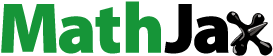
Abstract
This research aims to characterize low-temperature plasma-treated ramie fibers (Boehmeria nivea L. Gaud), create a mathematical model, and analyze them using image processing. The effects of low-temperature plasma treatment on ramie fibers were investigated using 30 kV output powers, 4-min treatment times, and a 4 cm electrode distance. SEM and FT-IR spectroscopy were used to investigate various aspects of the ramie fiber’s chemical properties and surface topography. This study used a statistical approach to analyze the SEM images. The statistical analysis was used to investigate the surface roughness using the SEM images. FT-IR analysis discovered that fibers that were exposed for four minutes differed from untreated fibers. According to the findings, plasma treatment caused: (1) ramie fiber became more hydrophilic, as indicated by the presence of hydrophilic functional groups such as hydroxyl (O–H), carboxyl (O–C), and carbonyl (C=O) in FT-IR with a deeper T%, (2) ramie fiber mass reduction was accompanied by an increase in surface roughness via SEM testing and image processing, and (3) plasma-treated fiber had a coefficient of mass variation (CV) of 0.7211 higher than untreated fiber. The novelty of this study is the use of image processing in SEM to observe surface roughness and its relationship to the chemical structure of ramie fibers observed with FT-IR. Another novelty of this research is the mathematical model of the interaction of plasma species with the ramie fibers on the plane electrode for the first time.
1. Introduction
Natural fibers such as hemp, jute, flax, ramie, sisal and bamboo have grown in popularity as people become more environmentally conscious. As reinforcement materials for green composites, these fibers can replace non-renewable manufactured fibers, reducing carbon emissions. Due to its biodegradability, recyclability, low cost, high specific strength, specific modulus and commercial availability, ramie fiber has been considered one of the most promising candidates for the green composite industry (Ding, Zhou, & Dong, Citation2023; Liu et al., Citation2013; Liu & Cheng, Citation2016; Xu et al., Citation2015; Zhang, Jiang, Yao, Jiang, & Qiu, Citation2015). The use of ramie fiber is limited by some problems despite its potential, for example the processing challenge, limited availability and high-cost production (Jose, Rajna, & Ghosh, Citation2016). In terms of material, ramie fiber has hydrophilic properties (Jagadeesh, Puttegowda, Mavinkere Rangappa, & Siengchin, Citation2021; Zhang et al., Citation2020). The natural fiber can be surface-modified to chemically or physically more hydrophilic or hydrophobic using plasma. Various surface modification techniques using chemical processes have been used in the past, such as alkaline treatments (Li, Tabil, & Panigrahi, Citation2007; Ray et al., Citation2001a, Citationb; Zhou, Yu, Sun, Yao, & Qiu, Citation2012), acetylation treatments (Bledzki, Mamun, Lucka-Gabor, & Gutowski, Citation2008; Kalaprasad et al., Citation2004), and stearic acid treatments (Kalaprasad et al., Citation2004; Torres & Cubillas, Citation2005). Some researchers have also studied the ramie fiber about fiber property modification (Huang et al., Citation2021; Citation2022; Li, Li, Ding, & Yu, Citation2016; Li, Meng, & Yu, Citation2015; Lin, Tang, Huang, Yu, Yu, et al., Citation2022; Lin et al., Citation2022; Li & Yu, Citation2014; Shen, Tang, Chen, & Li, Citation2022). It has been shown that some of these treatments improve interfacial adhesions. However, they still have problems with pollutant waste disposal, high energy and water consumption, and fiber damage. There is some evidence that plasma technology can be used for physical methods (Torres & Cubilas, Citation2005). Unlike traditional methods, this technique significantly reduces the use of harsh chemicals and minimizes environmental impact. The process operates at lower temperatures, decreasing energy consumption and emissions compared to conventional treatments. Additionally, by avoiding certain chemical agents, we contribute to a more sustainable and eco-friendly approach in the treatment of ramie fibers. Using plasma treatment to modify fiber surfaces is more environmentally friendly and practical, as it alters surfaces’ chemical and physical properties without affecting their bulk properties (Torres & Cubillas, Citation2005). Ionized plasma is made up of free particles, and it is the fourth material after solids, liquids, and gases. Several researchers have identified Corona discharge plasma as a powerful method for improving the adhesion properties of textile materials (e.g., woven, knitted, and non-woven fabrics) (Morent et al., Citation2008; Rauscher et al., Citation2010; Shishoo, Citation2007). There has been intense research on plasma sources for surface modification of polymers to replace conventional wet chemical methods (Rauscher et al., Citation2010; Shishoo, Citation2007). Some researchers reported using plasma to modify polymers, for example, work of adhesion and surface roughness (Barani & Calvimontes, Citation2014; Chi-Wai, Kwong, Chun-Wah, & Hom, Citation2004; Kan & Yuen, Citation2007; Lieberman & Lichtenberg, Citation1994; Ma et al., Citation2014; Morent et al., Citation2008; Shishoo, Citation2007). In previous studies, plasma treatment was investigated on ramie fibers (Hamad, Stehling, Hayes, Foreman, & Rodenburg, Citation2019; Li, Moyo, Ding, Shan, & Qiu, Citation2013; Liu & Cheng, Citation2016). Traditionally, researchers use the SEM method to examine the plasma treatment effect on fibers on the microscopic scale. Researchers, however, haven’t used image processing when analyzing scanning electron microscopes. The observation that relies on the result of the SEM can’t be used to measure the surface roughness quantitatively.
Researchers have studied the surface roughness of some materials by analyzing three-dimensional images (Lieberman & Lichtenberg, Citation1994; Morent et al., Citation2008; Shishoo, Citation2007; Neeraj & Veeresha, Citation2022). On the other hand, surface roughness on the fibers on the microscopic scale is tricky to measure directly (Ondra, Citation2000; Rauscher et al., Citation2010). The three-dimensional image processing analysis technique has some advantages: no need for physical contact, no surface material damage, and easy implementation (Reddy, Citation2004). Although many researchers utilize image processing to assess surface roughness in materials, to the author’s knowledge, image processing has not been applied to the analysis of surface roughness in the relationship of FT-IR chemical structure and adhesive characteristics of ramie fiber. In this study, image processing in SEM was used for the first time to observe surface roughness concerning FT-IR chemical structure. An infrared spectroscopy and three-dimensional image processing analysis were used to investigate the effects of corona plasma treatment on ramie fibers. Ramie fibers were treated with a corona plasma apparatus with 25 electrode tips and a flat electrode. SEM, FT-IR and three-dimensional images using MATLAB® R2022a analysis were used to study the modification effect. The scientific application of this research is that engineers and researchers can use this study’s findings to improve the quality of ramie fiber using environmentally friendly methods. As a novelty and contribution, this research employs image processing within SEM using MATLAB® R2022a to observe surface roughness and the relationship between surface roughness and ramie fibers’ chemical structure, as observed using FT-IR for the first time. Another novelty of this research is the mathematical model of the interaction of plasma species with the ramie fibers on the plane electrode for the first time. The scientific application of this study is that researchers and engineers can utilize the findings to improve ramie fiber quality using environmentally friendly technology.
2. Research methods
2.1. Instrumentation
In this study, a prototype apparatus, low-temperature plasma (corona plasma), was assembled at the Physics Laboratory of Politeknik STTT Bandung, the Ministry of Industry of the Republic of Indonesia. Corona plasma generator’s electrode configurations, including multi-point and flat electrode, were available. The electrodes were connected to a 30 kV power supply using ambient gas, atmospheric pressure, and constant room temperature. This experiment used twenty-five tipped bolts as multi-point electrodes (point electrodes) connected to a resistor and
capacitor. The ramie fibers were placed in the flat electrodes connected to a
resistor, as illustrated in . The multi-point electrodes were used as positive electrodes perpendicular to the flat electrodes. A.C. input and D.C. output voltages were measured using a high-voltage multi-tester (ISO 16750-2) at the Physics Laboratory of Politeknik STTT Bandung, Indonesia. illustrates the experimental design and instruments used in this study. shows the plasma apparatus utilized in this experiment.
2.2. Materials
Ramie fibers (Boehmeria nivea L. Gaud) were purchased from CV. Rabersa in Wonosobo, Central Java, Indonesia. There is 20 cm of fibers arranged in parallel in . The fibers vary in length from 2.5 to 5.0 cm with an average of 12.5–15 cm, their diameter ranges from 25 to 75 μm with an average of 30–50 μm with overall mass of g. This study included two samples, one with plasma treatment and one without plasma treatment. Plasma treatment was performed by placing ramie fibers into a plasma chamber at 30 kV, room temperature, atmospheric pressure, and 4 cm asymmetry between electrodes. The ramie fibers are arranged in a row and placed on a flat electrode. We repeated the experiment three times to analyze the characteristics of ramie fibers with and without plasma treatment.
2.3. Methods
A scanning electron microscope (SEM) and infrared spectroscopy were used to study the effects of corona plasma treatments on ramie fibers. illustrates how the ramie samples were placed on the flat electrode. A flat electrode was used in the chamber to apply plasma to the ramie samples. For this experiment, we adjusted the voltage to 30 kV, plasma exposure time to 4 min, and electrode distance to 4 cm. Ramie fiber plasma treatment is shown in . The ramie fibers were exposed to corona plasma at room temperature and atmospheric pressure, then characterized using FT-IR and SEM after they were placed at room temperature and atmospheric pressure. To investigate the effect of plasma treatment on fiber mass, we also measured the mass of the plasma-treated fibers before and after treatment. The mass of ramie fibers () was also measured using an analytical balance.
2.3.1. Scanning electron microscopy
We examined the surface morphology of ramie fibers using a scanning electron microscope (SEM) JEOL JSM-6360LA with an Energy Dispersive System (EDS) at the Marine Geological Research and Development Center, Bandung, Indonesia. In order to examine the surface morphology of ramie fibers and the roughness of their surfaces after plasma treatment, SEM imaging was performed at magnifications of 3000.
2.3.2. Fourier transform infrared spectroscopy
We investigated the chemical composition of the fibers using a Shimadzu Prestige 21 FT-IR apparatus at the Physics laboratory, Faculty of Mathematics and Natural Science, ITB, Indonesia. FT-IR analysis was performed between wavenumber frequencies of 500 and 4000 cm−1. To investigate th effect of plasma treatment, two scans were taken for the sample between 500 and 4000 cm−1, with a resolution of 2 cm−1. Ramie fibers were scraped with a knife with 20 cm in length and placed in an FT-IR spectrometer to scan the surface chemical changes before and after the plasma treatment.
2.3.3. Image processing
The experiment’s SEM image samples were considered for analysis. We found that SEM images could be used to visualize three-dimensional images. An SEM sample of a specific area in a ramie fiber was used for the investigation of the effect of plasma treatment on the surface ramie of the fiber. MATLAB code was used to create histograms and analyze image morphology in three dimensions. Obtaining the three-dimensional image and generating its histogram, the image was equalized to ensure the histogram was evenly distributed. SEM images were also analyzed using morphological gradients and operations. The images are analyzed using the following method: (1) obtain the original image from the SEM; (2) convert the images to greyscale; (3) examine the greyscale histogram; (4) produce a three-dimensional surface plot (a three-dimensional surface with solid edge and face colors); (5) computes the mean of all array values; and (6) calculates the standard deviation of all array values. Using EquationEqs. (1)(1)
(1) to Equation(4)
(4)
(4) , we also calculated the statistical variables of the N data, such as standard deviation (
mean (
standard error (S.E.), and coefficient of mass variation (CV%).
(1)
(1)
(2)
(2)
(3)
(3)
(4)
(4)
where
is the pixel intensity (0–255 in grayscale),
is the mean value of the pixel intensity,
is the number of pixels in the image and
is the standard deviation of the data.
3. Results and discussions
3.1. Infrared spectroscopy analysis
Surface modifications caused by atmospheric plasma treatment of samples were determined using an FT-IR spectrometer at room temperature and atmospheric pressure. FT-IR spectroscopy identified and quantified the chemical bonds between untreated and plasma-treated fiber. shows infrared spectra and some characteristics of absorption peaks between untreated and plasma-treated fibers. The treated fibers (plasma-treated for four minutes) were virtually changed compared to untreated fibers. As a result, this plasma treatment changed the chemical groups of the fiber. Some chemical bonds changed in the plasma-treated fiber, as shown in . The infrared spectrum showed many absorption bands for fiber compounds, including cellulose, hemicellulose, lignin, waxes, and water. Infrared spectra of ramie fiber showed some peaks that indicated its chemical structure. The C–O–C stretching mode, part of the cellulose structure, was visible at wavenumber 2135 cm−1. We discovered that O–H stretching occurred at wavenumber 3408 cm−1. It also occurred at wavenumbers 1722 and 1112 cm−1, which were related to the C=O and C–O stretching modes. summarizes the significant bands and their attributions. The observation in agrees with some previous research (Li et al., Citation2016; Lin et al., Citation2022). The main composition of raw ramie fiber cellulose and hemicellulose. According to the observed infrared spectra, plasma-treated transmittance (T%) was generally lower than untreated transmittance (T%). Due to the plasma reaction between the fiber surface and the plasma species provided by the treatment, it was possible to achieve this. shows that at wavenumber 3408 cm−1, transmittance dropped from 25 to 11%. As a result of the interaction between the fiber surface and the plasma species, there was a decrease in T% at this wavenumber 3408 cm−1, corresponding to O-H stretching. The transmittance (T%) of plasma-treated fibers also decreased at 1641 cm−1 compared to untreated fibers. Plasma probably removed fatty acids and waxes due to the reaction. According to the findings, plasma treatment caused ramie fiber to become more hydrophilic, as indicated by the presence of hydrophilic functional groups such as hydroxyl (O–H), carboxyl (O–C), and carbonyl (C=O) in FT-IR with a deeper transmittance (T%). This finding agrees with some previous studies which have also suggested that the plasma treatment can cause the functional groups such as (C=O), (O–H), (COOH), (COO–) were found in the surface of plasma treated sample (Chen & Ries, Citation1996; Huang et al., Citation2013; Lubis, Saraswati, Setiawan, & Kusumandari, Citation2019). These functional groups were formed on the surface of ramie fibers through an interaction between active species caused by plasma in the gas phase and fabric surface atoms. Plasma-treated ramie fibers exhibit better wetting and dye absorption due to an increase in oxygen-containing surface groups.
Table 1. Summary of observed FT-IR absorption peaks for ramie fiber with associated chemical components.
depicts the FT-IR spectra obtained for the untreated and atmospheric plasma-treated samples. The presence of additional hydroxyl groups (oxygen that is single bonded to a carbon and single bonded to a hydrogen atom) on the plasma-treated sample was revealed by a new sharp absorption band with peak intensity at 3408 cm−1, characteristics of C–OH. Moreover, the higher the hydroxyl groups on the sample, the higher the wettability. After plasma treatment the intensity of the peak at 3408 cm−1 increased, which is related to an increase in hydroxyl groups. A reaction between active species induced by plasma in the gas phase and the fabric surface atoms produced these functional groups on the surface of the ramie fibers. As a result of the increased oxygen content of surface groups on the ramie fibers, the fibers have an improved wetting ability. This finding agrees with some previous investigation showed that an increase in the intensity of the peak of FT-IR spectra was related to an increase in carbonyl –C=O groups after plasma treatment (Fakin, Ojstršek, & Benkovič, Citation2009; Haji & Qavamnia, Citation2015; Haji & Shoushtari, Citation2011). Using plasma treatment, ramie fibers are stripped of their lipid and surface cuticles without affecting their bulk properties. The FT-IR spectra also revealed an intense distinct band with peak intensity at 1112 cm−1, typical of the C–O peak in the plasma-treated sample, which was significantly higher than in the untreated sample, which revealed the presence of acidic groups. The FT-IR spectra showed the presence of the carbonyl group at wavenumber 1722 cm−1. The carbonyl group (C=O) comprises a carbon atom double-bounded to an oxygen atom. This showed that the fiber had hydrophilic properties. The carboxyl group is determined as a carbon atom to which two oxygen atoms are bonded. One of the two oxygen atoms is bonded to a double bond (C=O), and the other oxygen is connected to a hydrogen atom (–C–OH). The carboxyl group can be represented as –COO–H. As a result of plasma treatment on the fiber surface, new functional groups (i.e., –O.H. and –COOH) were formed, which affected wetting time and assisted graft polymerization. FT-IR spectra, including hydroxyl, carbonyl, and carboxyl groups, revealed hydrophilic functional groups were present on treated fibers. With enough energy, electrons could be separated from atoms and molecules in the volume between the electrodes to produce plasma. A surface’s modification ability depends on the number of electrons and ions present. In this study, the observations also agree with the results reported by some researchers who reported using plasma to change polymers, for example, work of adhesion, chemical structure, and surface roughness (Huang et al., Citation2021; Citation2022; Lin et al., Citation2022; Citation2022; Morent et al., Citation2008; Rauscher et al., Citation2010; Shishoo, Citation2007).
3.2. Surface morphology analysis
Ramie fibers were found to have improved surface properties, especially roughness, after plasma treatment. Based on our visual examination using scanning electron microscopy (SEM) of plasma-treated ramie fibers, as shown in , the surface roughness of the plasma-treated fibers appears rougher than that of the untreated fibers. To measure roughness quantitatively, we used image processing. The emphasis on SEM image processing in our study serves a dual purpose. Firstly, it allows for a precise and quantitative characterization of the treatment effects on ramie fibers. This analytical method helps us understand the structural changes and improvements brought about by the low-temperature plasma treatment. Secondly, SEM analysis aids in showcasing the environmentally friendly aspects by providing visual evidence of reduced damage to fibers and minimal use of chemical agents. The SEM images were first simulated using MATLAB® R2022a. The three-dimensional plot of the SEM images is shown in . In , the x and y-axis are the pixel size, and the z-axis is the pixel intensity (0–255 in grayscale). The relative frequency distribution of pixel intensity is shown in , and the results of statistical variables are summarized in . Plasma-treated fibers had a higher coefficient of variation, 0.7211, than untreated fibers. Based on this result, plasma-treated fiber was rougher than untreated fiber. As a result of the plasma treatment process, the fiber mass decreased. Before the treatment, the fiber mass was g; after the treatment, it was
g. Plasma species reacting with fiber surfaces caused a mass reduction of 0.22%. As a result of the plasma reaction, the fiber’s surface was etched and cleaned, resulting in mass reduction.
Figure 5. Three-dimensional SEM image processing: (a) untreated ramie fiber and (b) the plasma-treated ramie fiber.
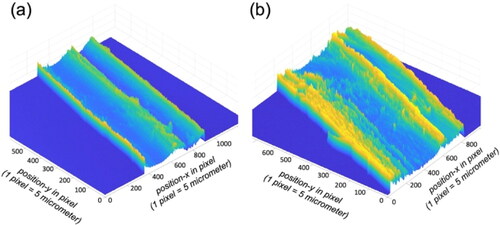
Figure 6. Histogram of the pixel intensity comparison from SEM image: (a) untreated fiber and (b) plasma-treated fiber.
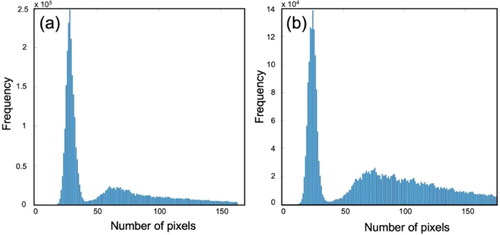
Table 2. Summary of the statistical value of SEM images analysis.
According to the findings, plasma treatment caused ramie fiber mass reduction was accompanied by increased surface roughness via SEM testing and image processing, and plasma-treated fiber had a coefficient of mass variation (CV%) of 0.7211 higher than untreated fiber (0.6940). According to some researchers (Barani & Calvimontes, Citation2014; Shen et al., Citation2022), the rougher the surface of a material, the higher its surface tension and work of adhesion. In this study, the observations are also consistent with the results of some researchers who reported using plasma to modify polymers, such as work of adhesion and surface roughness (Barani & Calvimontes, Citation2014; Chi-Wai et al., Citation2004; Kan & Yuen, Citation2007; Lieberman & Lichtenberg, Citation1994; Ma et al., Citation2014). According to the findings of research and studies from various sources of literature, plasma treatment of ramie fiber resulted in a decrease in fiber mass, changes in fiber surface roughness, increased surface tension and work of adhesion of the material, and increased hydrophilic functional groups such as hydroxyl (O–H), carboxyl (O–C), and carbonyl (C=O) (Rauscher et al., Citation2010; Shishoo, Citation2007). The 3-dimensional SEM image analysis revealed that ramie fibers improved surface properties after plasma treatment. The surface roughness of the plasma-treated fibers appeared rougher than that of the untreated fibers. When the ramie fibers were treated with plasma, the surface was bombarded with plasma species, increasing the surface roughness of the ramie fibers. depicts the reaction between plasma species and ramie fibers. In the present research, we also develop a mathematical model to explain the interaction between plasma species and fibers. We assume the tip electrode is an L-length bar with a uniform charge density
EquationEquation (5)(5)
(5) shows the electric force (
) in the area between the tip-plane electrodes.
(5)
(5)
where
is the charges of a plane electrode (with a specific area A and surface density
) on which to place the ramie fibers, and E is the generator’s electric field. The electric field
generated by a point charge
the tip electrode, can also be written as in EquationEq. (6)
(6)
(6) .
(6)
(6)
where
is the electrical permittivity. For the tip electrode with uniform charge density
EquationEq. (6)
(6)
(6) can be written as EquationEq. (7)
(7)
(7)
(7)
(7)
with
and the direction of the electric field is along the
-axis, as shown in EquationEq. (8)
(8)
(8)
(8)
(8)
Integrating EquationEq. (7)(7)
(7) with the boundary of integration from
to
results in the electric field at a point
below the tip electrode
as in EquationEqs. (9)
(9)
(9) and Equation(10)
(10)
(10) .
(9)
(9)
(10)
(10)
The electric force at point between the tip-plane electrodes with a voltage, V, is expressed as
(11)
(11)
(12)
(12)
When EquationEq. (12)(12)
(12) is substituted into EquationEq. (11)
(11)
(11) , the electric voltage between the two electrodes is
(13)
(13)
The interaction between the plasma species and the surface of the fibers is influenced by the distance of the fibers on the plane electrode (a) and the charge generated in the tip electrode (Q), according to EquationEq. (11)(11)
(11) . The positive-charged plasma species are accelerated toward the fibers on the plane electrode by electric force. They may cause a reaction with the fibers. According to EquationEq. (13)
(13)
(13) , the higher the separation a, the smaller the voltage, V, between the two electrodes. For a constant electrode distance, EquationEq. (13)
(13)
(13) demonstrates that the higher the number of plasma species created (Q), the higher the voltage between the electrodes. The closer the electrode distance is, the greater the impact force of the plasma species on the fibers. The more significant the plasma species’ impact on the fibers, the rougher the fiber’s surface and the lower the fiber’s mass. The interaction of plasma species with the fiber surface increases roughness while functionalizing or activating the fiber surface. The modeling results confirmed the findings from the experiments, which indicated that fibers exposed to an electric field for four minutes generated plasma species almost changed compared to untreated fibers. The transmittance intensity (T%) of plasma-treated fibers was lower than that of untreated fiber. According to our scanning electron microscopy (SEM) and image processing results, plasma-treated ramie fibers had increased surface roughness, indicating changes in their surface properties. A plasma-treated fiber had a coefficient of mass variation (CV%) of 0.7211, while an untreated fiber resulted in a coefficient of mass variation (CV%) of 0.6940. Our study also revealed that plasma treatment could reduce the mass of ramie fibers by 0.22%.
4. Conclusions
In the current research, we analyzed the effects of plasma treatment on the surface of ramie fiber through an infrared spectroscopy test and a scanning microscope electron (SEM) test. FT-IR testing showed that fibers exposed for 4 min almost changed compared to untreated fibers. Plasma-treated fiber had lower transmittance intensity (T%) than untreated fiber. According to our scanning electron microscopy (SEM) and image processing results, plasma-treated ramie fibers had increased surface roughness, indicating changes in their surface properties. A plasma-treated fiber had a coefficient of mass variation (CV%) of 0.7211, while an untreated fiber resulted in a coefficient of mass variation (CV%) of 0.6940. Our study also revealed that plasma treatment could reduce the mass of ramie fibers by 0.22%. According to the findings, plasma treatment caused: (1) Ramie fiber to become more hydrophilic, as indicated by the presence of hydrophilic functional groups such as hydroxyl (O–H), carboxyl (O–C), and carbonyl (C=O) in FT-IR with a deeper T%, (2) ramie fiber mass reduction was accompanied by an increase in surface roughness and work of adhesion (surface tension) via SEM testing and image processing, and (3) plasma-treated fiber had a coefficient of mass variation (CV%) of 0.7211 higher than untreated fiber. EquationEquations (11)(11)
(11) and Equation(13)
(13)
(13) also demonstrated that the interaction between the plasma species and the surface of the fibers was influenced by the distance of the fibers from the plane electrode (a) and the charge generated in the tip electrode (Q). An electric force causes positive-charged plasma species to move toward fibers on a plane electrode and may react with them. The characterization of ramie with Corona discharge plasma treatment was performed for the first time using image processing in SEM to observe surface roughness and its relationship to the chemical structure observed with FT-IR. The scientific application of this study is that researchers and engineers can use the findings to improve the quality of ramie fiber using environmentally friendly technology. In the future, we suggest using XPS analysis to determine the difference between untreated and plasma-treated structures based on some factors, such as the number of samples that may vary from run to run, altering the intensity response.
Acknowledgments
The authors thank Universitas Gadjah Mada, Indonesia, the Industrial Human Resources Development Agency (BPSDMI), Ministry of Industry of the Republic of Indonesia and the Indonesia Endowment Funds for Education (LPDP) for providing adequate facilities and funding. We also thank our colleagues who helped us with the research and analysis.
Disclosure statement
The authors declare that they have no known competing financial interests or personal relationships that could have influenced the work reported in this paper.
References
- Barani, H., & Calvimontes, A. (2014). Effects of oxygen plasma treatment on the physical and chemical properties of wool fiber surface. Plasma Chemistry and Plasma Processing, 34(6), 1291–1302. doi:10.1007/S11090-014-9581-X/TABLES/3
- Bledzki, A. K., Mamun, A. A., Lucka-Gabor, M., & Gutowski, V. S. (2008). The effects of acetylation on properties of flax fibre and its polypropylene composites. Express Polymer Letters, 2(6), 413–422. doi:10.3144/expresspolymlett.2008.50
- Chen, H. H., & Ries, M. D. (1996). Surface energy modification and characterization of a plasma-polymerized fluoropolymer. Journal of Adhesion Science and Technology, 10(6), 495–513. doi:10.1163/156856196X00553
- Chi-Wai, K., Kwong, C., Chun-Wah, M. Y., & Hom, H. (2004). The possibility of low-temperature plasma treated wool fabric for industrial use. AUTEX Research Journal, 4(1), 37–44. doi:10.1515/aut-2004-040107
- Ding, J., Zhou, C., & Dong, Z. (2023). Trend of ramie industry development: A review of green degumming and the utilization of processing residues. Journal of Cleaner Production, 384, 135487. doi:10.1016/j.jclepro.2022.135487
- Fakin, D., Ojstršek, A., & Benkovič, S. Č. (2009). The impact of corona modified fibres’ chemical changes on wool dyeing. Journal of Materials Processing Technology, 209(1), 584–589. doi:10.1016/j.jmatprotec.2008.02.034
- Haji, A., & Qavamnia, S. S. (2015). Response surface methodology optimized dyeing of wool with cumin seeds extract improved with plasma treatment. Fibers and Polymers, 16(1), 46–53. doi:10.1007/S12221-015-0046-5/METRICS
- Haji, A., & Shoushtari, A. M. (2011). Natural antibacterial finishing of wool fiber using plasma technology. Industria Textila, 62, 244–247.
- Hamad, S. F., Stehling, N., Hayes, S. A., Foreman, J. P., & Rodenburg, C. (2019). Exploiting Plasma Exposed, Natural Surface Nanostructures in Ramie Fibers for Polymer Composite Applications. Materials, 12(10), 1631. doi:10.3390/ma12101631
- Huang, H., Tang, Q., Lin, G., Liu, Y., Yu, J., Ding, B., & Li, Z. (2022). Anthraquinone-assisted deep eutectic solvent degumming of ramie fibers: Evaluation of fiber properties and degumming performance. Industrial Crops and Products, 185, 115115. doi:10.1016/j.indcrop.2022.115115
- Huang, H., Tang, Q., Lin, G., Yu, C., Wang, H., & Li, Z. (2021). High-efficiency and recyclable ramie cellulose fiber degumming enabled by deep eutectic solvent. Industrial Crops and Products, 171, 113879. doi:10.1016/j.indcrop.2021.113879
- Huang, C. Y., Wu, J. Y., Tsai, C. S., Hsieh, K. H., Yeh, J. T., & Chen, K. N. (2013). Effects of argon plasma treatment on the adhesion property of ultra high molecular weight polyethylene (UHMWPE) textile. Surface and Coatings Technology, 231, 507–511. doi:10.1016/j.surfcoat.2012.04.069
- Jagadeesh, P., Puttegowda, M., Mavinkere Rangappa, S., & Siengchin, S. (2021). A review on extraction, chemical treatment, characterization of natural fibers and its composites for potential applications. Polymer Composites, 42(12), 6239–6264. doi:10.1002/pc.26312
- Jose, S., Rajna, S., & Ghosh, P. (2016). Ramie fibre processing and value addition. Asian Journal of Textile, 7(1), 1–9. doi:10.3923/ajt.2017.1.9
- Kalaprasad, G., Francis, B., Thomas, S., Kumar, C. R., Pavithran, C., Groeninckx, G., & Thomas, S. (2004). Effect of fibre length and chemical modifications on the tensile properties of intimately mixed short sisal/glass hybrid fibre reinforced low density polyethylene composites. Polymer International, 53(11), 1624–1638. doi:10.1002/pi.1453
- Kan, C. W., & Yuen, C. W. M. (2007). Plasma technology in wool. Textile Progress, 39(3), 121–187. doi:10.1080/00405160701628839
- Lieberman, M., & Lichtenberg, A. (1994). Principles of plasma discharges and materials processing. New York, USA: John Wiley and Sons.
- Li, Z., Li, Z., Ding, R., & Yu, C. (2016). Composition of ramie hemicelluloses and effect of polysaccharides on fiber properties. Textile Research Journal, 86(5), 451–460. doi:10.1177/0040517515592811
- Li, Z., Meng, C., & Yu, C. (2015). Analysis of oxidized cellulose introduced into ramie fiber by oxidation degumming. Textile Research Journal, 85(20), 2125–2135. doi:10.1177/0040517515581589
- Li, Y., Moyo, S., Ding, Z., Shan, Z., & Qiu, Y. (2013). Helium plasma treatment of ethanol-pretreated ramie fabrics for improving the mechanical properties of ramie/polypropylene composites. Industrial Crops and Products, 51, 299–305. doi:10.1016/j.indcrop.2013.09.028
- Lin, G., Tang, Q., Huang, H., Yu, J., Li, Z., & Ding, B. (2022). Process optimization and comprehensive utilization of recyclable deep eutectic solvent for the production of ramie cellulose fibers. Cellulose, 29(7), 3689–3701. doi:10.1007/S10570-022-04501-0/FIGURES/6
- Lin, G., Tang, Q., Huang, H., Yu, C., Yu, J., Li, Z., & Ding, B. (2022). One-step extraction of ramie cellulose fibers and reutilization of degumming solution. Textile Research Journal, 92(19–20), 3579–3590. doi:10.1177/00405175221086886
- Li, X., Tabil, L. G., & Panigrahi, S. (2007). Chemical treatments of natural fiber for use in natural fiber-reinforced composites: A review. Journal of Polymers and the Environment, 15(1), 25–33. doi:10.1007/s10924-006-0042-3
- Liu, X., & Cheng, L. (2016). Influence of plasma treatment on properties of ramie fiber and the reinforced composites. Journal of Adhesion Science and Technology, 31(15), 1723–1734. doi:10.1080/01694243.2016.1275095
- Liu, L-j., Lao, C-y., Zhang, N., Chen, H-q., Deng, G., Zhu, C., & Peng, D-x (2013). The effect of new continuous harvest technology of ramie (Boehmeria nivea L. Gaud.) on fiber yield and quality. Industrial Crops and Products, 44, 677–683. doi:10.1016/j.indcrop.2012.09.003
- Li, Z., & Yu, C. (2014). Effect of peroxide and softness modification on properties of ramie fiber. Fibers and Polymers, 15(10), 2105–2111. doi:10.1007/S12221-014-2105-8/METRICS
- Lubis, R. W., Saraswati, T. E., Setiawan, U. H., & Kusumandari, K. (2019). Surface modification of activated carbon using an atmospheric pressure dielectric barrier discharge (DBD) plasma jet. IOP Conference Series: Materials Science and Engineering, 578(1), 012010. doi:10.1088/1757-899X/578/1/012010
- Ma, S., Bromberg, V., Liu, L., Egitto, F. D., Chiarot, P. R., & Singler, T. J. (2014). Low temperature plasma sintering of silver nanoparticles. Applied Surface Science, 293, 207–215. doi:10.1016/j.apsusc.2013.12.135
- Morent, R., De Geyter, N., Verschuren, J., De Clerck, K., Kiekens, P., & Leys, C. (2008). Non-thermal plasma treatment of textiles. Surface and Coatings Technology, 202(14), 3427–3449. doi:10.1016/J.SURFCOAT.2005.05.004
- Neeraj, R. H., & Veeresha, R. K. (2022). A review on surface roughness measurement using image processing. International Journal of Research Publication and Reviews, 3, 1104–1107. doi:10.55248/gengpi.2022.3.8.38
- Ondra, J. (2000). "Measurement of Roughness Using Image Processing," Measurement - Supports Science - Improves Technology - Protects Environment … and Provides Employment - Now and in the Future, Vienna, Austria.
- Rauscher, H., Perucca, M., & Buyle, G. (2010). Plasma technology for hyperfunctional surfaces. Weinheim, Germany: Wiley. doi:10.1002/9783527630455
- Ray, D., Sarkar, B. K., Rana, A. K., & Bose, N. R. (2001a). Effect of alkali treated jute fibres on composite properties. Bulletin of Materials Science, 24(2), 129–135. doi:10.1007/BF02710089
- Ray, D., Sarkar, B. K., Rana, A. K., & Bose, N. R. (2001b). The mechanical properties of vinylester resin matrix composites reinforced with alkali-treated jute fibres. Composites Part A: Applied Science and Manufacturing, 32(1), 119–127. doi:10.1016/S1359-835X(00)00101-9
- Reddy, A. C. (2004). Evaluation of Surface Roughness Using Image Processing Technique. In: Proceedings of the International Conference on Systemics, Cybernetics and Informatics; doi:10.13140/2.1.2687.5207
- Shen, P., Tang, Q., Chen, X., & Li, Z. (2022). Nanocrystalline cellulose extracted from bast fibers: Preparation, characterization, and application. Carbohydrate Polymers, 290, 119462. doi:10.1016/J.CARBPOL.2022.119462
- Shishoo, R. (2007). Plasma technologies for textiles (1st ed.). Cambridge, UK: Woodhead Publishing.
- Torres, F. G., & Cubillas, M. L. (2005). Study of the interfacial properties of natural fibre reinforced polyethylene. Polymer Testing, 24(6), 694–698. doi:10.1016/j.polymertesting.2005.05.004
- Xu, C., Gu, Y., Yang, Z., Li, M., Li, Y., & Zhang, Z. (2015). Mechanical properties of surface-treated ramie fiber fabric/epoxy resin composite fabricated by vacuum-assisted resin infusion molding with hot compaction. Journal of Composite Materials, 50(9), 1189–1198. doi:10.1177/0021998315590259
- Zhang, Z., Cai, S., Li, Y., Wang, Z., Long, Y., Yu, T., & Shen, Y. (2020). High performances of plant fiber reinforced composites—A new insight from hierarchical microstructures. Composites Science and Technology, 194, 108151. doi:10.1016/j.compscitech.2020.108151
- Zhang, Q., Jiang, Y., Yao, L., Jiang, Q., & Qiu, Y. (2015). Hydrophobic surface modification of ramie fibers by plasma-induced addition polymerization of propylene. Journal of Adhesion Science and Technology, 29(8), 691–704. doi:10.1080/01694243.2014.997380
- Zhou, N., Yu, B., Sun, J., Yao, L., & Qiu, Y. (2012). Influence of chemical treatments on the interfacial properties of ramie fiber reinforced poly(lactic acid) (PLA) composites. Journal of Biobased Materials and Bioenergy, 6(5), 564–568. doi:10.1166/jbmb.2012.1258