Abstract
Introduction: With 3 – 4 million new infections occurring annually, hepatitis C virus (HCV) is a major global health problem. There is increasing evidence to suggest that HCV will be highly amenable to a vaccine approach, and despite advances in treatment, a vaccine remains the most cost-effective and realistic means to significantly reduce the worldwide mortality and morbidity associated with persistent HCV infection.
Areas covered: In this review we discuss immune responses to HCV during natural infection, and describe how they may inform vaccine design. We introduce the current candidate vaccines for HCV and compare how these fare against the expected requirements of an effective prophylactic HCV vaccine in relation to the breadth, functionality, magnitude and phenotype of the vaccine-induced immune response.
Expert opinion: Although the correlates of immune protection against HCV are not completely defined, we now have vaccine technologies capable of inducing HCV-specific adaptive immune responses to an order of magnitude that are associated with protection during natural infection. The challenge next is to i) establish well-characterised cohorts of people at risk of HCV infection for vaccine efficacy testing and ii) to better understand the correlates of protection in natural history studies. If these can be achieved, a vaccine against HCV appears a realistic goal.
1. Introducing the problem
The prevention of persistent hepatitis C virus (HCV) infection is an area of real unmet clinical need. Of the estimated 3 – 4 million new HCV infections per year, 10 – 20% will go on to develop chronic liver disease and HCV is now the most common indicator for liver transplantation in many countries Citation[1,2]. HCV is able to persist in up to 70% of immune-competent hosts it infects, and this leads to a state of chronic hepatic inflammation, which can progress to fibrosis and cirrhosis of the liver, and ultimately liver failure or hepatocellular carcinoma Citation[1,3].
It was previously thought that an effective vaccine against HCV would be impossible; however, we now know that a significant number of individuals spontaneously clear the virus in the setting of an appropriate immune response, and there is evidence of protective immunological memory against HCV in chimpanzees (Pan troglodytes) and humans, where secondary infection is associated with reductions in peak viral titre, duration of viraemia, hepatic inflammation and an increased rate of viral clearance Citation[4-6]. Immunological memory does not appear as effective as is seen with Hepatitis A, B or E, as it is rarely, if ever, sterilising (reviewed in Citation[4,6-8]); however, an attenuated course of infection associated with early viral clearance prevents chronicity and significant liver disease Citation[4,6]. The goal for a vaccine against HCV is unique in that to prevent the majority of disease it need only prevent persistence of the virus rather than prevent infection.
Currently, there is no licensed vaccine for HCV and treatment is based on pegylated-interferon-α (IFNα) and the nucleoside analogue ribavirin. This is expensive, relatively toxic, prolonged (24 – 48 weeks) and leads to a sustained virological response (SVR) in only 50 – 60% of patients, depending on the infecting genotype Citation[9]. New directly acting antivirals (DAAs) that target specific HCV proteins are emerging for the treatment of HCV. Two first-generation protease inhibitors boceprevir (Victrelis®, Merck) and telaprevir (Incivek®, Vertex; Incivo®, Johnson & Johnson) have been approved for use in the treatment of genotype 1 HCV, and newer DAAs targeting a wider spread of genotypes and offering increased SVR rates are expected to be developed over the next decade Citation[10,11]. Despite continuing improvements in the prevention of HCV transmission, and in treatment regimens, HCV is likely to persist in areas with limited access to antivirals and poor blood product hygiene and needle usage. There is evidence that significant reductions in incidence of HCV infection, particularly for non-genotype 1 strains, are unlikely without new interventions and/or a vaccine Citation[12,13].
The characteristic of HCV that will offer the biggest problem for vaccine design is its viral variability. With sequence diversity believed to be 10 times that of human immunodeficiency virus (HIV), HCV strains are classified into 7 genotypes (numbered 1 – 7), which differ at 31 – 34% of their nucleotide positions, and which can be further divided into over 100 subtypes Citation[14,15]. This diversity is largely due to a lack of proof-reading capacity of the viral RNA-dependent polymerase (NS5b) used by HCV during replication; therefore, HCV exists within a host as a constantly evolving population of closely related but diverse quasispecies Citation[16].
Over the ∼1000 years HCV has been infecting humans, HCV genotypes have evolved in distinct geographical regions due to “neutral” sequence drift and by rapid adaptive changes due to immunological selection pressure Citation[17]. In recent decades epidemics of certain genotypes have spread through distinct risk groups, such as the epidemic of genotype 3a HCV amongst intravenous drug users (IVDUs) in the UK, and single-source outbreaks due to contaminated blood products Citation[3]. An effective prophylactic vaccine for HCV will need to effectively target these prevalent circulating viral genotypes and will need to cope with HCVs' inherent mutability.
2. The immune response to HCV
Comparative analysis of individuals with distinct clinical outcomes has been performed by several groups, and there is now some consensus on the immune response required to prevent persistence of HCV, but there is no clear correlate of protection (reviewed in Citation[18-20]). Most simply put, a strong, broad and persistent HCV-specific adaptive immune response during acute infection is required for clearance Citation[21]; however, in the face of such adaptive immune responses HCV persists in some patients, which likely reflects the importance of other antiviral mechanisms Citation[18,19,21].
2.1 Innate
As with other viral infections, the innate immune system – mediated by phagocytes, natural killer (NK) cells, complement and soluble antiviral factors, such as IFNs – has an important role in the control of HCV (reviewed in Citation[22]); however, HCV has been shown to suppress early innate immune responses by multiple mechanisms, most notably by altering the downstream effects of IFN expression or by blocking its production, and by down-regulation of NK activity Citation[23-26].
Recent genome wide association studies have highlighted the importance of innate host genes in the clearance of HCV. Single-nucleotide polymorphisms linked to the interferon-λ3 gene have been associated with spontaneous clearance of HCV and the genotype at this locus is the most powerful baseline predictor of an SVR in genotype 1 patients treated with standard of care Citation[27,28]; however, the overall role of IFNs in control of HCV infection remains unclear Citation[29].
Evidently, the innate immune response is involved in the effective control of HCV, but it is more difficult to manipulate practically for use in vaccines due to its lack of specificity; nevertheless, it is clear that the use of adjuvants or vectors that elicit an innate response is key in enhancing the adaptive immune response to vaccination.
2.2 Humoral responses
Neutralising antibodies provide the clearest correlate of protection for many viral infections, and generation of such antibodies is the basis of most successful vaccines Citation[30]. Furthermore, vaccines that generate protective antibody responses against HPV (human papillomavirus) and HBV (hepatitis B virus) demonstrate that prevention of chronic viral infection by antibody-inducing vaccination is possible Citation[31,32]. The relevance of antibody generation for control of HCV is complex for a variety of reasons.
Circulating antibodies against structural and non-structural (NS) regions of HCV develop in all patients, regardless of outcome, and a direct correlation between viral clearance and the rapid induction of high-titre cross-neutralising antibodies has been shown, but in most patients antibody responses are not neutralising or are isolate specific Citation[33-35]. Much of HCV sequence diversity is concentrated in areas of high variability, such as the major antibody target, hypervariable region 1 (HVR1) in E2, meaning antibodies often offer protection only against a single strain and are easily evaded by viral mutations Citation[14,36]. If vaccine-induced immunity to HCV is to be antibody driven, a strong and broadly cross-reactive response is needed to account for extensive global diversity and inherent mutability of the virus Citation[37-39].
Due to its hepatotropic nature, a mechanism for antibody evasion available to HCV is cell-to-cell spread via tight junctions, which are common between hepatocytes Citation[40]. It is also likely the glycosylated coating of HCV and its interactions with high-density lipoproteins are not only used in cell entry but also hinder antibody binding Citation[34].
A coordinated adaptive immune response involving both antibodies and T cells is normally required for pathogen control Citation[30]. Conceptually then, is it plausible that a vaccine inducing T cells alone can prevent persistent HCV infection? Several observations suggest that it can: chimpanzees and humans can clear HCV infection without a detectable antibody response and HCV-specific cellular immune responses can be detected in exposed uninfected persons without seroconversion Citation[34,41,42]. It has also been shown that hypogammaglobulinemic patients, deficient in antibody responses, can clear HCV Citation[43].
HCV uses multiple mechanisms to avoid antibodies, which can explain why in the face of a detectable antibody responses HCV can persist; this combined with mounting evidence that clearance can occur without the detection of antibodies suggests that although generation of effective antibody responses would be ideal, a vaccine against HCV need not necessarily induce HCV-specific antibodies.
2.3 Cellular responses
Comparative studies have shown that a functional early T-cell response of high magnitude, targeted at multiple major histocompatibility complex class I and II epitopes, is characteristic of effective immunity, and that conversely, the hallmark of persistent infection is a weak, narrowly targeted and dysfunctional T-cell response Citation[44-48]. Patients who go on to be chronically infected often do not lack a cell-mediated response initially, but there is evidence that the timing, persistence and functionality of the response are insufficient to control HCV Citation[21,48,49].
Some of the most convincing evidence for the importance of T cells in protection against HCV infection comes from chimpanzee studies in which antibody depletion of CD8 T cells lead to prolonged viraemia in convalescent chimpanzees that had previously cleared two rounds of infection Citation[50]; subsequent viral clearance was precisely correlated with a recovery of HCV-specific CD8 T cells Citation[50]. A complementary experiment depleting CD4 T cells again led to the abrogation of a previously protective immune response Citation[51]. Retention of an effective population of CD4 T helper cells appears to be a prerequisite for ongoing viral control, as shown by the reoccurrence of viraemia in individuals where CD4 responses wane, even after several months of apparent control Citation[19,21,52]. Kaplan et al. showed in their cohort of acutely infected patients that those who cleared HCV had a highly functional virus-specific CD4 response and broadly targeted IFNγ T-cell response, but that patients with CD8 T cells or neutralising antibodies alone did not clear HCV Citation[53]. In man, a drop in viral load and a rise in serum transaminase levels are often temporally linked to the emergence of CD4 and CD8 T-cell responses and increased intrahepatic IFNγ expression Citation[19,48,49,51].
Single-source outbreaks have shown a clear relationship between the patients' HLA type and the outcome of their infection, with HLA-B27, HLA-B57 and HLA-A3 being associated with protective responses, again emphasising the importance of effective antigen presentation and concomitant T-cell response in the clearance of HCV Citation[54-57].
It should be noted, however, that responses of similar breadth and magnitude to those affording protection from persistence in some patients have been seen in patients who go on to be chronically infected and there have been contrasting results when trying to correlate the magnitude of the intrahepatic cytotoxic T lymphocytes (CTL) and viraemia or outcome of infection Citation[33,45,58].
3. Current vaccine approaches
3.1 Recombinant protein vaccines
Several mechanisms have been investigated for the introduction of HCV-specific antigens to induce protective immunological memory (; ).
Figure 1. HCV genome structure and vaccine immunogens. (A) Organisation of the HCV genome: HCV, a single-stranded RNA virus of ∼ 9.5 kb, consists of a single open-reading frame and two untranslated regions. HCV is transcribed as a single polyprotein, which is cleaved by a host signal protease in the structural region and the HCV-encoded serine protease in the NS region. The hypervariable regions of E2 (HVR1 + HVR2) are indicated by dashed arrows. The protein products of cleavage are shown. The structural regions consist of core and the two envelope proteins, gp35 and gp76. The NS proteins are shown and their functions are described where known. (B) Prophylactic vaccines for HCV tested in primates (including man) are listed according to the lead author of the paper in which they are described. The relative coverage of the HCV genome by vaccine immunogen is shown. The genotype of the immunogen encoded in each vaccine is shown in parenthesis with the paper reference [].
![Figure 1. HCV genome structure and vaccine immunogens. (A) Organisation of the HCV genome: HCV, a single-stranded RNA virus of ∼ 9.5 kb, consists of a single open-reading frame and two untranslated regions. HCV is transcribed as a single polyprotein, which is cleaved by a host signal protease in the structural region and the HCV-encoded serine protease in the NS region. The hypervariable regions of E2 (HVR1 + HVR2) are indicated by dashed arrows. The protein products of cleavage are shown. The structural regions consist of core and the two envelope proteins, gp35 and gp76. The NS proteins are shown and their functions are described where known. (B) Prophylactic vaccines for HCV tested in primates (including man) are listed according to the lead author of the paper in which they are described. The relative coverage of the HCV genome by vaccine immunogen is shown. The genotype of the immunogen encoded in each vaccine is shown in parenthesis with the paper reference [].](/cms/asset/8119cc16-6003-420a-85d6-251ef7d7757a/iebt_a_791277_f0001_b.jpg)
Table 1. Primate and human studies describing candidate prophylactic HCV vaccines.
The genes encoding HCV viral proteins have been isolated and cloned into bacteria, yeast or mammalian cells, and the recombinant protein expressed purified for use in HCV vaccines. The advantage of recombinant protein vaccines is that they do not contain the pathogen or its genetic material and they do not require cultivation of the organism.
The first prophylactic vaccine candidate tested for HCV, T2S-918/InnoVac-C by Innogenetics, consisted of a C-terminally truncated recombinant E1 protein () with aluminium hydroxide (alum) adjuvant Citation[59]. This vaccine elicited antibody titres against E1 in healthy volunteers that were significantly higher than those seen in patients with persistent HCV infection, but Innogenetics ceased work on this vaccine in 2007 Citation[59].
The immunogenicity of E1 and E2 (with deletion of HVR1; ) was assessed as separate proteins, potentially uncovering new antibody targets not available in the natural heterodimeric form of E1E2 Citation[60]. After vaccination with either E1 or E2 adjuvanted with alum, antibodies were elicited in four chimpanzees, but only antibodies against E1 were shown to neutralise HCV pseudoparticles (HCVpp) and antibody titres declined after challenge Citation[60]. Chimpanzees were challenged with a 1b strain of HCV and only chimpanzees vaccinated with E1 were protected from viral persistence Citation[60].
Full-length heterodimeric E1E2 has also been tested () in vaccines and proved to be highly immunogenic, showing sterilising immunity in one study against a homologous HCV strain in five of seven chimpanzees Citation[61]. By combining results from chimpanzee vaccine studies using E1E2 glycoprotein it was shown to offer protection from persistent HCV infection in 10/12 and 8/9 chimpanzees when challenged with homologous or heterologous HCV strains, respectively Citation[62]. When this vaccine moved into Phase I human trials, strong antibody responses were detected by enzyme-linked immunosorbent assay Citation[63]; these antibodies could block viral E2 protein-binding CD81, a major entry receptor for HCV Citation[63]. Despite these promising results technical difficulties in the manufacture of E1E2 protein have hampered its use in vaccines.
Novartis is also pursuing a vaccine consisting of recombinant HCV core protein () produced in yeast, administered with a potent T-cell adjuvant immunostimulating complex matrix (IMX) Citation[64]. Promising results in rhesus macaques led to a Phase I dose escalation trial in 30 healthy volunteers, where all but one showed vaccine-induced antibodies against HCV core protein, but T cells were detectable in only 2 volunteers receiving a high dose of vaccine Citation[64]. Limited by the amount of vaccine available, doses higher than 50 µg of recombinant core protein have yet to be tested.
The use of whole heat-killed recombinant yeast that express targeted molecular immunogens (tarmogens) has also been assessed () Citation[65]. A core-NS3-5 fusion protein is encoded in the vaccine candidate GI-5005a and when combined with IMX and administered to five naïve chimpanzees a T-cell response was measurable in the liver and blood Citation[65]. Despite altered viral kinetics during the acute phase of infection in all vaccinated animals, relative to controls, none cleared HCV after challenge Citation[65].
3.2 Peptide vaccines
Synthetic HCV peptides have been used to induce T-cell immunity through direct presentation on antigen-presenting cells (). Peptide vaccines are HLA-specific and target only a selected subset of epitope sequences within HCV, limiting their breadth and coverage within the population but allowing closer control over the immunodominance hierarchy of vaccine responses.
Five synthetic HCV peptides containing T-cell epitopes (), administered with poly-L-arginine, make up the vaccine candidate IC41. When administered to 128 HLA-A2+ healthy volunteers in a Phase I study, IC41 was shown to be safe and immunogenic Citation[66]. Few IFNγ-producing cells were induced by IC41, as measured by IFNγ ELISpot (; median of 30 spot-forming cells [SFCs] per 106 peripheral blood mononuclear cells [PBMCs]) Citation[66].
Figure 2. Magnitude of T-cell response to vaccination. A comparison of the magnitude of vaccine-induced T-cell responses in primates is shown (median of peak response after vaccination as measured by IFNγ ELISpot). Vaccines contained HCV antigens unless otherwise stated. Successive vaccinations are separated by a forward slash, e.g., DNA/MVA refers to a vaccine regimen using DNA priming followed by an MVA boost. References in parenthesis [].
![Figure 2. Magnitude of T-cell response to vaccination. A comparison of the magnitude of vaccine-induced T-cell responses in primates is shown (median of peak response after vaccination as measured by IFNγ ELISpot). Vaccines contained HCV antigens unless otherwise stated. Successive vaccinations are separated by a forward slash, e.g., DNA/MVA refers to a vaccine regimen using DNA priming followed by an MVA boost. References in parenthesis [].](/cms/asset/d5d4c027-7b85-42f7-b93f-62c826257005/iebt_a_791277_f0002_b.jpg)
A further study testing the efficacy of this vaccine when administered subcutaneously or intradermally showed 65 – 100% of vaccinated healthy volunteers had lymphoproliferative responses to HCV proteins but again weak T-cell responses () Citation[67]. The use of the TLR7 agonist, Imiquimod, had no effect on vaccination Citation[67]. In the setting of chronic infection this vaccine caused a significant 0.47 log 10 drop in HCV RNA, but this did not correlate with the size of the T-cell response Citation[68,69]. The biotechnology company Intercell AG aims to enhance this approach by broadening the number of epitopes targeted and by investigating new adjuvants.
3.3 DNA vaccines
Injection of recombinant plasmids has been shown to result in effective protein expression in vivo and a subsequent immune response in mice, but this initial success has not yet been translated well into man. DNA uptake and gene expression decrease with the size of the immunised host; however, there has been much development in technologies to improve cell transfection, such as transdermal delivery with the gene gun, and in vivo electroporation (reviewed in Citation[70]).
Plasmids encoding HCV NS3/4a (ChronVac-c) or core/E1/E2 (CICGB-230) have shown some efficacy as potential therapeutic vaccines for HCV, but there is no published data on their effectiveness as prophylactic vaccines Citation[71,72].
3.4 Vector-based vaccines
Over the last decade great advances in molecular virology have enabled the manipulation of viruses for delivery of foreign genetic material to mammalian cells (; ) Citation[73-79]. Their highly evolved mechanisms for cell entry and gene expression within the host cell remain intact and viral vectors can be rendered non-pathogenic and non-replicative by deletions at specific locus Citation[80]. Some viral vectors at the earliest stages of testing as delivery vehicles for HCV genetic material include alphaviruses, canary pox, ovine atadenovirus and semliki-like viral particles Citation[81-84].
Virus-like particles (VLPs) are attractive vectors for gene delivery as they mimic the properties of native virions, are safe and are easily manufactured. VLPs encoding the HCV core-E1E2 genes () induced a large HCV-specific T-cell population in chimpanzees () and all four vaccines cleared challenge with homologous strain of HCV Citation[85]. Surprisingly, no HCV-specific antibody response was detected Citation[85].
Adenoviral (Ad) vectors are the best characterised viral vectors and have emerged as the most potent at T-cell priming in non-human primates (NHPs) and humans Citation[86]. Ad-based vaccines are particularly attractive gene vehicles as they can stably express large foreign inserts (∼10 kbp), they remain epichromosomal and can be easily rendered replication defective by deletion of the E1 locus Citation[80,87].
The major limitation with the use of adenoviruses is that pre-existing immunity to the vector can lead to its clearance before a response is elicited to the inserted immunogen Citation[86]; this can in part be overcome by the use of rare subtypes that are of low seroprevalence, or the use of adenovirus with altered surface proteins Citation[86]. An extensive study of chimpanzee adenoviruses by researchers at Okairos (Rome, Italy) isolated over a thousand strains and showed that their immunogenicity in mice varied widely Citation[88]. Chimpanzee adenovirus 3 (ChAd3) and Ad6 were selected for analysis in human and animal trials, with the whole NS region of HCV (genotype 1b, BK strain) Citation[76].
Support for the use of Ad-based vaccines to induce protective T-cell populations in man came from a therapeutic trial in which chimpanzees received heterologous Ad-Ad followed by further boosting with electroporated DNA, all containing the NS region of HCV () Citation[74]. All vaccinated chimpanzees produced a high-magnitude T-cell response (; peak total anti-HCV responses ranged from 615-2509 SFC/106 PBMCs and 1108-7678 SFC/106 PBMCs for CD4 and CD8 T cells, respectively) and when challenged with a heterologous HCV strain four of five cleared the virus Citation[74]. Vaccinated animals showed strong anamnestic responses that resulted in a blunted peak viraemia and shorter period of infection relative to controls Citation[74]. Prime-boost vaccination with heterologous adenovirus containing HCV NS also induced a large and broadly targeted HCV-specific T-cell response in rhesus macaques () Citation[75].
On the strength of this preclinical data the Ad vectors ChAd3 and Ad6 were tested in a Phase I clinical trial in healthy volunteers Citation[76]. All 10 patients receiving the highest dose of Ad responded to vaccination, with peak T-cell responses averaging over 1000 SFC/106 PBMCs (; range 443 – 4263). However, boosting with heterologous Ad in healthy volunteers was not as effective as predicted from the results in rhesus macaques, which is likely due to higher levels of cross-reactive antibodies against the Ads in humans Citation[75,76].
Modified vaccinia Ankara (MVA) is another attractive vaccine vector due to its excellent safety record and immunogenicity in man. It has been shown to be particularly effective as a boosting vector, broadening and increasing the magnitude of pre-existing T-cell responses Citation[86,89-92].
A heterologous prime-boost regimen with DNA and MVA encoding core-E1-E2 () and NS3 was tested in naïve chimpanzees by TRANSGENE Citation[73]. A large T-cell response was seen post-vaccination by IFNγ and IL-4 ELISpot (), but proliferative responses were transient and a high expression of IDO, CTLA-4 and PD-1 on HCV-specific T cells after challenge suggested that induced T cells could have been dysfunctional Citation[73]. Three of four went on to be chronically infected when challenged with a heterologous J4 strain of HCV Citation[73].
Using the malaria antigen ME.TRAP, priming vaccination with Ad elicited similar T-cell responses to Ad encoding HCV NS, and these responses were boosted 3.1 – 5.2-fold by vaccination with MVA containing ME.TRAP () Citation[92]. Building on the results outlined above, a trial to assess the safety and efficacy of a prime-boost regimen using ChAd3 boosted with MVA encoding HCV NS in patients and healthy volunteers is ongoing Citation[93]. This Ad/MVA vaccine regime has also progressed to a Phase II study, which will take place in an intravenous drug using community in Baltimore Citation[94]. This study will be the first double-blinded, randomised, placebo-controlled trial of a vaccine to prevent HCV persistence. The trial will enrol 350 subjects and is set up to assess whether this regimen can enhance the rate of spontaneous resolution of HCV infection.
A single meta-analysis has compared the overall outcome of HCV infection between differing prophylactic vaccine studies in chimpanzees, and also performed a comparison between naïve, re-challenged and vaccinated animals Citation[5]. The authors show that immunological memory after prior viral clearance, or vaccination, leads to a reduction in peak HCV RNA titre and increased HCV clearance rates after viral challenge Citation[5]. Additionally, the study dissects the outcome of infection after vaccination according to whether or not the vaccine included NS or structural regions of HCV, concluding that vaccines containing only structural antigens were more efficacious Citation[5]; however, these data should be interpreted with caution, since a large proportion of animals were vaccinated with the E1E2 recombinant protein heterodimer (Novartis; Citation[62,95]), and differences in the challenge strain and dose of virus between studies complicate the comparative analysis Citation[5]. Examples of protective and non-protective vaccines can be found for vaccines containing structural antigens, NS antigens or both, and more research is needed into which antigens offer the best protection Citation[73,74,78,95].
4. What is a protective T-cell response?
Several methodologies are progressing to clinical studies in humans but what do we know about the type of immune response offering protection from persistent HCV infection, and how do responses to the current vaccine candidates measure up against this ideal?
4.1 Magnitude
The most fundamental characteristic of a T-cell response is its magnitude, as measured directly by the frequency of antigen-specific T cells or by the number displaying a certain effector function, most commonly production of IFNγ.
Although no defined cut-off for a protective response against HCV exists, responses in individuals who clear acute infection are typically in the region of a few hundred IFNγ-producing cells per million PBMCs, and the responses often remain detectable for many years after infection Citation[21,53,74,96,97].
Prospective vaccines against HCV have induced T-cell populations with a wide range of magnitudes (). One of the few vaccines tested in humans for which T-cell responses were measured, IC41, had a median response of only 30 SFC/106 PBMCs (range 15 – 185) Citation[67]. The largest magnitude HCV-specific T-cell responses seen in animal models and humans have come from regimens using Ad vectors (). The responses to Ad6-ChAd32 heterologous prime-boost had an average of 4924 SFC/106 PBMCs in rhesus macaques and 1202/1400 SFC/106 PBMCs in the two Ad-Ad regimes tested in humans () Citation[75,76].
Although vaccines that induce high levels of HCV-specific T cells in animal models have protected against persistent HCV infection, this is not always the case; T-cell responses as high as 2368 SFC/106 PBMCs in chimpanzees vaccinated with DNA and boosted with MVA were not protective against challenge with a heterologous strain of HCV, highlighting the importance of other factors, in particular the functionality and antigen specificity of the vaccine-induced T cells Citation[5,73].
With the development of viral vectors we now have the means to induce large numbers of antigen-specific and IFNγ-producing T cells. However, it is apparent that the magnitude of the T-cell response alone is a poor predictor of protection for many viral infections and that an understanding of the combination of functions possessed by T-cell populations is needed Citation[91,98-101]. Several T-cell parameters of importance for vaccine design are discussed below.
4.2 Breadth
The selection pressure exerted by the adaptive immune response rapidly selects for escape variants, leading to persistence of HCV strains that are unrecognisable by circulating T cells and antibodies in both natural infection of humans and in animal infection models Citation[36,102-104]. The breadth of a T-cell response has been reproducibly associated with control in human correlative studies Citation[21,45,96,97]. For an effective HCV vaccine, a T-cell response targeting multiple epitopes may be required to limit the possibility of viral escape and to effectively block viral replication Citation[45,96,97].
The breadth of the T-cell response to vaccination is dependent upon the size and specificity of the immunogen (), and vaccine studies to date have induced T-cell responses with a wide variation in breadth. The breadth of the response using a peptide vaccine will be restricted to the HLA repertoire of the vaccinated population, whereas a virally vectored vaccine approach may induce a broadly targeted T-cell response on a diverse HLA background population Citation[66,76].
As well as targeting multiple HCV epitopes, an effective global vaccine will also need to target multiple viral genotypes Citation[14]. Using HCVpp recombinant E1E2 protein vaccines induced antibodies with cross-reactivity against genotypes 1a, 1b and 2a Citation[105]. Barnes et al. showed that response to Ad-Ad vaccination containing the NS region from a 1b BK strain recognised peptides from genotypes 1a, and to a more limited extent, 3a, the most prevalent genotypes in Europe and America Citation[76].
4.3 Proliferation and cytokine production
A dysfunction in the T-cell proliferative capacity has been repeatedly identified in those who fail to control HCV relative to those who clear Citation[46-48,106]. Good proliferative capacity is key for memory responses induced by prophylactic vaccination, as a rapid and large expansion of secondary effector T cells is needed during recall responses. Lymphoproliferative responses to HCV proteins have been seen in several HCV vaccine studies Citation[59,63,66,74,76,85].
Intracellular cytokine staining is used to assess the production of several cytokines relevant to viral control by antigen-stimulated T cells. The production of IFNγ and TNFα is often measured due to their direct antiviral effect, as well as IL-2, which promotes the clonal expansion of T-cell populations on activation and influences differentiation of T-cell subsets. These cytokines are often measured in conjugation with CD107α (lysosome-associated membrane protein-1), a marker of T-cell degranulation, and the pro-inflammatory cytokine MIP-1-β (macrophage inflammatory protein-1β; CCL4).
It has been assumed, but not convincingly shown, that a polyfunctional T cell, with the capacity to carry out multiple antiviral functions, is more effective at clearing a virus than a monofunctional T cell; evidence of this came from comparative studies of long-term non-progressors (LTNPs) and progressors in the setting of HIV infection, and mouse studies of Leishmania major Citation[99,107]. The T-cell population in LTNPs, relative to progressors, is enriched for single CD8 T cell that can co-produce MIP-1-β, TNFα, IFNγ and CD107α Citation[98]. But these highly polyfunctional T cells are lacking in some LTNPs, when present represents a small fraction of the total HIV-specific T-cell population, and could simply be an indicator of low antigenic load in these individuals Citation[98,107]. Polyfunctionality not only affords a larger repertoire of functions for the individual cell, but it can also mean a larger per-cell production of the cytokine relative to monocytokine producers (e.g., in some studies monocytokine-producing T cells made 10× less IFNγ per cell than polyfunctional T cells) Citation[99,108-110].
Evidence of a hierarchy in cytokine production is emerging, where increased antigen exposure and co-stimulation lead to an increase in functions expressed by a T cell Citation[111-114]. Viola et al. showed that MIP-1-β and IFNγ are most readily released by T cells on limited stimulation and that IL-2 production is only triggered when a T cell has been exposed to high levels of antigen and co-stimulation Citation[114]. The antigenic load resulting from vaccination is highlighted as a key attribute that will affect T-cell quality and vaccine efficacy. This hierarchy is evident in vaccine responses elicited by Ad-Ad and Ad-MVA vaccination, and polyfunctionality is a characteristic of T-cell responses to these vector combinations Citation[76,92].
4.4 Cytotoxicity
One functional attribute of human CD8 T cells that unequivocally combats acute viral infections is cytotoxicity Citation[21,115]. Direct cytotoxic killing of infected cells is primarily mediated by the activation of apoptotic pathways within the target cell by granzyme cleavage of intracellular caspases Citation[116]. On recognition of an infected cell, activated CTL secrete lysosomes containing the pore-forming protein perforin and granzymes, which are delivered to the target cell inducing its apoptosis Citation[117,118].
Rather than its ex vivo expression, the rapid up-regulation of perforin is a key effector function of T cells, and one that appears to be lacking in HCV-specific T cells taken from chronically infected patients Citation[98,119,120]. Although it has been shown that during chronic infection HCV-specific CD8 T cells are often deficient in perforin expression and up-regulation relative to CMV-specific populations, it remains unclear how much perforin is necessary to initiate granzyme-mediated killing Citation[120].
Surface mobilisation of CD107α has been used to identify cells that have degranulated on peptide stimulation and, although this assay does not show killing directly, or give information on the content of the granules released, it can be combined with staining for granzymes and perforin to give an indication of the cytolytic potential of a T cell Citation[121].
Few trials have assessed the cytolytic capacity of vaccine-induced CTL, but it remains a key parameter that should be assessed in any vaccine aiming to mediate protection through induction of T cells. After vaccination with heterologous Ad-Ad encoding the NS region of HCV, the vast majority of HCV-specific T cells express both granzyme A and B, and often high levels of perforin Citation[76]. It was also shown that these cells produced CD107α on peptide stimulation, showing that they have the capacity to kill HCV-infected cells Citation[76].
4.5 Phenotype
Research over the last decade has revealed an ever increasing complexity and division of labour within T lymphocytes, particularly within the memory compartment Citation[101,122-124].
Antigen-experienced cells in humans express the short, CD45RO, form of the protein tyrosine phosphatase CD45, and the long form, CD45RA, is expressed by naïve T cells and is re-expressed by a subset of CD8 T cells Citation[125]. In combination with the lymph node homing receptor CCR7, four broad populations of T cells can be described Citation[122]: Central memory T cells (Tcm; CD45RA−, CCR7+) that home to the lymph nodes and have limited effector function but high proliferative capacity; Effector memory T cells (Tem; CD45RA−,CCR7−) that show immediate effector and cytolytic function and circulate peripheral tissues; naïve, antigen-inexperienced T cells (CD45RA+, CCR7+); “terminally differentiated” effector memory T cells (Temra; CD45RA+, CCR7−) that have re-expressed CD45RA.
Additional complexity has been found in mice studies that have tried to identify precursors of long-lived memory cells in the effector pool. Effector T cells have been divided into memory precursor effector cells and short-lived effector cells by their expression of CD127 (IL-7Rα), and KLRG1 and a population of T cells with stem cell-like properties have been described (Tscm) Citation[126-128].
Rather than being a trivial pursuit, the description of these subsets of T cells has led to the identification of certain subsets as being the main mediators of protection after vaccination Citation[91,129]. An Ad-MVA heterologous prime-boost regimen encoding malaria antigens preferentially induced antigen-specific Tem that mediated protection against challenge with malaria sporozoites, as confirmed by transfer experiments Citation[91]. Tem were also shown to mediate protection against SIV challenge in rhesus macaques Citation[129].
It is now clear T cells play a key role in clearance of HCV, but we are lacking information about which subsets mediate this control and which subsets we should aim to elicit by vaccination. It is likely that a mixed population of lymph-node homing Tcm, with the potential to rapidly proliferate and differentiate into effector T cells, along side a population of Tem, which circulate the periphery and have a more immediate effector function, would in theory be most effective.
The vaccine platform used to deliver immunogens has a profound influence on the type of T-cell response elicited, due to differences in the innate signalling pathways stimulated and the persistence and amount of antigen after vaccination Citation[86,91,129,130].
Low levels of transcriptionally active Ad have been shown to persist long term at the site of vaccination, in the liver and in lymphatics, after vaccination with Ad in mice and primates; however, transgene expression by MVA becomes undetectable after ∼ 2 days Citation[131]; this may explain why MVA vaccination is characterised by the induction of Tcm, whereas a single Ad induces and maintains an active Tem population, as well as developing Tcm and Temra Citation[91,131]. It is clear the number of antigen encounters, or vaccinations, also influences the extent of T-cell differentiation Citation[132]. For example, repeated stimulation by antigen can lead to a larger population of Tem and Temra but fewer Tcm Citation[133].
Barnes et al. describe the phenotypic properties of T cells induced by heterologous Ad-Ad vaccination encoding the NS region of HCV Citation[76]. Vaccine-induced CD8 T cells peaked in magnitude 2 weeks post Ad priming, and subsequently contracted to a memory T-cell population in which a significant proportion had down-regulated PD-1, and re-expressed CD127 Citation[76]. A key feature of the CD8 T cells induced by Ad encoding the NS region of HCV is the re-expression of CD45RA, giving a mixed population consisting of Temra > Tem > Tcm Citation[76]. This phenotype is remarkably similar to that of the T cells induced by the highly efficacious vaccines for yellow fever and smallpox (Dryvax), which have excellent safety records in humans and elicit large T-cell responses that are associated with life-long protection Citation[134].
The use of classic phenotypic markers has under-represented the complexity of T-cell phenotypes, but the advent of sophisticated cytometric techniques (i.e., multiparametric flow cytometry, cytometry by time-of-flight, and gene expression profiling) capable of analysing multiple T-cell parameters simultaneously may enhance the stratification of T-cell subpopulations by function Citation[101,130,135]; for example, a recent study described phenotypically identical T-cell populations induced by different vaccine regimens that were distinct when the cell transcriptome was assessed Citation[130].
Three prime-boost regimens encoding HIV Env gene were compared by Flatz et al. (DNA-Ad, Ad-Ad and Ad-rLCMV [recombinant Lymphocytic choriomeningitis virus]) and despite inducing T-cell populations that were similar in magnitude, cytokine production and Tem/Tcm phenotype, the gene expression profile of the cells induced by different regimens was distinct Citation[130]. Using linear discriminant analysis, antigen-specific T cells clustered separately for each regimen and there were noticeable differences in expression in senescence and homing markers (e.g., Eomes, CCR7, CXCR3, CCR5, KLRG1 and Klrk1) Citation[130]. Therefore, even using the magnitude, cytokine production and classic phenotyping may not be sufficient to identify correlates of protection because they are insensitive to the full extent of heterogeneity in CD8 responses Citation[130].
Clearly, a better understanding of the division of labour between T-cell subsets and the steps involved in T-cell differentiation and memory formation should help identification of correlates of protection for viral pathogens.
5. Conclusion
The development of a vaccine capable of preventing chronic HCV infection remains the most cost-effective and realistic method of controlling HCV globally, and it would find a target population in at-risk groups in developed countries and in entire populations in many developing countries Citation[13,136].
The prospects for a HCV vaccine have improved greatly in the last decade and there is now strong evidence that HCV is highly amenable to a prophylactic T-cell vaccine. Evidence from secondary infections in patients and from vaccine studies in humans and NHP show that immunological memory can protect against persistence of HCV and therefore disease, and this protection appears to be prominently mediated by cellular immunity Citation[4-6,137].
We now understand that clearance of HCV can occur without the induction of a measurable antibody response; however, vaccine-induced neutralising antibodies, when targeting circulating virus, also show effective control of HCV Citation[60,95]. It is likely that a T-cell-based vaccine will show enhanced efficacy when combined with a vaccine that induces cross-reactive antibodies.
With the development of novel DNA delivery systems, viral vectors and VLPs we now have the tools to induce large antigen-specific T-cell populations () and we are developing adjuvants to enhance these further and to tailor the types of T-cell subsets induced by vaccination.
We now know that different subtypes of T cell exist, with different functionalities, and that these subsets offer different levels of protection against specific pathogens. As this understanding develops further we will be able to better asses and manipulate vaccine-induced T-cell populations. To do this it is essential that we better describe the T-cell parameters associated with control of HCV.
6. Expert opinion
A vaccine for the prevention of HCV infection would address a huge unmet clinical need globally. In developed countries we would envisage that an effective prophylactic vaccine would be administered to at-risk populations, but in large areas of Africa and Asia, where prevalence rates are high, a universal vaccination strategy would be optimal. It is this need that has driven an intense research program in HCV vaccinology over the last 15 years.
Detailed studies of natural infection have shown that complete HCV viral control is attainable through effective host anti-viral immunity. Multiple lines of evidence point to the crucial role played by host T-cell immunity in viral control, in addition to host innate immune genes. Effective humoral immunity has been less clearly associated with protection – nevertheless, a vaccine that induced appropriate B-cell responses may improve vaccine efficacy. Over the last decade numerous vaccine strategies have been assessed in small animal and primate models. Broadly these include HCV envelope protein vaccines designed to generate protective humoral responses, peptide vaccines that are restricted by host HLA, and more recently DNA and virally vectored approaches (). DNA vaccination is capable of generating robust T-cell immunity, but requires additional delivery mechanisms such as electroporation. Virally vectored vaccines have recently reached Phase II studies and show immense promise when used alone, or in the future alongside other approaches ().
To date, the exact correlates of immune protection against persistent HCV infection have not been clearly defined, and indeed this may never be achieved given the heterogeneity contained within small study populations of patients with primary infection and the real limitations of animal models of HCV infection. However, in broad terms, the generation of CD4+ and CD8+ T cells that target multiple HCV antigens at a high magnitude, and that persist over months to years, is seen in humans that control viraemia after primary infection, and also in primate prophylactic T-cell vaccine studies Citation[44-48,74,75,77,78]. Therefore, current vaccine strategies should at least aim to recapitulate these findings in Phase I human studies before efficacy testing. Ideally a potent T-cell vaccine would be combined with an antigen capable of generating broadly cross-reactive neutralising antibodies against HCV envelope. However, currently this is not technically feasible.
Current vaccine approaches are capable of inducing T-cell immunity of a breadth and magnitude that has been associated with viral clearance in humans. However, we do not know if these T cells possess all the “qualities” that are required for long-term viral control, since these qualities are not absolutely defined. This presents a real limitation in the design, assessment and ranking of vaccine candidates. Natural history studies that identify T-cell parameters associated with viral control in the context of HCV and also other pathogens will contribute to our understanding in this area (). However, Phase II studies of efficacy testing in humans may be required to further define immune correlates of protection, for optimal vaccine generation in the laboratory – a process termed “reverse vaccinology” ().
Figure 3. Progress to an effective prophylactic vaccine against HCV. A summary of some of the key interactions between basic research and vaccine studies is shown. Natural history studies of HCV infection are used to better understand the immune correlates of protection against HCV infection; this understanding informs all levels of vaccine design, in particular the design of vaccines for preclinical and Phase I studies and at the selection stages when assessing efficacy of candidate vaccines. Cohorts of at-risk populations need to be characterised before candidate vaccines can be tested in Phase II/III studies and so this work should be done in parallel with early-stage vaccine assessment. Phase II/III studies of vaccine efficacy may be required to further define correlates of protection for optimal vaccine generation – a process termed “reverse vaccinology”. Basic research into vaccine modalities, adjuvants and the biology of T and B cells can be fed into the process of vaccine development at all stages to allow us to better design, assess and implement novel vaccines.
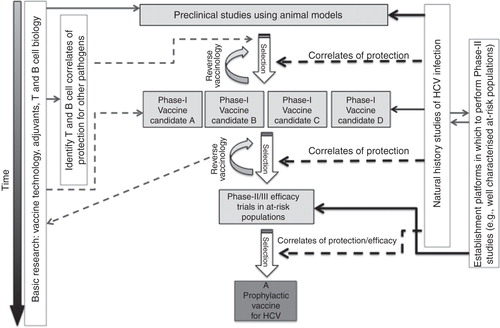
We believe that in order to now advance the field, comparative Phase I studies in human trials that address the magnitude, breadth and functionality of T and B cells induced by leading vaccine candidates, incorporating a range of immunogens, should be developed. Ultimately, Phase II studies assessing efficacy of the most promising regimens will be required. Since efficacy studies are logistically challenging, consortiums of investigators that care for well characterised at-risk cohorts (e.g., IVDUs and men who have sex with men) could be established now. If this approach is taken then a platform will be in place for efficacy testing of the most promising vaccine candidates when required (). These studies would assess the change of incidence of primary HCV infection that progresses to chronic disease. A placebo-controlled, Phase II study assessing vaccine efficacy using these principles in IVDUs is currently underway in Baltimore, USA, and provides proof of principle that this kind of study is now feasible Citation[94]. This approach can move forward in parallel with laboratory studies that aim to improve small animal models of HCV and to further define the correlates of immune protection in natural history studies.
Another challenge in the field is the generation of cross-reactive immune responses that are capable of protecting people from diverse HCV strains. This may be less of an issue in populations where only one viral genotype commonly circulates, though even in this case there is considerable diversity between individuals infected with the same HCV genotype. However, in many countries multiple viral genotypes circulate, for example in the United Kingdom approximately 50% of people are infected with genotypes 1a or 1b and 50% with genotype 3. Ideally then, an effective vaccine would be capable of protecting from multiple HCV strains. This may be achieved through administering more than one genotype-specific vaccine at a population level, or through the design of immunogens within a single vaccine that target multiple genotypes.
The HCV research community can be proud of the progress made over the last two decades in understanding HCV pathogenesis through in vitro replication models, and in the recent advances in the treatment of HCV. In the next decade we will see the implementation of multiple new DAAs that will cure many of those already infected. However, these treatments will come at a significant financial burden, and will be unavailable to most, either because infected people exist in resource poor settings, or because people are unaware that they are infected. Furthermore, these drugs are least effective in people with advanced disease who need them most. For these reasons we believe that the adage “prevention is better than cure” holds true for HCV today.
This, combined with new vaccine technologies that are really capable of delivering potent anti-HCV viral immunity, argues for major new investment in HCV vaccine Phase I, and efficacy studies. This investment will require not only significant financial resourcing, but also appropriate infrastructure and collaborative working between investigators that care for carefully characterised patient and “HCV at-risk” cohorts, across national boundaries. If these practical steps are taken, a preventative vaccine for HCV can be achieved.
Article highlights.
There is currently no approved vaccine for HCV, which is a major global health problem, newly infecting ∼ 3 – 4 million people annually worldwide.
HCV persists in up to 70 – 80% of immune-competent hosts it infects, leading to a state of chronic hepatic inflammation that can progress to liver failure or hepatocellular carcinoma.
The goal for a prophylactic vaccine against HCV is unique in that to prevent disease it need only prevent persistence of the virus, rather than prevent infection.
Antibody responses to HCV are often strain specific or do not neutralise circulating HCV strains because they target highly variable sequences.
We now know that a small but significant proportion of humans and NHPs spontaneously clear HCV and immunological memory can provide protection on reinfection. Initial clearance of HCV and protection against persistence in subsequent infections appear to be prominently mediated by cellular immunity.
Current HCV vaccine approaches include peptide, plasmid DNA, recombinant proteins and vector-based vaccines.
Virally vectored vaccines are the most promising in terms of T-cell induction – in particular Ad and MVA vectors.
There is an ever-increasing complexity seen within T lymphocyte populations and phenotype has been linked to functionality and protection; therefore, an understanding of the combination of functions possessed by the T-cell population is needed to effectively assess vaccine efficacy.
Parameters of interest when assessing a vaccine-induced HCV-specific T-cell population include breadth, cytokine production, cytolytic capacity, magnitude, phenotype and proliferative capacity.
New technologies capable of analysing multiple T-cell parameters simultaneously may enhance the stratification of T-cell subpopulations by function, allowing identification of better correlates of protection for HCV.
A major challenge in the next era of HCV vaccinology will be the assessment of vaccine efficacy in well-characterised at-risk populations.
Declaration of interest
E Barnes is funded by the Medical Research Council (MRC) UK, the Oxford NIHR Biomedical Research Centre and the James Martin School. Leo Swadling is funded by the MRC UK and Paul Klenerman is funded by the MRC UK, the Oxford NIHR Biomedical Research Centre and the James Martin School. The authors are currently working on Phase I HCV vaccine studies using adenoviral and MVA vectors in collaboration with an industrial partner Okairos. The authors have no other relevant affiliations or financial involvement with any organisation or entity with a financial interest in or financial conflict with the subject matter or materials discussed in the manuscript apart from those disclosed. No writing assistance was utilised in the production of this manuscript.
Notes
This box summarises key points contained in the article.
Bibliography
- Lauer GM, Walker BD. Hepatitis C virus infection. N Engl J Med 2001;345:41-52
- WHO. WHO fact sheet: HCV vaccines number 164. 2012. Available from: http://www.who.int/mediacentre/factsheets/fs164/en/ [Last] [accessed 07 February 2013]
- Lavanchy D. Evolving epidemiology of hepatitis C virus. Clin Microbiol Infect 2011;17:107-15
- Osburn WO, Fisher BE, Dowd KA, et al. Spontaneous control of primary hepatitis C virus infection and immunity against persistent reinfection. Gastroenterology 2010;138:315-24
- Dahari H, Feinstone SM, Major ME. Meta-analysis of hepatitis C virus vaccine efficacy in chimpanzees indicates an importance for structural proteins. Gastroenterology 2010;139:965-74
- Mehta SH, Cox A, Hoover DR, et al. Protection against persistence of hepatitis C. Lancet 2002;359:1478-83
- Thomas D, Zoulim F. New challenges in viral hepatitis. Gut 2012;61:i1-5
- Suneetha PV, Pischke S, Schlaphoff V, et al. Hepatitis E virus (HEV)-specific T-cell responses are associated with control of HEV infection. Hepatology 2012;55:695-708
- Manns MP, Wedemeyer H, Cornberg M. Treating viral hepatitis C: efficacy, side effects, and complications. Gut 2006;55:1350-9
- Welsch C, Jesudian A, Zeuzem S, Jacobson I. New direct-acting antiviral agents for the treatment of hepatitis C virus infection and perspectives. Gut 2012;61:i36-46
- Halfon P, Locarnini S. Hepatitis C virus resistance to protease inhibitors. J Hepatol 2011;55:192-206
- Van Den Berg C, Smit C, Van Brussel G, et al. Full participation in harm reduction programmes is associated with decreased risk for human immunodeficiency virus and hepatitis C virus: evidence from the Amsterdam Cohort Studies among drug users. Addiction 2007;102:1454-62
- Hahn JA, Wylie D, Dill J, et al. Potential impact of vaccination on the hepatitis C virus epidemic in injection drug users. Epidemics 2009;1:47-57
- Simmonds P. Genetic diversity and evolution of hepatitis C virus–15 years on. J Gen Virol 2004;85:3173-88
- Murphy DG, Willems B, Deschenes M, et al. Use of sequence analysis of the NS5B region for routine genotyping of hepatitis C virus with reference to C/E1 and 5′ untranslated region sequences. Hepatology 2007;46:623a
- Farci P, Shimoda A, Coiana A, et al. The outcome of acute hepatitis C predicted by the evolution of the viral quasispecies. Science 2000;288:339-44
- Pybus OG, Barnes E, Taggart R, et al. Genetic history of hepatitis C virus in East Asia. J Virol 2009;83:1071-82
- Ishii S, Koziel MJ. Immune responses during acute and chronic infection with hepatitis C virus. Clin Immunol 2008;128:133-47
- Cooper S, Erickson AL, Adams EJ, et al. Analysis of a successful immune response against hepatitis C virus. Immunity 1999;10:439-49
- Klenerman P, Thimme R. T cell responses in hepatitis C: the good, the bad and the unconventional. Gut 2012;61:1226-34
- Lechner F, Wong DK, Dunbar PR, et al. Analysis of successful immune responses in persons infected with hepatitis C virus. J Exp Med 2000;191:1499-512
- Randall RE, Goodbourn S. Interferons and viruses: an interplay between induction, signalling, antiviral responses and virus countermeasures. J Gen Virol 2008;89:1-47
- Gale M, Foy EM. Evasion of intracellular host defence by hepatitis C virus. Nature 2005;436:939-45
- Li K, Lemon SM. Innate immune responses in hepatitis C virus infection. Semin Immunopathol 2013;35:53-72
- Cheent K, Khakoo SI. Natural killer cells and hepatitis C: action and reaction. Gut 2011;60:268-78
- Khakoo SI, Thio CL, Martin MP, et al. HLA and NK cell inhibitory receptor genes in resolving hepatitis C virus infection. Science 2004;305:872-4
- Naggie S, Osinusi A, Katsounas A, et al. Dysregulation of innate immunity in hepatitis C virus genotype 1 IL28B-unfavorable genotype patients: impaired viral kinetics and therapeutic response. Hepatology 2012;56:444-54
- Kelly C, Klenerman P, Barnes E. Interferon lambdas: the next cytokine storm. Gut 2011;60:1284-93
- Schreiber J, Moreno C, Garcia BG, et al. Meta-analysis: the impact of IL28B polymorphisms on rapid and sustained virological response in HCV-2 and -3 patients. Aliment Pharmacol Ther 2012;36:353-62
- Burton DR. Antibodies, viruses and vaccines. Nat Rev Immunol 2002;2:706-13
- Kwak K, Yemelyanova A, Roden RB. Prevention of cancer by prophylactic human papillomavirus vaccines. Curr Opin Immunol 2011;23:244-51
- Zuckerman JN. Protective efficacy, immunotherapeutic potential, and safety of hepatitis B vaccines. J Med Virol 2006;78:169-77
- Freeman AJ, Marinos G, Ffrench RA, Lloyd AR. Immunopathogenesis of hepatitis C virus infection. Immunol Cell Biol 2001;79:515-36
- Logvinoff C, Major ME, Oldach D, et al. Neutralizing antibody response during acute and chronic hepatitis C virus infection. Proc Natl Acad Sci USA 2004;101:10149-54
- Pestka JM, Zeisel MB, Blaser E, et al. Rapid induction of virus-neutralizing antibodies and viral clearance in a single-source outbreak of hepatitis C. Proc Natl Acad Sci USA 2007;104:6025
- Hahn von T, Yoon JC, Alter H, et al. Hepatitis C virus continuously escapes from neutralizing antibody and T-cell responses during chronic infection in vivo. Gastroenterology 2007;132:667-78
- Law M, Maruyama T, Lewis J, et al. Broadly neutralizing antibodies protect against hepatitis C virus quasispecies challenge. Nat Med 2008;14:25-7
- Perotti M, Mancini N, Diotti RA, et al. Identification of a broadly cross-reacting and neutralizing human monoclonal antibody directed against the hepatitis C virus E2 protein. J Virol 2008;82:1047-52
- Meunier J-C, Russell RS, Goossens V, et al. Isolation and characterization of broadly neutralizing human monoclonal antibodies to the e1 glycoprotein of hepatitis C virus. J Virol 2008;82:966-73
- Brimacombe CL, Grove J, Meredith LW, et al. Neutralizing antibody-resistant hepatitis C virus cell-to-cell transmission. J Virol 2011;85:596-605
- Post JJ, Pan Y, Freeman AJ, et al. Clearance of hepatitis C viremia associated with cellular immunity in the absence of seroconversion in the hepatitis C incidence and transmission in prisons study cohort. J Infect Dis 2004;189:1846-55
- Abdelwahab SF, Zakaria Z, Sobhy M, et al. Hepatitis C virus-multispecific T-cell responses without viremia or seroconversion among egyptian health care workers at high risk of infection. Clin Vaccine Immunol 2012;19:780-6
- Semmo N. Maintenance of HCV-specific T-cell responses in antibody-deficient patients a decade after early therapy. Blood 2006;107:4570-1
- Zhang X, Dou J, Germann MW. Characterization of the cellular immune response in hepatitis C virus infection. Med Res Rev 2009;29:843-66
- Urbani S, Amadei B, Fisicaro P, et al. Outcome of acute hepatitis C is related to virus-specific CD4 function and maturation of antiviral memory CD8 responses. Hepatology 2006;44:126-39
- Wedemeyer H, He XS, Nascimbeni M, et al. Impaired effector function of hepatitis C virus-specific CD8+ T cells in chronic hepatitis C virus infection. J Immunol 2002;169:3447-58
- Diepolder HM, Zachoval R, Hoffmann RM, et al. Possible mechanism involving T-lymphocyte response to non-structural protein 3 in viral clearance in acute hepatitis C virus infection. Lancet 1995;346:1006-7
- Gerlach JT, Diepolder HM, Jung MC, Gruener NH. Recurrence of hepatitis C virus after loss of virus-specific CD4+ T-cell response in acute hepatitis C. Gastroenterology 1999;117:933-41
- Cox AL, Mosbruger T, Lauer GM, et al. Comprehensive analyses of CD8+ T cell responses during longitudinal study of acute human hepatitis C. Hepatology 2005;42:104-12
- Shoukry NH, Grakoui A, Houghton M, et al. Memory CD8+ T cells are required for protection from persistent hepatitis C virus infection. J Exp Med 2003;197:1645-55
- Grakoui A, Shoukry NH, Woollard DJ, et al. HCV persistence and immune evasion in the absence of memory T cell help. Science 2003;302:659-62
- Matloubian M, Concepcion RJ, Ahmed R. CD4+ T cells are required to sustain CD8+ cytotoxic T-cell responses during chronic viral infection. J Virol 1994;68:8056-63
- Kaplan DE, Sugimoto K, Newton K, et al. Discordant role of CD4 T-cell response relative to neutralizing antibody and CD8 T-cell responses in acute hepatitis C. Gastroenterology 2007;132:654-66
- Fitzmaurice K, Petrovic D, Ramamurthy N, et al. Molecular footprints reveal the impact of the protective HLA-A*03 allele in hepatitis C virus infection. Gut 2011;60:1563-71
- Kim AY, Kuntzen T, Timm J, et al. Spontaneous control of HCV is associated with expression of HLA-B 57 and preservation of targeted epitopes. Gastroenterology 2011;140:686-696.e1
- Neumann-Haefelin C, Thimme R. Impact of the genetic restriction of virus-specific T-cell responses in hepatitis C virus infection. Genes Immun 2007;8:181-92
- Dazert E, Neumann-Haefelin C, Bressanelli S, et al. Loss of viral fitness and cross-recognition by CD8+ T cells limit HCV escape from a protective HLA-B27-restricted human immune response. J Clin Invest 2009;119:376-86
- Rehermann B, Chang KM, McHutchison JG, et al. Quantitative analysis of the peripheral blood cytotoxic T lymphocyte response in patients with chronic hepatitis C virus infection. J Clin Invest 1996;98:1432-40
- Leroux-Roels G, Depla E, Hulstaert F, et al. A candidate vaccine based on the hepatitis C E1 protein: tolerability and immunogenicity in healthy volunteers. Vaccine 2004;22:3080-6
- Verstrepen BE, Depla E, Rollier CS, et al. Clearance of genotype 1b hepatitis C virus in chimpanzees in the presence of vaccine-induced E1-neutralizing antibodies. J Infect Dis 2011;204:837-44
- Choo QL, Kuo G, Ralston R, et al. Vaccination of chimpanzees against infection by the hepatitis C virus. Proc Natl Acad Sci USA 1994;91:1294-8
- Houghton M, Abrignani S. Prospects for a vaccine against the hepatitis C virus. Nature 2005;436:961-6
- Frey SE, Houghton M, Coates S, et al. Safety and immunogenicity of HCV E1E2 vaccine adjuvanted with MF59 administered to healthy adults. Vaccine 2010;28:6367-73
- Drane D, Maraskovsky E, Gibson R, et al. Priming of CD4+ and CD8+ T cell responses using a HCV core ISCOMATRIX vaccine: a Phase I study in healthy volunteers. Hum Vaccin 2009;5:151-7
- Habersetzer F, Baumert TF, Stoll-Keller F. GI-5005, a yeast vector vaccine expressing an NS3-core fusion protein for chronic HCV infection. Curr Opin Mol Ther 2009;11:456-62
- Firbas C, Jilma B, Tauber E, et al. Immunogenicity and safety of a novel therapeutic hepatitis C virus (HCV) peptide vaccine: a randomized, placebo controlled trial for dose optimization in 128 healthy subjects. Vaccine 2006;24:4343-53
- Firbas C, Boehm T, Buerger V, et al. Immunogenicity and safety of different injection routes and schedules of IC41, a hepatitis C virus (HCV) peptide vaccine. Vaccine 2010;28:2397-407
- Klade CS, Schuller E, Boehm T, et al. Sustained viral load reduction in treatment-naive HCV genotype 1 infected patients after therapeutic peptide vaccination. Vaccine 2012;30:2943-50
- Klade CS, Wedemeyer H, Berg T, et al. Therapeutic vaccination of chronic hepatitis C nonresponder patients with the peptide vaccine IC41. Gastroenterology 2008;134:1385-95
- Sallberg M, Frelin L, Weiland O. DNA vaccine therapy for chronic hepatitis C virus (HCV) infection: immune control of a moving target. Expert Opin Biol Ther 2009;9:805-15
- Ahlen G, Nystrom J, Pult I, et al. In vivo clearance of hepatitis C virus nonstructural 3/4A–expressing hepatocytes by DNA vaccine–primed cytotoxic T lymphocytes. J Infect Dis 2005;192:2112-16
- Alvarez-Lajonchere L, Shoukry NH, Gra B, et al. Immunogenicity of CIGB-230, a therapeutic DNA vaccine preparation, in HCV-chronically infected individuals in a Phase I clinical trial. J Viral Hepat 2009;16:156-67
- Rollier CS, Paranhos-Baccala G, Verschoor EJ, et al. Vaccine-induced early control of hepatitis C virus infection in chimpanzees fails to impact on hepatic PD-1 and chronicity. Hepatology 2007;45:602-13
- Folgori A, Capone S, Ruggeri L, et al. A T-cell HCV vaccine eliciting effective immunity against heterologous virus challenge in chimpanzees. Nat Med 2006;12:190-7
- Fattori E, Zampaglione I, Arcuri M, et al. Efficient immunization of rhesus macaques with an HCV candidate vaccine by heterologous priming–boosting with novel adenoviral vectors based on different serotypes. Gene Ther 2006;13:1088-96
- Barnes E, Folgori A, Capone S, et al. Novel adenovirus-based vaccines induce broad and sustained T cell responses to HCV in man. Sci Transl Med 2012;4:115ra1
- Park SH, Shin EC, Capone S, et al. Successful vaccination induces multifunctional memory T-cell precursors associated with early control of hepatitis C virus. Gastroenterology 2012;143:1048-460
- Youn JW, Hu YW, Tricoche N, et al. Evidence for protection against chronic hepatitis C virus infection in chimpanzees by immunization with replicating recombinant vaccinia virus. J Virol 2008;82:10896-905
- Buchbinder SP, Mehrotra DV, Duerr A, et al. Efficacy assessment of a cell-mediated immunity HIV-1 vaccine (the Step Study): a double-blind, randomised, placebo-controlled, test-of-concept trial. Lancet 2008;372:1881-93
- Brave A, Ljungberg K, Wahren B, Liu MA. Vaccine delivery methods using viral vectors. Mol Pharm 2007;4:18-32
- Lin Y, Kwon T, Polo J, et al. Induction of broad CD4+ and CD8+ T-cell responses and cross- neutralizing antibodies against hepatitis C virus by vaccination with Th1-adjuvanted polypeptides followed by defective alphaviral particles expressing envelope glycoproteins gpE1 and gpE2 and nonstructural proteins 3, 4, and 5. J Virol 2008;82:7492-503
- Pancholi P, Perkus M, Tricoche N, et al. DNA immunization with hepatitis C virus (HCV) polycistronic genes or immunization by HCV DNA priming-recombinant canarypox virus boosting induces immune responses and protection from recombinant HCV-vaccinia virus infection in HLA-A2.1-transgenic mice. J Virol 2003;77:382-90
- Wuest T, Both GW, Prince AM, et al. Recombinant ovine atadenovirus induces a strong and sustained T cell response against the hepatitis C virus NS3 antigen in mice. Vaccine 2004;22:2717-21
- Brinster C, Chen M, Boucreux D, et al. Hepatitis C virus non-structural protein 3-specific cellular immune responses following single or combined immunization with DNA or recombinant Semliki Forest virus particles. J Gen Virol 2002;83:369-81
- Elmowalid GA, Qiao M, Jeong S-H, et al. Immunization with hepatitis C virus-like particles results in control of hepatitis C virus infection in chimpanzees. Proc Natl Acad Sci USA 2007;104:8427-32
- Casimiro DR, Chen L, Fu T-M, et al. Comparative immunogenicity in rhesus monkeys of DNA plasmid, recombinant vaccinia virus, and replication-defective adenovirus vectors expressing a human immunodeficiency virus type 1 gag gene. J Virol 2003;77:6305-13
- Tatsis N, ERTL H. Adenoviruses as vaccine vectors. Mol Ther 2004;10:616-29
- Colloca S, Barnes E, Folgori A, et al. Vaccine Vectors Derived from a Large Collection of Simian Adenoviruses Induce Potent Cellular Immunity Across Multiple Species. Sci Transl Med 2012;4:115ra2-2
- Barouch DH, Liu J, Li H, et al. Vaccine protection against acquisition of neutralization-resistant SIV challenges in rhesus monkeys. Nature 2012;482:89-93. doi:10.1038/nature10766
- Reyes-Sandoval A, Rollier CS, Milicic A, et al. Mixed vector immunization with recombinant adenovirus and MVA can improve vaccine efficacy while decreasing antivector immunity. Mol Ther 2009;20:1633-47
- Reyes-Sandoval A, Wyllie DH, Bauza K, et al. CD8+ T effector memory cells protect against liver-stage malaria. J Immunol 2011;187:1347-57
- Capone S, Reyes-Sandoval A, Naddeo M, et al. Immune responses against a liver-stage malaria antigen induced by simian adenoviral vector AdCh63 and MVA primea€“boost immunisation in non-human primates. Vaccine 2010;29:256-65
- ClinicalTrials.gov Identifier: NCT01296451. Available from: http://www.clinicaltrials.gov/ct2/show/NCT01296451 [Last accessed 21 March 2013]
- ClinicalTrials.gov Identifier: NCT01436357. Available from: http://www.clinicaltrials.gov/ct2/show/NCT01436357 [Last accessed 21 March 2013]
- Houghton M. Prospects for prophylactic and therapeutic vaccines against the hepatitis C viruses. Immunol Rev 2011;239:99-108
- Spada E. Multispecific T cell response and negative HCV RNA tests during acute HCV infection are early prognostic factors of spontaneous clearance. Gut 2004;53:1673-81
- Lauer GM, Barnes E, Lucas M, et al. High resolution analysis of cellular immune responses in resolved and persistent hepatitis C virus infection. Gastroenterology 2004;127:924-36
- Makedonas G, Betts MR. Living in a house of cards: re-evaluating CD8+ T-cell immune correlates against HIV. Immunol Rev 2010;239:109-24
- Darrah PA, Patel DT, De Luca PM, et al. Multifunctional TH1 cells define a correlate of vaccine-mediated protection against Leishmania major. Nat Med 2007;13:843-50
- Sallusto F, Lanzavecchia A, Araki K, Ahmed R. From vaccines to memory and back. Immunity 2010;33:451-63
- Newell EW, Sigal N, Bendall SC, et al. Cytometry by time-of-flight shows combinatorial cytokine expression and virus-specific cell niches within a continuum of CD8+ T cell phenotypes. Immunity 2012;36:142-52
- Erickson AL, Kimura Y, Igarashi S, et al. The outcome of hepatitis C virus infection is predicted by escape mutations in epitopes targeted by cytotoxic T lymphocytes. Immunity 2001;15:883-95
- Puig M, Mihalik K, Tilton JC, et al. CD4+ immune escape and subsequent T-cell failure following chimpanzee immunization against hepatitis C virus. Hepatology 2006;44:736-45
- Klenerman P. Viral escape and the failure of cellular immune responses. Science 2000;289:2003a–2003
- Stamataki Z, Coates S, Abrignani S, et al. Immunization of human volunteers with hepatitis C virus envelope glycoproteins elicits antibodies that cross-neutralize heterologous virus strains. J Infect Dis 2011;204:811-13
- Spangenberg HC, Viazov S, Kersting N, et al. Intrahepatic CD8+ T-cell failure during chronic hepatitis C virus infection. Hepatology 2005;42:828-37
- Betts MR, Nason MC, West SM, et al. HIV nonprogressors preferentially maintain highly functional HIV-specific CD8+ T cells. Blood 2006;107:4781-9
- Kannanganat S, Ibegbu C, Chennareddi L, et al. Multiple-cytokine-producing antiviral CD4 T cells are functionally superior to single-cytokine-producing cells. J Virol 2007;81:8468-76
- Bogdan C, Moll H, Solbach W, Rollinghoff M. Tumor necrosis factor-alpha in combination with interferon-gamma, but not with interleukin 4 activates murine macrophages for elimination of Leishmania major amastigotes. Eur J Immunol 1990;20:1131-5
- Precopio ML, Betts MR, Parrino J, et al. Immunization with vaccinia virus induces polyfunctional and phenotypically distinctive CD8(+) T cell responses. J Exp Med 2007;204:1405-16
- Wherry EJ, Teichgraber V, Becker TC, et al. Lineage relationship and protective immunity of memory CD8 T cell subsets. Nat Immunol 2003;4:225-34
- Seder RA, Darrah PA, Roederer M. T-cell quality in memory and protection: implications for vaccine design. Nat Rev Immunol 2008;8:247-58
- La Gruta NL, Turner SJ, Doherty PC. Hierarchies in cytokine expression profiles for acute and resolving influenza virus-specific CD8+ T cell responses: correlation of cytokine profile and TCR avidity. J Immunol 2004;172:5553-60
- Viola A, Lanzavecchia A. T cell activation determined by T cell receptor number and tunable thresholds. Science 1996;273:104-6
- Thimme R, Bukh J, Spangenberg HC, et al. Viral and immunological determinants of hepatitis C virus clearance, persistence, and disease. Proc Natl Acad Sci USA 2002;99:15661-8
- Lechner F, Sullivan J, Spiegel H, et al. Why do cytotoxic T lymphocytes fail to eliminate hepatitis C virus? Lessons from studies using major histocompatibility complex class I peptide tetramers. Philos Trans R Soc Lond B Biol Sci 2000;355:1085-92
- Bots M, Medema JP. Granzymes at a glance. J Cell Sci 2006;119:5011-14
- Peters PJ, Borst J, Oorschot V, et al. Cytotoxic T lymphocyte granules are secretory lysosomes, containing both perforin and granzymes. J Exp Med 1991;173:1099-109
- Makedonas G, Hutnick N, Haney D, et al. Perforin and IL-2 upregulation define qualitative differences among highly functional virus-specific human CD8+ T cells. PLoS Pathog 2010;6:e1000798
- Jo J, Bengsch B, Seigel B, et al. Low perforin expression of early differentiated HCV-specific CD8+ T cells limits their hepatotoxic potential. J Hepatol 2012;57:9-16
- Betts MR, Brenchley JM, Price DA, et al. Sensitive and viable identification of antigen-specific CD8+ T cells by a flow cytometric assay for degranulation. J Immunol Methods 2003;281:65-78
- Sallusto F, Lenig D, Forster R, et al. Two subsets of memory T lymphocytes with distinct homing potentials and effector functions. Nature 1999;401:708-12
- Appay V, van Lier RAW, Sallusto F, Roederer M. Phenotype and function of human T lymphocyte subsets: consensus and issues. Cytometry 2008;73A:975-83
- Appay V, Rowland-Jones SL. Lessons from the study of T-cell differentiation in persistent human virus infection. Semin Immunol 2004;16:205-12
- Merkenschlager M, Terry L, Edwards R, Beverley PCL. Limiting dilution analysis of proliferative responses in human lymphocyte populations defined by the monoclonal antibody UCHL1: implications for differential CD45 expression in T cell memory formation. Eur J Immunol 1988;18:1653-62
- Kaech SM, Tan JT, Wherry EJ, et al. Selective expression of the interleukin 7 receptor identifies effector CD8 T cells that give rise to long-lived memory cells. Nat Immunol 2003;4:1191-8
- Gattinoni L, Lugli E, Ji Y, et al. A human memory T cell subset with stem cell-like properties. Nat Med 2011;17:1290-7
- Kaech SM, Wherry EJ. Heterogeneity and cell-fate decisions in effector and memory CD8+ T cell differentiation during viral infection. Immunity 2007;27:393-405
- Hansen SG, Vieville C, Whizin N, et al. Effector memory T cell responses are associated with protection of rhesus monkeys from mucosal simian immunodeficiency virus challenge. Nat Med 2009;15:293-9
- Flatz L, Roychoudhuri R, Honda M, et al. Single-cell gene-expression profiling reveals qualitatively distinct CD8 T cells elicited by different gene-based vaccines. Proc Natl Acad Sci USA 2011;108:5724-9
- Tatsis N, Fitzgerald JC, Reyes-Sandoval A, et al. Adenoviral vectors persist in vivo and maintain activated CD8+ T cells: implications for their use as vaccines. Blood 2007;110:1916-23
- Gattinoni L, Zhong X-S, Palmer DC, et al. Wnt signaling arrests effector T cell differentiation and generates CD8+ memory stem cells. Nat Med 2009;15:808-13
- Masopust D, Ha S-J, Vezys V, Ahmed R. Stimulation history dictates memory CD8 T cell phenotype: implications for prime-boost vaccination. J Immunol 2006;177:831-9
- Ahmed R, Akondy RS. Insights into human CD8+ T-cell memory using the yellow fever and smallpox vaccines. Immunol Cell Biol 2011;89:340-5
- Bendall SC, Nolan GP, Roederer M, Chattopadhyay PK. A deep profiler's guide to cytometry. Trends Immunol 2012;33:323-32. doi:10.1016/j.it.2012.02.010
- Krahn MD, John-Baptiste A, Yi Q, et al. Potential cost-effectiveness of a preventive hepatitis C vaccine in high risk and average risk populations in Canada. Vaccine 2005;23:1549-58
- Gerlach JT, Diepolder HM, Zachoval R, et al. Acute hepatitis C: high rate of both spontaneous and treatment-induced viral clearance. Gastroenterology 2003;125:80-8