Abstract
Introduction: The number of pulmonary diseases that are effectively treated by aerosolized medicine continues to grow.
Areas covered: These diseases include chronic obstructive pulmonary disease (COPD), lung inflammatory diseases (e.g., asthma) and pulmonary infections. Dry powder inhalers (DPIs) exhibit many unique advantages that have contributed to the incredible growth in the number of DPI pharmaceutical products. To improve the performance, there are a relatively large number of DPI devices available for different inhalable powder formulations. The relationship between formulation and inhaler device features on performance of the drug–device combination product is critical. Aerosol medicine products are drug–device combination products. Device design and compatibility with the formulation are key drug–device combination product aspects in delivering drugs to the lungs as inhaled powders. In addition to discussing pulmonary diseases, this review discusses DPI devices, respirable powder formulation and their interactions in the context of currently marketed DPI products used in the treatment of COPD, asthma and pulmonary infections.
Expert opinion: There is a growing line of product options available for patients in choosing inhalers for treatment of respiratory diseases. Looking ahead, combining nanotechnology with optimized DPI formulation and enhancing device design presents a promising future for DPI development.
1. Introduction
Lung disease is a worldwide medical condition with millions suffering from a considerable number of pulmonary disorders. The complexity of lung disease is complicated by the organ itself with various components including the large and smaller lung airways, the deep lung alveolar region, the interstitium and pulmonary vasculature. Patients can suffer from one or more pulmonary diseases coexisting together. The immensity of lung disease is described by the World Health Organization, which estimated in 2004 that 235 million people suffered from asthma Citation[1] while chronic obstructive pulmonary disorder (COPD) affected an estimated 64 million people worldwide Citation[1]. More than 3 million people died of COPD in 2005 (equal to 5% of all deaths globally that year) Citation[1]. In the past decade, lower respiratory infections and COPD have contributed to significantly larger number of death in the world Citation[2].
Although there are several potential risk factors in the development of COPD, the primary cause continues to be tobacco smoking Citation[3]. There is mounting evidence that childhood asthma is a risk factor for developing COPD later in life Citation[4]. COPD can affect both the lower airways and the tissue parenchyma, contributing to the phenotypic characteristics, such as airway obstruction and emphysema. Environmental factors and occupational exposures that influence air quality play a significant role in the disease process Citation[5-12]. Recent reports suggest that a warming climate may be a contributing factor in chronic lung disease in general, including asthma and COPD Citation[5,6]. These exposures over time result in physiologic alterations as visualized by bronchoscopy, shown in .
Figure 1. Image on bronchoscopy of normal carina in the trachea (A) and complication of chronic obstructive pulmonary disease, saber sheath trachea (B).
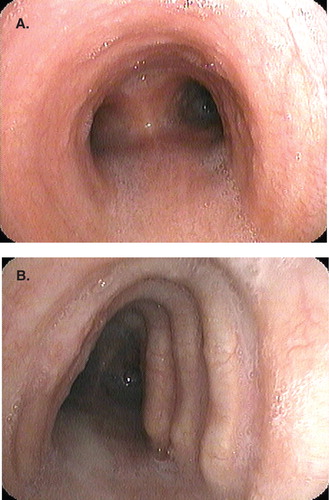
In the setting of incessant lung disease, chronic airway inflammation results with some lung diseases associated with chronic infection as well, which leads to a never-ending cycle. Acute bacterial and viral infections can heighten the infectious and inflammatory state of pulmonary disorders. Viral infections in the setting of chronic bacterial infections were associated with more severe acute exacerbation of diseases like asthma and COPD Citation[11]. demonstrates this acute on chronic state that is often seen. With barriers and physiologic alterations that are ever-changing in the setting of chronic lung disease, therapeutic delivery is often beneficial with direct application to the lung. A lung disease with significant research regarding the direct application of pharmaceuticals, especially antimicrobial, to the lower airways through aerosols includes cystic fibrosis (CF). Patients afflicted with CF eventually develop bronchiectasis due to impaired mucociliary clearance leading to accumulation of mucopurulent secretions and subsequent chronic bacterial colonization with Pseudomonas aeruginosa ().
Figure 2. Image on bronchoscopy of the right main bronchus in a patient with chronic obstructive pulmonary disorder with acute pneumonia. The lower airway mucosa is denuded with mucopurulent secretion that later cultured Staphylococcus aureus.
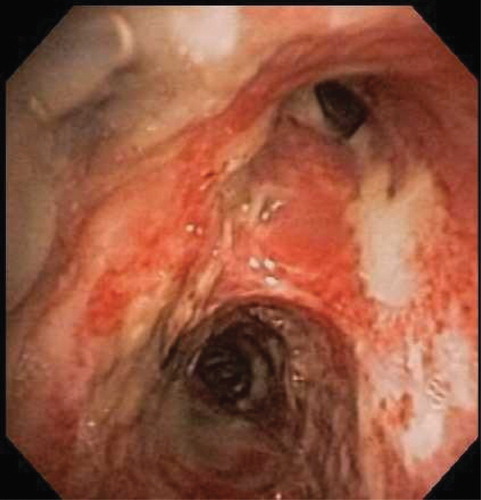
Figure 3. Image on bronchoscopy of the right upper lobe in a patient with cystic fibrosis who had diffuse mucopurulent secretions that later cultured Pseudomonas aeruginosa (A) and underlying lower airway mucosal inflammation after removal of secretions (B).
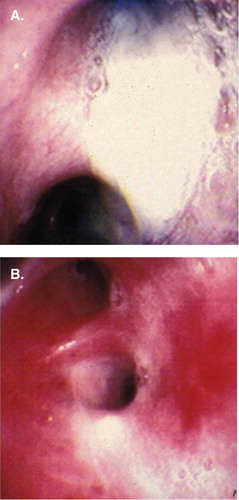
Exploration of inhalation aerosols for drug delivery has contributed vastly in treating pulmonary diseases for decades. Various inhalation delivery systems have been developed to treat lung diseases such as asthma, CF and pulmonary infections. Among them, nebulizers (‘nebs’) and pressurized metered dose inhalers (pMDIs) were the main inhalation aerosol delivery systems to treat respiratory illness for several decades until dry powder inhalers (DPIs) began to reappear. Interestingly, inhalation of powders has been used for many centuries dating back to ancient times by the ancient Egyptians and Greeks. In the 19th century, Newton and Nelson each patented a DPI Citation[10], after which the inhalation aerosol therapy took a detour away from DPI until 1948, when Abbott introduced Aerohalor for penicillin Citation[10]. Although drug delivery through inhalation was achieved many years ago, dose control was poor Citation[13]. When the pharmaceutical industry succeeded in delivering controlled doses of drug, there was no looking back. Treatment of pulmonary diseases, especially asthma, was revolutionized.
Today, drug delivery has come a long way in successfully delivering drug to the lungs not only for local action but also for systemic application. Yet, inhalation aerosol drug delivery faces challenge in achieving consistent dose delivery and toxicity related to higher dosages delivered to the lungs. The four major classes of inhalation aerosol delivery systems are nebulizers, pMDIs, soft-mist inhalers and DPIs. Each has its own advantages, disadvantages and limitations in regard to the type of formulation that can be used, the types of drugs that can be used, and the amount of respirable dose that can be generated from these devices. In the past two decades, respiratory drug delivery has focused on two main aspects of drug delivery: replacing chlorofluorocarbon propellants and methodology to increase drug bioavailability. Hydrofluoroalkane propellant and DPI have come to the rescue in replacing chlorofluorocarbon propellant Citation[14], while nanotechnology continues to be explored for targeted pulmonary delivery Citation[15]. However, the challenge that still exists is achieving higher fraction of respirable drug and consistency in dose delivery. Part of the challenge stems from the operating principles of the devices. Other challenges are formulation optimization, patient noncompliance, incorrect handling of the inhaler device, wrong choice of treatment option and patient’s personal preference to certain device types.
2. Inhaler selection
There is no hard and fast rule for the choice of inhaler for a given disease. Choosing the right inhaler device can be the first step toward successful treatment. Pathology of every disease is different; similarly, performance and operation of every inhaler (powder vs liquid based) are different Citation[16,17]. Further complication includes that the pathology of certain diseases renders patients with decreased ability to perform the functions required for successful treatment. Hence, careful consideration has to be given in choosing the inhaler device since different devices have different techniques, and prescribing more than one type of inhaler may confuse some patients (e.g., prescribing both a pMDI and DPI Citation[18]). On the other hand, not all medications are available in all inhaler types, thus confining the inhalers that can be used for multi-drug treatment in certain diseases.
In prescribing a DPI, inspiratory efficiency of the patient is a vital consideration since all DPI products currently on the market are dependent on patient inspiratory flow. The current methods available to assess the inspiratory flow of patients are checklist method and Clement Clarke check-in dial™. A ‘3W-H’ approach is suggested, where by answering the questions of who? what? where? and how? can help decide the right treatment options Citation[18]. Recently, an acoustics/sound-based methodology has been developed to assess patients’ inhalation technique Citation[19]. This technique can be used by physicians to understand a patient’s inhalation effort during treatment. This would better help the physicians to decide on the patients’ ability to use the inhaler and advice appropriate technique to achieve optimal therapy Citation[19]. Moreover, the device chosen should be suitable for the patient during stable and unstable conditions Citation[18].
A major contributor to the success of inhaled therapy is proper and correct use of the device chosen for therapy. There are several reports regarding the improper use of various inhaler devices Citation[16,20-22]. Hence, it is of the utmost importance for every patient to understand the technique of handling inhaler. It becomes important to have appropriately trained professionals to educate patients about proper use of the device to minimize user error. Patients prefer inhalers that are small sized, portable, convenient and simple to use Citation[23]. Some devices have readable dose counters that aid patients in tracking doses administered and doses remaining, so as to prevent underdosing or accidentally overdosing. Disease state management is enhanced when patient compliance to prescribed therapy is achieved, and this in turn reduces overall healthcare costs and patient morbidity and mortality. Favorable aspects in patient inhalation aerosol therapy can be correlated with reduced dosing frequency, inhaler technique, and patient satisfaction that includes ease of use Citation[23].
3. Dry powder inhalers
Pulmonary drug delivery continues to demonstrate steady growth in the global market. According to market research reports, the DPI market was $6.6 billion in 2010 Citation[24] and reached $17.5 billion in 2013 Citation[25]. It is expected to grow to $31.5 billion in 2018 at a compound annual growth rate of 12.5% Citation[25]. The need for improved treatment of various pulmonary diseases is the driving force for increased DPI research and growth of DPI pharmaceuticals. Inhalation of respirable powders by DPIs has found a strong niche in pulmonary drug delivery. Advantages of DPI include solid-state physical and chemical drug formulation stability, more inhaler device design options for testing with different formulations, ease of use Citation[23], portability compared to nebulizer Citation[17], absence of liquid propellants Citation[17,26] and hand–lung coordination Citation[17,26] compared to pMDI, noninvasiveness compared to intravenous administration, ability to include long-acting hydrophobic water-insoluble drugs Citation[27] and the ability to achieve higher fraction of deep lung deposition Citation[17,28]. Yet, some DPIs exhibit some limitations owing to the need of patient’s inspiratory effort for aerosol generation Citation[17], and different DPI devices have significantly different design anatomies that make standardized comparisons across all DPI devices difficult, and moisture sensitivity of the powders Citation[17]. Additionally, there are opportunities for errors in DPI management and handling by patients, such as incorrect loading of the device Citation[17], failure to pierce the capsule Citation[17], inappropriate inhalation method Citation[16,17], improper storage Citation[17], variability in device techniques, cost and improper inhaler positioning Citation[16]. Nevertheless, the advantages of DPI can outweigh the handling errors if patients are properly trained.
4. DPI for respiratory diseases
Asthma is a multifactorial inflammatory lung disease that features both bronchoconstriction and airway inflammation. Various forms of asthma (mild, moderate, severe) are known to exist and are thought to be due to genetic or environmental factors. When treated solely by the oral route, asthma was not so effectively treated, but the inhalation of aerosols has significantly altered the course of the disease and is now the first-line treatment for decades. Treatment of asthma involves preventive measures where the patient is given daily medication doses for prevention of asthmatic attacks and to stabilize long-term pulmonary function. Acute treatment (i.e., ‘rescue’) is given to stop an acute exacerbation, which can be life-threatening, where the patient experiences difficulty in respiration due to severe bronchoconstriction. Asthma management is individualized based on symptomology, severity and frequency of exacerbations, lung function and other factors. COPD is a progressive inflammatory disease characterized by airflow limitation. Commonly prescribed medications for both asthma and COPD are inhaled corticosteroids (i.e., anti-inflammatory), bronchodilators, leukotriene receptor antagonists, mast cell inhibitors, anticholinergics, muscarinic antagonists and methyl xanthine preparations Citation[28,29]. Narcotic opiates have been reported as palliative measure for end-stage COPD Citation[30]. Therapies used in the treatment of COPD and asthma are similar but the nature of inflammation and cells involved may affect the outcome of treatment Citation[31]. Corticosteroids (anti-inflammatory) are the choice to control inflammation in asthma while its use in COPD is controversial. Bronchodilators (β2 agonist, anticholinergics, theophylline) are central to reduce COPD symptoms and useful in treating asthma Citation[31]. Bronchodilators include LABAs (i.e., long-acting β2 agonists, duration of action 12 h Citation[32]) for preventative maintenance therapy and SABAs (i.e., short-acting β2 agonists, duration of action 2 – 6 h) for rescue (fast-acting treatment) of acute asthma exacerbation. There are successful combination products of inhaled corticosteroids/LABA to treat asthma; however, this recent study might open doors in research for their use in COPD too Citation[33]. and list the drugs that are marketed for asthma and COPD as DPIs. There are additional products approved for systemic therapeutic action through delivery by the pulmonary route of administration, which are currently Afrezza® for diabetes type I/II and Adasuve® for CNS therapy. shows the chemical structures of some currently marketed pulmonary drugs.
Table 1. Listing of DPI products currently on the US market (i.e., approved by the US FDA) Citation[101].
Table 2. Listing of DPI products currently in European market Citation[102].
Figure 4. Chemical structures (ACD/ChemSketch 2012, version 14.01, Advanced Chemistry Development, Inc., Toronto, Canada) of example drugs made into dry powder aerosol: (A) Umeclidinium bromide; (B) Indacaterol; (C) Aclidinium bromide; (D). Mometsone furoate; (E) Formoterol fumarate; and (F) Vilanterol.
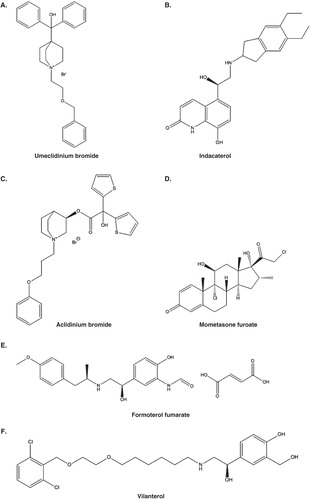
Acute respiratory infections are caused by several microorganisms including viruses, bacteria, fungi, parasites and protozoa Citation[34]. Among these, viruses and bacteria are the most common for causing respiratory infections. Occasionally, respiratory infections can be chronic or a comorbidity of other diseases (i.e., a secondary lung disease concomitantly existing with the primary lung disease). For example, chronic bacterial infections of the lung significantly contribute to the progress of CF, while acute viral infections can result in exacerbating the majority of pulmonary disorders, such as asthma, COPD and CF. In CF, chronic respiratory infections require administration of antibiotics Citation[35], while influenza and pneumococcal are advised in pulmonary disorders Citation[36].
4.1 Types of DPIs
DPIs are classified into different types according to their metering system or dispersion mechanism or their device design. Based on the metering system, they can be unit dose inhaler dispensing single dose of drug (capsules made of hydroxyl propyl methyl cellulose or gelatin), multi-unit dose inhaler dispensing several single doses (blister packs), or multiple dose inhaler dispensing multiple doses of drug (powder reservoir). shows images of DPI product in market with the three types of devices. The device design is an important factor in deciding its efficiency because the dimensions and internal anatomy of the device introduce resistance to air flow. Hence, devices can be classified based on the resistance into low-, medium- or high-resistance devices. Classification of devices based on their resistance has not gained importance as much as dispersion mechanism has. However, this classification of devices would aid in the selection of correct inhalers that is appropriate to patient condition and ability. On the basis of drug dispersion, devices can be active or passive, where active dispersion of the drug is aided by mechanical or electrical means, while passive dispersion of the drug is brought about by patient inspiratory flow Citation[37-39]. Therefore, success of passive DPI depends on patient’s ability to generate an inspiratory flow rate (IFR) that is strong enough to overcome the resistance of the inhaler and set particles in motion. is selected list of DPI devices with their classification. A DPI product is a combination of the drug formulation and the device used to dispense it. Hence, metering and dispersion classifications are more appropriate to drug products while resistance classification is for the device alone. Device resistance plays an important role in successful therapy and is described in detail in Section 5.2 Device Resistance.
Figure 5. Image of dry powder inhalers (DPIs) with different DPI device metering systems: (A) Unit dose with the capsule (Aerolizer®). (B) Prefilled multi-unit dose (Diskus®). (C) Multiple-dose powder reservoir bed (Twisthaler®).
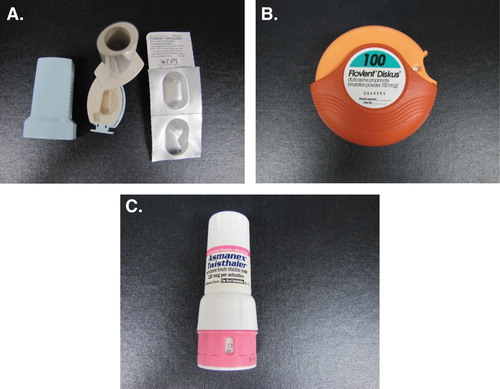
Table 3. Selected DPI devices currently available in the USA and European Union (US) Citation[14,40,46,59,61,103-109].
5. DPI functionality
Drug delivery from DPI involves fluidization (aerosolization), de-aggregation, dispersion and deposition of particles into the lung. There are three main forces responsible for these activities: interparticulate forces between the powder particles, dispersion forces generated during inhalation, and deposition forces in the respiratory tract. A DPI product is made up of a device, drug formulation and metering system. The influence of device design and drug formulation with respect to the above said forces, on product performance, is discussed in this section.
A DPI device consists of an air entry port, drug-holding chamber and a mouth piece. The different aspects of an inhaler including pressure drop, resistance, and so on are related to one another in determining the overall performance of the device. From a device perspective dimensions, shape, fluid mechanics, air flow pattern, interaction with the formulation contributes to performance characteristics Citation[40]. The physicochemical factors of the drug formulation that affect DPI performance include particle size, shape, density, surface roughness, surface area and morphology, and crystallinity Citation[40-43]. Surface roughness of carrier particles increases the performance of a DPI Citation[43], whereas the aerodynamic particle size distribution generated from a passive DPI device depends (inversely) on patient’s IFR Citation[44].
5.1 Fluidization and de-aggregation
Fluidization (aerosolization) and de-aggregation of powder from the inhaler is the foremost step in determining efficiency of an inhaler. The mechanism of DPI fluidization includes shear, capillary and mechanical forces (vibrational, impaction) Citation[45]. Shear force fluidization is important in passive devices, which is caused by air flow velocity in the device Citation[45]. This was studied using two marketed passive DPI devices, the Handihaler® and Cyclohaler® (Aerolizer®) Citation[46]. The Handihaler® exhibited high-velocity inlet air that pushed the capsule away from the air inlet point, while a low pressure created at the base of the capsule pulled it down. Repetition of this push-and-pull motion caused radial vibration and pressure difference that entrained the powder from the capsule. In the Cyclohaler®, the air inlet was tangential to the capsule, which created a tangential pressure drop and eventually swirling movement of capsule. It was also observed that the influence of airflow was more pronounced in the Handihaler® than the Cyclohaler® Citation[46]. This study suggested that aerosolization is improved by increasing the velocity of air or causing a pressure drop. Air velocity can be increased when patient inhales at a higher rate and pressure drop can be achieved with the device design Citation[41]. shows the difference in design between Handihaler® and Cyclohaler® (Aerolizer®). From a solid-state formulation perspective, aerosolization of a powder also depends on other factors like particle interactions. The interaction between active pharmaceutical ingredient (API) and excipient(s) is influenced by the manufacturing process, which can also create ‘surface hot spots’ (i.e., high– surface-energy active sites) Citation[40] that can lead to particle aggregation. Particle interaction is attributed to intermolecular forces between particles, mainly adhesive/cohesive forces such as electrostatic attraction, van der Waals interaction and capillary forces Citation[45]. Among these, van der Waals force is a short-range force, electrostatic is a long-range force and capillarity is humidity dependent. Exact measurement of parameters leading to these forces is difficult; however, particle size is a common factor that is involved in measuring these forces Citation[45]. The force required to fluidize the powder depends on the resistance of the device and patient inspiratory flow, which is discussed later. In any case, the force required to aerosolize a formulation must be higher than the interparticulate interaction and the weight of the particles. Alternately, if the particles are less dense or hollow, it will be more readily aerosolized Citation[47]. It is noteworthy to mention that formulation of less-dense or porous particles is a popular area of research in nanotechnology Citation[48,49].
Figure 6. Images of various parts for two unit-dose DPI devices: Left column – HandiHaler®, right column – Aerolizer®. (A & E) – device external view; (B & F) – capsule chamber and grid; (C & G) – mouth piece; (D & H) – capsule-piercing pins within the capsule chamber.
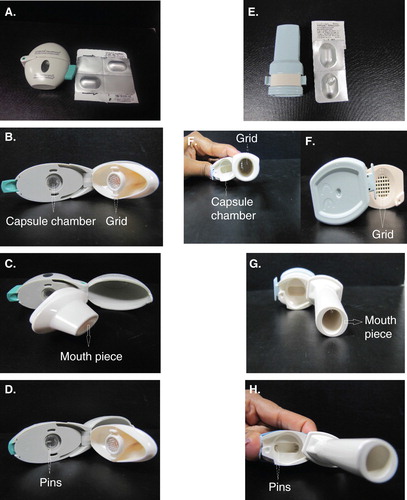
In general, particles with an aerodynamic diameter (Da) 5 – 10 µm can enter the lungs with deposition in the larger airways (i.e., trachea and first several divisions of the bronchi) Citation[50]. Aerosol particles with an aerodynamic diameter of ≤ 5 µm can efficiently reach the mid-lung region (i.e., larger non-respiratory bronchioles) and smaller airways (i.e., smaller non-respiratory bronchioles), while particles ≤ 2 um can target the bronchioalveolar (i.e., respiratory bronchioles to the alveoli) and deep lung alveolar regions. However, particles of this size are very difficult to handle while processing or manufacturing Citation[41,51]. Hence, the drug is formulated as an adhesive mixture or soft spherical agglomerate Citation[41,52]. Adhesive mixture consists of drug with inert carrier particles where the drug is distributed over the carrier particle surface. Spherical agglomerate can be pure drug or drug with excipients (to improve handling and dosing) Citation[53], which congregate together to form bigger particles. De-aggregation (de-agglomeration) is required to separate the particles from the carrier or from the spherical agglomerate. Inertial forces Citation[54], frictional forces Citation[54], turbulent shear Citation[54] and collision are the forces that cause de-aggregation of particles in inhaler. The foremost roadblock to de-aggregation is interparticulate interaction; nonetheless, device design also contributes to de-aggregation. An efficient device design utilizes the energy from patient inspiratory air flow Citation[52] to increase the residence time of the powder Citation[55] or cause mechanical impaction of the particles with the device walls Citation[56] for complete separation of particles. Higher kinetic energy in the inspiratory air increases inertial and frictional forces and leads to better separation of particles Citation[54]. A good example of this is the internal geometry of the marketed inhaler Aerolizer®, which uses a swirling motion from the air flow that facilitates the particle–device collision leading to better separation Citation[56]. Other device features like narrow mouth piece, impeller and rotating helical blade or grid cause rotational motion, impaction, shear and turbulence that can aerosolize and disperse the powder Citation[41,55]. Some recent advancements in de-aggregation include a device containing breath-driven rotor that causes agitation/vibration, cyclone chamber with a mesh to sieve powder Citation[57], reverse cyclone technology Citation[58] and cyclone separator Citation[41].
5.2 Device resistance
Dispersion of powder from a DPI device requires energy, which can come from pneumatic, vibrational or mechanical means Citation[38]. A passive DPI device depends on the patient’s IFR for the required energy to disperse the powder while an active DPI device uses mechanical (vibration, impact force, compressed air, impellers) or electrical source built in the device (i.e., not dependent on the patient’s IFR) Citation[39]. In a passive DPI device, the aerosol dispersion and delivery occur simultaneously, while in the active device it could occur simultaneously or separately Citation[38]. The forces responsible for dispersion of the powder in DPI include aerodynamic forces (drag and lift), inertial forces (vibrational, rotational, centrifugal and collision), and shear and frictional forces Citation[59].
During DPI inhalation, the patient’s inspiratory effort with the resistance of the device creates turbulent energy, which is measured as a pressure drop that causes de-aggregation of powder Citation[60]. The relationship between inhaler device resistance (R) and pressure drop (PD) is given in the following equation Citation[61]:
where flow (Q) is directly related to the PD and inversely related to the R. From this equation, inhalers with higher resistance can create more turbulence, which is more likely to assist in de-agglomeration Citation[52]. The different designs and geometry of devices cause difference in pressure drop and resistance within the device. There is a critical inspiratory pressure (PI) for every device where an inspiratory flow below PI is laminar and above is turbulent. Hence, it is important for patients to inhale with pressure higher than PI Citation[62]. Higher pressure drop in the device causes strong turbulence, which leads to more shear stress and increased fine particle fraction (FPF) Citation[60,62]. FPF is the ratio of mass of particles in the aerosol with aerodynamic diameter (Da) less than equal to 5 μm (≤ 5 µm) to the total mass of emitted particles. Additionally, the pressure change of high-resistance device is greater than low-resistance device Citation[60]. All of these factors lead to a design with higher pressure drop and higher resistance to demonstrate better inhalation performance in in vitro testing Citation[63]. It is observed that medium–high-resistance DPI device have the better lung deposition at pressure drop 2 – 4 kPa Citation[64]. It should be noted that device resistance is not the only reason for good performance, and de-aggregation principle of the device is equally as important Citation[58]. EquationEquation (11 ) suggests that airflow is inversely proportional to device resistance, that is, high-resistance devices require low flow to create the required pressure drop. However, there are concerns about using high-resistance device for patients during exacerbation of asthma or COPD. Studies have shown that, even during exacerbation, patients are able to generate the required pressure drop and achieve better performance in higher-resistance inhaler Citation[52,65].
Drug formulation characteristics affecting dispersion include particle size, shape and density. Interparticulate forces also play a vital role where insufficient detachment of drug from carrier due to strong interparticulate forces can lead to suboptimal dosing Citation[66]. Spherical particles with narrow size distribution and smooth surface can decrease solid-state interparticulate interactions (i.e., mechanical interlocking/structural cohesion, van der Waals, electrostatic and capillary forces) by reducing the points of contact between adjacent particles, thereby increasing dispersion of the formulation Citation[67]. The FPF of currently available DPI varies from 9 to 78.7% Citation[41]. It is imperative that force generated by DPI device disperses the drug from its aggregate or from the carrier particle leading to lung deposition.
5.3 Deposition
There are three main mechanisms by which particles deposit in the lungs, namely impaction, sedimentation and diffusion Citation[14], which in turn is dependent on the particle size. While larger particles settle in the upper airways by impaction and sedimentation, smaller particles tend to reach lungs through diffusion. However, in some cases submicron particles fail to deposit in the lungs and are exhaled Citation[14]. Nanotechnology and particle engineering techniques are making immense progress in developing inhalation products that can improve particle dispersion and lung deposition of the formulation Citation[26,68,69].
Numerical modeling methods, in vitro and in vivo methods, have been used by researchers in designing DPI devices. Some of the methods used in the past are discrete element method, computational fluid dynamics to predict particle motion Citation[70], particle deposition Citation[70], inhalation flow stream Citation[56], pressure profiles Citation[56] and particle trajectories Citation[56,71], γ scintigraphy to predict particle distribution Citation[72] and particle deposition Citation[72], single photon emission tomography to predict particle deposition Citation[73] and particle distribution Citation[73].
6. Other considerations
6.1 Device considerations
Despite all device design and formulation efforts to improve inhaler performance, retention of drug in the device remains to be a concern that can potentially lead to underdosing. The FPF of a DPI is anywhere between 20 and 30% Citation[74], compared to device retention up to 15 – 60% in another study Citation[75]. Reducing device retention and enhancing the emitted dose can be achieved when using anti-adherent or anti-friction excipients (e.g., magnesium stearate, leucine, lecithin), which reduces friction drag and improves drug dispersion Citation[75]. These agents, also called as force-control agents, are added to the formulation in addition to coating the device surface and capsule Citation[71,75]. Another important factor previously considered is patient’s IFR. Every device has a range of IFR for optimal performance, for example, the IFR-independent active device produces constant FPF over a range of IFR; but at higher IFRs, the FPF decreases Citation[52]. This emphasizes the need for the physician to know about the patient’s inspiratory capacity and device performance capacity in prescribing DPI. There has been inter-and intrapatient variability in peak inhalation flow rate measurements making this selection difficult Citation[76]. Nevertheless, the ultimate relationship between the physicochemical characteristics of the powder and the inhaler design will decide the aerosolization, de-aggregation and dispersion characteristics of the drug particles. Additional device design features that can enhance the performance of DPI device are reported to be wider mouth piece Citation[55], aperture orientation in capsule Citation[71,77], decrease in air inlet size Citation[76,78], and audible and/or visual aid that would help patient understand that the inhaler has been operated correctly or not Citation[79].
6.2 Formulation considerations
Drug formulation for DPI is aimed at making particles that are in the respirable size range, which is aided through particle engineering techniques and nanotechnology Citation[15,26,69,80-82]. A DPI formulation contains micronized drug in a pure form or drug with excipients or blended with coarse carrier particles. The choice of excipients and carrier particles greatly influences drug detachment and deposition characteristics. Several micronization processes render the powder cohesive with high energy, while the inclusion of the carrier particles decreases the cohesiveness of the drug and improves aerosolizing. The only approved non-respirable carrier in the USA is inhalation grade α-lactose monohydrate. Alternative non-respirable carriers include mannitol, glucose, erythritol, xylitol, sorbitol, raffinose, sucrose and maltitol Citation[83,84]. Any excipient that is added to a DPI formulation must overcome clearance by pulmonary enzymes; in other words, compounds that closely resemble endogenous substance to lungs when used as excipient can better evade lung clearance, for example, phospholipids Citation[85]. Excipients are not always required in a DPI formulation. The first carrier-free DPI approved by FDA was Exubera®, which contains engineered particles with molecularly mixed excipients. A successful product that is currently marketed as TOBI® Podhaler™ has engineered particles through a technology called Pulmosphere®. This DPI is a significant advancement in treating pulmonary infection secondary to CF. Powder formulation of the drug (tobramycin) is of greater convenience, easy to use and does not require refrigeration to store compared to the liquid formulation (nebulizer) Citation[86]. It also reduced the administration time by one-third compared to liquid formulation Citation[87]. Particle engineering techniques are used to improve simultaneous administration of two drugs in single formulation by coating the drug with a solution containing other drug Citation[35] or co-spray drying a solution where both drugs are dissolved in same solution Citation[67].
Formulation improvements through nanotechnology have tremendous potential in advanced pulmonary drug delivery. Hence, studies have been conducted to evaluate the usefulness of polymeric nanoparticles Citation[88-91] and lipid nanoparticles Citation[68,92,93] in delivering drug to the lungs in addition to imparting sustained-release functionality. Some types of nanoparticles employed in drug delivery are nanoaggregates, nanocomposites Citation[94], magnetic nanoparticles Citation[95] and effervescent nanoparticles Citation[96].
A recent successful formulation approach to improve DPI performance is the use of excipient enhanced growth, where the API is formulated with a hygroscopic excipient like sodium chloride or citric acid Citation[97]. The submicron aerosol particles grow in size due to the humid environment of the respiratory tract and ensure deposition in the lungs Citation[97-99].
7. Metering system
In a capsule-based unit-dose DPI device, a single dose of powder formulation is prefilled in a capsule, which is then prepackaged in individually sealed aluminum foil by the manufacturer. The capsule-based unit-dose DPI device is not preloaded with the capsule by the manufacturer. The patient breaks the presealed aluminum foil package, removes the prefilled capsule, loads the device and presses the button that pierces or cuts the capsule with pins or blade to release the formulation upon inhalation Citation[55,100]. The capsule opening (piercing/cutting) offers the initial de-agglomeration and turbulence within the capsule. The capsule-opening mechanism is important, since it has to be consistent to release the powder completely and uniformly Citation[55]. The mechanical process of piercing the capsule is done with sets of two or four pins. Initially, the capsule resists puncture by the pin and undergoes deformation. On further force from the pins, capsule puncture occurs after which the force reduces and the pin progressively proceeds into the capsule. Capsule puncture tends to be easier at lower humidity (11%) than higher Citation[100]. Emptying of the powder depends on the number and diameter of holes made in the capsule Citation[64].
In addition to capsule-based unit-dose DPI devices, there are multi-unit dose and multiple-dose DPI devices. Multi-unit-dose DPI devices, which are preloaded by the manufacturer with an aluminum foil blister (i.e., where the powder formulation is prepacked in sealed aluminum foil units on a disk or on an aluminum foil blister strip), and multidose DPI devices are powder bed reservoir DPIs. shows images of DPI product in market with the three types of devices. Capsule-based unit-dose DPI devices and multi-unit-dose DPIs are prepacked in aluminum-foil-sealed unit doses, which offer better protection from the environment compared to reservoir type where the powder bed is exposed to the environmental and patient’s breath humidity with each administered dose that may contribute to drug degradation. Due to this significant limitation, desiccant must be present inside the powder bed reservoir DPI device (in contrast to the many capsule-based unit-dose and multi-unit dose DPI products).
8. Conclusions
This paper discussed the fundamental aspects of DPIs and focused on their application to treat COPD, lung inflammatory diseases and pulmonary infections. An outlook on different aspects of device design and drug formulation that can influence the performance of the inhaler was also presented. The ultimate performance of a DPI pharmaceutical product is determined by the interaction of formulation with the device design, physicochemical characteristics of the drug, and the patient’s ability to correctly use the device. From the considerations discussed, an ideal DPI would have complete de-aggregation and dispersion, deep lung deposition with increased FPF (≤ 5 μm) and minimum deposition elsewhere, maximum fraction of drug emitted from the device, decreased device retention, uniform dose delivery, and ease of use. It is also anticipated to be independent of patient’s IFR, easy to learn and use, portable and low-priced.
9. Expert opinion
Comparison of two dry powder inhalation products is complex, since there are multifactorial differences that confound any meaningful comparison, which include device variables, powder formulation variables and device/formulation combination variables. For this comparison to be reasonable, the formulation used must be consistent and the device properties must be as similar as possible. The device performance and reproducibility must be considered during this comparison.
There is a growing line of product options available for patients in choosing inhalers for treatment of respiratory diseases. Most inhalers have similar steps in use, for example, placing the device at the same place in mouth, breathing from the device and not breathing into the device, a breath-hold step for a few seconds after inhalation to enhance deposition by sedimentation due to gravity, and cleaning the device mouthport. However, the subtle difference in the use of different devices can confuse patients. Successful use of the DPI device is vital to effective pulmonary delivery and treatment. There is no DPI device commercially available that is independent of operator (patient) error. A particular problem with passive DPIs, which are currently available, is dose variation confounded by a patient’s inspiratory rate. To better control this problem, more active DPIs should be created in a cost-effective way and implemented into pharmaceutical products for local treatment of lung disease such that aerosol generation will not depend on patient effort.
Nanotechnology is a unique and exciting platform for drug delivery. Pulmonary drug delivery is no exception to this novelty. The different types of nanoparticles available offer plenty of opportunity for formulation. Increased drug-loading capacity, increased surface area, decreased particle size, enhanced targeting by surface moieties and enhanced mucus penetration are noteworthy features of nanoparticles to be considered in pulmonary delivery. Recent research has demonstrated the usefulness of nanoparticles combined with particle engineering design technologies in the application of pulmonary drug delivery. Combining nanotechnology with optimized DPI formulation and enhancing device design present a particularly promising future for DPI development. This is particularly timely at a time of need with an ever-growing patient population with pulmonary disease and to reduce the complicated treatment burden for these patients.
Targeted pulmonary drug delivery as inhalation aerosols has been demonstrated to be essential in effectively treating respiratory diseases and is the primary delivery system used clinically in the treatment and prevention of pulmonary diseases such as asthma, chronic obstructive pulmonary disorder (COPD), certain pulmonary infections, and pulmonary inflammation.
The choice of correct inhaler devices plays an important role in successful treatment.
Dry powder inhaler (DPI) pharmaceutical products are classified as drug–device combination products. Dry powder inhalers (DPIs) are compact, easy to use and exhibit superior physicochemical stability properties with effective performance to successful targeted clinical treatment of pulmonary diseases.
Prescribed therapies for COPD and asthma are similar; hence, many DPI products are prescribed for both conditions. Dual drug combination inhalation aerosol products are well accepted by patients and provide enhanced therapy due to co-deposition of both drugs at the same target site promoting synergistic therapeutic effects.
Particle engineering techniques and nanotechnology are contributing in developing high-performing DPIs with controlled drug release and enhanced targeting to specific lung regions.
Powder formulation physicochemical characteristics, solid-state particle properties, deaggregation and dispersion mechanisms, DPI device resistance, the patient’s inspiratory flow rate, and DPI device anatomical design all influence aerosol performance of DPIs.
Acknowledgments
The authors acknowledge The College of Pharmacy Graduate Fellowship (The University of Arizona, Tucson, AZ, USA) support awarded to P Muralidharan.
Declaration of interest
The authors have no relevant affiliations or financial involvement with any organization or entity with a financial interest in or financial conflict with the subject matter or materials discussed in the manuscript. This includes employment, consultancies, honoraria, stock ownership or options, expert testimony, grants or patents received or pending, or royalties.
Notes
This box summarizes key points contained in the article.
Bibliography
- World Health Organization. Available from: www.who.int/mediacentre/factsheets/fs307/en/ [Cited 14 July 2014]
- World Health Organization. Available from: http://who.int/mediacentre/factsheets/fs310/en/ [Cited 18 July 2014]
- Cunningham TJ, Ford ES, Rolle IV, et al. Associations of self-reported cigarette smoking with chronic obstructive pulmonary disease and co-morbid chronic conditions in the United States. COPD 2014: 10.3109/15412555.2014.949001
- Tai A, Tran H, Roberts M, et al. The association between childhood asthma and adult chronic obstructive pulmonary disease. Thorax 2014;69:805-10
- Ault A. Report blames global warming for rising asthma. Lancet 2004;363(9420):1532
- Bernstein AS, Rice MB. Lungs in a warming world: climate change and respiratory health. Chest 2013;143(5):1455-9
- Dadvand P, Nieuwenhuijsen MJ, Agusti A, et al. Air pollution and biomarkers of systemic inflammation and tissue repair in COPD patients. Eur Respir J 2014;44(3):603-13
- Graber JM, Stayner LT, Cohen RA, et al. Respiratory disease mortality among US coal miners; results after 37 years of follow-up. Occup Environ Med 2014;71(1):30-9
- Rodriguez E, Ferrer J, Zock JP, et al. Lifetime occupational exposure to dusts, gases and fumes is associated with bronchitis symptoms and higher diffusion capacity in COPD patients. PLoS One 2014;9:2
- Sanders M. Inhalation therapy: an historical review. Prim Care Respir J 2007;16(2):71-81
- Wark PA, Tooze M, Powell H, Parsons K. Viral and bacterial infection in acute asthma and chronic obstructive pulmonary disease increases the risk of readmission. Respirology 2013;18(6):996-1002
- Gordon SB, Bruce NG, Grigg J, et al. Respiratory risks from household air pollution in low and middle income countries. Lancet Resp Med 2014;2(10):823-60
- Hickey AJ. Back to the future: inhaled drug products. J Pharm Sci 2013;102(4):1165-72
- Mansour HM, Hickey AJ. Chapter 5: delivery of drugs by the pulmonary route. In: Florence AT, Siepmann J, editors. Modern pharmaceutics volume 2: applications and advances. Taylor & Francis, Inc; New York: 2009. p. 191-219
- Mansour HM, Rhee YS, Wu X. Nanomedicine in pulmonary delivery. Int J Nanomedicine 2009;4:299-319
- Sanchis J, Corrigan C, Levy ML, Viejo JL. Inhaler devices - from theory to practice. Respir Med 2013;107(4):495-502
- Newman SP. Inhaler treatment options in COPD. Eur Respir Rev 2005;14(96):102-8
- Dekhuijzen PN, Vincken W, Virchow JC, et al. Prescription of inhalers in asthma and COPD: towards a rational, rapid and effective approach. Respir Med 2013;107(12):1817-21
- Holmes MS, Seheult JN, Geraghty C, et al. A method of estimating inspiratory flow rate and volume from an inhaler using acoustic measurements. Physiol Meas 2013;34(8):903-14
- Rootmensen GN, van Keimpema ARJ, Jansen HM, de Haan RJ. Predictors of incorrect inhalation technique in patients with asthma or COPD: a study using a validated videotaped scoring method. J Aerosol Med Pulm Drug Deliv 2010;23(5):323-8
- Wilson DS, Gillion MS, Rees PJ. Use of dry powder inhalers in COPD. Int J Clin Pract 2007;61(12):2005-8
- Lurslurchachai L, Krauskopf K, Roy A, et al. Metered dose inhaler technique among inner-city asthmatics and its association with asthma medication adherence. Clin Respir J 2014;8(4):397-403
- Svedsater H, Dale P, Garrill K, et al. Qualitative assessment of attributes and ease of use of the ELLIPTA dry powder inhaler for delivery of maintenance therapy for asthma and COPD. BMC Pulm Med 2013;13:72
- BCC market research report PDDSTaGM. 2012. Available from: www.bccresearch.com/market-research/healthcare/pulmonary-drug-delivery-systems-hlc094a.html [Cited 1 July 2014]
- BCC market research report PDDSTaGM. 2014. Available from: www.marketwatch.com/story/pulmonary-drug-delivery-systems-technologies-and-global-markets-2014-06-10 [Cited 1 July 2014]
- Bailey MM, Berkland CJ. Nanoparticle formulations in pulmonary drug delivery. Med Res Rev 2009;29(1):196-212
- Al-Hallak MH, Sarfraz MK, Azarmi S, et al. Pulmonary delivery of inhalable nanoparticles: dry powder inhalers. Therapeutic delivery 2011;2(10):1313-24
- Anna M. Asthma in Focus. Pharmaceutical Press/RPS publishing; London, UK: 2007
- Pascual RM, Peters SP. Chapter 63: management of chronic asthma : in adults. In: Barnes PJ, Drazen JM, Rennard SI, Thomson NC, editors. Asthma and COPD: basic mechanisms and clinical management. Academic Press/Elsevier; San Diego, CA, USA: 2009. p. 763-74
- Rennard SI. Chapter 66: treatment of stable COPD. In: Barnes PJ, Drazen JM, Rennard SI, Thomson NC, editors. Asthma and COPD: basic mechanism and clinical management. 2nd edition. Academic Press/Elsevier; San Diego, CA, USA: 2009. 823-36
- Buist AS. Similarities and differences between asthma and chronic obstructive pulmonary disease: treatment and early outcomes. Eur Respi J Suppl 2003;39:30s-5s
- Undem BJ, Lichtenstein LM. Chapter 28: drugs USED in the treatment of asthma. In: Hardman JG, Limbird LE, editors. Goldman & Gilmans’s: the pharmacological basis of therapeutics. 10th edition. McGraw-Hill; NewYork: p. 733-54
- Gershon AS, Campitelli MA, Croxford R, et al. Combination long-acting beta-agonists and inhaled corticosteroids compared with long-acting beta-agonists alone in older adults with chronic obstructive pulmonary disease. JAMA 2014;312(11):1114-21
- Denny J. Floyd W Denny, Jr Chapter 1: the impact of respiratory virus infections on the world’s children. In: Skoner DP, editor. Asthma and respiratory infections. Marcel Dekker, Inc; New York,NY: 2001. p. 1-22
- Pilcer G, De Bueger V, Traina K, et al. Carrier-free combination for dry powder inhalation of antibiotics in the treatment of lung infections in cystic fibrosis. Int J Pharm 2013;451(1-2):112-20
- Wedzicha JA. Chapter 67: acute exacerbations of COPD. In: Barnes PJ, Drazen JM, Rennard SI, Thomson NC, editors. Asthma and COPD : basic mechanisms and clinical management. 2nd edition. Academic Press/Elsevier; San Diego, CA,USA: 2009. p. 837-46
- Zhang X, Ma Y, Zhang L, et al. The development of a novel dry powder inhaler. Int J Pharm 2012;431(1-2):45-52
- Hickey AJ, Mansour HM. Chapter 43: formulation challenges of powders for the delivery of small molecular weight molecules as aerosols. In: Rathbone MJ, Hadgraft J, Roberts MS, Lane ME, editors. Modified-release drug delivery technologies. 2nd edition. Informa Healthcare; New York: 2008. p. 573-601
- Hickey AJ, Crowder TM. Chapter 16: next generation dry powder inhalation delivery systems. In: Hickey AJ, editors. Inhalation aerosols : physical and biological basis for therapy. 2nd edition. Informa Healthcare USA, Inc; New York,NY: 2007. p. 445-60
- Adams WP, Lee SL, Plourde R, et al. Effects of device and formulation on in vitro performance of dry powder inhalers. AAPS J 2012;14(3):400-9
- Islam N, Cleary MJ. Developing an efficient and reliable dry powder inhaler for pulmonary drug delivery–a review for multidisciplinary researchers. Med Eng Phys 2012;34(4):409-27
- Wu X, Adedoyin OO, Mansour HM. Pulmonary and nasal anti-inflammatory and anti-allergy inhalation aerosol delivery systems. Anti-Inflammatory & Anti-Allergy Agents in Medicinal Chemistry 2011;10:215-29
- Zellnitz S, Redlinger-Pohn JD, Kappl M, et al. Preparation and characterization of physically modified glass beads used as model carriers in dry powder inhalers. Int J Pharm 2013;447(1-2):132-8
- Nielsen KG, Skov M, Klug B, et al. Flow-dependent effect of formoterol dry-powder inhaled from the Aerolizer. Eur Respir J 1997;10(9):2105-9
- Xu Z, Mansour HM, Hickey AJ. Particle interactions in dry powder inhaler unit processes: a review. J Adhes Sci Technol 2011;25(4-5):451-82
- Shur J, Lee S, Adams W, et al. Effect of device design on the in vitro performance and comparability for capsule-based dry powder inhalers. AAPS J 2012;14(4):667-76
- Tsapis N, Bennett D, Jackson B, et al. Trojan particles: large porous carriers of nanoparticles for drug delivery. Proc Nat Acad Sci USA 2002;99(19):12001-5
- Edwards DA, Hanes J, Caponetti G, et al. Large porous particles for pulmonary drug delivery. Science 1997;276(5320):1868-71
- Hadinoto K, Phanapavudhikul P, Kewu Z, Tan RB. Dry powder aerosol delivery of large hollow nanoparticulate aggregates as prospective carriers of nanoparticulate drugs: effects of phospholipids. Int J Pharm 2007;333(1-2):187-98
- Suarez S, Hickey AJ. Drug properties affecting aerosol behavior. Respir Care 2000;45(6):652-66
- Watts AB, Williams RO. Chapter 15: nanoparticles for pulmonary delivery. In: Smyth HD, Hickey AJ, editors. Controlled pulmonary drug delivery. Springer; NewYork: 2011. p. 335-66
- Demoly P, Hagedoorn P, de Boer AH, Frijlink HW. The clinical relevance of dry powder inhaler performance for drug delivery. Respir Med 2014;108(8):1195-203
- Louey MD, Stewart PJ. Particle interactions involved in aerosol dispersion of ternary interactive mixtures. Pharm Res 2002;19(10):1524-31
- de Boer AH, Hagedoorn P, Gjaltema D, et al. Air classifier technology (ACT) in dry powder inhalation Part 4. Performance of air classifier technology in the Novolizer multi-dose dry powder inhaler. Int J Pharm 2006;310(1-2):81-9
- Friebel C, Steckel H, Muller BW. Rational design of a dry powder inhaler: device design and optimisation. J Pharm Pharmacol 2012;64(9):1303-15
- Donovan MJ, Kim SH, Raman V, Smyth HD. Dry powder inhaler device influence on carrier particle performance. J Pharm Sci 2012;101(3):1097-107
- Wang ZL, Grgic B, Finlay WH. A dry powder inhaler with reduced mouth-throat deposition. J Aerosol Med 2006; Summer 19(2):168-74
- Crowder TM, Donovan MJ. Chapter 9: science and technology of dry powder inhaler In: Smyth HD, Hickey AJ, editors. Controlled pulmonary drug delivery. Springer; NewYork: 2011. p. 203-22
- Louey MD, Van Oort M, Hickey AJ. Standardized entrainment tubes for the evaluation of pharmaceutical dry powder dispersion. J Aerosol Sci 2006;37(11):1520-31
- Azouz W, Chetcuti P, Hosker HS, et al. The inhalation characteristics of patients when they use different dry powder inhalers. J Aerosol Medicine Pulm Drug Deliv 2014: 10.1089/jamp.2013.1119
- Clark AR, Hollingworth AM. The Relationship between Powder Inhaler Resistance and Peak Inspiratory Conditions in Healthy-Volunteers - Implications for in-Vitro Testing. J Aerosol Med 1993;6(2):99-110
- Kanabuchi K, Kondo T, Tanigaki T, et al. Minimal inspiratory flow from dry powder inhalers according to a biphasic model of pressure vs. flow relationship. Tokai J Exp Clin Med 2011;36(1):1-4
- Hira D, Okuda T, Ichihashi M, et al. Influence of peak inspiratory flow rates and pressure drops on inhalation performance of dry powder inhalers. Chem Pharm Bull 2012;60(3):341-7
- Hoppentocht M, Hagedoorn P, Frijlink HW, de Boer AH. Technological and practical challenges of dry powder inhalers and formulations. Adv Drug Deliv Rev 2014;73C:18-31
- Malmberg LP, Rytila P, Happonen P, Haahtela T. Inspiratory flows through dry powder inhaler in chronic obstructive pulmonary disease: age and gender rather than severity matters. Int J Chron Obstruct Pulmon Dis 2010;5:257-62
- Zeng XM, Martin GP, Marriott C. Chapter 5: particulate interactions in dry powder aerosols. Particulate interactions in dry powder formulations for inhalation. Taylor & Francis Inc; New York, NY: 2001. p. 133-73
- Li X, Vogt FG, Hayes DJr, Mansour HM. Design, characterization, and aerosol dispersion performance modeling of advanced co-spray dried antibiotics with mannitol as respirable microparticles/nanoparticles for targeted pulmonary delivery as dry powder inhalers. J Pharm Sci 2014;103(9):2937-49
- Park CW, Li X, Vogt FG, et al. Advanced spray-dried design, physicochemical characterization, and aerosol dispersion performance of vancomycin and clarithromycin multifunctional controlled release particles for targeted respiratory delivery as dry powder inhalation aerosols. Int J Pharm 2013;455(1-2):374-92
- Mansour HM, Park CW, Hayes DJr. Chapter 3: nanoparticle lung delivery and inhalation aerosols for targeted pulmonary nanomedicine. Nanomedicine in drug delivery. CRC Press Taylor & Francis group; Boca Raton, FL, USA: 2013. p. 43-74
- Milenkovic J, Alexopoulos AH, Kiparissides C. flow and particle deposition in the Turbuhaler: a CFD simulation. Int J Pharm 2013;448(1):205-13
- Behara SR, Longest PW, Farkas DR, Hindle M. Development and comparison of new high-efficiency dry powder inhalers for carrier-free formulations. J Pharm Sci 2014;103(2):465-77
- Warren S, Taylor G, Smith J, et al. Gamma scintigraphic evaluation of a novel budesonide dry powder inhaler using a validated radiolabeling technique. J Aerosol Med 2002;15(1):15-25
- Glover W, Chan HK, Eberl S, et al. Effect of particle size of dry powder mannitol on the lung deposition in healthy volunteers. Int J Pharm 2008;349(1-2):314-22
- Steckel H, Muller BW. In vitro evaluation of dry powder inhalers. 1. Drug deposition of commonly used devices. Int J Pharm 1997;154(1):19-29
- Heng D, Lee SH, Ng WK, et al. Novel alternatives to reduce powder retention in the dry powder inhaler during aerosolization. Int J Pharm 2013;452(1-2):194-200
- Zhou QT, Tong Z, Tang P, et al. Effect of device design on the aerosolization of a carrier-based dry powder inhaler–a case study on Aerolizer((R)) Foradile ((R)). AAPS J 2013;15(2):511-22
- Behara SR, Farkas DR, Hindle M, Longest PW. Development of a high efficiency dry powder inhaler: effects of capsule chamber design and inhaler surface modifications. Pharm Res 2014;31(2):360-72
- Coates MS, Chan HK, Fletcher DF, Raper JA. Effect of design on the performance of a dry powder inhaler using computational fluid dynamics. Part 2: air inlet size. J Pharm Sci 2006;95(6):1382-92
- Chrystyn H, Niederlaender C. The Genuair(R) inhaler: a novel, multidose dry powder inhaler. Int J Clin Pract 2012;66(3):309-17
- Chow AH, Tong HH, Chattopadhyay P, Shekunov BY. Particle engineering for pulmonary drug delivery. Pharm Res 2007;24(3):411-37
- Buxton DB. Nanomedicine for the management of lung and blood diseases. Nanomedicine 2009;4(3):331-9
- Xu LM, Zhang QX, Zhou Y, et al. Engineering drug ultrafine particles of beclomethasone dipropionate for dry powder inhalation. Int J Pharm 2012;436(1-2):1-9
- Momin MN, Hedayati A, Nokhodchi A. Investigation into alternative sugars as potential carriers for dry powder formulation of budesonide. BI 2011;1(2):105-11
- Rahimpour Y, Kouhsoltani M, Hamishehkar H. Alternative carriers in dry powder inhaler formulations. Drug Discov Today 2014;19(5):618-26
- Willis L, Hayes DJr, Mansour HM. Therapeutic liposomal dry powder inhalation aerosols for targeted lung delivery. Lung 2012;190(3):251-62
- Vandevanter DR, Geller DE. Tobramycin administered by the TOBI((R)) Podhaler((R)) for persons with cystic fibrosis: a review. Med Devices 2011;4:179-88
- McKeage K. Tobramycin inhalation powder: a review of its use in the treatment of chronic Pseudomonas aeruginosa infection in patients with cystic fibrosis. Drugs 2013;73(16):1815-27
- Menon JU, Ravikumar P, Pise A, et al. Polymeric nanoparticles for pulmonary protein and DNA delivery. Acta biomaterialia 2014
- Cheow WS, Chang MW, Hadinoto K. Antibacterial efficacy of inhalable levofloxacin-loaded polymeric nanoparticles against E. coli biofilm cells: the effect of antibiotic release profile. Pharm Res 2010;27(8):1597-609
- Meenach SA, Anderson KW, Zach Hilt J, et al. Characterization and aerosol dispersion performance of advanced spray-dried chemotherapeutic PEGylated phospholipid particles for dry powder inhalation delivery in lung cancer. Eur J Pharm Sci 2013;49(4):699-711
- Meenach SA, Vogt FG, Anderson KW, et al. Design, physicochemical characterization, and optimization of organic solution advanced spray-dried inhalable dipalmitoylphosphatidylcholine (DPPC) and dipalmitoylphosphatidylethanolamine poly(ethylene glycol) (DPPE-PEG) microparticles and nanoparticles for targeted respiratory nanomedicine delivery as dry powder inhalation aerosols. Int J Nanomedicine 2013;8:275-93
- Bhavane R, Karathanasis E, Annapragada AV. Agglomerated vesicle technology: a new class of particles for controlled and modulated pulmonary drug delivery. J Control Release 2003;93(1):15-28
- Duan J, Vogt FG, Li X, et al. Design, characterization, and aerosolization of organic solution advanced spray-dried moxifloxacin and ofloxacin dipalmitoylphosphatidylcholine (DPPC) microparticulate/nanoparticulate powders for pulmonary inhalation aerosol delivery. Int J Nanomedicine 2013;8:3489-505
- Yang M, Yamamoto H, Kurashima H, et al. Design and evaluation of poly(DL-lactic-co-glycolic acid) nanocomposite particles containing salmon calcitonin for inhalation. Eur J Pharm Sci 2012;46(5):374-80
- Ragab DM, Rohani S. Cubic magnetically guided nanoaggregates for inhalable drug delivery: in vitro magnetic aerosol deposition study. AAPS PharmSciTech 2013;14(3):977-93
- Al-Hallak MH, Sarfraz MK, Azarmi S, et al. Distribution of effervescent inhalable nanoparticles after pulmonary delivery: an in vivo study. Ther deli 2012;3(6):725-34
- Longest PW, Hindle M. Condensational growth of combination drug-excipient submicrometer particles for targeted high efficiency pulmonary delivery: comparison of CFD predictions with experimental results. Pharm Res 2012;29(3):707-21
- Hindle M, Longest PW. Condensational growth of combination drug-excipient submicrometer particles for targeted high-efficiency pulmonary delivery: evaluation of formulation and delivery device. J Pharm Pharmacol 2012;64(9):1254-63
- Longest PW, Son YJ, Holbrook L, Hindle M. Aerodynamic factors responsible for the deaggregation of carrier-free drug powders to form micrometer and submicrometer aerosols. Pharm Res 2013;30(6):1608-27
- Torrisi BM, Birchall JC, Jones BE, et al. The development of a sensitive methodology to characterise hard shell capsule puncture by dry powder inhaler pins. Int J Pharm 2013;456(2):545-52
- Administration USFaD. Available from: www.fda.gov/drugs/drugsafety/informationbydrugclass/ucm082370.htm [Cited 13 June 2014]
- European Medicines Agency. Available from: www.ema.europa.eu/ema/index.jsp?curl=pages/medicines/landing/epar_search.jsp&mid=WC0b01ac058001d124 [Cited 14 July 2014]
- Duddu SP, Sisk SA, Walter YH, et al. Improved lung delivery from a passive dry powder inhaler using an Engineered PulmoSphere powder. Pharm Res 2002;19(5):689-95
- Meakin BJ, Ganderton D, Panza I, Ventura P. The effect of flow rate on drug delivery from the Pulvinal, a high-resistance dry powder inhaler. J Aerosol Med 1998; Fall 11(3):143-52
- Janssens W, VandenBrande P, Hardeman E, et al. Inspiratory flow rates at different levels of resistance in elderly COPD patients. Eur Respir J 2008;31(1):78-83
- Vogelberg C, Kremer HJ, Ellers-Lenz B, et al. Clinical evaluation of the peak inspiratory flow generated by asthmatic children through the Novolizer. Respir Med 2004;98(10):924-31
- Lobo JM, Schiavone H, Palakodaty S, et al. SCF-engineered powders for delivery of budesonide from passive DPI devices. J Pharm Sci 2005;94(10):2276-88
- Nelson H, Kemp JP, Bieler S, et al. Comparative efficacy and safety of albuterol sulfate Spiros inhaler and albuterol metered-dose inhaler in asthma. Chest 1999;115(2):329-35
- Vehring R. Webinar: introduction to the formulation science and technology of respiratssory drug delivery. 2014. Available from: https://live.blueskybroadcast.com/bsb/client/CL_DEFAULT.asp?Client=825785&CAT=8739&PCAT=3932 [Cited 18 July 2014]