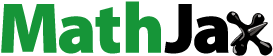
Abstract
Background
Recent studies have shown that the growth and proliferation of cancer cells in vivo exhibit circadian rhythm, and the efficacy and adverse reactions of platinum-based anticancer drugs administered at different times of the day vary significantly on colon cancer. However, since the circadian rhythms of growth and proliferation of various cancer cells often differ, the question of whether the administration of platinum anticancer drugs at different times of the day exerts significantly different efficacy and adverse effects on oral cancers remains to be elucidated. This study has compared the efficacy and adverse effects of oxaliplatin (L-OHP) administration at different times during a day on oral squamous cell carcinoma in mice and has analyzed cellular circadian rhythms.
Methods
The mouse model for oral squamous cell carcinoma was established in 75 nude mice, housed in a 12 hour light/12 hour dark cycle environment. The mice were randomly divided into five groups; four experimental groups were intravenously injected with L-OHP at four time points within a 24-hour period (4, 10, 16, and 22 hours after lights on [HALO]). The control group was intravenously injected with the same volume of saline. Treatment efficacy and adverse reactions were compared on the seventh day after the injection, at 22 HALO. The existence of circadian rhythms was determined by cosine analysis.
Results
Only injections of L-OHP at 16 and 22 HALO significantly prolonged animal survival time. The adverse reactions in mice injected with L-OHP at 16 and 22 HALO were significantly less than those observed in mice administered L-OHP at 4 and 10 HALO. The cosine fitting curve showed that the survival time and adverse reactions exhibited circadian rhythm.
Conclusion
The time factor should be considered when treating patients with oral squamous cell carcinoma with L-OHP in order to achieve better efficacy, reduce the adverse reactions, and improve the patients’ survival time and quality of life.
Introduction
Oral cancers account for 2% of cancer incidences in the population, and 90% of those cases are squamous cell carcinoma.Citation1 Although there has been much progress in the treatment of oral cancers with surgery, chemotherapy, and radiotherapy, the 5-year overall survival rate of oral cancer patients remains only 50%–60%.Citation1,Citation2 Therefore, to explore novel and effective treatment of oral cancer is of great significance.
Recent studiesCitation3–Citation6 have shown that the growth, proliferation, DNA synthesis, and secretion activities of both normal and tumor cells in mammals display a characteristic 24-hour circadian rhythm. The rhythmic changes in proliferation and DNA synthesis of tumor cells showed an 8–12 hour difference compared to those of normal cells such as bone marrow, gastrointestinal mucosal epithelium, etc. Because proliferating cells with active DNA synthesis are more sensitive to chemotherapeutic drugs, a theoretical rationale is provided for the administration of chemotherapeutic drugs at certain times of day, in order to maximize the killing of cancer cells and minimize the damage to normal cells. Chronochemotherapy is administered by selecting the appropriate medication time based on the differences in circadian rhythm of proliferation and DNA synthesis activities between tumor cells and normal cells in order to obtain the maximum anticancer efficacy, minimize adverse reactions, and improve the patients’ survival rate and quality of life.Citation5,Citation6
Recent animal experiments and multiple center studies of chronochemotherapy in treating colon cancer, breast cancer, and lung cancer have demonstrated that platinum drugs (cisplatin, carboplatin, and oxaliplatin), 5-fluorouracil, leucovorin, and doxorubicin showed significant differences in anticancer efficacy and adverse reactions when administered at different times during a day.Citation4–Citation10 The rhythmic changes in growth and proliferation of different tumors often vary.Citation11 There are no reports regarding the chronochemotherapy against oral cancers; however, our previous studiesCitation12 have shown that the growth and cell proliferation of oral squamous cell carcinoma, in vivo, also exhibited the characteristics of circadian rhythm, which implied that there would be great differences in anticancer drug efficacy and adverse reactions with oral squamous cell carcinoma patients when drugs are administered at different times of the day. The results of recent studies are often derived from combinatorial chemotherapy using several anticancer drugs and thus lack the opportunity to observe the efficacy of chronochemotherapy with a single drug. In addition, the previous studies often compared the difference in drug efficacy and adverse reactions for drugs administered at different times during a day, but did not validate and analyze whether there was the characteristic of circadian rhythm.Citation4–Citation10 Currently, cosine analysis, developed using cosine function modeling, has become an effective method for the analysis of biological rhythmic data. It can exclude the interference of other biological factors, and hence can isolate and visually present the characteristics of the biological rhythm, and show apparent characteristics of circadian rhythm by a cosine fitted curve.Citation13
Chemotherapy is an important part of the comprehensive treatment of oral squamous cell carcinoma,Citation1,Citation2 and platinum-based anticancer drugs are one of the most common and effective drugs for treating almost all solid tumors including oral cancers.Citation9,Citation10,Citation14 Recent studies have shown that the efficacy of platinum-based anticancer drugs on colon, breast, and lung cancers, as well as the patients’ adverse reactions, vary significantly among drugs administered at different times during a day. Citation4–Citation10 To explore the effect of chronochemotherapy with platinum anticancer drugs on oral squamous cell carcinoma and its circadian rhythm, this study established an oral squamous cell carcinoma model in nude mice by injecting human oral squamous cell carcinoma Tca8113 cells into mouse cheek. The third generation platinum anticancer drug oxaliplatin (L-OHP) was administered at four different time points during a day, and the respective effects of L-OHP on tumor inhibition rate, survival time, and adverse reactions of the tumor-bearing mice were observed and compared. In order to provide evidence for a safer and more effective application of medications in the clinical treatment of oral cancer, cosine analysis was performed to test for the presence of circadian rhythmicity in the efficacy of drugs administered at different times and in the adverse reactions of corresponding animals.
Materials and methods
Reagents and instruments
Oral squamous cell carcinoma cell line Tca8113 was obtained from the College of Life Sciences, Chongqing Medical University (Chongqing, People’s Republic of China). Oxaliplatin and Roswell Park Memorial Institute (RPMI) 1640 medium were purchased from Sigma-Aldrich (St Louis, MO, USA). Fetal bovine serum was a product of Life Technologies (Carlsbad, CA, USA), and the microliter centrifuge was a product of HERMLE Labortechnik (Model Z233MK; Wehingen, Germany). An optical microscope used for the analysis of tumor tissue sections was from Nikon Corporation (Model CYMML-EPXFU-068; Tokyo, Japan).
Experimental animals
A total of 75 specific pathogen free, BALB/c nude mice (females, 5–6-weeks-old, 19–20 g) were purchased from the Experimental Animal Center of Chongqing Medical University. All experimental procedures were approved by the Experimental Animal Use Management Committee, Institute of Experimental Animals, Chongqing Medical University.
Establishment of the Tca8113 oral squamous cell carcinoma model in nude mice
All of the mice (five per cage) were placed in a standardized 12 hour light/12 hour dark environment, maintained at room temperature (24°C ± 1°C), with an air humidity level of 60% ± 10%. The bedding, feed and water were all sterilized. The hours after lights on (HALO) was used as a reference: 0 HALO denotes the time the lights go on, and 12 HALO is when lights go off; the time before 12 HALO is the rest phase, and after 12 HALO is the activity phase. All mice were synchronized in the same environment for 3 weeks before the experiment.
Tca8113 cells were cultured with RPMI 1640 medium containing 10% fetal bovine serum in a 37°C incubator with 5% CO2. Tca8113 cells in logarithmic growth phase were collected by 0.25% trypsin digestion and centrifuged at 4°C, 1000 rpm (centrifugal radius, 3 cm), for 5 minutes. Cells were then resuspended with sterile phosphate buffered saline into a single cell suspension. A total of 0.2 mL of Tca8113 cell suspension containing 2 × 106 cells was injected into the cheek of nude mice, which were then placed in the same standardized 12 hour light/12 hour dark environment in separate cages. The growth of implanted tumors was observed daily. Three weeks later, evident tumors were observed and the experiments were started.
Drug administration and timing
According to the random number table, the 75 tumor-bearing nude mice were divided into five groups (four experimental groups and one control group, with 15 animals in each group). L-OHP powder was dissolved with 0.9% saline to a concentration of 3.4 mg/mL. Mice from each of the four experimental groups were injected via the tail vein, with L-OHP solution (17 mg/kg) within a 24-hour period at 4, 10, 16, and 22 HALO. The control group was injected with the same volume of 0.9% saline.
Efficacy evaluation
At 22 HALO on the seventh day after drug administration, the maximum and minimum diameters of the tumors in the five groups of animals were measured with a vernier caliper. The tumor volume (V) was calculated as follows,
where a and b are the maximum and minimum of the diameters of the tumors, respectively. The following equation was used to determine tumor inhibition rate,
The survival time was determined as the number of days that each tumor-bearing mouse survived after the drug administration.
Adverse reactions
The adverse reactions were assessed using the following indexes: peripheral white blood cell (WBC) count, body weight of tumor-bearing mice, and rate of perianal swelling. Briefly, at 22 HALO on the seventh day after drug administration, mouse body weight was measured with an electronic weighing scale. The perianal swelling of nude mice was observedCitation15,Citation16 and 20 μL of venous blood was drawn from the tail vein of mice by puncture with a clean, dry trace hemoglobin pipette, and peripheral WBCs were counted manually using a hemocytometer.
Statistical analysis
The data were analyzed using SigmaPlot 12.0 software (Systat Software, Inc, San Jose, CA, USA). The comparison between multiple means was performed with analysis of variance, while the comparison between rates was carried out using χ2 test. Cosine analysis was done using CircWave V1.4 software (Groningen, The Netherlands) to test whether there was circadian rhythm with each indicator. Survival times among groups were tested by Kaplan-Meier survival curves. The results were presented as mean ± standard deviation, and differences with P < 0.05 were considered statistically significant.
Results
Histological examination of Tca8113 tumors formed in nude mice
Tumor formation was evident in the cheeks of all 75 nude mice 3 weeks after the implantation of Tca8113 cells. A large amount of tumor cells were visible under the light microscope in sections of tumor tissues stained with hematoxylin and eosin. Tumor cells were poorly differentiated and arranged tightly, and the formation of tumor nests was also observed ().
Comparison of tumor inhibition rate among groups of tumor-bearing mice
The tumor inhibition rate of each group is shown in , which exhibits significant differences among groups administered L-OHP at various time points of the day (χ2 = 50.49, P = 0.00). Injection of L-OHP at 10 HALO showed the minimum inhibitory rate, which was significantly lower than those of 4, 16, and 22 HALO groups; however, the tumor inhibition rates were not significantly different among the 4, 16, and 22 HALO groups (P > 0.05).
Table 1 Drug efficacy and adverse reactions in control and experimental tumor-bearing mice 7 days after L-OHP injection
Comparison of survival time among groups of tumor-bearing mice
The mean survival time of tumor-bearing mice in each group is shown in , which indicates that there was significant difference in the mean survival time among groups of tumor-bearing mice (F = 15.02, P < 0.001). However, the animal survival times in the 4 and 10 HALO groups showed no significant difference compared with that of the control group (P > 0.05), indicating that injection of L-OHP at 4 and 10 HALO did not significantly prolong animal survival time. In contrast, the animal survival times in the 16 and 22 HALO groups were significantly different from that in the control group (P < 0.05), suggesting that injection of L-OHP at 16 and 22 HALO significantly extended the survival time of tumor-bearing mice, while the animal survival times between the 16 and 22 HALO groups showed no significant difference (P > 0.05). These data clearly suggest that injection of L-OHP during the activity phase can significantly extend the survival time of tumor-bearing mice.
Cosine analysis also indicates that there is circadian rhythmicity in the mean survival time of tumor-bearing mice injected with L-OHP at various times (F = 12.47, P < 0.001). As shown in , the cosine fitting curve suggests that tumor-bearing mice injected with L-OHP at 6.98 HALO have the shortest survival time (11.03 days), while tumor-bearing mice injected with L-OHP at 18.97 HALO display the longest survival time (20.41 days).
Figure 2 Cosine fitting curve of the survival times of tumor-bearing mice injected with L-OHP at four different time points.
Abbreviations: L-OHP, oxaliplatin; HALO, hours after light onset.

Kaplan–Meier survival curve analysis indicates that tumor-bearing nude mice injected with L-OHP at 10 HALO had the shortest survival time, while the longest survival time was observed in animals injected with L-OHP at 16 HALO (log-rank χ2 = 27.36, P < 0.001).
Comparison of the adverse reactions among groups of tumor-bearing mice
The data collected from WBC count, body weight, and perianal swelling are shown in . The WBC counts were significantly different among the five groups of tumor-bearing mice (F = 292.21, P < 0.001), and the WBC counts in the four experimental groups were significantly lower than that in the control group (P < 0.001). The lowest WBC count was measured in animals injected with L-OHP at 10 HALO, while the WBC count least reduced from control levels was observed in mice injected L-OHP at 16 HALO.
Cosine analysis shows that circadian rhythm was involved with the reduction in WBC count among animals injected with L-OHP at different times (F = 100.25, P = 0.00). Cosine curve fitting () also indicates that mice injected with L-OHP at 18.52 HALO show the least reduction in WBC count from the control levels (15.43 × 103 cells/μL), while mice injected with L-OHP at 6.46 HALO suffer the most severe reduction in WBC count (14.19 × 103 cells/μL).
Figure 3 Cosine fitting curve of the WBC counts of tumor-bearing mice injected with L-OHP at four different time points.
Abbreviations: L-OHP, oxaliplatin; HALO, hours after light onset; WBC, white blood cell.

The body weight of tumor-bearing mice showed significant differences among the five groups (F = 51.87, P < 0.001). The body weight of mice in the 10 HALO group was similar to that in the control group (P > 0.05), whereas the body weight of animals in the 4, 16, and 22 HALO groups was significantly different from that of the control group (P < 0.05). However, there was no significant difference in body weight between the 4 and 22 HALO groups (P > 0.05), whereas the body weight of animals in the 16 HALO group was significantly different from that in the 4 and 22 HALO groups (P < 0.05). Cosine analysis indicates that there is circadian rhythmicity in body weight of mice injected with L-OHP at different time points (F = 24.61, P = 0.00), and cosine curve fitting () shows that mice injected with L-OHP at 17.75 HALO have the highest body weight (17.81 g), whereas animals injected with L-OHP at 5.81 HALO have the lowest body weight (17.32 g).
Figure 4 Cosine fitting curve of the body weights of tumor-bearing mice injected with L-OHP at four different time points.
Abbreviations: L-OHP, oxaliplatin; HALO, hours after light onset.

The rate of perianal swelling was significantly different among the five groups of tumor-bearing mice (χ2 = 17.11, P < 0.01), the highest of which was seen in mice injected with L-OHP at 10 HALO (60.00%, 9/15), and the lowest rate was in the 16 HALO group (13.33%, 2/15). The rate of perianal swelling in the 16 HALO group was significantly different from that of the 4 and 10 HALO groups (P < 0.05) but not from that of the 22 HALO group (P > 0.05), indicating that injection of L-OHP during the activity phase significantly reduces the perianal swelling rate in tumor-bearing mice compared with drug injections administered during the rest phase.
Discussion
L-OHP is a third generation platinum anticancer drug, containing a ring of 1,2-diaminocyclohexane. After activation, the ring and platinum in L-OHP bind to DNA in cancer cells and form a platinum-DNA complex as well as intra- and interstrand crosslinks, thereby blocking DNA replication and transcription, resulting in noncell cycle-specific death.Citation17 Currently, L-OHP is often applied in combination with other anticancer drugs. In this study, however, L-OHP treatment alone was used, in order to better discern the circadian characteristics of L-OHP in treating oral squamous cell carcinoma. We found that the tumor inhibition rates at 7 days after the injection of L-OHP at four different time points (4, 10, 16, and 22 HALO), within a 24-hour period were 23.97%, 7.05%, 31.55%, and 28.33%, respectively, while the corresponding survival times were 14.07 ± 4.02, 10.73 ± 3.51, 20.73 ± 5.41, and 17.33 ± 6.11 days, respectively. The tumor inhibition rate and survival time in the 10 HALO group was significantly lower than that in other treatment groups. Although the 4 HALO group showed a similar tumor inhibition rate compared with the 16 and 22 HALO groups, only injections with L-OHP at 16 and 22 HALO significantly prolonged the survival time of tumor-bearing mice. Also, cosine analysis proved a role for circadian rhythmicity in average survival time of mice injected with L-OHP at different time points, ie, tumor-bearing mice injected with L-OHP at 6.98 HALO (middle rest phase) had the shortest survival time (11.03 days), while animals injected with L-OHP at 18.97 HALO (middle activity phase) showed the longest survival time (20.41 days).
Because myelosuppression and gastrointestinal mucosal epithelial damage are major adverse reactions to L-OHP,Citation17 the present study used indexes such as body weight (reflecting physical condition), WBC count (reflecting bone marrow suppression), and perianal swelling (reflecting gastrointestinal toxicity) to evaluate adverse reactions of experimental animals.Citation16,Citation17 All three indexes showed significant differences among animals injected with L-OHP at four different time points (4, 10, 16, and 22 HALO). The lowest body weight was observed in mice injected with L-OHP at 10 HALO, whereas mice injected with L-OHP at 16 HALO showed the highest body weight, suggesting the minimal detrimental impact of L-OHP injection at 16 HALO on the general physical condition of mice. Cosine analysis indicates the circadian rhythmicity in body weight of mice injected with L-OHP at different time points, ie, injection of L-OHP at 17.75 HALO (middle activity phase) exerts minimal impact on the body weight of tumor-bearing animals, whereas injection of L-OHP at 5.81 HALO (middle rest phase) shows the greatest impact on the body weight of tumor-bearing mice. The results of WBC count also indicated that the most reduction in WBC count was in animals injected with L-OHP at 10 HALO, while the 16 HALO group showed the least reduction in WBC count as compared to controls, implying that injection of L-OHP at 16 HALO exerts the least suppression on mouse bone marrow. Cosine analysis also indicates the existence of circadian rhythm in WBC counts of mice injected with L-OHP at different time points, ie, L-OHP injection at 18.52 HALO (mid activity phase) resulted in the least reduction in WBC count (ie, least bone marrow suppression), whereas injection of L-OHP at 6.46 HALO (middle rest phase) led to the most reduction in WBC count (ie, most severe bone marrow suppression). The results of perianal swelling showed that injection of L-OHP at 10 HALO (late rest phase) led to the highest incidence rate of perianal swelling (60.00%), indicating the greatest toxic effect on the gastrointestinal tract, while L-OHP injection at 16 HALO (transition from early to middle activity phase) resulted in the lowest rate of perianal swelling (13.33%) (ie, the lowest gastrointestinal toxicity).
The mechanism of the circadian effects of L-OHP is closely related to the circadian variations of cancer cell proliferation, cell cycle distribution, DNA synthesis, drug-metabolizing enzyme activity, blood flow in tumor tissue, and other factors.Citation3–Citation6 Since L-OHP can easily form crosslinks with the DNA of cancer cells, injection of L-OHP in the phase of high cancer cell proliferation and high DNA synthesis can elicit greater anti-cancer effects.Citation6,Citation17 However, alterations in circadian rhythms of cancer cell proliferation and DNA synthesis seem to depend upon tumor type, growth rate, and level of differentiation.Citation6,Citation11 Blumenthal et alCitation18 reported that blood flow in tumors and normal organs of tumor-bearing mice had circadian rhythms. Blood flow in mouse tumors was significantly higher during 15–21 HALO than during 3–9 HALO, and tumor blood flow reached peak value at 17 HALO, whereas the circadian rhythm of blood flow in normal organs (such as liver, lung, and kidney) was opposite to that in tumors. Since the circadian rhythm variations of blood flow in tumors and normal organs can affect the local concentration of drug, it then can affect drug efficacy and adverse reactions of a drug given at different times in a day.Citation18,Citation19 Reduced glutathione, which can reverse cytotoxic effects of platinum drugs, also has circadian rhythm.Citation20–Citation22 This may be one of the reasons for different drug efficacy and adverse reactions of L-OHP injected at different times in a day. Therefore, the different drug efficacy and adverse reaction results observed in this study are consistent with the overall conclusions resulting from the combination of the aforementioned factors. The details of the specific mechanism remains the focus of further in-depth study.
Currently, a growing number of researchers recognize that a reasonable time regimen for the administration of anticancer drugs plays an equally important role as does the drug dosage.Citation23 This study has shown that injection of L-OHP at different time points during a day resulted in significantly different efficacy and adverse reactions in treating oral squamous cell carcinoma in mice. Such differences fluctuated with a 24 hour circadian rhythm, and injections of L-OHP at 16 and 22 HALO significantly prolonged the survival time in mice with oral squamous cell carcinoma. In general, injection of L-OHP in the early and middle activity phases achieved the maximum efficacy and minimum adverse reactions, whereas injection of L-OHP in the early and middle rest phases led to the worst efficacy and maximum adverse reactions. Although the rodents are nocturnal animals, and their phases of rest and activity are contrary to those of human beings, recent studies have shownCitation5,Citation6,Citation24 that the experimental results obtained from the rest and activity phases of rodents can be directly extended to the corresponding rest and activity phases in humans. Therefore, circadian time factors should be considered when applying chemotherapy in patients with oral squamous cell carcinoma, in order to achieve better efficacy, reduce toxic effect, and improve patients’ survival time and quality of life.
Disclosure
The authors report no conflicts of interest in this work.
References
- Kademani D Bell RB Schmidt BL Oral and maxillofacial surgeons treating oral cancer: a preliminary report from the American Association of Oral and Maxillofacial Surgeons Task Force on Oral Cancer J Oral Maxillofac Surg 2008 66 10 2151 2157 18848117
- Kademani D Bell RB Bagheri S Prognostic factors in intraoral squamous cell carcinoma: the influence of histologic grade J Oral Maxillofac Surg 2005 63 11 1599 1605 16243176
- Eismann EA Lush E Sephton SE Circadian effects in cancer-relevant psychoneuroendocrine and immune pathways Psychoneuroendocrinology 2010 35 7 963 976 20097011
- Huang XL Fu CJ Bu RF Role of circadian clocks in the development and therapeutics of cancer J Int Med Res 2011 39 6 2061 2066 22289520
- Lévi F Okyar A Dulong S Innominato PF Clairambault J Circadian timing in cancer treatments Annu Rev Pharmacol Toxicol 2010 50 377 421 20055686
- Lévi F Chronotherapeutics: the relevance of timing in cancer therapy Cancer Causes Control 2006 17 4 611 621 16596317
- Levi F Zidani R Misset JL Randomised multicentre trial of chronotherapy with oxaliplatin, fluorouracil, and folinic acid in metastatic colorectal cancer. International Organization for Cancer Chronotherapy Lancet 1997 350 9079 681 686 9291901
- Lemmer B Chronobiology, drug-delivery, and chronotherapeutics Adv Drug Deliv Rev 2007 59 9–10 825 827 17825947
- Santini D Vincenzi B Schiavon G Chronomodulated administration of oxaliplatin plus capecitabine (XELOX) as first line chemotherapy in advanced colorectal cancer patients: phase II study Cancer Chemother Pharmacol 2007 59 5 613 620 16944151
- Garufi C Brienza S Pugliese P Overcoming resistance to chronomodulated 5-fluorouracil and folinic acid by the addition of chronomodulated oxaliplatin in advanced colorectal cancer patients Anticancer Drugs 2000 11 6 495 501 11001391
- Davidson AJ Straume M Block GD Menaker M Daily timed meals dissociate circadian rhythms in hepatoma and healthy host liver Int J Cancer 2006 118 7 1623 1627 16231323
- Zhao N Tang H Yang K Chen D Circadian rhythm characteristics of oral squamous cell carcinoma growth in an orthotopic xenograft model Onco Targets Ther 2013 6 41 46 23378773
- Manoharan S Baskar AA Manivasagam T Subramanian P Circadian rhythmicity of plasma lipid peroxidation and antioxidants in oral squamous cell carcinoma Singapore Med J 2005 46 4 184 188 15800725
- Kelland L The resurgence of platinum-based cancer chemotherapy Nat Rev Cancer 2007 7 8 573 584 17625587
- Singletary J Wood PA Du-Quiton J Imaging multidimensional therapeutically relevant circadian relationships Int J Biomed Imaging 2009 2009 231539 19688113
- Wood PA Du-Quiton J You S Hrushesky WJ Circadian clock coordinates cancer cell cycle progression, thymidylate synthase, and 5-fluorouracil therapeutic index Mol Cancer Ther 2006 5 8 2023 2033 16928823
- Kelland L Broadening the clinical use of platinum drug-based chemotherapy with new analogues. Satraplatin and picoplatin Expert Opin Investig Drugs 2007 16 7 1009 1021
- Blumenthal RD Osorio L Ochakovskaya R Ying Z Goldenberg DM Regulation of tumour drug delivery by blood flow chronobiology Eur J Cancer 2000 36 14 1876 1884 10974637
- Hori K Zhang QH Li HC Saito S Sato Y Timing of cancer chemotherapy based on circadian variations in tumor tissue blood flow Int J Cancer 1996 65 3 360 364 8575858
- Lévi F Focan C Karaboué A Implications of circadian clocks for the rhythmic delivery of cancer therapeutics Adv Drug Deliv Rev 2007 59 9–10 1015 1035 17692427
- Zeng ZL Sun J Guo L Circadian rhythm in dihydropyrimidine dehydrogenase activity and reduced glutathione content in peripheral blood of nasopharyngeal carcinoma patients Chronobiol Int 2005 22 4 741 754 16147904
- Granda TG Lévi F Tumor-based rhythms of anticancer efficacy in experimental models Chronobiol Int 2002 19 1 21 24 11962677
- Vincenzi B Santini D La Cesa A Tonini G Cancer chronotherapy: principles, applications, and perspectives Cancer 2003 98 4 881 883 12910534
- Innominato PF Lévi FA Bjarnason GA Chronotherapy and the molecular clock: clinical implications in oncology Adv Drug Deliv Rev 2010 62 9–10 979 1001 20600409