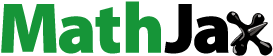
Abstract
Introduction
The current study was designed to synthesize derivatives of succinimide and compare their biological potency in anticholinesterase, alpha-glucosidase inhibition, and antioxidant assays.
Methods
In this research, two succinimide derivatives including (S)-1-(2,5-dioxo-1-phenylpyrrolidin-3-yl) cyclohexanecarbaldehyde (Compound 1) and (R)-2-((S)-2,5-dioxo-1-phenylpyrrolidin-3-yl)-2-phenylpropanal (Compound 2) were synthesized using Michael addition. Both the compounds, ie, 1 and 2 were evaluated for in-vitro acetylcholinesterase (AChE), butyrylctcholinesterase (BChE), antioxidant, and α-glucosidase inhibitory potentials. Furthermore, molecular docking was performed using Molecular Operating Environment (MOE) to explore the binding mode of both the compounds against different enzymes. Lineweaver–Burk plots of enzyme inhibitions representing the reciprocal of initial enzyme velocity versus the reciprocal of substrate concentration in the presence of synthesized compounds and standard drugs were constructed using Michaelis–Menten kinetics.
Results
In AChE inhibitory assay, compounds 1 and 2 exhibited IC50 of 343.45 and 422.98 µM, respectively, against AChE enzyme. Similarly, both the compounds showed IC50 of 276.86 and 357.91 µM, respectively, against BChE enzyme. Compounds 1 and 2 displayed IC50 of 157.71 and 471.79 µM against α-glucosidase enzyme, respectively. In a similar pattern, compound 1 exhibited to be more potent as compared to compound 2 in all the three antioxidant assays. Compound 1 exhibited IC50 values of 297.98, 332.94, and 825.92 µM against DPPH, ABTS, and H2O2 free radicals, respectively. Molecular docking showed a triple fold in the AChE and BChE activity for compound 1 compared with compound 2. The compound 1 revealed good interaction against both the AChE and BChE enzymes which revealed the high potency of this compound compared to compound 2.
Conclusion
Both succinimide derivatives exhibited considerable inhibitory activities against cholinesterases and α-glucosidase enzymes. Of these two, compound 1 revealed to be more potent against all the in-vitro targets which was supported by molecular docking with the lowest binding energies. Moreover, compound 1 also proved to have antiradical properties.
Introduction
Alzheimer’s disease (AD) is a neurodegenerative disorder of the aging people and is the common cause of dementia. AD is clinically characterized by progressive cognitive decline, behavioral turbulence, and imperfection in routine activities.Citation1,Citation2 Although the complex pathophysiological aspects of AD are not quite clear, yet numerous therapeutic agents including cholinesterase (AChE/BChE) inhibitors, BACE1 inhibitors, metal chelators, amyloid-β-peptide vaccination, cholesterol-lowering, and anti-inflammatory agents have been tested as potential AD therapeutics.Citation3–Citation5
Acetylcholine is an important neurotransmitter implicated in the communication of impulses across the synapse.Citation6–Citation8 This neurotransmitter is cleared by two vital enzymes, acetylcholinesterase (AChE) and butyrylcholinesterase (BChE).Citation9,Citation10 In Alzheimer’s disease, cholinergic neurons are more effected and there is deficiency of acetylcholine (ACh) at the synaptic cleft. Consequently, inhibiting enzymes involved in the degradation of ACh at synapse is an important and successful tool to restore its level and activity.Citation11,Citation12 Till now, among the five clinically approved drugs for AD, four are cholinesterase inhibitors.Citation13 The commercially available inhibitors of AChE such as rivastigmine, donepezil, and galanthamine might also help to prevent, for a constrained time, some signs from turning into worse for a few people in the early and middle levels of the disease.Citation14,Citation15 This signifies the search for more useful and safe cholinesterase inhibitors for the effective management of AD. In ongoing research, our group synthesized numerous synthetic inhibitors of AChE and BChE for the potential management of AD.
Oxidative stress mediated by free radicals is implicated in the progression of various diseases including neurological disorders.Citation16,Citation17 Free radicals are generated in the body during normal metabolic process and are neutralized by immune system antioxidant enzymes like catalase, superoxide dismutase, glutathione, and hydroperoxidase. However, due to excessive generation of the free radicals or suppression of immune system leads to improper combating of free radicals, which leads to oxidative stress. Further, amyloid beta (Aβ) is an abnormal protein generated from amyloid precursor protein (APP) through the action of beta amyloid cleaving enzyme 1 (BACE1) causing the formation of amyloid plaques.Citation18 Once Aβ is generated, it accumulates around the neurons, causing their deterioration and disturb electron transport chain which can cause mitochondrial dysfunction and inflammation.Citation11 Aβ is considered to be mitochondrial poison causing excessive release of free radicals, which disrupt mitochondrial function, electron transport chain, and function of immune system antioxidant enzymes like catalase and hydroperoxides. Consequently, using antioxidants and anti-inflammatory agents can potentially sluggish down AD development and neurodegeneration.Citation7,Citation11,Citation19 In this regard, supplementation of exogenous antioxidants has a vital impact in stopping various illnesses, such as cancer and cardiovascular diseases, and in delaying the growing older populations.Citation20
Diabetes mellitus (DM) is another metabolic disorder associated with excessive blood glucose levels within the body. DM is a persistent ailment of metabolism due to either less secretion of insulin or the cells of the body not responding properly to the insulin produced and resist the action of insulin.Citation20,Citation21 It is characterized by excessive blood sugar level (hyperglycemia) in postprandial and is followed by keto-acidosis and protein-losing.Citation23 α-Glucosidase is a vital biological target/enzyme which catalyzes the degradation of diet polysaccharides to monosaccharide. Naturally or synthetically prepared glucosidase inhibitors are of therapeutic interest to put off postprandial hyperglycemia in type 2 diabetes. Amongst those, saccharine derivatives, for example, miglitol and acarbose have been approved anti-diabetic drugs.Citation24 Commercially available α-glucosidase inhibitors, which include voglibose, acarbose, and miglitol are currently used against DM. However, all of these drugs are accompanied by adverse effects like renal tumors, hepatic injury, abdominal pain, diarrhea, and flatulence. Therefore, the scientists are in constant search of safe and effective alternatives for the management of DM.25
Succinimides or 2.5-dioxopyrrolidines, a well-known class of drugs, possess a variety of biological potentials.Citation26,Citation27 The basic structure can be derivatized by various aryl and/or alkyl groups on nitrogen or carbon positions.Citation28,Citation29 Formerly, succinimides had been synthesized through various methods.Citation30 The primary nucleus of succinimides can be replaced by employing each C and N-position substitutions, leading to diverse derivatives having various functionalities. We have previously synthesized the keto-esters derivatives (ethyl 3-oxo-2-(2,5-dioxopyrrolidin-3-yl)butanoate) of succinimide with their positive anticholinesterase and antioxidant potentials.Citation31 The C and N substituted succinimides showed antimicrobial,Citation32 antitumor,Citation33 analgesic,Citation34 anti-inflammatory,Citation35 and antispasmodic activities.Citation36 Apart from their biological significance, they also find an application in the liquid crystal displays (LCD) and the production of water-soluble reactive copolymers and polymers.Citation28
Basically, succinimide class of drugs is known as anticonvulsant in the market. And, also previously we have reported the ketoester derivatives of succinimides as anti-Alzheimer’s agents.Citation31 So, being a class of drugs for neuropharmacology based on previously evaluated nucleus of pyrrolidine-2,5-dione, this study was a proposed rationale for the designed compounds as anti-Alzheimer’s agents. Secondly, the pyrrolide-2,5-dione moiety has a close structural resemblance with thiazolidinedione which is a known class of anti-diabetic drugs as shown in . This is also obvious from previous reported studies that asymmetric nitrogenous molecules are important building blocks of medicinal importance and may possesses diverse pharmacological potential.Citation37–Citation40
Figure 1 (A) General structure of aldehyde derivatives of succinimides, (B) Generic structure of thiazolidinedione class of anti-diabetic drugs

Based on the importance of succinimide scaffold and the dire need of the novel drugs, the current study has been designed in an attempt to synthesize potential derivatives of succinimide and to evaluate them for various biological activities including the anti-Alzheimer’s and anti-diabetic using in-vitro and in-silico simulation approaches.
Materials and Methods
Chemicals and Drugs
For synthesis of succinimides, all chemicals, reagents and solvents, which include N-phenyl-maleimide (CAT No. A14616), cyclohexane carboxaldehyde (CAT No. 108464), 2-phenyl propionaldehyde (CAT No. 241369), potassium hydroxide (CAT No. 757551), ethyl acetate (EtOAc), dichloromethane (DCM), dimethyl sulfoxide (DMSO), methanol, n-hexane, and analytical chromatographic plates (TLC Silica gel 60 F254) for reaction progresses observation were purchased from Sigma-Aldrich USA. For inhibition assays of cholinesterase, AChE electric eel (CAT No. C 1682) and BChE (lyophilized equine serum (CAT No C 1057) were purchased from authorized dealers of Sigma-Aldrich USA, in Pakistan. While substrates naming acetylthiocholine iodide (CAT No.A 22300) and butyrylthiocholine iodide (CAT No. B 3253-5G) along with indicator substance, 5,5-dithio-bis-nitrobenzoic acid (DTNB) CAT No. D 218200 and a standard drug galanthamine hydrobromide Lycoris Sp. (CAT No. Y0001190) were acquired from Sigma-Aldrich, UK.. For antioxidant assays and α-glucosidase studies, DPPH (CAT No. D 9132 Sigma-Aldrich USA), ABTS (CAT No. 10102946001 Sigma-Aldrich USA), Gallic acid (CAT No. G 7384 USA), and α-glucosidase from Saccharomyces cerevisiae (CAT No. G 0660) were purchased from Sigma-Aldrich USA, while acarbose (CAT No. A 8980) and ascorbic acid were acquired from Sigma-Aldrich France.1H and 13C-NMR spectra were recorded in deuterated solvents on a Bruker spectrometer at 400 and 100 MHz, respectively, using tetramethyl silane (TMS) as internal reference.
Chemistry
Both of these compounds were synthesized as per the previously reported procedure.Citation41 Generally, L-isoleucine (0.1 mol%, 13.12 mg) and potassium hydroxide (0.1 mol%, 5.6 mg) were added into a small reaction vessel containing DCM (1M) to make a non-covalent catalyst assembly. Afterwards, excess amount (2.0 equivalent) of the respective aldehydes (cyclohexanecarboxaldehyde and 2-phenyl-propionaldehyde) were added into each reaction and stirred for 2 min at ambient temperature to form enamine. Added one equivalent ratio of phenyl-maleimide with continuously stirring at room temperature. The reaction progresses were checked with the help of TLC (thin layer chromatography). Silica plates 60 (TLC) were used to perceive the reactions and then observed under UV (254 nm) to detect reaction spot. Solvent system was n-hexane and ethyl acetate and in 60:40 ratio, respectively. Once the reactions completed, it was diluted with distilled water (H2O) and extracted three times with dichloromethane using separating funnel. The organic layer was collected and dried in a rotary evaporator. Then purified with the help of column chromatography (silica gel 60; 0.063-0.200 mm) using ethyl acetate and n-hexane as eluents. The Rf values of the synthesized compounds 1 and 2 were 0.46 and 0.42, respectively. Synthetic schemes of the compounds are presented in .
(S)-1-(2,5-Dioxo-1-Phenylpyrrolidin-3-yl) Cyclohexanecarbaldehyde (Compound 1)
1H NMR (400 MHz, CDCl3) (ppm): 1.33–1.55 (m, 7H), 1.76–1.79 (m, 1H), 1.84–1.88 (m, 2H), 2.58 (dd, J = 18.17, 5.91 Hz, 1H), 2.78 (dd, J = 18.16, 9.48 Hz, 1H), 3.13 (dd, J = 9.46, 5.89 Hz, 1H), 7.18–7.22 (m, 2H), 7.29–7.32 (m, 1H), 7.36–7.41 (m, 2H), 9.45 (s, 1H). (Figure S1).
13C NMR (100 MHz, CDCl3) (ppm): 21.28, 21.47, 25.18, 28.15, 28.69, 31.63, 42.76, 52.25, 126.72, 128.74, 129.23, 132.02, 174.96, 177.19, 204.70. (Figure S2).
(R)-2-((S)-2,5-Dioxo-1-Phenylpyrrolidin-3-yl)-2-Phenylpropanal (Compound 2)
1H NMR (400 MHz, CDCl3) (ppm): 1.79 (s, 3H), 2.53 (dd, J = 18.91, 4.65 Hz, 1H), 2.99 (dd, J = 18.92, 9.45 Hz, 1H), 3.83 (dd, J = 9.45, 4.64 Hz, 1H), 7.05.7.10 (m, 2H), 7.26–7.30 (m, 2H), 7.36–7.46 (m, 6H), 9.69 (s, 1H).13C NMR (100 MHz, CDCl3) (ppm): 16.54, 21.05, 31.37, 33.54, 40.18, 42.51, 51.99, 123.67, 125.99, 127.96, 128.06, 128.60, 128.67, 128.71, 130.97, 133.44, 135.56, 173.64, 176.94, 200.57 (Figures S3 and 4).
Anti-Cholinesterase Studies
The synthesized compounds were tested against cholinesterase inhibitory potentials following Ellman’s assay.Citation42 The assays are based on acetylthiocholine iodide or butyrylthiocholine iodide hydrolysis by particular enzymes AChE and BChE upon the addition of 5-thio-2-nitrobenzoate anion which give yellow colour complex. The resultant yellow color compound is detected with spectrophotometer.
Preparation of Solutions
Our tested samples were dissolved in methanol, ranging from 15.62 to 1000 µg/mL. The dilutions of AChE (518 U/mg) and BChE (7–16 U/mg) were freshly prepared in phosphate buffer with pH 8 until the concentrations of 0.03 and 0.01 U/mL, respectively, were achieved. Along with that, solutions of DTNB (0.2273 mM), ATChI and BTChI (0.5 mM), each in distilled water were prepared and were preserved in air-tight Eppendorf tubes in the refrigerator at the temperature of 8°C for 15 min as a final solution. A calculated positive control drug (galantamine) was solubilized in methanol having the same concentrations as mentioned before.
Spectroscopic Analysis
Using UV spectroscopic analysis, previously prepared solutions of an enzyme (5 μl) was taken in cleaned cuvette, then our tested samples (205 μl), and finally added reagent DTNB (5 μl). The resultant three reagents mixture was incubated for fifteen min at 30°C using a water bath. Afterwards, 5 μL of the substrate was added. Absorbance was recorded via double beam UV spectrophotometer (Model UV-1800, Japan) at 412 nm. For negative control, cuvette contained all components except test samples. Whereas in the case of positive control, galantamine (10 µg/mL) was used as standard. UV absorbance values, for 4 min at 30°C, beside the reaction time were recorded.Citation43 All experiments were performed in triplicate and inhibitory potential values were obtained as
where, V is the rate of reaction of the tested sample which is A2-A1 and Vmax is the enzyme activity in the absence of inhibitor or tested drug.
Antioxidant Studies
DPPH Radicals Scavenging Assay
The synthesized compounds were screened for antiradical potentials against DPPH inhibitory analysis following our previously reported method.Citation44,Citation45 Different dilutions of tested compounds were prepared (15.62–1000 µg/mL) in methanol, 0.1 mL of each was mixed with 3000 μL of the previously prepared DPPH solution. The tested samples were incubated for 30 min at 25°C and the absorbance values were noted at 517 nm using a spectrophotometer. Ascorbic acid was used as a standard drug in this assay. In this assay, each concentration was taken in triplicate and the data obtained were presented as mean ± SEM. The % scavenging activity was calculated using the following standard formula:
ABTS Free Radicals Scavenging Assay
Further confirming antioxidant potentials of synthesized succinimides, free radicals scavenging of 2, 2-azinobis [3 ethylbenzthiazoline]- 6-sulfonic acid (ABTS).Citation46,Citation47 The mixture of ABTS 7 mM and potassium persulfate 2.45 mM was prepared. The solution was kept in a dark cabinet for the production of free radicals. The absorbance of ABTS solution was adjusted to 0.7 at 745 nm using methanol (50%) as diluent. To execute the assay, ABTS solution (3 ml) was mixed with samples (300 μl) and incubated at 25°C for 15 minutes. The absorbance of the incubated mixture was recorded at 745 nm. Ascorbic acid was used as a positive control. The procedure was implicated in triplicate and ABTS free radicals scavenging activity was obtained in percentage using the formula;
Hydrogen Peroxide Free Radicals Scavenging Assay
The hydrogen peroxide scavenging activity of the samples was conducted following the procedure of Ruch et al.Citation20 Hydrogen peroxide solution (2 mM) was prepared in 50 mM phosphate buffer having pH of 7.4. Various samples having volume of 0.1 mL were taken in test tubes and their volumes were made 0.4 mL by addition of 50 mM phosphate buffer. Hydrogen peroxide solution (0.6 mL) was added to it and vortexed. After 10 min, the absorbance of each sample was measured at 230 nm against the blank. The hydrogen peroxide scavenging activity was measured using the following standard formula:
α-Glucosidase Inhibition Assay
α-Glucosidase inhibitory activity was carried out with chromogenic assay.Citation48 The solution of α-glucosidase was prepared to have concentration of 0.5 unit/mL. The freshly prepared solution (20 μL) was mixed thoroughly with phosphate buffer (pH 6.9) having a volume of 120 μL. Likewise, p-nitrophenyl- α-D-glucopyranoside substrate solution (5 mM) was prepared in the same buffer. Then, the test samples with concentration ranges of 31.25–1000 μL having volume 10 μL of the test sample were added to them in a test tube and the sample mixture was kept back for 15 min at 37°C. After 15 min of incubation, the substrate solution having a volume of 20 μL was added and once again incubated for the same time at the same temperature. After incubation, 0.2 M sodium carbonate solution (80 μL) was added to terminate the reaction. Finally, the optical densities of the samples were measured via double beam spectrophotometer at 405 nm. Acarbose served as a positive control. The calculation of percent enzyme inhibition was carried out using the following equation;
Molecular Docking Studies
Molecular docking was performed using Dock Module implemented in Molecular Operating Environment (MOE) softwareCitation49,Citation50 to explore the binding mode of the tested compounds against acetylcholinesterase (AChE), butyrylcholinesterase (BChE), and alpha-glucosidase enzymes. First, the 3D structures for both the compounds were generated using MOE-builder module implemented in MOE. Next, the compounds were protonated, and energy minimized using the default parameters of the MOE (gradient: 0.05, Force Field: MMFF94X). The structural coordinates for AChE and BChE were retrieved from protein databank (PDB code; 1acl and 1p0p). Due to the unavailability of the crystallographic structure of the α-glucosidase enzyme, we have used the homology model described by Carreiro et al for docking purpose. All the structure was subjected to MOE for preparation.
Further, the protonation was done using default parameters of structure preparation module of MOE. Next, all the structure was subjected to energy minimization to get minimal energy conformation. Finally, refined structures were used for docking study using the default parameters of MOE; Placement: Triangle Matcher, rescoring 1: London dG, Refinement: Forcefield, Rescoring 2: GBVI/WSA. Before running the docking protocol, we have selected a total of ten conformations for the ligand. The top-ranked conformations based on docking score were selected for protein–ligand interaction (PLI) analysis.
Statistical Analysis
All experiments were performed in triplicate and data was presented as mean ±SEM. One-way ANOVA followed by multiple comparison DUNNET test was used for the statistical comparison of test compounds with standard drugs. P-value < 0.05 was considered as statistically significant.
Results and Discussion
Cholinesterase Inhibition Studies
In the current study, two potent cholinesterase inhibitors were selected from the synthesized compounds for detailed study. The anticholinesterase potentials of both compounds (S)-1-(2,5-dioxo-1-phenylpyrrolidin-3-yl) cyclohexanecarbaldehyde and (R)-2-((S)-2,5-dioxo-1-phenylpyrrolidin-3-yl)-2-phenylpropanal have been recorded to be significant in comparison with the standard drug galanthamine. Among the two compounds, (S)-1-(2,5-dioxo-1-phenylpyrrolidin-3-yl) cyclohexanecarbaldehyde has shown higher enzyme inhibition with IC50 values of 343.45 and 276.86 μM against AChE and BChE, respectively (). Similarly, (R)-2-((S)-2,5-dioxo-1-phenylpyrrolidin-3-yl)-2-phenylpropanal showed IC50 values of 422.98 and 357.91 μM against AChE and BChE, respectively. Briefly, it may be concluded that both compounds have considerable inhibitory activities against AChE and BChE and could be possibly effective against Alzheimer’s disease.
Table 1 Results of Cholinesterase Inhibitory Potentials of Synthesized Compounds
Numerous researchers are trying to explore novel compounds which could surpass the efficacy of galanthamine (anti-Alzheimer’s disease), acarbose (anti-diabetic), and many more.Citation51,Citation52 Various drugs are still under trials to get approved by the FDA. The exploration should go onwards to get efficacious, feasible, and economic drugs with eternal and easy availability. So, the current research work is an attempt to sort out some novel potential compounds which could be a panacea soon.
To cure a specific disease determines the target in the etiology of that specific disease. As this is known from decades that AD is effectively managed by those drugs which stop the breakdown of neurotransmitter acetylcholine (ACh) and thus increasing the concentration in the body. By effectively blocking the enzyme responsible for the breakdown of acetylcholine, ie, acetylcholinesterase/butyrylcholinesterase, the amount of acetylcholine could be an increase in the synaptic cleft and hence the symptoms of AD are avoided.Citation53
Antioxidant Studies
Oxidative stress is implicated in the progression of various diseases including AD and DM. The etiological factors of the majority of diseases have been sorted out to be the oxidative stress, which is developed due to increased concentration of free radicals inside our body including the brain. The free radicals could be able to initiate neuronal anomalies which lead to AD.Citation54 The free radicals have also been reported to be one of the etiological factors of diabetes mellitus.Citation55 So in the current study, it has also been evidenced that the synthesized compounds can effectively scavenge the free radicals, ie, the DPPH, ABTS, and H2O2. Therefore, in the current research project, the succinimide derivatives have proved to be effective against free radicals, AD, and diabetes mellitus.
The synthesized compounds showed considerable DPPH radicals scavenging as shown in . The antioxidant potential of compound 1 has been recorded to be comparatively higher than 2. In comparison with ascorbic acid, the results of compound 1 are encouraging which can be depicted by the IC50 values (IC50 of compound 1 = 297.89 μM, IC50 of Ascorbic acid = 96.53 μM). The DPPH assay shows that the range of concentrations from 15.62 to 1000 μg/mL is active against the free radicals.
Table 2 DPPH Free Radicals Scavenging Activity Results
The ABTS free radicals scavenging assay has been tabulated as . Compound 1 has shown significant antioxidant potential by inhibiting 79.87±1.27***, 67.31±0.58***, 61.22±1.28***, 54.49±0.60***, 40.51±0.54***, 26.87±0.85***, 16.60 ±1.63*** percent ABTS free radicals at the concentrations of 1000 to 15.62 μg/mL, respectively. The IC50 calculated for compound 1 and standard sample (ascorbic acid) were 332.94 and 85.17 μM, respectively. In the same way, the antioxidant activity of compound 2 was also recorded to be noteworthy as shown in .
Table 3 ABTS Free Radicals Scavenging Activity Results
The results of hydrogen peroxide free radicals scavenging have been summarized in . It is obvious from the table, that compound 1 was observed to be more potent than compound 2 in this assay. The observed IC50 values for compounds 1 and 2 were 45 and 145 µM, respectively. In comparison, the standard drug ascorbic acid exhibited an IC50 of 26 µM.
Table 4 Hydrogen per Oxide (H2O2) Free Radicals Scavenging Activity Result
α-Glucosidase Inhibitory Assay
represents the summarized results of the a-glucosidase assay. The percent a-glucosidase inhibition by compound 1 goes parallel with the results of standard drug, ie, acarbose. The IC50 value of compound 1 and acarbose was recorded as 157.71 and 40.27 μM, respectively. The percent enzyme inhibition of compound 1 was calculated as 79.58±1.12, 72.90±0.96, 68.77±1.24, 58.76±0.71, 44.90±1.55***, 34.42±0.43***, and 28.03 ±0.35*** at the concentrations of 1000, 500, 250, 125, 62.5, 31.25, and 15.62 μg/mL, respectively. Similarly, compound 2 also demonstrated notable enzyme inhibition.
Table 5 Alpha-Glucosidase Inhibitory Potentials of Succinimide Derivatives
Diabetes mellitus (DM) is indicated by the increased blood glucose level in the body due to a metabolic disorder of carbohydrates, fats and proteins. Two main reasons are there for increased concentration of glucose, ie, decreased secretion of insulin and cellular resistance to the action of insulin.Citation56 The postprandial concentration of glucose could be effectively decreased by the acarbose, which is the α-glucosidase inhibitor drug.Citation57 The α-glucosidase is responsible for the conversion of large units of carbohydrates into glucose units which is effectively absorbed from the intestine. As far as the α-glucosidase inhibition is concerned, the succinimide derivatives in our current study have shown considerable inhibitions of this enzyme which goes parallel with the activity of standard drug acarbose.
Molecular Docking Studies
Acetylcholinesterase and Butyrylcholinesterase
Molecular docking was performed to explore the binding mode of both compounds against the target enzyme (AChE and BChE). Molecular docking results showed good agreement with experimental results. We have noticed a triple fold in the AChE and BChE activity for compound 1 compared with compound 2.
Similarly, molecular docking results for compound 1 revealed good interaction against both the AChE and BChE enzymes (), which evident the high potency of this compound compared with compound 2. In the case of AChE, 1-methylpyrrolidin-2-one moiety of compound 1 involved in an interaction with the side chain and backbone donor of residue Gly118, Ser122. Also, residue Trp84 shared his pi-electron with the benzene ring via π-π interaction. Compound 2 only shared the π-electron with the Trp84 via π-π interaction. In the case of the docking results for BChE activity revealed that both the compounds shared a typical interaction with Trp82. Also, the compound 1 is involved in some additional interactions with essential residues in the active site; Gly116 and Trp430 (), while the compound 2, unfortunately, lack additional interaction, which indicate the decreased potency of compound 2 as compared with high potent compound 1 against both the enzymes.
Alpha-Glucosidase Inhibition
The molecular docking results for both the compounds against the α-glucosidase enzyme revealed that compound 1 showed best fit-well binding mode in the active site of the corresponding enzyme and adopted various interactions; Arg439 and Arg212 and some additional hydrophobic interaction, ie, Phe300 (). While compound 2 only showed interaction with Phe300, which evident the less potency of this compound compared with compound 1 against the corresponding enzyme. Overall, the molecular docking results delineated that the result for compound 1 agrees with the experimental results as compared with the results for compound 2, which showed less PL profile. Most importantly, the compound 1 shared a common moiety (1-methylpyrrolidin-2-one) with various interaction with different residues, hence separate the high potent compound 1 from less potent compound 2.. The details for docking score for compound 1 and compound 2 are enlisted in . The 2D interaction for both compounds embedded in .
Figure 3 The PL interaction profiles for Compound 1 and Compound 2 against AChE, BChE, and α-glucosidase enzyme. (A–C) indicates the surface of the corresponding enzyme. (A1–C1) represent the PL interaction profile for compound 1 against AChE, BChE, and α-glucosidase enzyme. (A2–C2) Represent the PL interaction profile for compound 2 against AChE, BChE, and α-glucosidase enzyme. Compound 1 and Compound 2 were colored into Magenta, while residues into white. Hydrogen bonding is shown in black color dotted lines, and the both-sided arrows indicate the pi-stacking interaction.

Figure 4 Represent the 2D interaction for both compounds. (A1–C1) represent the 2D interaction for compound 1 against AChE, BChE, and α-glucosidase enzyme. (A2–C2) for compound 2 against AChE, BChE, and α-glucosidase enzyme.

Table 6 The Docking Score for Both the Compounds Against AChE, BChE, and α-Glucosidase Enzymes
Michaelis–Menten Kinetics
The synthesized compounds showed strong inhibitory potentials against acetylcholinesterase, butyrylcholinesterase, and glucosidase as revealed from the corresponding Vmax and Km values which were determined using Michaelis–Menten kinetics and confirmed from the Lineweaver–urk plots for the respective enzymes (–). For acetylcholinesterase inhibition, the Vmax and Km values were determined as 84.93µg/min and 69.70 µg/mL for Compound 1, and 78.65 µg/min and 63.17µg/mL for Compound 2, respectively. The standard galantamine showed a robust inhibition of acetylcholinesterase having a Vmax and Km values of 1.875 µg/min and 2.566 µg/mL and 1.488 µg/min and 2.633 µg/mL, respectively.
Lineweaver–Burk plots of acetylcholinesterase inhibition representing the reciprocal of initial enzyme velocity versus the reciprocal of substrate concentration in the presence of compound 1 and galantamine.
Figure 5 Lineweaver–Burk plots of acetylcholinesterase inhibition representing the reciprocal of initial enzyme velocity versus the reciprocal of substrate concentration in the presence of compound 1 and galantamine.

Figure 6 Lineweaver–Burk plots of acetylcholinesterase inhibition representing the reciprocal of initial enzyme velocity versus the reciprocal of substrate concentration in the presence of Compound 2 and galantamine.

Similarly, the Vmax and Km values for butyrylcholinesterase inhibition also revealed a potent inhibitory potential of Compound 1 (1.553 µg/min and 4.250 µg/mL) and Compound 2 (2.867 µg/min and 7.438 µg/mL). A high-grade inhibitory activity was observed with the standard galantamine (2.566 and 2.641 µg/min and 2.633 and 2.574 µg/mL, respectively).
Figure 7 Lineweaver–Burk plots of butyrylcholinesterase inhibition representing the reciprocal of initial enzyme velocity versus the reciprocal of substrate concentration in the presence of Compound 1 and the standard galantamine.

Figure 8 Lineweaver–Burk plots of butyrylcholinesterase inhibition representing the reciprocal of initial enzyme velocity versus the reciprocal of substrate concentration in the presence of Compound 2 and the standard galantamine.

The Lineweaver–Burk plots for the inhibition of α-glucosidase by compounds 1 and 2 and acarbose are shown in and . The dissociation constant (Km) and Vmax values of compound 1 for α-glucosidase were 5.017 µg/mL and 2.360 µg/min, respectively. Similarly, for compound 2, the Km and Vmax were observed as 7.565 µg/mL and 3.542 µg/min, respectively. The effect was comparable to that of standard, acarbose (2.328 and 3.176 µg/mL and 2.639 and 2.616 µg/min), thus showing potent inhibitory proclivity against α-glucosidase.
Conclusions
In conclusion, both succinimide derivatives, ie, (S)-1-(2,5-dioxo-1-phenylpyrrolidin-3-yl) cyclohexanecarbaldehyde (1) and (R)-2-((S)-2,5-dioxo-1-phenylpyrrolidin-3-yl)-2-phenylpropanal (2) exhibited considerable inhibitory activities against cholinesterases and α-glucosidase enzymes. Among both, compound 1 revealed more potent activity against all enzymes which was supported by molecular docking with the lowest binding energies against all enzymes. Likewise, compounds showed considerable anti-radical potentials and thus warranted further in-vivo studies for potential application in AD and diabetes.
Author Contributions
All authors contributed towards data analysis, drafting and critically revising the paper, gave final approval of the version to be published, and agreed to be accountable for all aspects of the work.
Disclosure
The authors report no conflicts of interest in this work.
Acknowledgments
The authors extend their appreciation to the Deanship of Scientific Research at King Saud University, Riyadh, Saudi Arabia for funding this work through research group No. 1441-341. AS is also grateful to the Higher Education Commission of Pakistan for NRPU project No. 10562/KPK/NRPU/R&D/HEC/2017.
References
- SadiqA, ZebA, UllahF, et al. Chemical characterization, analgesic, antioxidant, and anticholinesterase potentials of essential oils from Isodon rugosus wall. ex. Benth. Front Pharmacol. 2018;9.29422861
- AyazM, SadiqA, JunaidM, et al. Flavonoids as prospective neuroprotectants and their therapeutic propensity in aging associated neurological disorders. Front Aging Neurosci. 2019;11:155. doi:10.3389/fnagi.2019.0015531293414
- OvaisM, ZiaN, AhmadI, et al. phyto-therapeutic and nanomedicinal approach to cure Alzheimer disease: present status and future opportunities. Front Aging Neurosci. 2018;10:284. doi:10.3389/fnagi.2018.0028430405389
- ZafarR. et al. Zinc metal carboxylates as potential anti-Alzheimer’s candidate: in-vitro anticholinesterase, antioxidant and molecular docking studies. J Biomol Struct Dyn. 2020;1–15. doi:10.1080/07391102.2020.1724569
- LovemanE, GreenC, KirbyJ, et al. The clinical and cost-effectiveness of donepezil, rivastigmine, galantamine and memantine for Alzheimer’s disease. Health Tech Assess. 2006;10. doi:10.3310/hta10010
- AyazM, SadiqA, JunaidM, UllahF, SubhanF, AhmedJ. Neuroprotective and anti-aging potentials of essential oils from aromatic and medicinal plants. Front Aging Neurosci. 2017;9:168. doi:10.3389/fnagi.2017.0016828611658
- AyazM, SadiqA, JunaidM, et al. Flavonoids as prospective neuroprotectants and their therapeutic propensity in aging associated neurological disorders. Front Aging Neurosci. 2019;11.30837862
- AyazM, UllahF, SadiqA, KimMO, AliT. Natural products-based drugs: potential therapeutics against Alzheimer’s disease and other neurological disorders. Front Pharmacol. 2019;10:1417.31849668
- AhmadS, ZebA, AyazM, MurkovicM. Characterization of phenolic compounds using UPLC–HRMS and HPLC–DAD and anti-cholinesterase and anti-oxidant activities of Trifolium repens L. Leaves. Eur Food Res Tech. 2020;1–12.
- JabeenM, et al. SAR based in-vitro anticholinesterase and molecular docking studies of nitrogenous progesterone derivatives. Steroids. 2020;158:108599. doi:10.1016/j.steroids.2020.10859932126219
- AyazM, JunaidM, UllahF, et al. Anti-Alzheimer’s studies on β-sitosterol isolated from polygonum hydropiper L. Front Pharmacol. 2017;8:697. doi:10.3389/fphar.2017.0069729056913
- SultanaN, SarfrazM, TanoliST, et al. Synthesis, crystal structure determination, biological screening and docking studies of N1-substituted derivatives of 2, 3-dihydroquinazolin-4 (1H)-one as inhibitors of cholinesterases. Bioorg Chem. 2017;72:256–267. doi:10.1016/j.bioorg.2017.04.00928495556
- AyazM, JunaidM, UllahF, et al. Comparative chemical profiling, cholinesterase inhibitions and anti-radicals properties of essential oils from polygonum hydropiper L: a preliminary anti-Alzheimer’s study. Lipids Health Dis. 2015;14(1):141. doi:10.1186/s12944-015-0145-826530857
- AliM, MuhammadS, ShahMR, et al. Neurologically potent molecules from crataegus oxyacantha; isolation, anticholinesterase inhibition, and molecular docking. Front Pharmacol. 2017;8:327. doi:10.3389/fphar.2017.0032728638340
- YiannopoulouKG, PapageorgiouSG. Current and future treatments for Alzheimer’s disease. Ther Adv Neurol Disord. 2013;6(1):19–33. doi:10.1177/175628561246167923277790
- ShahS, MuhammadM, SadiqA, ShahSMH, UllahF. Antioxidant, total phenolic contents and antinociceptive potential of teucrium stocksianum methanolic extract in different animal models. BMC Complement Altern Med. 2014;14(1):181. doi:10.1186/1472-6882-14-18124893601
- AyazM, OvaisM, AhmadI, SadiqA, KhalilAT, UllahF. Biosynthesized metal nanoparticles as potential Alzheimer’s disease therapeutics. Metal Nanoparticles Drug Delivery Diagn Appl. 2020;31–42.
- SarfrazM, SultanaN, RashidU, AkramMS, SadiqA, TariqMI. Synthesis, biological evaluation and docking studies of 2, 3-dihydroquinazolin-4 (1H)-one derivatives as inhibitors of cholinesterases. Bioorg Chem. 2017;70:237–244. doi:10.1016/j.bioorg.2017.01.00428126287
- AhmadG, et al. Synthesis, in-vitro cholinesterase inhibition, in-vivo anticonvulsant activity and in-silico exploration of N-(4-methylpyridin-2-yl) thiophene-2-carboxamide analogs. Bioorg Chem. 2019;92:103216. doi:10.1016/j.bioorg.2019.10321631491567
- EruygurN, UcarE, AkpulatHA, ShahsavariK, SafaviSM, KahriziD. In vitro antioxidant assessment, screening of enzyme inhibitory activities of methanol and water extracts and gene expression in hypericum lydium. Mol Biol Rep. 2019;1–9.
- ZohraT, OvaisM, KhalilAT, QasimM, AyazM, ShinwariZK. Extraction optimization, total phenolic, flavonoid contents, HPLC-DAD analysis and diverse pharmacological evaluations of Dysphania ambrosioides (L.) Mosyakin & Clemants. Nat Prod Res. 2019;33(1):136–142. doi:10.1080/14786419.2018.143742829430965
- RahimH, SadiqA, KhanS, et al. Fabrication and characterization of glimepiride nanosuspension by ultrasonication-assisted precipitation for improvement of oral bioavailability and in vitro α-glucosidase inhibition. Int J Nanomed. 2019;14:6287. doi:10.2147/IJN.S210548
- AslamH, KhanA-U, NaureenH, AliF, UllahF, SadiqA. Potential application of Conyza canadensis (L) Cronquist in the management of diabetes: in vitro and in vivo evaluation. Trop J Pharm Res. 2018;17(7):1287–1293. doi:10.4314/tjpr.v17i7.9
- de MeloEB, da Silveira GomesA, CarvalhoI. α-and β-Glucosidase inhibitors: chemical structure and biological activity. Tetrahedron. 2006;62(44):10277–10302. doi:10.1016/j.tet.2006.08.055
- BarutB, BarutEN, EnginS, ÖzelA, SezenFS. Investigation of the antioxidant, α-Glucosidase inhibitory, anti-inflammatory, and DNA protective properties of vaccinium arctostaphylos L. Turkish J Pharm Sci. 2019;16:2. doi:10.4274/tjps.galenos.2018.28247
- JanMS, AhmadS, HussainF, et al. Design, synthesis, in-vitro, in-vivo and in-silico studies of pyrrolidine-2, 5-dione derivatives as multitarget anti-inflammatory agents. Eur J Med Chem. 2020;186:111863. doi:10.1016/j.ejmech.2019.11186331740050
- HussainF, KhanZ, JanMS, et al. Synthesis, in-vitro α-glucosidase inhibition, antioxidant, in-vivo antidiabetic and molecular docking studies of pyrrolidine-2, 5-dione and thiazolidine-2, 4-dione derivatives. Bioorg Chem. 2019;91:103128. doi:10.1016/j.bioorg.2019.10312831369977
- LovićJ, Avramov IvićM, BĐB, LađarevićJ, MijinD. Voltammetric investigation of inclusion complexes of the selected succinimides with β-cyclodextrin and (2-hydroxypropyl)-β-cyclodextrin. Acta Chim Slov. 2019;66:182–189. doi:10.17344/acsi.2018.4767
- AhmadA, UllahF, SadiqA, et al. Pharmacological evaluation of aldehydic-pyrrolidinedione against HCT-116, MDA-MB231, NIH/3T3, MCF-7 cancer cell lines, antioxidant and enzyme inhibition studies. Drug Des Devel Ther. 2019;13:4185. doi:10.2147/DDDT.S226080
- BibiA, ShahT, SadiqA, KhalidN, UllahF, IqbalA. l-Isoleucine-catalyzed michael synthesis of N-Alkylsuccinimide derivatives and their antioxidant activity assessment. Russian J Organic Chem. 2019;55(11):1749–1754. doi:10.1134/S1070428019110174
- SadiqA, MahmoodF, UllahF, et al. Synthesis, anticholinesterase and antioxidant potentials of ketoesters derivatives of succinimides: a possible role in the management of Alzheimer’s. Chem Cent J. 2015;9(1):31. doi:10.1186/s13065-015-0107-226064188
- MahmoodF, JanMS, AhmadS, et al. Ethyl 3-oxo-2-(2, 5-dioxopyrrolidin-3-yl) butanoate derivatives: anthelmintic and cytotoxic potentials, antimicrobial, and docking studies. Front Chem. 2017;5:119. doi:10.3389/fchem.2017.0011929312926
- WangF, YinH, YueC, ChengS, HongM. Syntheses, structural characterization, in vitro cytotoxicities and DNA-binding properties of triphenylantimony di (N-oxy phthalimide) and di (N-oxy succinimide) complexes. J Organomet Chem. 2013;738:35–40. doi:10.1016/j.jorganchem.2013.03.046
- PatilM, RajputS. Succinimides: synthesis, reaction, and biological activity. Int J Pharm Pharm Sci. 2014;6(11):8–14.
- JanMS, ShahidM, AhmadS, et al. Synthesis of pyrrolidine-2, 5-dione based anti-inflammatory drug: in vitro COX-2, 5-LOX inhibition and in vivo anti-inflammatory studies. Latin Am J Pharm. 2019;38(11):2287–2294.
- SelvakumarV, DuraipandiS, DevdasS. Synthesis and psychopharmacological activities of some succinimide mannich bases. J Pharm Res. 2011;4:3168–3170.
- NugentTC, NegruDE, El‐ShazlyM, et al. Sequential reductive amination‐hydrogenolysis: a one‐pot synthesis of challenging chiral primary amines. Adv Synth Catal. 2011;353(11‐12):2085–2092.
- NugentTC, BibiA, SadiqA, Mohammad ShoaibM, UmarN, TehraniFN. Chiral picolylamines for Michael and aldol reactions: probing substrate boundaries. Org Biomol Chem. 2012;10(46):9287–9294.23104278
- IftikharF, YaqoobF, TabassumN, et al. Design, synthesis, in-vitro thymidine phosphorylase inhibition, in-vivo antiangiogenic and in-silico studies of C-6 substituted dihydropyrimidines. Bioorg Chem. 2018;80:99–111. doi:10.1016/j.bioorg.2018.05.02629894893
- TanoliST, RamzanM, HassanA, et al. Design, synthesis and bioevaluation of tricyclic fused ring system as dual binding site acetylcholinesterase inhibitors. Bioorg Chem. 2019;83:336–347. doi:10.1016/j.bioorg.2018.10.03530399465
- NugentTC, SadiqA, BibiA, et al. Noncovalent bifunctional organocatalysts: powerful tools for contiguous quaternary‐tertiary stereogenic carbon formation, scope, and origin of enantioselectivity. Chem a Eur J. 2012;18(13):4088–4098. doi:10.1002/chem.201103005
- UllahF, AyazM, SadiqA, et al. Phenolic, flavonoid contents, anticholinesterase and antioxidant evaluation of iris germanica var; florentina. Nat Prod Res. 2016;30(12):1440–1444. doi:10.1080/14786419.2015.105758526166432
- AhmadS, IftikharF, UllahF, SadiqA, RashidU. Rational design and synthesis of dihydropyrimidine based dual binding site acetylcholinesterase inhibitors. Bioorg Chem. 2016;69:91–101. doi:10.1016/j.bioorg.2016.10.00227750058
- ShahS, ShahSMM, AhmadZ, et al. Phytochemicals, in vitro antioxidant, total phenolic contents and phytotoxic activity of cornus macrophylla wall bark collected from the North-West of Pakistan. Pak J Pharm Sci. 2015;28(1):23–28.25553682
- JabeenM, AhmadS, ShahidK, et al. Ursolic acid hydrazide based organometallic complexes: synthesis, characterization, antibacterial, antioxidant, and docking studies. Front Chem. 2018;6:55. doi:10.3389/fchem.2018.0005529594100
- ZebA, SadiqA, UllahF, AhmadS, AyazM. Investigations of anticholinesterase and antioxidant potentials of methanolic extract, subsequent fractions, crude saponins and flavonoids isolated from Isodon rugosus. Biol Res. 2014;47(1):76. doi:10.1186/0717-6287-47-7625723481
- ZafarR, UllahH, ZahoorM, et al. Isolation of bioactive compounds from Bergenia ciliata (haw.) Sternb rhizome and their antioxidant and anticholinesterase activities. BMC Complement Altern Med. 2019;19(1):296. doi:10.1186/s12906-019-2679-131694704
- KumkraiP, WeeranantanapanO, ChudapongseN. Antioxidant, alpha-glucosidase inhibitory activity and sub-chronic toxicity of Derris reticulata extract: its antidiabetic potential. BMC Complement Altern Med. 2015;15:35. doi:10.1186/s12906-015-0552-425887793
- FarooqU, NazS, ShamsA, et al. Isolation of dihydrobenzofuran derivatives from ethnomedicinal species polygonum barbatum as anticancer compounds. Biol Res. 2019;52(1):1. doi:10.1186/s40659-018-0209-030612577
- JabeenM, ChoudhryMI, MianaGA, et al. Synthesis, pharmacological evaluation and docking studies of progesterone and testosterone derivatives as anticancer agents. Steroids. 2018;136:22–31. doi:10.1016/j.steroids.2018.05.00829772243
- SramekJJ, FrackiewiczEJ, CutlerNR. Review of the acetylcholinesterase inhibitor galanthamine. Expert Opin Investig Drugs. 2000;9(10):2393–2402. doi:10.1517/13543784.9.10.2393
- CarreiroEP, LouroP, AdrianoG, et al. 3-hydroxypyrrolidine and (3, 4)-dihydroxypyrrolidine derivatives: inhibition of rat intestinal α-glucosidase. Bioorg Chem. 2014;54:81–88. doi:10.1016/j.bioorg.2014.04.00724859324
- RosenWG, MohsRC, DavisKL. A new rating scale for Alzheimer’s disease. Am J Psychiatry. 1984.
- ButterfieldDA, HensleyK, HarrisM, MattsonM, CarneyJ. β-amyloid peptide free radical fragments initiate synaptosomal lipoperoxidation in a sequence-specific fashion: implications to Alzheimer′ s disease. Biochem Biophys Res Commun. 1994;200(2):710–715. doi:10.1006/bbrc.1994.15088179604
- WolffS. Diabetes mellitus and free radicals: free radicals, transition metals and oxidative stress in the aetiology of diabetes mellitus and complications. Br Med Bull. 1993;49(3):642–652. doi:10.1093/oxfordjournals.bmb.a0726378221029
- AdeghateE, SchattnerP, DunnE. An update on the etiology and epidemiology of diabetes mellitus. Ann N Y Acad Sci. 2006;1084(1):1–29. doi:10.1196/annals.1372.02917151290
- ShimabukuroM, HigaN, ChinenI, YamakawaK, TakasuN. Effects of a single administration of acarbose on postprandial glucose excursion and endothelial dysfunction in type 2 diabetic patients: a randomized crossover study. J Clin Endocrinol Metab. 2006;91(3):837–842. doi:10.1210/jc.2005-156616368744