and IFNγ production, an effect similar to that reported for anti-PD-L1 monoclonal antibodies,Citation35 and triggered TRAIL-mediated apoptosis in cancer cells. TRAIL has strong pro-apoptotic activity toward various cancers in the absence of deleterious activity toward normal cells.Citation36 Clinical evaluation of first-generation recombinant human TRAIL (rhTRAIL, brand name “dulanermin”) and TRAIL receptor antibodies typically yielded low to absent toxicity toward normal cells and tissues (reviewed inCitation37), with a maximum tolerable dose (MTD) not being reached for dulanermin.Citation38 Of note, dulanermin did not have toxicity toward immune cells in these studies.Citation37,38 Thus, the use of TRAIL as additional effector domain is anticipated to have no or only minimal side-effects when combined with PD-L1-blockade strategies. In line with this, we did not detect any negative effects of the TRAIL domain of PD-L1:TRAIL on T cells, with no increase in apoptosis in activated T cells. A recent study did report suppression in T cell activation and proliferation when T-cells were co-stimulated with recombinant TRAIL and CD3/CD28 antibodies.Citation39 However, this suppressive effect was only observed at a concentration of recombinant TRAIL of 50 μg/mL, which is ∼50-fold higher than the highest concentration of 1 ug/mL typically used in literature and by us here for PD-L1:TRAIL.
Of note, early clinical trials in various types of malignancies yielded only limited clinical benefit for dulanermin with stable disease being the best-reported activity.Citation38,40,41 However, it has become evident that first generation TRAIL receptor-agonists do not optimally exploit the unique apoptotic signaling characteristics of the various TRAIL receptors (reviewed inCitation42-44). Most notably, apoptotic signaling via TRAIL-R2, one of the two agonistic TRAIL receptors, is not efficiently achieved by soluble homotrimeric rhTRAIL, as TRAIL-R2 requires binding of membrane-bound TRAIL or secondarily cross-linked rhTRAIL.Citation21,45,46 Since TRAIL-R2 is often highly expressed on cancer cells it forms an important target for TRAIL-based therapy. Importantly, we and others have previously shown that tumor-directed scFv:TRAIL fusion proteins effectively activate TRAIL-R2 on targeted cancer cells only. In this process, high affinity and tumor-selective binding via its scFv domain converts a soluble and essentially inactive scFv:TRAIL fusion protein into membrane-bound TRAIL with highly potent agonistic activity toward both TRAIL-R1 and TRAIL-R2.Citation21,47,48 Thus, anti-PD-L1:TRAIL has combined and mutually reinforcing PD-L1-blocking and TRAILR agonistic activities within one therapeutic anticancer fusion protein, which outperforms combined treatment with PD-L1-blocking antibody and TRAIL.
Importantly, in mixed culture experiments using primary patient-derived melanoma cells and autologous TILs, treatment with anti-PD-L1:TRAIL enhanced IFNγ production and augmented TIL-mediated cancer cell apoptosis. Additionally, when T cells were redirected to carcinoma cells using an anti-EpCAM:anti-CD3 bispecific antibody, anti-PD-L1:TRAIL synergistically enhanced their pro-apoptotic antitumor activity. A similar potentiating effect of PD-L1 blockade has been previously reported for T cell retargeting BiTEs that target CEA or CD33.Citation49,50 This suggests that anti-PD-L1:TRAIL may be exploited to augment tumor-specific activity of T cells. In line with this, anti-PD-L1:TRAIL also increased IFNγ secretion in a model antigen-specific reaction where T-cells from CMV-positive donors were stimulated with CMV protein pp65.
Tumor cells that do not constitutively express PD-L1 can rapidly upregulate PD-L1 when the tumor micro-environment is infiltrated by T cells. As previously reported, this upregulation is likely due to IFNγ generated by T cells upon tumor cell recognition and attempted elimination.Citation12 In the context of anti-PD-L1:TRAIL, this IFNγ production may contribute to its antitumor efficacy as IFNγ not only sensitizes cancer cells to TRAIL but also upregulates PD-L1 expression.Citation11,30,31 Thus, anti-PD-L1:TRAIL may trigger a feed-forward loop of increasing IFNγ, increasing PD-L1 expression, and increasing TRAIL sensitivity.
In the tumor microenvironment, various myeloid cells such as macrophages and DCs, also express PD-L1 and hereby can suppress antitumor immunity.Citation33,34 The presence of these suppressive cell types correlated with disease progression and reduced survival in HCC and breast cancer patients.Citation6,51 Here, we showed that anti-PD-L1:TRAIL can arm PD-L1-expressing monocytes, DCs, and macrophages with TRAIL, turning these suppressive cell types into pro-apoptotic effector cells while simultaneously blocking potential PD-L1-mediated immunosuppressive effects. The obvious promise of this arming strategy is illustrated by reports where PD-L1 blockade alone significantly improved the in vivo antitumor activity of T cells treated with suppressive DCs or monocytes.Citation6,33
Interestingly, based on the known receptor interactions of PD-1 ligands (reviewed inCitation3), PD-1 antibodies may have distinct biological activities from PD-L1 antibodies and their activities may not be redundant, depending on the dominant interaction for a particular cancer. In this respect, simultaneous blockade of PD-1 and PD-L1 maximized cytolytic T cell activity of tumor-directed bispecific T cell engaging antibodies.Citation49 These findings suggest that combining anti-PD-L1:TRAIL with PD-1-blocking antibodies may further optimize checkpoint inhibitor-based therapy.
In conclusion, fusion protein anti-PD-L1:TRAIL has promising multi-fold and mutually reinforcing therapeutic effects comprised of PD-L1 checkpoint blockade and simultaneous induction of TRAIL-mediated apoptosis. This new fusion protein may provide possibilities to enhance the efficacy of therapeutic PD-L1/PD-1 checkpoint inhibition alone or in combination with other immunotherapeutic strategies.
Disclosure of potential conflicts of interest
No potential conflicts of interest were disclosed.
KONI_A_1202390_supplementary_materials.zip
Download Zip (694.5 KB)Funding
This work was supported by grants of the Dutch Cancer Society (RUG2009-4355, RUG2009-4542, RUG2011-5206, RUG2012-5541, RUG2013-6209, RUG2014-6986) and the China Scholarship Council.
References
- Brahmer JR, Tykodi SS, Chow LQ, Hwu WJ, Topalian SL, Hwu P, Drake CG, Camacho LH, Kauh J, Odunsi K et al. Safety and activity of anti-PD-L1 antibody in patients with advanced cancer. N Engl J Med 2012; 366:2455; PMID:22658128; http://dx.doi.org/10.1056/NEJMoa1200694
- Topalian SL, Hodi FS, Brahmer JR, Gettinger SN, Smith DC, McDermott DF, Powderly JD, Carvajal RD, Sosman JA, Atkins MB et al. Safety, activity, and immune correlates of anti-PD-1 antibody in cancer. N Engl J Med 2012; 366:2443-54; PMID:22658127; http://dx.doi.org/10.1056/NEJMoa1200690
- Keir ME, Butte MJ, Freeman GJ, Sharpe AH. PD-1 and its ligands in tolerance and immunity. Annu Rev Immunol 2008; 26:677-704; PMID:18173375; http://dx.doi.org/10.1146/annurev.immunol.26.021607.090331
- Freeman GJ, Long AJ, Iwai Y, Bourque K, Chernova T, Nishimura H, Fitz LJ, Malenkovich N, Okazaki T, Byrne MC et al. Engagement of the PD-1 immunoinhibitory receptor by a novel B7 family member leads to negative regulation of lymphocyte activation. J Exp Med 2000; 192:1027-34; PMID:11015443; http://dx.doi.org/10.1084/jem.192.7.1027
- Loke P, Allison JP. PD-L1 and PD-L2 are differentially regulated by Th1 and Th2 cells. Proc Natl Acad Sci U S A 2003; 100:5336-41; PMID:12697896; http://dx.doi.org/10.1073/pnas.0931259100
- Kuang DM, Zhao Q, Peng C, Xu J, Zhang JP, Wu C, Zheng L. Activated monocytes in peritumoral stroma of hepatocellular carcinoma foster immune privilege and disease progression through PD-L1. J Exp Med 2009; 206:1327-37; PMID:19451266; http://dx.doi.org/10.1084/jem.20082173
- Nishimura H, Nose M, Hiai H, Minato N, Honjo T. Development of lupus-like autoimmune diseases by disruption of the PD-1 gene encoding an ITIM motif-carrying immunoreceptor. Immunity 1999; 11:141-51; PMID:10485649; http://dx.doi.org/10.1016/S1074-7613(00)80089-8
- Ishida M, Iwai Y, Tanaka Y, Okazaki T, Freeman GJ, Minato N, Honjo T. Differential expression of PD-L1 and PD-L2, ligands for an inhibitory receptor PD-1, in the cells of lymphohematopoietic tissues. Immunol Lett 2002; 84:57-62; PMID:12161284; http://dx.doi.org/10.1016/S0165-2478(02)00142-6
- Yamazaki T, Akiba H, Iwai H, Matsuda H, Aoki M, Tanno Y, Shin T, Tsuchiya H, Pardoll DM, Okumura K et al. Expression of programmed death 1 ligands by murine T cells and APC. J Immunol 2002; 169:5538-45; PMID:12421930; http://dx.doi.org/10.4049/jimmunol.169.10.5538
- Dong H, Strome SE, Matteson EL, Moder KG, Flies DB, Zhu G, Tamura H, Driscoll CL, Chen L. Costimulating aberrant T cell responses by B7-H1 autoantibodies in rheumatoid arthritis. J Clin Invest 2003; 111:363-70; PMID:12569162; http://dx.doi.org/10.1172/JCI16015
- Dong H, Strome SE, Salomao DR, Tamura H, Hirano F, Flies DB, Roche PC, Lu J, Zhu G, Tamada K et al. Tumor-associated B7-H1 promotes T-cell apoptosis: A potential mechanism of immune evasion. Nat Med 2002; 8:793-800; PMID:12091876; http://dx.doi.org/10.1038/nm730
- Taube JM, Anders RA, Young GD, Xu H, Sharma R, McMiller TL, Chen S, Klein AP, Pardoll DM, Topalian SL et al. Colocalization of inflammatory response with B7-h1 expression in human melanocytic lesions supports an adaptive resistance mechanism of immune escape. Sci Transl Med 2012; 4:127ra37; PMID:22461641; http://dx.doi.org/10.1126/scitranslmed.3003689
- Ahmadzadeh M, Johnson LA, Heemskerk B, Wunderlich JR, Dudley ME, White DE, Rosenberg SA. Tumor antigen-specific CD8 T cells infiltrating the tumor express high levels of PD-1 and are functionally impaired. Blood 2009; 114:1537-44; PMID:19423728; http://dx.doi.org/10.1182/blood-2008-12-195792
- Massi D, Brusa D, Merelli B, Ciano M, Audrito V, Serra S, Buonincontri R, Baroni G, Nassini R, Minocci D et al. PD-L1 marks a subset of melanomas with a shorter overall survival and distinct genetic and morphological characteristics. Ann Oncol 2014; 25:2433-42; PMID:25223485; http://dx.doi.org/10.1093/annonc/mdu452
- Thompson RH, Gillett MD, Cheville JC, Lohse CM, Dong H, Webster WS, Krejci KG, Lobo JR, Sengupta S, Chen L et al. Costimulatory B7-H1 in renal cell carcinoma patients: Indicator of tumor aggressiveness and potential therapeutic target. Proc Natl Acad Sci U S A 2004; 101:17174-9; PMID:15569934; http://dx.doi.org/10.1073/pnas.0406351101
- Larkin J, Chiarion-Sileni V, Gonzalez R, Grob JJ, Cowey CL, Lao CD, Schadendorf D, Dummer R, Smylie M, Rutkowski P et al. Combined nivolumab and ipilimumab or monotherapy in untreated melanoma. N Engl J Med 2015; 373:23-34; PMID:26027431; http://dx.doi.org/10.1056/NEJMoa1504030
- Twyman-Saint Victor C, Rech AJ, Maity A, Rengan R, Pauken KE, Stelekati E, Benci JL, Xu B, Dada H, Odorizzi PM et al. Radiation and dual checkpoint blockade activate non-redundant immune mechanisms in cancer. Nature 2015; 520:373-7; PMID:25754329; http://dx.doi.org/10.1038/nature14292
- Park SS, Dong H, Liu X, Harrington SM, Krco CJ, Grams MP, Mansfield AS, Furutani KM, Olivier KR, Kwon ED. PD-1 restrains radiotherapy-induced abscopal effect. Cancer Immunol Res 2015; 3:610-9; PMID:25701325; http://dx.doi.org/10.1158/2326-6066.CIR-14-0138
- Bremer E. Targeting of the tumor necrosis factor receptor superfamily for cancer immunotherapy. ISRN Oncol 2013; 2013:371854; PMID:23840967; http://dx.doi.org/10.1155/2013/371854
- Ashkenazi A, Holland P, Eckhardt SG. Ligand-based targeting of apoptosis in cancer: The potential of recombinant human apoptosis ligand 2/Tumor necrosis factor-related apoptosis-inducing ligand (rhApo2L/TRAIL). J Clin Oncol 2008; 26:3621-30; PMID:18640940; http://dx.doi.org/10.1200/JCO.2007.15.7198
- Wajant H, Moosmayer D, Wuest T, Bartke T, Gerlach E, Schonherr U, Peters N, Scheurich P, Pfizenmaier K. Differential activation of TRAIL-R1 and -2 by soluble and membrane TRAIL allows selective surface antigen-directed activation of TRAIL-R2 by a soluble TRAIL derivative. Oncogene 2001; 20:4101-6; PMID:11494138; http://dx.doi.org/10.1038/sj.onc.1204558
- de Bruyn M, Rybczynska AA, Wei Y, Schwenkert M, Fey GH, Dierckx RA, van Waarde A, Helfrich W, Bremer E. Melanoma-associated chondroitin sulfate proteoglycan (MCSP)-targeted delivery of soluble TRAIL potently inhibits melanoma outgrowth in vitro and in vivo. Mol Cancer 2010; 9:301; PMID:21092273; http://dx.doi.org/10.1186/1476-4598-9-301
- Bremer E, Samplonius DF, van Genne L, Dijkstra MH, Kroesen BJ, de Leij LF, Helfrich W. Simultaneous inhibition of epidermal growth factor receptor (EGFR) signaling and enhanced activation of tumor necrosis factor-related apoptosis-inducing ligand (TRAIL) receptor-mediated apoptosis induction by an scFv:STRAIL fusion protein with specificity for human EGFR. J Biol Chem 2005; 280:10025-33; PMID:15644326; http://dx.doi.org/10.1074/jbc.M413673200
- Wiersma VR, He Y, Samplonius DF, van Ginkel RJ, Gerssen J, Eggleton P, Zhou J, Bremer E, Helfrich W. A CD47-blocking TRAIL fusion protein with dual pro-phagocytic and pro-apoptotic anticancer activity. Br J Haematol 2014; 164:304-7; PMID:24164421; http://dx.doi.org/10.1111/bjh.12617
- de Bruyn M, Wei Y, Wiersma VR, Samplonius DF, Klip HG, van der Zee AG, Yang B, Helfrich W, Bremer E. Cell surface delivery of TRAIL strongly augments the tumoricidal activity of T cells. Clin Cancer Res 2011; 17:5626-37; PMID:21753155; http://dx.doi.org/10.1158/1078-0432.CCR-11-0303
- Wiersma VR, de Bruyn M, Shi C, Gooden MJ, Wouters MC, Samplonius DF, Hendriks D, Nijman HW, Wei Y, Zhou J et al. C-type lectin-like molecule-1 (CLL1)-targeted TRAIL augments the tumoricidal activity of granulocytes and potentiates therapeutic antibody-dependent cell-mediated cytotoxicity. MAbs 2015; 7:321-30; PMID:25760768; http://dx.doi.org/10.1080/19420862.2015.1007811
- Bremer E, Kuijlen J, Samplonius D, Walczak H, de Leij L, Helfrich W. Target cell-restricted and -enhanced apoptosis induction by a scFv:STRAIL fusion protein with specificity for the pancarcinoma-associated antigen EGP2. Int J Cancer 2004; 109:281-90; PMID:14750182; http://dx.doi.org/10.1002/ijc.11702
- Kroesen BJ, Nieken J, Sleijfer DT, Molema G, de Vries EG, Groen HJ, Helfrich W, The TH, Mulder NH, de Leij L. Approaches to lung cancer treatment using the CD3 x EGP-2-directed bispecific monoclonal antibody BIS-1. Cancer Immunol Immunother 1997; 45:203-6; PMID:9435874; http://dx.doi.org/10.1007/s002620050433
- Mia S, Warnecke A, Zhang XM, Malmstrom V, Harris RA. An optimized protocol for human M2 macrophages using M-CSF and IL-4/IL-10/TGF-β yields a dominant immunosuppressive phenotype. Scand J Immunol 2014; 79:305-14; PMID:24521472; http://dx.doi.org/10.1111/sji.12162
- Langaas V, Shahzidi S, Johnsen JI, Smedsrod B, Sveinbjornsson B. Interferon-gamma modulates TRAIL-mediated apoptosis in human colon carcinoma cells. Anticancer Res 2001; 21:3733-8; PMID:11911240
- Ruiz-Ruiz C, Lopez-Rivas A. Mitochondria-dependent and -independent mechanisms in tumour necrosis factor-related apoptosis-inducing ligand (TRAIL)-induced apoptosis are both regulated by interferon-gamma in human breast tumour cells. Biochem J 2002; 365:825-32; PMID:11936954; http://dx.doi.org/10.1042/BJ20020184
- Flieger D, Hoff AS, Sauerbruch T, Schmidt-Wolf IG. Influence of cytokines, monoclonal antibodies and chemotherapeutic drugs on epithelial cell adhesion molecule (EpCAM) and LewisY antigen expression. Clin Exp Immunol 2001; 123:9-14; PMID:11167991; http://dx.doi.org/10.1046/j.1365-2249.2001.01435.x
- Curiel TJ, Wei S, Dong H, Alvarez X, Cheng P, Mottram P, Krzysiek R, Knutson KL, Daniel B, Zimmermann MC et al. Blockade of B7-H1 improves myeloid dendritic cell-mediated antitumor immunity. Nat Med 2003; 9:562-7; PMID:12704383; http://dx.doi.org/10.1038/nm863
- Bloch O, Crane CA, Kaur R, Safaee M, Rutkowski MJ, Parsa AT. Gliomas promote immunosuppression through induction of B7-H1 expression in tumor-associated macrophages. Clin Cancer Res 2013; 19:3165-75; PMID:23613317; http://dx.doi.org/10.1158/1078-0432.CCR-12-3314
- Stewart R, Morrow M, Hammond SA, Mulgrew K, Marcus D, Poon E, Watkins A, Mullins S, Chodorge M, Andrews J et al. Identification and pre-clinical characterization of MEDI4736, an antagonistic anti-PD-L1 monoclonal antibody. Cancer Immunol Res 2015; 3:1052-1062; PMID:25943534; http://dx.doi.org/10.1158/2326-6066.CIR-14-0191
- Ashkenazi A, Pai RC, Fong S, Leung S, Lawrence DA, Marsters SA, Blackie C, Chang L, McMurtrey AE, Hebert A et al. Safety and antitumor activity of recombinant soluble Apo2 ligand. J Clin Invest 1999; 104:155-62; PMID:10411544; http://dx.doi.org/10.1172/JCI6926
- Fox NL, Humphreys R, Luster TA, Klein J, Gallant G. Tumor necrosis factor-related apoptosis-inducing ligand (TRAIL) receptor-1 and receptor-2 agonists for cancer therapy. Expert Opin Biol Ther 2010; 10:1-18; PMID:19857186; http://dx.doi.org/10.1517/14712590903319656
- Herbst RS, Eckhardt SG, Kurzrock R, Ebbinghaus S, O'Dwyer PJ, Gordon MS, Novotny W, Goldwasser MA, Tohnya TM, Lum BL et al. Phase I dose-escalation study of recombinant human Apo2L/TRAIL, a dual proapoptotic receptor agonist, in patients with advanced cancer. J Clin Oncol 2010; 28:2839-46; PMID:20458040; http://dx.doi.org/10.1200/JCO.2009.25.1991
- Lehnert C, Weiswange M, Jeremias I, Bayer C, Grunert M, Debatin KM, Strauss G. TRAIL-receptor costimulation inhibits proximal TCR signaling and suppresses human T cell activation and proliferation. J Immunol 2014; 193:4021-31; PMID:25217163; http://dx.doi.org/10.4049/jimmunol.1303242
- Soria JC, Smit E, Khayat D, Besse B, Yang X, Hsu CP, Reese D, Wiezorek J, Blackhall F. Phase 1b study of dulanermin (recombinant human Apo2L/TRAIL) in combination with paclitaxel, carboplatin, and bevacizumab in patients with advanced non-squamous non-small-cell lung cancer. J Clin Oncol 2010; 28:1527-33; PMID:20159815; http://dx.doi.org/10.1200/JCO.2009.25.4847
- Soria JC, Mark Z, Zatloukal P, Szima B, Albert I, Juhasz E, Pujol JL, Kozielski J, Baker N, Smethurst D et al. Randomized phase II study of dulanermin in combination with paclitaxel, carboplatin, and bevacizumab in advanced non-small-cell lung cancer. J Clin Oncol 2011; 29:4442-51; PMID:22010015; http://dx.doi.org/10.1200/JCO.2011.37.2623
- de Bruyn M, Bremer E, Helfrich W. Antibody-based fusion proteins to target death receptors in cancer. Cancer Lett 2013; 332:175-83; PMID:21215513; http://dx.doi.org/10.1016/j.canlet.2010.11.006
- Ashkenazi A. Targeting the extrinsic apoptotic pathway in cancer: Lessons learned and future directions. J Clin Invest 2015; 125:487-9; PMID:25642709; http://dx.doi.org/10.1172/JCI80420
- de Miguel D, Lemke J, Anel A, Walczak H, Martinez-Lostao L. Onto better TRAILs for cancer treatment. Cell Death Differ Advance online publication 4 March 2016; 23(5):733-47; PMID:26943322; http://dx.doi.org/10.1038/cdd.2015.174
- Nair PM, Flores H, Gogineni A, Marsters S, Lawrence DA, Kelley RF, Ngu H, Sagolla M, Komuves L, Bourgon R et al. Enhancing the antitumor efficacy of a cell-surface death ligand by covalent membrane display. Proc Natl Acad Sci U S A 2015; 112:5679-84; PMID:25902490; http://dx.doi.org/10.1073/pnas.1418962112
- De Miguel D, Gallego-Lleyda A, Galan-Malo P, Rodriguez-Vigil C, Marzo I, Anel A, Martinez-Lostao L. Immunotherapy with liposome-bound TRAIL overcomes partial protection to soluble TRAIL-induced apoptosis offered by downregulation of bim in leukemic cells. Clin Transl Oncol 2015; 17:657-67; PMID:25967100; http://dx.doi.org/10.1007/s12094-015-1295-x
- Bremer E, de Bruyn M, Wajant H, Helfrich W. Targeted cancer immunotherapy using ligands of the tumor necrosis factor super-family. Curr Drug Targets 2009; 10:94-103; PMID:19199904; http://dx.doi.org/10.2174/138945009787354593
- Aronin A, Amsili S, Prigozhina TB, Tzdaka K, Rachmilewitz J, Shani N, Tykocinski ML, Dranitzki Elhalel M. Fn14*TRAIL effectively inhibits hepatocellular carcinoma growth. PLoS One 2013; 8:e77050; PMID:24130833; http://dx.doi.org/10.1371/journal.pone.0077050
- Osada T, Patel SP, Hammond SA, Osada K, Morse MA, Lyerly HK. CEA/CD3-bispecific T cell-engaging (BiTE) antibody-mediated T lymphocyte cytotoxicity maximized by inhibition of both PD1 and PD-L1. Cancer Immunol Immunother 2015; 64:677-88; PMID:25742933; http://dx.doi.org/10.1007/s00262-015-1671-y
- Krupka C, Kufer P, Kischel R, Zugmaier G, Lichtenegger FS, Kohnke T, Vick B, Jeremias I, Metzeler KH, Altmann T et al. Blockade of the PD-1/PD-L1 axis augments lysis of AML cells by the CD33/CD3 BiTE antibody construct AMG 330: Reversing a T-cell-induced immune escape mechanism. Leukemia 2016; 30:484-491; PMID:26239198; http://dx.doi.org/10.1038/leu.2015.214
- Treilleux I, Blay JY, Bendriss-Vermare N, Ray-Coquard I, Bachelot T, Guastalla JP, Bremond A, Goddard S, Pin JJ, Barthelemy-Dubois C et al. Dendritic cell infiltration and prognosis of early stage breast cancer. Clin Cancer Res 2004; 10:7466-74; PMID:15569976; http://dx.doi.org/10.1158/1078-0432.CCR-04-0684