Abstract
This review concentrates on how artificial cells can contribute to helping patients with COVID-19. Artificial cells have led to mRNA vaccines with more improvements to come. Excessive cytokines in severe COVID-19 can damage organs leading to death. Artificial cell-based collodion macroporous activated charcoal adsorbent can effectively remove middle molecular weight range molecules in patients. A novel hemoperfusion device based on collodion membrane macroporous synthetic resin effectively removes cytokines and recovery in COVID-19 patients. This has been approved as an emergency treatment for COVID-19 in China, Europe, and Canada. A recent nanobiotherapeutic containing haemoglobin and up to six times the concentration of red blood cell enzymes: catalase, superoxide dismutase and carbonic anhydrase. In an animal study, this can effectively lower the damaging increase in free radicals and the removal of increased tissue pCO2. This can also help as blood substitute for the severe and critical problem of COVID-19 pandemic donor blood supply crisis.
COVID-19 and its variants have resulted in major pandemics, severe sicknesses, and deaths around the world. COVID-19 and its variants has only started less than 3 years ago, and it is even more recently that we know more about its mechanisms, requirements, prevention, and treatment. This being the case, this is the first review on the present status and future perspectives of the use of the principle of artificial cells for COVID-19 related to vaccines, treatment, and critical donor blood supply shortage.
KEY MESSAGES
Why do we need artificial cells for COVID-19?
General: COVID-19
In December 2019, there was an outbreak of coronavirus disease (COVID-19), caused by the severe acute respiratory syndrome coronavirus 2 (SARS-CoV-2). Epidemiology, clinical characteristics, risk factors for mortality and clinical course have been described [Citation1]. This has become a pandemic with major outbreaks around the world. What is worse is that new variants develop and within 2 years, many countries have already experienced 6 waves. Many patients with very severe COVID-19 have died despite ventilator and oxygenator support. Furthermore, the pandemic has resulted for the first time a severe donor blood supply crisis. Thus, it is important to (1) develop suitable vaccines (2) Find a possible way of treatment (3) way to solve the critical donor blood supply crisis. This paper describes examples of the use of the idea of artificial cells towards these aims. First, what are artificial cells?
What are artificial cells?
Artificial cells are attempts to mimic some of the properties of biological cells for use in medicine. This author prepared the first artificial cells by enclosing the content of red blood cells inside ultrathin polymer membranes of cellular dimensions () [Citation3,Citation4]. He then extended this research by going outside the box with variations in contents, membrane composition, and configurations (). Contents of artificial cells include haemoglobin, enzymes, cells, vaccines, compartments, cytosol, organelles, magnetics, adsorbent, insulin and later, stem cells, gene, DNA, mRNA, silver, gold, miroorganisms, biotechnological products and others (). Membrane composition include polymeric membrane, lipid membrane, biodegradable membrane, crosslinked protein membrane, conjugation, lipid-polymeric membrane, PEGalated membrane and others (). It has since been developed around the world into many configurations and dimensions under different names for different specific applications () [Citation2,Citation5–19]. One can now taylor-made Artificial Cells to suit specific applications. It has now evolved into many different areas including blood substitutes, hemoperfusion, nanomedicine, nanobiotherapeutics, drug delivery, regenerative medicine, cell/stem cell encapsulation, nanoparticles, liposomes, bioencapsulation, and other areas. Artificial cell is now a very large area and reviews on artificial cells are available elsewhere [Citation2,Citation5,Citation7]. is a summary of the area.
Figure 1. The evolution of the idea of artificial cells with extensive variations of contents, membrane material, dimensions (left) and configurations (centre) often with new terminology for each configuration. Right: mainly shows medical applications. (From Chang [Citation2] with written copyright permission to reproduce this figure from the publisher Taylor and Francis.)
![Figure 1. The evolution of the idea of artificial cells with extensive variations of contents, membrane material, dimensions (left) and configurations (centre) often with new terminology for each configuration. Right: mainly shows medical applications. (From Chang [Citation2] with written copyright permission to reproduce this figure from the publisher Taylor and Francis.)](/cms/asset/2232987c-5cb5-4f21-b074-88586f500359/ianb_a_2126491_f0001_c.jpg)
The role of artificial cells in the fight against COVID-19
The present paper is not a review of the very large area of artificial cells that has already been reviewed in 2019 [Citation2]. It is only the present status and future perspectives of the use of artificial cells for COVID-19 since the 2019 pandemic: (1) as carriers for vaccine (2) Novel artificial cell-based hemoperfusion for the removal of damaging cytokines and for the partial support of kidney and liver failure (3) Nanobiotherapeutics for the removal of oxygen radicals and CO2 (4) for blood supply in pandemic donor blood supply crisis ().
Table 1. Artificial cell and the needs related to COVID-19.
Artificial cell as carrier for COVID-19 vaccine
Introduction
Many excellent and detailed reviews have been written on COVID-19 vaccines [Citation14,Citation20–22]. The successful development of the COVID-19 vaccine is the result of research by many groups around the world over many years. It takes time for an idea or basic research to be developed for eventual clinical use and we cannot wait for the need to come before doing serious research and development. This author first proposed the use of artificial cells for vaccine in the mid-1970s [Citation12,Citation13]. It was too early for its time and there was not much follow-up research on this. Similarly, there was not too much interest in synthetic mRNA, but researchers also persisted and came out with a suitable mRNA [Citation14]. However, there are problems with mRNA that include (1) the destruction of mRNA by ribonucleases when injected (2) the negatively charged mRNA has problem entering the cell because of the negative potential across the cell membrane. Fortunately, centres around the world have been developing artificial cells as carriers for other biologics and therapeutics and the combined effort of many centres around the world over many years have resulted in readily available solution ( and ) [Citation2–13,Citation15–22].
Figure 2. The many steps from many laboratories around the world over many years to finally result in successful clinical uses of mRNA vaccines. Research over many years (right) have made it possible to taylor-made an artificial cell carrier suitable for mRNA (lower left). mRNA is bioencapsulated inside lipid-polymeric membrane artificial cells to prevent it from being inactivated by ribonucleases, positively charged membrane facilitates its uptake by the COVID-19 virus. Polyethylene glycol on the surface of lipid membrane prevents the nanoparticles from quick removal by macrophages after infusion.
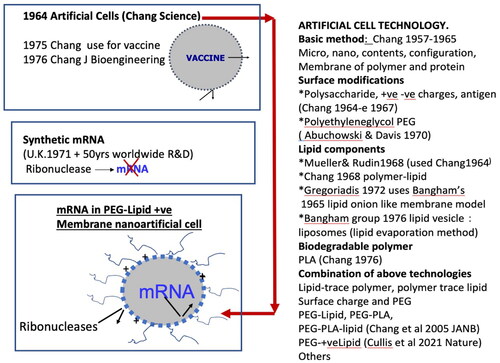
Figure 3. Routes of intracellular drug delivery. Left to right: (1) It can fuse with cell membrane to release the drug into the cell. (2) It can be taken up by endocytosis to form endosome that can enter lysosomes where the drug is released. This is the mechanism for the present PEG-lipid-mRNA (3) It can release the drug outside the cell. (4) with surface antibody can bind to antigen receptor and enter the cell as endosome. (From Chang [Citation7] with written copyright permission to reproduce this figure from the publisher World Science Publisher.)
![Figure 3. Routes of intracellular drug delivery. Left to right: (1) It can fuse with cell membrane to release the drug into the cell. (2) It can be taken up by endocytosis to form endosome that can enter lysosomes where the drug is released. This is the mechanism for the present PEG-lipid-mRNA (3) It can release the drug outside the cell. (4) with surface antibody can bind to antigen receptor and enter the cell as endosome. (From Chang [Citation7] with written copyright permission to reproduce this figure from the publisher World Science Publisher.)](/cms/asset/3dfee941-95e1-47ea-a1bb-2a4c605554d1/ianb_a_2126491_f0003_b.jpg)
Development towards a suitable carrier for mRNA
Components of the membrane of artificial cells. In 1968 a group reported that they were able to use of the original 1964 artificial cell method [Citation3] to prepare single bilayer membrane vesicles [Citation16]. In 1972, artificial cells with protein-lipid membrane or polymer-lipid membrane were prepared [Citation4]. Microspheres of onion-like concentric multilamellar lipid bilayers were originally used as membrane models in basic research [Citation17]. This was later developed into drug carrier [Citation18].
However, these onion-like multi-lamellar structures limit the loading of drugs. In 1976 researchers used an ‘ether evaporation’ method to form single bilayer lipid membrane vesicles [Citation19]. This ‘ether evaporation method’ is an extension of one of the original methods using ether for the preparation of artificial cells [Citation3,Citation4]. These lipid membrane artificial cells, also called liposomes, have since been extensively studied and used as drug delivery systems around the world [Citation2,Citation6,Citation9–11,Citation20–22]. All the above research has resulted in the availability of suitable vaccine carriers and the rapid progress and success of the mRNA and other vaccines ().
Final version of the mRNA vaccine
Thus, mRNA is enclosed within PEG-lipid positively charged membrane nanodimension artificial cells with the following functions () The enclosing membrane excludes ribonucleases from destroying the enclosed mRNA, The PEG component prevents the nano artificial cells from being removed quickly by macrophage. The positive charge surface facilitates the entrance of the nano artificial cells across the negatively charged membrane potential by endocytosis into the cell in the form of endosome and is later released to carry out its function ().
Future
The present widely used mRNA vaccines are successful. However, much improvement for longer term function is on the horizon with other approaches in addition to mRNA and the use of other configurations and membrane material for artificial cell including biodegradable polymeric artificial cells and other configurations [Citation2,Citation9–11,Citation20–22].
Removal of damaging cytokine in COVID-19 patients
Cytokines STORM in COVID-19
Patients with very severe COVID-19 may die despite ventilator and oxygenator support due to ‘cytokine storm’, a sudden and massive increase in cytokines including interleukin (IL)-6, IL-2, IL-7, IL-10, and tumour necrosis factor [Citation1,Citation23].
Pro-inflammatory IL-6 is the most important causative cytokine in CRS (Cytokine release syndrome) also called ‘cytokine storm’ can damage organs resulting in multi-organ failure and death of the patients [Citation1,Citation23]. Thus, it is important to remove these inflammatory cytokines. However, these are rather large molecules. Furthermore, these patients are severely sick, and the treatment must involve methods that do not aggravate the condition. We have earlier developed artificial cell containing adsorbents like activated charcoal or resin [Citation24]. This has been used to prepare artificial cell based hemoperfusion device [Citation4,Citation24–27], that is (1) effective in removing from blood molecules in the larger middle molecular weight range and (2) Unlike haemodialysis it did not cause hypotension in severely ill patients.
Artificial cell-based hemoperfusion for toxin removal and partial kidney and liver support
Basic principle
My analysis of the effect of diameter and membrane thickness of artificial cells on mass transfer shows unexpected results [Citation24]. shows the theoretical mass transfer of a fixed volume of 10 nm membrane thickness artificial cells with different diameters. This is compared to an artificial kidney (haemodialysis) machine available at that time with a mass transfer of 1. At the micro diameter, the theoretical mass transfer of artificial cells can be 100 times that of an artificial kidney. It is even higher at the nanodimension. This forms the basis of using artificial cells as highly efficient micro/nano dialyser/bioreactor ().
Figure 4. Centre: Theoretical mass transfer of 5 ml 0.01 um membrane thickness artificial cells with different diameters. This is compared an artificial kidney machine with a mass transfer of 1. Upper right: Thus, artificial cells containing bioactive material can become efficient micro/nano dialyser/bioreactor. Lower: 70 grams 90-micron diameter adsorbent artificial cells retained inside a small container by screens at either end. Left: Its small size (yellow circle) is compared to an artificial kidney. (From Chang [Citation11] with copyright permission to reproduce this figure from the publisher Taylor and Francis.)
![Figure 4. Centre: Theoretical mass transfer of 5 ml 0.01 um membrane thickness artificial cells with different diameters. This is compared an artificial kidney machine with a mass transfer of 1. Upper right: Thus, artificial cells containing bioactive material can become efficient micro/nano dialyser/bioreactor. Lower: 70 grams 90-micron diameter adsorbent artificial cells retained inside a small container by screens at either end. Left: Its small size (yellow circle) is compared to an artificial kidney. (From Chang [Citation11] with copyright permission to reproduce this figure from the publisher Taylor and Francis.)](/cms/asset/ed6539dc-f130-4cf7-af4c-fe39d2cdeed7/ianb_a_2126491_f0004_c.jpg)
Collodion membrane-activated charcoal
For example, 70 g of 90-micron diameter artificial cells containing activated charcoal are retained inside a small container by screens at either end. The artificial cell membrane separates the adsorbent from damaging the blood cells and prevents adsorbent particles from being released to cause adverse effects. The sorbent in the artificial cells removes toxins or drugs from the blood of patients perfusing through the column (). The result is a miniaturised cup size hemoperfusion device with much higher efficiency than a washing machine size haemodialysis machine (). In addition to adsorbent artificial cells can also contain other bioreactors ().
Clinical use of artificial cell hemoperfusion (collodion membrane activated charcoal) in patients
The author personally carried out a study on the use of artificial cells containing adsorbents for hemoperfusion. This included personally carried out scaled up, animal testing and clinical trial in patients. He showed the safety and effectiveness for using this first in animals then in patients. shows the result of one of the many patients the author has treated [Citation25,Citation26,Citation28]. This is a suicidal patient who ingests 3 times the lethal dose of a sleep pill, methryprylon [Citation25]. Five hours of standard haemodialysis treatment cannot lower the drug level and the patient remained comatose, hypotensive with cardiac arrests. When the author started hemoperfusion treatment the plasma methryprylon level decreased rapidly in 2 h and the patient was no longer comatose nor hypotensive and shortly recovers completely. This was also carried out successfully in 12 other drug poisoning patients [Citation25,Citation26]. He has also used this for the partial support of kidney failure and liver failure patients [Citation26,Citation28]. Thus, FDA has approved this artificial cell based hemoperfusion for routine clinical uses. What is most relevant to its use in COVID-19 cytokine storm is that it is more effective in removing larger molecules than haemodialysis available at that time () [Citation25,Citation28].
Figure 5. Upper: Activated charcoal is microporous with openings from the surface allowing the easy entrance of larger molecules in the middle molecular range. Ultrathin collodion membrane coating does not obstruct the opening. Thick membrane will obstruct the openings. Lower left: Clinical result in a suicidal overdose patient treated by haemodialysis then by hemoperfusion. Lower centre and lower right: Separate study on a group of renal failure patients using hemoperfusion. The clearance and decrease of middle molecules is much higher compared to haemodialysis at that time. (From Chang [Citation28] with written copyright permission to reproduce this figure from the publisher World Science Publisher.)
![Figure 5. Upper: Activated charcoal is microporous with openings from the surface allowing the easy entrance of larger molecules in the middle molecular range. Ultrathin collodion membrane coating does not obstruct the opening. Thick membrane will obstruct the openings. Lower left: Clinical result in a suicidal overdose patient treated by haemodialysis then by hemoperfusion. Lower centre and lower right: Separate study on a group of renal failure patients using hemoperfusion. The clearance and decrease of middle molecules is much higher compared to haemodialysis at that time. (From Chang [Citation28] with written copyright permission to reproduce this figure from the publisher World Science Publisher.)](/cms/asset/d9034186-1a92-41d0-b2d8-ef9e0ea8703c/ianb_a_2126491_f0005_c.jpg)
It is important to note that the activated charcoal adsorbent is macroporous and 10 nm thin collodion membrane coating does not obstruct the openings () [Citation4]. On the other hand, thick membrane coating would interfere with the ease of entrance of molecules to be removed ().
Commercial devices and a recent novel synthetic microporous resin with collodion membrane for cytokine removal
General
Hemoperfusion is now an accepted routine clinical use for the treatment of patients around the world. A 2017 book [Citation27] by specialists around the world shows that this approach is being used extensively around the world. There are now at least 10 commercial devices around the world [Citation28]. Each company has their own proprietary methods. There are variations in the type of adsorbent, membrane material and membrane thickness and as a result, they are different in effectiveness [Citation28]. There is a new commercial hemoperfusion device (HA330) not included in the 2017 review that has been approved for emergency use for COVID-19 in China, Europe and Canada. This is based on the use of collodion membrane with novel adsorbent in the form of synthetic styrene divinylbenzene copolymers macroporous resin [Citation29,Citation30].
Synthetic macroporous resin with collodion membrane (HA330), for patients with elevated systemic cytokines
Before the onset of COVID-19 this commercial device has been studied in other conditions with the elevation of systemic cytoklines [Citation31–34]. For example, a detailed study in a porcine acute respiratory distress syndrome model shows that hemoperfusion cartridge (HA330), with a blood flow of 200–250 ml/min reduces the circulating and alveolar levels of proinflammatory cytokines, improves oxygenation and helps the recovery of lung injuries in the exudative phase [Citation35]. Another study in patients with multiple organ dysfunction syndrome (MODS) shows the effectiveness of hemoperfusion [Citation36]. Hemoperfusion significantly improves the biochemical indicators, inhibits the activation of NK-κB and significantly reduces the levels of inflammatory cytokines IL-6 and TNF-α in peripheral blood of the patients. A similar effect on the reduction of systemic cytokines are observed in patients with extrapulmonary sepsis-induced acute lung injury [Citation37], Leptospirosis with acute respiratory failure and acute kidney injury [Citation31] and septic shock patients [Citation32].
Use in COVID-19 patients
Very severe cases of COVID-19 can progress rapidly to death. The emergency nature is such that no large-scale control clinical trial has yet been published. However, based on the above large scale clinical result showing its effectiveness for the removal of cytokines in other conditions with cytokine storms, this has been approved for emergency use for severe cases of COVID-19 in China, Europe and Canada.
Case reports of emergency treatments are available [Citation33,Citation34,Citation38]. A Case report of a COVID-19 patient with cytokine storm on day 18 after admission with an elevation of particularly IL-6, IL-8 and TNF-a. Hemoperfusion starts on day 23 and lowers systemic cytokines, especially IL-6, IL-8, and TNF-a and on day 33 lab result reaches normal level and the patient fully recovers [Citation34]. Another case report is a COVID-19 patient with severe acute respiratory distress syndrome (ARDS) with high plasma level of interlukin-1 (IL-1), IL-6, IL-8, and tumour necrosis factor alpha [Citation38]. The chest X-ray shows a recovery of both lungs following the completion of 3 sessions of hemopurification. The inflammatory cytokines 48 h following the last session of hemoperfusion shows the decrease of IL-1, from 523.3 pg/mL to 38.25 pg/mL, IL-6 from 226.35 pg/mL to 210.18 pg/mL, IL-8 from 886.5 pg/mL to 482.4 pg/mL. The patient is finally transferred to the ward with an acceptable clinical condition. Another case report of the successful recovery of severe COVID-19 with cytokine storm treated with hemoperfusion [Citation34]. On day 12 after admission, the IL-6 (198.66 pg/mL) is almost 10 times the normal range (5.9 pg/mL). Treatment includes a double plasma molecular adsorption system and plasma exchange. The levels of interleukin 6 (IL-6) and interferon g (INF) reach peak on day 14 after admission and falling to near normal on day 16. On day 36 the patient is asymptomatic at rest and had no shortness of breath [Citation34]. One looks forward to published results from control clinical trials in COVID-19 patients [Citation39].
Oxygen radicals, elevated tissue pCO2 and donor blood supply crisis in COVID-19
General
Body response to coronavirus includes the release of high levels of oxygen radicals [Citation40–42]. These high levels of oxygen radicals damage body tissue including the lung and the heart resulting in organ damage and failure. The hypothesis of free radicals playing a crucial pathogenic role in damage in COVID-19-induced pneumonia suggests an antioxidative therapeutic strategy for COVID-19 [Citation40]. Others suggest the potential role of free-radical processes in biomolecules damage during COVID-19 and ways of their regulation [Citation41]. There is also a group that proposes that COVID-19: captures iron and generates reactive oxygen species to damage the human immune system [Citation42]. Damage to the lung also interfere with the normal removal of CO2 and the Extracorporeal Oxygenator is more efficient in exchange of oxygen rather than CO2. Inability to remove CO2 can result in the increase of pCO2 in the skeletal muscle and heart muscle resulting in worsening of the patients’ condition [Citation43,Citation44]. There is also severe shortage of donor blood supply, for example, the US Red Cross declares ‘first-ever blood crisis amid Omicron surge’ with resulting severe shortage of blood supply and the delay of many lifesaving procedures [45]. Artificial cells in the form of nanobiotherapeutics can be used to solve these problems ().
Table 2. Nanobiotherapeutics.
Nanobiotherapeutic in COVID-19 for controlling free radicals and pCO2 and also for blood supply in COVID-19 donor blood supply crisis
Nanobiotherapeutics have been developed originally as red blood cell substitutes. Red blood cells have three major functions: (1) transport oxygen from the lung to the tissue, (2) remove damaging oxygen radicals and (3) carry carbon dioxide CO2. from the tissue to the lung to be removed. This has been developed in 3 progressing steps: Step 1. Oxygen carrier; Step 2. oxygen carrier with antioxidant properties and Step 3. oxygen carrier with antioxidant function and CO2 removal functions. Originally these are only to replace red blood cells and not to function as nanobiotherapeutics. We have recently prepared a nanobiotherapeutic with up to 6 times enhancement of red blood cell functions by using enzyme concentration of superoxide dismutase catalase and carbonic anhydrase that are six times that of rbc. This can now function also as a nanobiotherapeutic to enhance the removal of oxygen radicals and CO [Citation46–48].
Oxygen carrier (HBOC)
Step 1 is to prepare an oxygen carrier based on Chang’s glutraldehyde crosslinked polyhemoglobin (PolyHb) [Citation49] later developed independently by Biopure (Hemapure: bovine PolyHb) [Citation50] and Northfield (human PolyHb) [Citation51]. into commercial products. Clinical trials using Hemapure: bovine PolyHb in surgical patients have led to approval for routine clinical use in South Africa to avoid the use of H.I.V contaminated donor blood [Citation50]. Clinical trials for haemorrhagic shock patients using human PolyHb in the ambulance without the need for typing or cross matching [Citation51]. There is a slight increase in cardiac side effect when compared to the control group. As will be discussed later, it is likely that surgical patients only need oxygen carrier [Citation50] whereas, some haemorrhagic shock patients [Citation51] may need all three of the rbc functions.
Oxygen carriers + removal of oxygen radicals
We prepared Polyhemoglobin-Catalase-superoxide dismutase (PolyHb-SOD-CAT) as an oxygen carrier and antioxidant to remove oxygen radicals () [Citation52]. This is effective in removing oxygen radical. Thus, in a 90 min haemorrhagic shock- cerebral ischaemia rat model, there was no brain edoema with the infusion of PolyHb-SOD-CAT () [Citation53]. There was brain edoema with infusion of PolyHb or with infusion of Hb, SOD and CAT that are not crosslinked. Ischaemic small intestine releases damaging oxygen radicals when reperfused with PolyHb. However, PolyHb-SOD-CAT reperfusion does not increase oxygen radical release () [Citation54]. These results show the possible use of this nanobiotherapeutic for removing damaging oxygen radicals.
Figure 6. A soluble complex of Polyhemoglobin containing antioxidant enzymes to remove oxygen radicals (PolyHb-SOD-CAT) [Citation52]. Upper right: Arterial obstruction can result in stroke and heart attack. Red blood cells cannot flow through. PolyHb, a solution, can perfuse through. Upper left: PolyHb-SOD-CAT, a solution can perfuse through to supply oxygen and remove oxygen radicals Lower right: Unlike PolyHb, reinfusion of PolyHb-SOD-CAT does not cause brain edoema in rat brain ischaemia. Lower left: Unlike PolyHb, PolyHb-SOD-CAT reperfusion in ischaemic small intestine does not releases damaging oxygen. (From Chang [Citation11] with written copyright permission to reproduce this figure from the publisher Taylor and Francis.)
![Figure 6. A soluble complex of Polyhemoglobin containing antioxidant enzymes to remove oxygen radicals (PolyHb-SOD-CAT) [Citation52]. Upper right: Arterial obstruction can result in stroke and heart attack. Red blood cells cannot flow through. PolyHb, a solution, can perfuse through. Upper left: PolyHb-SOD-CAT, a solution can perfuse through to supply oxygen and remove oxygen radicals Lower right: Unlike PolyHb, reinfusion of PolyHb-SOD-CAT does not cause brain edoema in rat brain ischaemia. Lower left: Unlike PolyHb, PolyHb-SOD-CAT reperfusion in ischaemic small intestine does not releases damaging oxygen. (From Chang [Citation11] with written copyright permission to reproduce this figure from the publisher Taylor and Francis.)](/cms/asset/a1f8f0ef-9269-447b-b062-02d4e6d6cc72/ianb_a_2126491_f0006_c.jpg)
The work of Hsia’s group using conjugated haemoglobin containing synthetic antioxidants (PNPH) is another way to solve the problem [Citation55]. Simoni et al. uses pharmcological agents [Citation56]. Zal uses HEMO2life [Citation57]. Alayash’s group uses haptoglobin [Citation58]. Bulow’s group uses recombinant foetal hemoglobin [Citation59]. Ascorbic acid is another approach [Citation60].
Oxygen carriers + removal of oxygen radicals + removal of CO2
Increase of tissue pCO2 results in a decrease in the recovery of haemorrhagic shock animals [Citation43]. Furthermore, increase in tissue pCO2 is correlated with the degree of myocardial ischaemia [Citation44]. In COVID-19 patients, damage to the lung interfere with the normal removal of CO2 and the Extracorporeal Oxygenator is more efficient in exchange of oxygen rather than CO2. Our recent 3rd step is to prepare a Polyhemoglobin-Catalase-superoxide dismutase-carbonic anhydrase (PolyHb-SOD-CAT-CA) complex () [Citation46]. This soluble nanobiotechnological complex contains all three major rbc enzymes at up to 6 times the enzyme concentration of rbc. This results in up to 6 times the rbc functions [Citation46]. In a 90-min haemorrhagic shock animal model () [Citation46]. It is better than whole blood in removing elevated intracellular pCO2, avoid ischaemia in the heart, and the histology of the heart and intestine ().
Figure 7. Left: PolyHb-CAT-SOD-CA with up to 6× enzyme enhancement also enzyme stability. Upper right: In a 90 min haemorrhagic shock animal model with 2/3 blood volume loss: it is superior to. PolyHb and whole blood in the lowering of elevated intracellular pCO2. Lower right: Recovery of ST elevation. Lower left: histology of the heart. (From Bian and Chang [Citation46] with written copyright permission to reproduce this figure from the publisher Taylor and Francis.)
![Figure 7. Left: PolyHb-CAT-SOD-CA with up to 6× enzyme enhancement also enzyme stability. Upper right: In a 90 min haemorrhagic shock animal model with 2/3 blood volume loss: it is superior to. PolyHb and whole blood in the lowering of elevated intracellular pCO2. Lower right: Recovery of ST elevation. Lower left: histology of the heart. (From Bian and Chang [Citation46] with written copyright permission to reproduce this figure from the publisher Taylor and Francis.)](/cms/asset/ad1543c7-7322-47fa-b82b-042c49fc2642/ianb_a_2126491_f0007_c.jpg)
This result shows the effectiveness in preventing cardiac side effect that is even better than blood. This is because the enzyme concentration can be up to 6 times that of red blood cells ().
Avoid the critical and severe COVID-19 donor blood supply crisis
So far, we have discussed the therapeutic uses of the above nanobiotherapeutics to remove free radicals and elevated tissue pCO2. However, COVID-19 Pandemic has also caused a severe crisis for donor blood supply. Careful checking of donor blood and donors can prevent the transmission of COVID-19 from donor blood. However, this also decreases the number of qualified donors since those tested positive or those with exposures cannot donate blood. Shut down and avoidance of possible contacts also resulted in the unavailability of blood donors. For example, a recent publication [Citation61] shows that during the pandemic, whole blood donation in a Chinese blood centre dropped by 67% and 81% of potential donors are worried about contact with COVID-19 during donation. The result is a crisis for blood supply during pandemic. The US Red Cross also declares a first-ever blood crisis amid Omicron surge with resulting severe shortage of blood supply and the delay of many lifesavings surgical procedures [Citation45]. The third-generation blood substitutes, PolyHb-SOD-CAT-CA, as described above no longer has problem related to cardiac side effects and is better than donor blood in the following ways (). Unlike donor blood. it has no blood group and can be given immediately on the spot. Donor blood can only be stored for 1 day at room temperature and 42 days at 4° centigrade. Lyophilised PolyHb-SOD-CAT-CA can be stored for 320 days in room temperature and longer at 4° centigrade. Donor blood cannot withstand pasteurised, but lyophilised PolyHb-SOD-CAT-CA can be heat pasteurised at 68 F for 2 h to inactivate inactive organisms. The rbc enzymes can be extracted from bovine rbc inexpensively [Citation62].
Figure 8. Long term study of bovine PolyHb-SOD-CAT-CA in rats. Upper left: Protocol of 4 weekly 5% blood volume infusion followed by 30% volume exchange transfusion. Left and centre: Long term and immunological result. Right: Histamine and tryptase showing no anaphylactic reaction. (From Guo and Chang [Citation47] with writtencopyright permission to reproduce this figure from the publisher Taylor and Frances.)
![Figure 8. Long term study of bovine PolyHb-SOD-CAT-CA in rats. Upper left: Protocol of 4 weekly 5% blood volume infusion followed by 30% volume exchange transfusion. Left and centre: Long term and immunological result. Right: Histamine and tryptase showing no anaphylactic reaction. (From Guo and Chang [Citation47] with writtencopyright permission to reproduce this figure from the publisher Taylor and Frances.)](/cms/asset/d0693f2e-e3c3-4dfc-ac0f-ba3582a9a98c/ianb_a_2126491_f0008_c.jpg)
We studied in rats 4 weekly 5% blood volume infusion of bovine PolyHb- SOD-CAT-CA followed by 30% volume exchange transfusion [Citation47]. The result shows long term safety with no immunological problems. Measurement of histamine and tryptase also show no anaphylactic reaction (). When used in patients bovine PolyHb itself shows no immunological problems [Citation50]. In PolyHb-SOD-CAT-CA, the large excess of haemoglobin molecules bioencapsulated the small fraction of enzyme molecules () [Citation47,Citation48].
Thus, PolyHb-SOD-CAT-CA can help to solve the critical and severe donor blood supply crisis, especially for the following situations [Citation63,Citation64].
Cardic. Cancer and other urgent Surgery that requires the use of donor blood.
Accidents, disasters, or conflicts resulting in severe blood loss require an immediate urgent blood transfusion.
Arterial obstruction can lead to heart attack and stroke. Blood substitute being a solution can perfuse through partially obstructed vessels to reach the heart and brain.
There are many medical conditions that may need donor blood. However, blood substitutes are only good for shorter term use in more acute conditions.
If condition does not allow for rbc to be stored in the frig, lyophilised nanobiotherapeutic can be stored at room temperature for 1 year [Citation65].
Thus, in addition to its therapeutic value, these nanobiotherapeutics can also help to avoid the lack of donor blood supply during COVID-19 pandemics [Citation45,Citation61].
Highlights and discussions: COVID-19 and artificial cells
Artificial cells have resulted in successful carriers for mRNA vaccines but with needs for improvements for longer-term effect. In severe COVID-19, increase in cytokines, can damage organs and tissues leading to death. Our collodion membrane-activated charcoal adsorbent hemoperfusion can effectively remove middle molecular weight range molecules in patients. Recent commercial hemoperfusion of novel macroporous resin adsorbent with collodion membrane can effectively remove cytokines in patients and in COVID-19 patients. It has been approved for emergency use for severe COVID-19 patients. A recent nanobiotherapeutic containing haemoglobin plus up to six times the concentration of red blood cell enzymes: catalase, superoxide dismutase and carbonic anhydrase. In an animal study, this can effectively lower the damaging increase in free radicals and increase the removal of increase tissue CO2. This can also help in the first ever critical donor blood supply crisis caused by the pandemic.
Ethical approval
This is a review article, thus for all references related to the source of research funds and ethical permissions in animal or human research, please refer to the original articles cited.
Author contributions
The author TMS Chang is the sole person responsible for the conception and design, analysis, and interpretation of the data; the drafting of the paper, revising it critically for intellectual content; and the final approval of the version to be published; he agrees to be accountable for all aspects of the work.
Disclosure statement
No potential conflict of interest was reported by the author(s).
Data availability statement
This is a review paper and does not contain original research data. The information for this review is obtained from published papers from the author and other authors with references to each. The author’s website contains books and papers by him available at no cost to all: www.artcell.mcgill.ca.
References
- Zhou F, Yu T, Du R, et al. Clinical course and risk factors for mortality of adult inpatients with COVID-19 in Wuhan, China: a retrospective cohort study. Lancet. 2020;395(10229):1054–1062.
- Chang TMS. Artificial cell evolves into nanomedicine, biotherapeutics, blood substitutes, drug delivery, enzyme/gene therapy, cancer therapy, cell/stem cell therapy, nanoparticles, liposomes, bioencapsulation, replicating synthetic cells, cell encapsulation/scaffold, biosorbent/immunosorbent haemoperfusion/plasmapheresis, regenerative medicine, encapsulated microbe, nanobiotechnology, nanotechnology, artificial cells. Nanomed Biotechnol. 2019;47(1):997–1013.
- Chang TMS. Semipermeable microcapsules. Science. 1964;146(3643):524–525.
- Chang TMS. Artificial cells. Springfield (IL): Charles C. Thomas; 1972. Out of print but available for free online viewing/download at www.artcell.mcgill.ca/1972bookcovercr.pdf.
- Chang TMS. Therapeutic applications of polymeric artificial cells. Nat Rev Drug Discov. 2005;4(3):221–235.
- Torchilin VP. Recent advances with liposomes as pharmaceutical carriers. Nat Rev Drug Discov. 2005;4(2):145–160.
- Chang TMS. Monograph on “Artificial cells: biotechnology, nanotechnology, blood substitutes, regenerative medicine, bioencapsulation, cell/stem cell therapy”. Singapore: World Scientific Publisher/Imperial College Press; 2007. p. 435. Available for free online viewing at https://www.medicine.mcgill.ca/artcell/2007%20ebook%20artcell%20web.pdf
- Editorial (2018): focus on the benefits of building life’s systems from scratch. Nature. 2018;563:155. doi:10.1038/d41586-018-07285-1.
- Mitchell MJ, Billingsley MM, Haley RM, et al. Engineering precision nanoparticles for drug delivery. Nat Rev Drug Discov. 2021;20(2):101–124.
- Kulkarni JA, Tam YYC, Chen S, et al. Rapid synthesis of lipid nanoparticles containing hydrophobic inorganic nanoparticles. Nanoscale. 2017;9(36):13600–13609.
- Dhiman N, Awasthi R, Sharma B, et al. Lipid nanoparticles as carriers for bioactive delivery. Front Chem. 2021;9:580118.
- Chang TMS. The one-shot vaccine. In: Heden C-G, editor. Socio-economic and ethical implications of enzyme engineering. Stockholm (Sweden): International Federation of Institutes for Advanced Studies; 1975. p. 17–18.
- Chang TMS. Biodegradable semipermeable microcapsules containing enzymes, hormones, vaccines, and other biologicals. J Bioeng. 1976;1:25–32.
- Holtkamp S, Kreiter S, Selmi A, et al. Modification of antigen-encoding RNA increases stability, translational efficacy, and T-cell stimulatory capacity of dendritic cells. Blood. 2006;108(13):4009–4017.
- Abuchowski A, Kazo GM, Verhoest CR, Jr., et al. Cancer therapy with chemically modified enzymes. I. Antitumor properties of polyethylene glycol-asparaginase conjugates. Cancer Biochem Biophys. 1984;7(2):175–186.
- Mueller P, Rudin D. Resting and action potential in experimental bilayer lipid membranes. J Theor. Biol. 1968;18(2):222–258.
- Bangham AD, Standish MM, Watkins JC, et al. Diffusion of univalent ions across the lamellae of swollen phospholipids. J Mol Biol. 1965;13(1):238–252.
- Gregoriadis G, editor. Drug carriers in biology and medicine. New York: Academic Press, Inc.; 1976.
- Deamer DW, Bangham AD. Large-volume liposomes by an ether vaporization method. Biochim Biophys Acta. 1976;443(3):629–634.
- Kowalski PS, Rudra A, Miao L, et al. Delivering the messenger: advances in technologies for therapeutic mRNA delivery. Mol Ther. 2019;27(4):710–728.
- Hajj KA, Whitehead KA. Tools for translation: non-viral materials for therapeutic mRNA delivery. Nat. Rev. Mater. 2017;2:17056.
- Sahin U, Karikó K, Türeci Ö. mRNA-based therapeutics–developing a new class of drugs. Nat Rev Drug Discov. 2014;13(10):759–780.
- Ronco C, Reis T, De Rosa S. Coronavirus epidemic and extracorporeal therapies in intensive care: si vis pacem para bellum. Blood Purif. 2020;49(3):255–258.
- Chang TMS. Semipermeable aqueous microcapsules (“artificial cells”): with emphasis on experiments in an extracorporeal shunt system. Trans Am Soc Artif Intern Organs. 1966;12:13–19.
- Chang TM, Coffey JF, Barré P, et al. Microcapsule artificial kidney: treatment of patients with acute drug intoxication. Can Med Assoc J. 1973;108(4):429–433.,.
- Chang TMS. Microencapsulated adsorbent hemoperfusion for uremia, intoxication and hepatic failure. Kidney Int. 1975;7:S387–S392.
- Chang TMS, Endo Y, Nicolaev VG, et al., editors. Hemoperfusion and plasma-perfusion and other clinical uses of general, biospecific, immune and leucocyte adsorbents. Regenerative medicine, artificial cells and nanomedicine. Vol. 4. Singapore: World Scientific Publisher/Imperial College Press; 2017. p. 1004.
- Chang TMS. First design and clinical use in patients of surface modified sorbent hemoperfusion based on artificial cells for poisoning, kidney failure, liver failure and immunology. Chang TMS, Endo Y, Nicolaev VG, et al., editors. Hemoperfusion and plasma- perfusion and other clinical uses of general, biospecific, immune and leucocyte adsorbents. Regenerative medicine, artificial cells and nanomedicine. Vol. 4. Singapore: World Scientific Publisher/Imperial College Press; 2017. p. 3–77. www.medicine.mcgill.ca/artcell/HPBk_Ch1.pdf
- Wang YJ, Yu YT. Development of resin adsorbents for blood purification at Nankai University in China. Artif Cell Blood Sub. 2011;39(2):92–97.
- Chen J, Han W, Su R, et al. Non-ionic macroporous polystyrene adsorbents for removal of serum toxins in liver failure by hemoperfusion. Artif Cells Nanomed Biotechnol. 2017;45(1):174–183.
- Chavez JR, Danguilan RA, Arakama MI, et al. A case of leptospirosis with acute respiratory failure and acute kidney injury treated with simultaneous extracorporeal membrane oxygenation and haemoperfusion. BMJ Case Rep. 2019;12(5):e229582–6.
- Arslan B, Kucukbingoz C, Kutuk M, et al. Single-center experience with resin adsorption hemoperfusion combined with continuous veno-venous hemofiltration for septic shock patients. Med Sci. 2019;8(2):390–394.
- Du Y, Qi Z, Ma J, et al. Case report: a patient with COVID-19 who benefited from hemoadsorption. Front Med. 2020;7:607849.
- Wang Q, Hu Z. Successful recovery of severe COVID-19 with cytokine stormtreating with extracorporeal blood purification. Int J Infect Dis. 2020;96:618–620.
- Xu X, Jia C, Luo S, et al. Effect of HA330 resin-directed hemoadsorption on a porcine acute respiratory distress syndrome model ann. Ann Intensive Care. 2017;7(1):84–87.
- Wang Y-T, Fu J-J, Li X-L, et al. Effects of hemodialysis and hemoperfusion on inflammatory factors and nuclear transcription factors in peripheral blood cell of multiple organ dysfunction syndrome. Eur Rev Med Pharm Sci. 2016;20:745–750.
- Huang Z, Wang SR, Yang ZL, et al. Effect on extrapulmonary sepsis-induced acute lung injury by hemoperfusion with neutral microporous resin column. Ther Apher Dial. 2013;17(4):454–461.
- Dastan F, Saffaei A, Mortazavi SM, et al. Continues renal replacement therapy (CRRT) with disposable hemoperfusion cartridge: a promising option for severe COVID-19. J Glob Antimicrob Resist. 2020;21:340–341.
- Liang Y, et al. Extracorporeal blood purification therapy using hemoadsorption type hemoperfusion improves ICU outcomes in critically ill patients with SAR-CoV-2 infection – a prospective cohort study. Lancet. 2020.
- Wu J. Tackle the free radicals damage in COVID-19. Nitric Oxide. 2020;102:39–41.
- Shadyro O, Samovich S, Edimecheva I, et al. Potential role of free-radical processes in biomolecules damage during COVID-19 and ways of their regulation. Free Radical Res. 2021;55(7):665–676.
- Wenzhong L, Hualan L. COVID-19: captures iron and generates reactive oxygen species to damage the human immune system. Autoimmunity. 2021;54(4):213–224.
- Sims C, Seigne P, Menconi M, et al. Skeletal muscle acidosis correlates with the severity of blood volume loss during shock and resuscitation. J Trauma. 2001;51:1137–1146.
- Tronstad C, Pischke SE, Holhjem L, et al. Early detection of cardiac ischemia using a conductometric PCO2 sensor: real-time drift correction and parameterization. Physiol Meas. 2010;31(9):1241–1255.
- Red Cross declares first-ever blood crisis amid omicron surge. https://www.redcross.org/about-us/news-and-events/press-release/2022/blood-donors-needed-now-as-omicron-intensifies.html.
- Bian Y, Chang TMS. A novel nanobiotherapeutical poly-[hemoglobin- superoxide dismutase-catalase-carbonic anhydrase] with no cardiac toxicity for the resuscitation of a 90 minutes sustained severe hemorrhagic shock rat model with 2/3 blood volume loss artificial cells. Nanomedicine and Biotechnology. 2015;43(1):1–9.
- Guo C, Chang TMS. Long term safety and immunological effects of a nanobiotherapeutic, bovine poly-[hemoglobin-catalase-superoxide dismutase- carbonic anhydrase], after four weekly 5% blood volume top-loading followed by a challenge of 30% exchange transfusion. Artif Cells Nanomed Biotechnol. 2018;46(7):1349–1363.
- Chang TMS. Translational feasibility of soluble nanobiotherapeutics with enhanced red blood cell functions. Artif Cells Nanomed Biotechnol. 2017;45(4):671–676.
- Chang TMS. Stabilisation of enzymes by microencapsulation with a concentrated protein solution or by microencapsulation followed by cross- linking with glutaraldehyde. Biochem Biophys Res Commun. 1971;44(6):1531–1536.
- Jahr JS. Hemoglobin-glutamer 250 (bovine) [HBOC-201, Hemopure®] clinical use in South Africa and comprehensive review of cardiac outcomes and risk/benefit in Peer-Reviewed, indexed studies in humans and animal models. In: Chang TMS, Bulow L, Jahr JS, et al., editors. Nanobiotherapeutic based blood substitutes. Singapore: World Science Publisher; 2021.
- Moore E, Moore FA, Fabian TC, et al. Human polymerized hemoglobin for the treatment of hemorrhagic shock when blood is unavailable: the USA multicenter trial. J Am Coll Surg. 2009;208(1):1–13.
- D'Agnillo F, Chang TM. Polyhemoglobin-superoxide dismutasecatalase as a blood substitute with antioxidant properties. Nat Biotechnol. 1998;16(7):667–671.
- Powanda D, C, TMS. Cross-linked polyhemoglobin- superoxide dismutase-catalase supplies oxygen without causing blood brain barrier disruption or brain edema in a rat model of transient global brain ischemia-reperfusion. Artif Cells Blood Substit Immobil Biotechnol. 2002;30:25–42.
- Razack S, D'Agnillo F, Chang TM. Crosslinked hemoglobin-superoxide dismutase-catalase scavenges free radicals in a rat model of intestinal ischemia-reperfusion injury. Artif Cells Blood Substit Immobil Biotechnol. 1997;25(1–2):181–192.
- Ma L, Hsia CJC. Polynitroxylated hemoglobin as a multifunctional therapeutic for critical care and transfusion medicine. In: Chang TMS, editor. Selected topics in nanomedicine. Singapore: World Science Publisher/Imperial College Press; 2013.
- Simoni J, Simoni G, Lox CD, et al. Modified hemoglobin solution with desired pharmacological properties does not activate nuclear transcription factor NF-kappa B in human vascular endothelial cells. Artif Cells Blood Substit Immobil Biotechnol. 1997;25(1–2):193–210.
- Zal F. 2017. Use of HEMO2life – an innovative oxygen carrier in organ transplantation abstract. 2017 International Symposium on Blood Substitutes. www.artcell.mcgill.ca
- Jia YP, Alayash AI. Molecular basis of haptoglobin and hemoglobin complex formation and protection against oxidative stress and damage. In: Chang TMS, editor. Selected topics in nanomedicine. Singapore: World Science Publisher/Imperial College Press; 2013.
- Kettisen K, Bülow L. Cysteine mutations in recombinant fetal hemoglobin influence oxidative side-reactions. In: Chang TMS, Bulow L, Jahr JS, et al., editors. Nanobiotherapeutic based blood substitutes. Singapore: World Science Publisher; 2021.
- Chan G, Chang TMS. Dual effects include antioxidant and pro- oxidation of ascorbic acid on the redox properties of bovine hemoglobin. In: Chang TMS, Bulow L, Jahr JS, et al., editors. Nanobiotherapeutic based blood substitutes. Singapore: World Science Publisher; 2021.
- Liu JX, Yang CM. Development and future of Chinese transfusion medicine and blood substitutes. In: Chang TMS, Bulow L, Jahr JS, et al., editors. Nanobiotherapeutic based blood substitutes. Singapore: World Science Publisher; 2021. p. 161–168.
- Guo C, Gynn M, Chang TMS. Extraction of superoxide dismutase, catalase and carbonic anhydrase from stroma-free red blood cell hemolysate for the preparation of the nanobiotechnological complex of polyhemoglobin-superoxide dismutase-catalase-carbonic anhydrase. Artif Cells Nanomed Biotechnol. 2015;43(3):157–62162.
- Chang TMS, BulowL, JahrJS, et al., editors. Nanobiotherapeutic based blood substitutes. Singapore: World Science Publisher; 2021. p. 1140.
- Chang TMS. 2022. Artificial cells, blood substitutes and nanomedicine website containing reviews and monographs for free online viewing or download www.artcell.mcgill.ca.
- Bian Y, Guo C, Chang TMS. Temperature stability of poly-[hemoglobin-superoxide dismutase–catalase-carbonic anhydrase] in the form of a solution or in the lyophilized form during storage at -80 °C, 4 °C, 25 °C and 37 °C or pasteurization at 70 °C. Artif Cells Nanomed Biotechnol. 2016;44(1):41–47.