Background
Diabetes mellitus is a serious, chronic disorder that significantly harms people’s lives, families, and societies everywhere. The three primary types of diabetes are type 1 diabetes mellitus (T1DM), type 2 diabetes mellitus (T2DM), and gestational diabetes mellitus (GDM). The International Diabetes Federation (IDF) has been documenting the prevalence of diabetes globally, regionally, and nationally since 2000. Despite the sustainable development goal (SDG) of reducing premature death from non-communicable diseases (NCDs) by one-third by 2030, the prevalence of diabetes mellitus is expected to be 700 million by 2045 [Citation1,Citation2], and although there are numerous antidiabetic agents, many of them have unfavorable side effects and known consequences that impair quality of life. Hence, there is a need to develop new therapeutic agents with lesser complications(these lines written by author).
The creation of novel medicines to improve glycemic control and diabetes management is a great opportunity to target sodium-dependent glucose transport (SGLT) proteins. SGLT1 and SGLT2 are two different isoforms of SGLT that serve to distinguish both of them [Citation3,Citation4]. In kidneys, SGLT plays an important role in glucose homeostasis by filtering and reabsorbing glucose from nearby tissues. Every day, the kidneys filter about 180 g of glucose [Citation5]. Since SGLT1 is a high-affinity transporter, low volume that is situated in the intestine and kidney and secretes about 10% of glucose from the distal tubule. Moreover, SGLT2 is a low-affinity transporter, high-volume that is situated in the proximal convoluted tubule(PCT) and secretes about 90% of reabsorbing glucose filtered by the kidney [Citation6,Citation7].
Glucose Renal Reabsorption process in Kidney
Under normal physiological conditions, SGLT2ʹs primary function is to reabsorb glucose that has been filtered by the renal glomeruli. Sodium-glucose cotransporter 2 (SGLT2) absorbs roughly 90% of the kidney’s glucose and reabsorbed glucose transferred to plasma through GLUT-2 while sodium-glucose cotransporter 1(SGLT1), absorbs a remaining portion of glucose and then transferred to plasma by GLUT-1()
Figure 1. Describes the Glucose renal reabsorption process and the mechanism of SGLT2 inhibitors (The Figures has been created by the author).
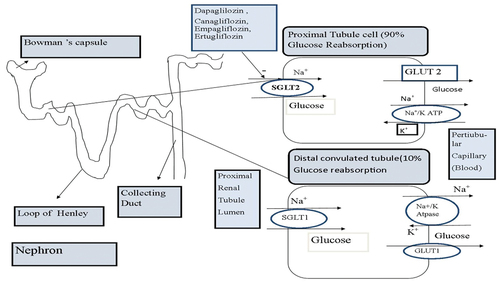
In GIT(gastrointestinal tract), SGLT1 is a vital transporter for glucose uptake but the effect in kidneys is less significant whereas SGLT2 serves as important transporter (reabsorbs 90% glucose) in kidneys which causes hyperglycemia in diabetes mellitus. Thus, this transporter (SGLT2) has gained interest in the treatment for the T2DM. Especially in hypoglycemic patients SGLT2 inhibitors oppose the effects of SGLT2 transporter, thereby increasing glucosuria and decreasing serum glucose levels. SGLT2 inhibitors have been demonstrated to improve CVS outcomes and provided much-needed advantages for type −2 diabetes mellitus. SGLT2 inhibition is therefore considered a strategy for the treatment of diabetes mellitus [Citation8]. This review encapsulates the first plant-derived SGLT inhibitory compound, Phlorizin, and a description of phlorizin-derived synthetic SGLT2 inhibitors which are commercially available and it also summarizes active constituents with SGLT2 inhibitory effects from natural plants.
Phlorizin Natural SGLT inhibitor
Phlorizin, an O-glucoside dihydrochalcone molecule, was initially isolated from apple tree bark discovered in 1835 and has been found to improve healthy individuals’ excretion of glucose in urine [Citation9]. Phlorizin was discovered to have the potential to block both SGLT1 and SGLT2 sodium-glucose cotransporters, however, it has not been developed as an antihyperglycemic medication for usage in humans due to its poor bioavailability and GIT side effects like diarrhea, dehydration, and malabsorption by inhibiting SGLT1(in the small intestine) [Citation10]. Keeping in view the adverse effects, phlorizin was deemed unsuitable for continued research as an antihyperglycemic drug. Phlorizin derivates with more focused SGLT2 inhibition (), were created to prevent SGLT1-dependent side effects [Citation8]
Table 1. List of Synthetic drugs investigated for Antidiabetic action through SGLT2 inhibition.
Synthetic SGLT inhibitors
Phlorizin-derived synthetic SGLT-2 inhibitor- O-glucoside analogs
In the early stage, O-glucoside analogs like T-1095 were derived from phlorizin which is a prodrug that showed antidiabetic activity [Citation11]. T-1095 inhibits the activity of SGLT1 and SGLT2 in the kidney however, it showed some poor metabolic stability in preclinical trials and was abandoned [Citation9]. Other O-glucoside derivatives sergliflozin and remogliflozin are the prodrugs that were evaluated in various pharmacokinetic and pharmacodynamics parameters. Sergliflozin was discontinued/due to a lack of sufficient stability in the gut and possesses poor metabolic properties [Citation12]. Recently, sergliflozin A derivates were synthesized and the 4-o-methyl derivative of sergliflozin A was found to have enhanced pharmacokinetic stability and therapeutic potency when compared with sergliflozin [Citation13].
Remogliflozin etabonate (RE)
RE- a prodrug that is a selective SGLT2 inhibitor recently got global approval on 30 April 2019 as a drug for the treatment of T2DM in India. It improves glucose control by improving urinary glucose excretion (UGE). RE was studied over a while to assess safety, tolerance, pharmacokinetics, and pharmacodynamics [Citation14]. Patients with type 2 diabetes mellitus were associated in the phase 1 research clinical trials with a dose-dependent increase in urine glucose excretion from 0 to 24 hours after receiving RE (50–500 mg) in healthy volunteers. In comparison to healthy volunteers, urine glucose excretion was found to be higher in T2DM [Citation15].
In the phase 2 study, efficacy was studied for T2DM, and a modest drop in Hb1Ac and decreases in body weight and fasting plasma glucose levels were observed. During the study phase III double-blind research clinical trials, the efficacy of remagliflozin was studied with dapagliflozin which results in reductions in Hb1Ac, body weight, diastolic blood pressure (DBP), and systolic blood pressure (SBP) was observed.
In phase 3 trials, urinary tract infections, headaches, constipation, ketonuria, diarrhea, and dyslipidemia were the most frequently reported side effects and hypoglycemia was seen in participants receiving metformin in proportional amounts [Citation15].To treat T2DM patients, RE can be given in conjunction with metformin which is safe, tolerable, and effective [Citation16].
Phlorizin-derived synthetic SGLT-2 inhibitor- C-glucoside analogs
Dapagliflozin
Dapagliflozin, a highly potent and specific SGLT2 inhibitor, was approved by the FDA in 2014 and is used to treat T2DM throughout the world, including in the EU and the US. It is 1400 times more selective for SGLT2 than SGLT1. Recent studies have shown that dapagliflozin induces osmotic diuresis, reduces blood pressure and body weight without increasing heart rate, and therefore it may have a valuable effect on heart failure patients [Citation17]. As an addition to insulin, dapagliflozin demonstrated good safety and tolerability and resulted in dose-related increases in urine glucose excretion, decreases in 24-hour mean glucose, and declines in glucose variability [Citation18]. Higher research is required to determine the effectiveness, safety, and efficacy of dapagliflozin, which exhibited side effects like hypotension, dehydration, vaginal infections, and renal impairment [Citation19].
The majority of the drug’s metabolism via uridine disphosphoglucuronosytransferase UGT1A9 results in the inactive metabolite 3-O-glucuronide. Neither the primary nor other metabolites of dapagliflozin are involved in the drug’s effects on blood sugar levels. Approximately 75% of a dosage of dapagliflozin and its metabolites are recovered in the urine and 21% in the feces [Citation20].
In addition to being used as monotherapy, dapagliflozin is also effective in patients who cannot tolerate metformin or as a replacement for metformin, when metformin is contraindicated. Dapagliflozin lowers HbA1c levels as effectively as metformin when used alone. However, in patients with poor glycemic control, it can be used to supplement other antihyperglycemic drugs [Citation19]. However, this drug is safe, well-tolerated, and effective for type 1 and 2 diabetes.
Canagliflozin
Canagliflozin is the first SGLT2 inhibitor approved by the FDA in 2013 for the treatment of T2DM. There are more than 80 countries that use canagliflozin for the treatment of type 2 diabetes, including Europe, The United States, Japan, and Australia. Compared to SGLT2, it is around 158 times more selective than SGLT1. By increasing urine excretion of glucose and decreasing renal reabsorption of glucose, the inhibition of SGLT2 lowers blood glucose levels without affecting insulin levels. Adults with T2DM who were older or at higher cardiovascular (CV) risk had better glycemic control because of canagliflozin, and their body weight and blood pressure also improved. It can be used as a first-line monotherapy as well given in combination with metformin and it reduces Hb1Ac, body weight, and fasting plasma glucose levels [Citation21].
Canagliflozin is largely metabolized through O glucuronidation [by the enzymes uridine 50-diphospho-glucuronosyltransferase (UGT) 2B4 and 1A9], which results in the formation of two major inactive metabolites. When taken as directed, canagliflozin has renal clearance rates of 1.30–1.55 mL/min and is excreted in the urine and feces [Citation22].
Empagliflozin
For the treatment of individuals with type 2 diabetes mellitus, Empagliflozin, a subclass of Gliflozin (FDA approved in 2014), is extremely successful and well-tolerated in many nations, including the EU, the USA, Japan, and others. It exerts higher selectivity for SGLT2 (>2500-fold) over SGLT1 compared to other SGLT2 inhibitors [Citation23,Citation24]. The medication lowers the kidneys’ glucose threshold and limits the reabsorption of filtered glucose because it inhibits SGLT2, which raises urinary glucose excretion and decreases blood glucose levels, and also suppressed SGLT2 in a dose-dependent manner. Moreover, in the clinical trials, participants tolerated empagliflozin well, with most adverse events being mild or moderate in severity. Empagliflozin is mostly metabolized in humans by glucuronidation by 50-uridine diphosphoglucuronosyltransferases (UGTs) 1A3, 1A8, 1A9, and 2B7 and about 54% was eliminated as an unaltered drug in the urine [Citation25].
As monotherapy or in conjugation with additional antidiabetic medications, empagliflozin is often well tolerated. When metformin and empagliflozin are combined, the glycemic control in T2DM is improved, and blood pressure and body weight are reduced when taken at doses of 10 or 25 mg. This combination therapy is effective and well-tolerated in T2DM patients. In another study, patients with T2DM who received empagliflozin as an addition to metformin and a sulfonylurea experienced reductions in body weight and Hb1Ac levels [Citation26,Citation27].
Ertugliflozin
Ertugliflozin, a novel family of selective SGLT2 inhibitors newly approved by the FDA for the treatment of T2DM in 2017. Similar to other SGLT2 inhibitors, ertugliflozin very selectively inhibits SGLT2 to decrease renal glucose sensitivity and filtered glucose reabsorption in the kidney, increasing urine glucose excretion. Ertugliflozin does not affect insulin secretion or sensitivity, unlike other SGLT2 inhibitors. Additionally, it can be taken in combination with some medications, such as metformin, sitagliptin, and sulfonylureas, in addition to insulin [Citation28].
Ertugliflozin is converted to two pharmacologically inactive glucuronides through O-glucuronidation, which is catalyzed by the enzymes uridine diphosphate glucuronosyltransferase (UGT) 1A9 and UGT2B7. Metabolic activity mediated by cytochrome P450 is modest [Citation28]. Although, ertugliflozin and desethyl ertugliflozin glucuronides made up 43.9% of the dose that was delivered in the urine. Ertugliflozin’s oxidative metabolites made up 4.1% and 1.1% of the dosage in feces and urine, respectively [Citation29].
Ertugliflozin has been used as an add-on therapy to improve therapeutic outcomes for antidiabetic agents. It involves some fixed-dose combinations such as ertugliflozin and sitagliptin, ertugliflozin and metformin have been recently approved by the USFDA to control glycemic levels [Citation30].
Ipragliflozin
Ipragliflozin is a new, selective SGLT2 inhibitor with a C-glucoside structure approved in Japan (2014) and is co-produced by Astellas Pharma Inc. and Kotobuki Pharmaceutical Companies in Japan. Later on, it was approved by Russia and South Korea [Citation31]. Ipragliflozin reduces body weight, enhances glycemic management, and lessens the risk of hypoglycemia and stomach discomfort. Since SGLT2 inhibitors are insulin-independent, they are believed to have an advantage in providing steady glucose control without the likelihood of hypoglycemia [Citation32].
Ipragliflozin is metabolized mostly via glucuronidation to inactive metabolites, with minor contributions from the UGT2B4 and UGT1A9 isoforms and a significant contribution from the UGT2B7 isoform. Ipragliflozin can be found in stools, and only 1–2% of an oral dosage is unchanged and eliminated in urine [Citation31].
Ipragliflozin can be used alone or in conjugation with other hypoglycemic drugs or diabetes medications such as metformin, sitagliptin, pioglitazone, glimepiride, miglitol, and mitiglinide to lower blood sugar levels [Citation33,Citation34].
Tofogliflozin
Tofogliflozin, a member of the Gliflozin class, was produced by Chugai Pharma in partnership with Kowa and Sanofi and was given its first approval in Japan in 2014. It is a highly selective SGLT2 inhibitor and demonstrated a 3000-fold increase in SGLT-2 selectivity over SGLT1 [Citation35]. Tofogliflozin lowers blood sugar levels, by preventing the kidney from reabsorbing glucose and generating an increase in the excretion of extra blood sugar in the urine and include some benefits in terms of systolic and diastolic blood pressure, body weight and fat mass, and overall health [Citation36].
Tofogliflozin and its main phenylacetic acid metabolite M1 made up 42 and 52% of the total amount of drug-related material in circulation, respectively. M1 was also the main substance eliminated in urine and feces and was estimated to make up more than half of the dose [Citation36]. Tofogliflozin can be given in combination with other antidiabetic drugs, such as dipeptidylpeptidase-4 inhibitors, metformin, and sulfonylureas [Citation35]. Overall, tofogliflozin is proving to be an extremely effective oral hypoglycemic medication.
Luseogliflozin
Taisho Pharmaceutical Co., Ltd. created luseogliflozin, a selective SGLT2 inhibitor, which received approval in Japan for the treatment of T2DM. Luseogliflozin significantly decreased body weight and improved glycemic control in preapproval studies in Japanese patients with T2DM. It was also linked with minor side effects [Citation37,Citation38]. Luseogliflozin is an oral anti-diabetic drug(OADs) that can be used alone or in conjunction with sulfonylurea, metformin, pioglitazone, and dpp4 inhibitors, but it is likely to cause hypoglycemia and decrease in Hb1Ac and body weight [Citation39].
Several liver enzymes, including cytochrome P450 (CYP) isoforms: CYP3A4, CYP3A5, and UDP-glucuronosyltransferase 1A1, efficiently metabolize luseogliflozin through three pathways, two of which involve oxidation and one of which involves glucuronate conjugation (UGT1A1). In conclusion, when taken properly, luseogliflozin is safe and well tolerated and significantly reduces hyperglycemia, and can help T2DM patients all over the world [Citation40].
Sotagliflozin
Sotagliflozin was approved in the EU on 26 April 2019 for use as a supplement to insulin in people with T1DM. It is an SGLT1 and SGLT2 inhibitor developed for the treatment of T1DM and T2DM. In comparison to SGLT1, sotagliflozin has a 20-fold better SGLT2 selectivity. Dual SGLT inhibitor sotaglifozin binds to and blocks both SGLT1 and SGLT 2. This slows down and delays, respectively, the absorption of glucose from the digestive tract and the proximal tubule of the kidney [Citation41]. There are serious concerns regarding the prospective use of sotagliflozin in the T1DM population due to the higher prevalence of diabetic ketoacidosis (DKA) and the unknown dangers for women experiencing conception and pregnancy [Citation42]. However, it was developed by Lexicon Pharmaceuticals and Sanof but in contrast, the FDA declined to approve sotagliflozin for usage in T1DM patients although the drug’s sponsor has submitted New Drug Applications(NDA) in the US [Citation43]. Moreover , some chemical strutures of different phlorizin-derived synthetic SGLT-2 inhibitors as antidiabetic as T2DM are shown in .
Novel SGLT2 inhibitors from natural product
Although many synthetic SGLT2 inhibitors are now available and used for the treatment of T2DM, several efforts are made to find new SGLT2 inhibitors from natural products. A few active compounds from natural plants SGLT2 inhibitors activity like Sophora Flavescens, Schinsandrae Chinesis Fructus, Gnetum Gnemonoides, Acer Nikoense, and Alstonia Macrophylla are further discussed ().
Table 2. List of Natural active Constituents investigated for antidiabetic action through SGLT2 inhibition.
Sophora Flavescens
There are 52 species, 19 variants, and 7 forms of the traditional Chinese medicine plant known as Sophora Flavescens, which can be found throughout Asia and the Pacific Islands [Citation59]. Two thousand years ago, Sophora Flavescens was listed as the first Chinese classic medicinal plant and has a bitter, naturally cold taste. It is sometimes referred to as ‘Kushen’, and it has been used for thousands of years to cure a variety of illnesses including fevers, diarrhea, dermatitis, jaundice, and vaginal itching with leukorrhagia, gastrointestinal hemorrhage, inflammatory disorders, ulcers, and skin burns [Citation60].
The traditional Chinese medicinal S. flavescens methanol extract had strong SGLT inhibitory activity with respect to kurarinone, sophoraflavanone, and seven other compounds were extracted for more research into their SGLT inhibition properties. Interestingly, only kurarinone and sophoraflavanone inhibit both SGLT1 and SGLT2, while three other substances – maackiain, variabilin, and formononetin – that include hydroxyl groups in their structures also have inhibitory effects on SGLT2 [Citation61].
Acer Nikoense
Acer Nikoense (A. Nikoense) is a traditional plant that is indigenous to Japan. It’s traditionally used to treat hepatic disorders and eye diseases with its stem bark extracts, which are Japanese folk medicines [Citation62]. A. nikoense contains a variety of phenolic compounds that have biological properties including anti-inflammatory, antioxidant, hepatoprotective, nitric oxide (NO) generation inhibitory, and sodium-glucose cotransporter (SGLT) inhibitory actions [Citation63]. Although it contains the series of Acerogenins A and B, two cyclic diarylheptanoids, had a strong inhibitory effect on SGLT1, but only a moderate effect on SGLT2. The chemical structures of some components, such as aceroside I and VII, contain glucose, which prevents their ability to inhibit SGLT1 and SGLT2 activity. Additionally, whereas ketone compounds showed inhibitory activity against SGLT2, acetyl and methyl ether acerogenin derivatives had a modest inhibitory effect on SGLT1 [Citation64]
Alstonia Macorphylla
Alstonia Macrophylla is a traditional plant that grows widely in the Southeast Asia region, India, Indonesia, Thailand, and Vietnam. It is commonly known as Big-leaved macrophyllum, Hard common Alstonia, or Hard milkwood [Citation65]. It is used in traditional medicine as a common tonic, antifever, emmenagogue, aphrodisiac, antidysentery, anticholinergic, and vulnerary agents [Citation66]. A. macrophylla leaves were used to isolate twenty alkaloid compounds, and their ability to inhibit SGLT was evaluated. Five picraline-type alkaloids out of twenty compounds exhibited effective SGLT1 and SGLT2 inhibition. Other alkaloid compounds of the ajmaline and macroline types, however, exhibited no inhibitory effects on SGLT1 and/or SGLT2 [Citation67].
Gnetum Gnemonoides
The widespread growth of a Gnetum gnemonoides species can be found across Southeast Asia and the Pacific, including New Guinea, Malaysia, the Philippines, and Indonesia. It is reported to contain stilbenoids, which exhibit biological qualities as antibacterial, antioxidant, hepatoprotective, and enzyme inhibitory action. Bronchitis, asthma, and arthritis have all been treated with this species as a kind of traditional medicine [Citation68]. Gnetum gnemonoides include several stilbenoids that have been isolated, resveratrol, oxyresveratrol, piceatannol, and isorhapontigenin have been shown SGLT inhibitory activity. Gneyulins A and B, two stilbene trimmers made up of oxyresveratrol constituent units, demonstrated modest inhibitory activity against SGLT1 and SGLT2, whereas Noidesols A and B, two dihydroflavonol-C-glucosides, had no SGLT inhibitory potential [Citation12].
Schinsandrae Chinesis Fructus (SCF)
The typical dry ripe fruit of Schisandra Chinensis (Turcz.) Baill, also known as the ‘Five Taste Fruit’, is known as Schisandra Chinensis Fructus (SCF). It is commonly called Five Flavor berry. The name of S. Chinensis tells us a great deal about the qualities of this herb. It is a herbal plant and is widely distributed in the region of northeastern China, Japan, the Russian Far East, and Korea [Citation69]. This traditional Chinese plant is mainly used for the treatment of palpitation and dysphoria, insomnia, and many dreams resulting from the poor preservation of the patient’s spirit [Citation70]. The fruit of Schisandra Chinensis (Turcz.) Baill is said to have anti-diabetic and tonic properties. Deoxyschisandrin, schisandrin B (-schisandrin), and schisandrin were three active constituents found in F8 that had their quantities measured. But the later analysis in the [14C]-AMG absorption assay revealed that none of these three substances were able to reduce SGLT 2 activity and demonstrating the lack of identification of the SGLT active components from SCF [Citation71].
Conclusion
A relative or complete shortage of insulin characterizes diabetes mellitus, a metabolic condition that is dangerous to human health. It also causes several difficulties in various organs. An attractive treatment method for hyperglycemia is to stop renal glucose reabsorption by blocking SGLT2. The new SGLT-2 selective inhibitor medication family promises an innovative therapeutic strategy for blood glucose management in type II diabetes mellitus. Additionally, this new class demonstrates other benefits like a decrease in blood pressure and body weight, reduce hospitalizations for heart failure, and delay in the course of renal disease which is particularly desirable in several linked conditions. SGLT2 inhibitors are considered most appropriate in overweight or obese T2DM patients but they may increase your risk of developing vaginal infections and diabetic ketoacidosis.
Beginning in the early twenty-first century, several SGLT2 inhibitors derived from the naturally occurring active component phlorizin started to be launched and were quickly utilized either alone or in conjunction with other oral hypoglycemic medications but phlorizin was inhibiting SGLT1 more significant thereby adverse effects associated with inhibition of SGLT1 were more prominent which made it inappropriate for the treatment of T2DM. Soon, phlorizin-derived synthetic analogs like O-analogs and C-analogs were available in the market, O-Analogs had some limitations due to which they were discontinued in the clinical trial except Remagliflozin Etabonate which was recently approved and used for the treatment of type 2 diabetes. Though, Mostly C-Analogs are used for the treatment of T2DM. Some mild or moderated adverse events have been reported with C analogs and further studies are needed to investigate the safety and efficacy of the drugs.
Future Perspective of Synthetic Drugs
Several SGLT2 inhibitors are available and extensively used. There are also several additional comparable compounds in development that might receive approval soon like Bexagliflozin and Enavogliflozin. DWP16001 (Envogliflozin) is being developed by Daewoong Pharmaceutical Co. Ltd. (Yongin, Korea), which is a selective SGLT2 inhibitor and is being under phase 3 clinical studies [Citation73] and that study has evaluated the long-term safety and efficacy of DWP16001 as an add-on to metformin in Patients with T2DM patients. While Bexagliflozin is a highly selective SGLT2 inhibitor that can be taken orally and lowers blood sugar and is being studied under phase 3 clinical studies [Citation74]. According to clinical trials, an interventional clinical study has been going on, which will tell about the risk of cardiovascular and effects on Hb1Ac and body weight on T2DM patients. So further there is another drug called Henagliflozin which is in the 3rd phase of clinical trials previous phases’ results of this drug showed a reduction in Hb1Ac and improvements in body weight and blood pressure [Citation75]. In addition, some of the recently approved SGLT2 drugs like empagliflozin, canagliflozin, and tofogliflozin are still under interventional phases and some are under adjunct therapy for the treatment of T2DM.
Acknowledgments
The authors are thankful to the institute for providing facilities such as a library, journals, and internet sources for writing the article.
Disclosure statement
No potential conflict of interest was reported by the author(s).
Additional information
Funding
References
- Bommer C, Sagalova V, Heesemann E, et al. Global Economic Burden of Diabetes in Adults: projections From 2015 to 2030. Diabetes Care. 2018;41(5):963–970.
- Saeedi P, Petersohn I, Salpea P, et al. ‘Global and regional diabetes prevalence estimates for 2019 and projections for 2030 and 2045: results from the International Diabetes Federation Diabetes Atlas. Diabetes Res Clin Pract. 2019; 157:107843.
- Castaneda-Sceppa C, Castaneda F. Sodium-dependent glucose transporter protein as a potential therapeutic target for improving glycemic control in diabetes. Nutr Rev. 2011;69(12):720–729.
- Tsushima Y, Lansang MC, Makin V. The role of SGLT-2 inhibitors in managing type 2 diabetes. Cleve Clin J Med. 2021;88(1):47–58.
- Fisher M, McKay G. Essentials of SGLT2 Inhibitors in Diabetes. Springer International Publishing Switzerland; 2017. 13–35. Doi:10.1007/978-3-319-43296-0
- Boeder S, Edelman SV. Sodium-glucose co-transporter inhibitors as adjunctive treatment to insulin in type 1 diabetes: a review of randomized controlled trials. Diabetes Obesity Metab. 2019;21(S2):62–77.
- Chao EC, Henry RR. SGLT2 inhibition-A novel strategy for diabetes treatment. Nat Rev Drug Discov. 2010;9(7):551–559.
- Fattah H, Vallon V. The Potential Role of SGLT2 Inhibitors in the Treatment of Type 1 Diabetes Mellitus. Drugs. 2018;78(7):717–726.
- Rieg T, Vallon V. Development of SGLT1 and SGLT2 inhibitors. Diabetologia. 2018;61(10):2079–2086.
- Haider K, Pathak A, Rohilla A, et al. Synthetic strategy and SAR studies of C-glucoside heteroaryls as SGLT2 inhibitor: a review. Eur J Med Chem. 2019;184:111773.
- Oku A, Ueta K, Arakawa K, et al. T-1095, an inhibitor of renal Na+-glucose cotransporters, may provide a novel approach to treating diabetes. Diabetes. 1999;48(9):1794–1800.
- Choi CI. Sodium-Glucose Cotransporter 2 (SGLT2) inhibitors from natural products: discovery of next-generation antihyperglycemic agents. Molecules. 2016;21(9):1–18.
- Moradi-Marjaneh R, Paseban M, Sahebkar A. Natural products with SGLT2 inhibitory activity: possibilities of application for the treatment of diabetes. Phytother Res. 2019;33(10):2518–2530.
- Dobbins RL, O’Connor-Semmes R, Kapur A, et al. Remogliflozin etabonate, a selective inhibitor of the sodium-dependent transporter 2 reduces serum glucose in type 2 diabetes mellitus patients. Diabetes Obesity Metab. 2012;14(1):15–22.
- Markham A. Remogliflozin Etabonate: first Global Approval. Drugs. 2019;79(10):1157–1161.
- Dobbins R, Hussey KE, Connor-Semmes RO, Andrews S, et al. ‘Assessment of safety and tolerability of remogliflozin etabonate (GSK189075) when administered with total daily dose of 2000 mg of metformin’. BMC Pharmacology and Toxicology. 2021; 22(1): 1–11. DOI:10.1186/s40360-021-00502-0
- Kosiborod M, Gause-Nilsson I, Xu J, et al. Efficacy and safety of dapagliflozin in patients with type 2 diabetes and concomitant heart failure. J Diabetes Complications. 2017;31(7):1215–1221.
- Dandona P, Mathieu C, Phillip M, et al. Efficacy and safety of dapagliflozin in patients with inadequately controlled type 1 diabetes (DEPICT-1): 24 week results from a multicentre, double-blind, phase 3, randomised controlled trial. Lancet Diabetes Endocrinol. 2017;5(11):864–876.
- Saleem F. Dapagliflozin: cardiovascular Safety and Benefits in Type 2 Diabetes Mellitus. Cureus. 2017;9:10.
- Dhillon S. Dapagliflozin: a Review in Type 2 Diabetes. Drugs. 2019;79(10):1135–1146.
- Deeks ED, Scheen AJ. Canagliflozin: a Review in Type 2 Diabetes. Drugs. 2017;77(14):1577–1592.
- Minami T, Kameda A, Terauchi Y. An evaluation of canagliflozin for the treatment of type 2 diabetes: an update. Expert Opin Pharmacother. 2021;22(16):2087–2094.
- Frampton JE. Empagliflozin: a Review in Type 2 Diabetes. Drugs. 2018;78(10):1037–1048.
- Wanner C, Lachin JM, Inzucchi SE, et al. Empagliflozin and clinical outcomes in patients with type 2 diabetes mellitus, established cardiovascular disease, and chronic kidney disease. Circulation. 2018;137(2):119–129.
- Scott LJ. Empagliflozin: a review of its use in patients with type 2 diabetes mellitus. Drugs. 2014;74(15):1769–1784.
- Goldman JD. Combination of Empagliflozin and Metformin Therapy: a Consideration of its Place in Type 2 Diabetes Therapy. Clin Med Insights. 2018; 11. doi:10.1177/1179551418786258
- Scheen AJ. Pharmacokinetic and pharmacodynamic profile of empagliflozin, a sodium glucose co-transporter 2 inhibitor. Clin Pharmacokinet. 2014;53(3):213–225.
- Marrs JC, Anderson SL. Ertugliflozin in the treatment of type 2 diabetes mellitus. Drugs Context. 2021;9:1–10.
- Markham A. Ertugliflozin: first Global Approval. Drugs. 2018;78(4):513–519.
- Hu J, Deng A, Zhao Y. Ertugliflozin as a monotherapy for the treatment of type 2 diabetes. Expert Opin Pharmacother. 2018;19(16):1841–1847.
- Alkabbani W, Gamble JM. Profile of ipragliflozin, an oral sglt-2 inhibitor for the treatment of type 2 diabetes: the evidence to date. Drug Des Devel Ther. 2021;15:3057–3069.
- Ohkura T. Ipragliflozin: a novel sodium-glucose cotransporter 2 inhibitor developed in Japan. World J Diabetes. 2015;6(1):136.
- Kadokura T, Zhang W, Krauwinkel W, et al. Clinical Pharmacokinetics and Pharmacodynamics of the Novel SGLT2 Inhibitor Ipragliflozin. Clin Pharmacokinet. 2014;53(11):975–988.
- Koshizaka M, Ishikawa K, Ishibashi R, et al. Effects of ipragliflozin versus metformin in combination with sitagliptin on bone and muscle in Japanese patients with type 2 diabetes mellitus: subanalysis of a prospective, randomized, controlled study (PRIME-V study). J Diabetes Investig. 2021;12(2):200–206.
- Aharon-Hananel G, Raz I. An evaluation of the efficacy and safety of Tofogliflozin for the treatment of type II diabetes. Expert Opin Pharmacother. 2019;20(7):781–790.
- Pafili K, Maltezos E, Papanas N. Pharmacokinetic and pharmacodynamic drug evaluation of tofogliflozin for the treatment of type 2 diabetes. Expert Opin Drug Metab Toxicol. 2016;12(11):1367–1380.
- Yabe D, Hamamoto Y, Seino Y, et al. Sodium glucose co-transporter 2 inhibitor luseogliflozin in the management of type 2 diabetes: a drug safety evaluation. Expert Opin Drug Saf. 2017;16(10):1211–1218.
- Yang J, Yang X, Wang C, et al. Sodium-glucose-linked transporter 2 inhibitors from Sophora flavescens. Med Chem Res. 2015;24(3):1265–1271.
- Seino Y. Luseogliflozin for the treatment of type 2 diabetes. Expert Opin Pharmacother. 2014;15(18):2741–2749.
- Samukawa Y, Sata M, Furihata K, et al. Luseogliflozin, an SGLT2 Inhibitor, in Japanese Patients With Mild/Moderate Hepatic Impairment: a Pharmacokinetic Study. Clin Pharmacol Drug Dev. 2017;6(5):439–447.
- Markham A, Keam SJ. Sotagliflozin: first Global Approval. Drugs. 2019;79(9):1023–1029.
- Rendell MS. Efficacy and safety of sotagliflozin in treating diabetes type 1. Expert Opin Pharmacother. 2018;19(3):307–315.
- Chatzopoulos G, Tziomalos K. An up-to-date evaluation of sotagliflozin for the treatment of type 1 diabetes. Expert Opin Pharmacother. 2020;21(15):1799–1803.
- Mohan V, Mithal A, Joshi SR, et al. Remogliflozin etabonate in the treatment of type 2 diabetes: design, development, and place in therapy. Drug Des Devel Ther. 2020;14:2487–2501.
- Beitelshees AL, Leslie BR, Taylor SI. Sodium–glucose cotransporter 2 inhibitors: a case study in translational research. Diabetes. 2019;68(6):1109–1120.
- Han S, Hagan DL, Taylor JR, et al. Dapagliflozin, a selective SGLT2 inhibitor, improves glucose homeostasis in normal and diabetic rats. Diabetes. 2008;57(6):1723–1729.
- Kshirsagar RP, Kulkarni AA, Chouthe RS, et al. SGLT inhibitors as antidiabetic agents: a comprehensive review. RSC Adv. 2020;10(3):1733–1756.
- Devineni D, Polidori D, Curtin C, et al. Single-dose Pharmacokinetics and Pharmacodynamics of Canagliflozin, a Selective Inhibitor of Sodium Glucose Cotransporter 2, in Healthy Indian Participants. Clin Ther. 2016;38(1):89e1–98.e1.
- Ohgaki R, Wei L, Yamada K, et al. Interaction of the sodium/glucose cotransporter (SGLT) 2 inhibitor canagliflozin with SGLT1 and SGLT2: inhibition kinetics, sidedness of action, and transporter-associated incorporation accounting for its pharmacodynamic and pharmacokinetic featuress. J Pharmacol Exp Ther. 2016;358(1):94–102.
- Grempler R, Thomas L, Eckhardt M, et al. Empagliflozin, a novel selective sodium glucose cotransporter-2 (SGLT-2) inhibitor: characterisation and comparison with other SGLT-2 inhibitors A journal of Pharamacology and Therapeutics . 2012;14(1):83–90 doi:10.1111/j.1463-1326.2011.01517.x.
- Fediuk DJ, Nucci G, Dawra VK, et al. Overview of the Clinical Pharmacology of Ertugliflozin, a Novel Sodium-Glucose Cotransporter 2 (SGLT2) Inhibitor. Clin Pharmacokinet. 2020;59(8):949–965.
- Tahara A, Kurosaki E, Yokono M, et al. Pharmacological profile of ipragliflozin (ASP1941), a novel selective SGLT2 inhibitor, in vitro and in vivo. Naunyn-Schmiedeberg’s Arch Pharmacol. 2012;385(4):423–436.
- Faillie JL. Pharmacological aspects of the safety of gliflozins. Pharmacol Res. 2017;118:71–81.
- Ikeda S, Takano Y, Schwab D, et al. Effect of Renal Impairment on the Pharmacokinetics and Pharmacodynamics of Tofogliflozin (A SELECTIVE SGLT2 Inhibitor) in Patients with Type 2 Diabetes Mellitus. Drug Res (Stuttg). 2019;69(6):314–322.
- Poole RM, Prossler JE. Tofogliflozin: first global approval. Drugs. 2014;74(8):939–944.
- Markham A, Elkinson S. Luseogliflozin: first global approval. Drugs. 2014;74(8):945–950.
- Rosenstock J, Cefalu WT, Lapuerta P, et al. Greater dose-ranging effects on A1C levels than on glucosuria with lx4211, a dual inhibitor of SGLT1 and SGLT2, in patients with type 2 diabetes on metformin monotherapy. Diabetes Care. 2015;38(3):431–438.
- Zambrowicz B, Freiman J, Brown PM, et al. LX4211, a dual SGLT1/SGLT2 inhibitor, improved glycemic control in patients with type 2 diabetes in a Randomized, placebo-controlled trial. Clin Pharmacol Ther. 2012;92(2):158–169.
- He X, Fang J, Huang L, et al. Sophora flavescens Ait.: traditional usage, phytochemistry and pharmacology of an important traditional Chinese medicine. J Ethnopharmacol. 2015;172:10–29.
- Li JJ, Zhang X, Shen X-C, et al. Phytochemistry and biological properties of isoprenoid flavonoids from Sophora flavescens Ait. Fitoterapia. 2020;143:104556.
- Sato S, Takeo J, Aoyama C, et al. Na+-Glucose cotransporter (SGLT) inhibitory flavonoids from the roots of Sophora flavescens. Bioorg Med Chem. 2007;15(10):3445–3449.
- Morikawa T, Tao J, Toguchida I, et al. Structures of New Cyclic Diarylheptanoids and Inhibitors of Nitric Oxide Production from Japanese Folk Medicine Acer n ikoense. J Nat Prod. 2003;66(1):86–91.
- Kurimoto SI, Sasaki YF, Suyama Y, et al. Acylated Triterpene Saponins from the Stem Bark of Acer nikoense (Aceraceae). Chem Pharm Bull. 2016;64(7):924–929.
- Shimokawa Y, Akao Y, Hirasawa Y, et al. Gneyulins A and B, Stilbene Trimers, and Noidesols A and B, Dihydroflavonol- C -Glucosides, from the Bark of Gnetum gnemonoides. J Nat Prod. 2010;73(4):763–767.
- Khyade MS, Kasote DM, Vaikos NP. Alstonia scholaris (L.) R. Br. and Alstonia macrophylla Wall. ex G. Don: a comparative review on traditional uses, phytochemistry and pharmacology. J Ethnopharmacol. 2014;153(1):1–18.
- Changwichit K, Khorana N, Suwanborirux K, et al. Bisindole alkaloids and secoiridoids from Alstonia macrophylla Wall. ex G. Don. Fitoterapia. 2011;82(6):798–804.
- Arai H, Hirasawa Y, Rahman A, et al. Alstiphyllanines E-H, picraline and ajmaline-type alkaloids from Alstonia macrophylla inhibiting sodium glucose cotransporter. Bioorg Med Chem. 2010;18(6):2152–2158.
- Iliya I, Tanaka T, Iinuma M, et al. Stilbene derivatives from two species of Gnetaceae. Chem Pharm Bull. 2002;50(6):796–801.
- Chan SW. Panax ginsengRhodiola rosea and Schisandra chinensis. Int J Food Sci Nutr. 2012;63(SUPPL.1):75–81.
- Zhang M, Xu L, Yang H. Schisandra chinensis fructus and its active ingredients as promising resources for the treatment of neurological diseases. Int J Mol Sci. 2018;19(7). DOI:10.3390/ijms19071970
- Qu Y, Chan JY-W, Wong C-W, et al. Antidiabetic Effect of Schisandrae Chinensis Fructus Involves Inhibition of the Sodium Glucose Cotransporter. Drug Dev Res. 2015;76(1):1–8.
- Morita H, Deguchi J, Motegi Y, et al. Cyclic diarylheptanoids as Na+-glucose cotransporter (SGLT) inhibitors from Acer nikoense. Bioorganic Med Chem Lett. 2010;20(3):1070–1074.
- Choi MK, Nam SJ, Ji H-Y, et al. Comparative pharmacokinetics and pharmacodynamics of a novel sodium-glucose cotransporter 2 inhibitor, DWP16001, with dapagliflozin and ipragliflozin. Pharmaceutics. 2020;12(3):1–16.
- Azzam O, Carnagarin R, Lugo-Gavidia LM, et al. Bexagliflozin for type 2 diabetes: an overview of the data. Expert Opin Pharmacother. 2021;22(16):2095–2103.
- Weng J, Zeng L, Zhang Y, et al. Henagliflozin as add-on therapy to metformin in patients with type 2 diabetes inadequately controlled with metformin: a multicentre, randomized, double-blind, placebo-controlled, phase 3 trial. Diabetes Obesity Metab. 2021;23(8):1754–1764.