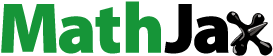
Abstract
Background
Small metal nanoparticles obtained from animal blood were observed to be toxic to cultured cancer cells, whereas noncancerous cells were much less affected. In this work, engineered zinc and copper metal nanoparticles were produced from bulk metal rods by an underwater high-voltage discharge method. The metal nanoparticles were characterized by atomic force microscopy and X-ray photoelectron spectroscopy. The metal nanoparticles, with estimated diameters of 1 nm–2 nm, were determined to be more than 85% nonoxidized. A cell viability assay and high-resolution light microscopy showed that exposure of RG2, cultured rat brain glioma cancer cells, to the zinc and copper nanoparticles resulted in cell morphological changes, including decreased cell adherence, shrinking/rounding, nuclear condensation, and budding from cell bodies. The metal-induced cell injuries were similar to the effects of staurosporine, an active apoptotic reagent. The viability experiments conducted for zinc and copper yielded values of dissociation constants of 0.22±0.08 nmol/L (standard error [SE]) and 0.12±0.02 nmol/L (SE), respectively. The noncancerous astrocytes were not affected at the same conditions. Because metal nanoparticles were lethal to the cancer cells at sub-nanomolar concentrations, they are potentially important as nanomedicine.
Purpose
Lethal concentrations of synthetic metal nanoparticles reported in the literature are a few orders of magnitude higher than the natural, blood-isolated metal nanoparticles; therefore, in this work, engineered metal nanoparticles were examined to mimic the properties of endogenous metal nanoparticles.
Materials and methods
RG2, rat brain glioma cells CTX TNA2 brain rat astrocytes, obtained from the American Type Culture Collection, high-voltage discharge, atomic force microscope, X-ray photoelectron spectroscopy, high-resolution light microscopy, zeta potential measurements, and 3-[4,5-dimethylthiazol-2-yl]-2,5-diphenyltetrazolium bromide (MTT) assay were used in this work.
Results
Engineered zinc and copper metal nanoparticles of size 1 nm–2 nm were lethal to cultured RG2 glioma cancer cells. Cell death was confirmed by MTT assay, showing that the relative viability of RG2 glioma cells is reduced in a dose-dependent manner at sub-nanomolar concentrations of the nanoparticles. The noncancerous astrocytes were not affected at the same conditions.
Conclusion
The engineered and characterized zinc and copper nanoparticles are potentially significant as biomedicine.
Introduction
Small concentrations of protein nucleating centers (PNCs) isolated from animal blood were observed to be toxic to cultured cancer cells. PNCs were composed of metal nanoparticles of size 1 nm–2 nm. After incubation with PNCs, the viability of two rat glioma cell lines (F98 and RG2) decreased by 90% and 75%, respectively, while that of rat astrocytes (CTX) was reduced by only 25%. Total suppression of growth in vitro required ≈1×1012 PNCs/mL (ie, a few nmol/L), a concentration smaller than what is typically found in a healthy animal ().Citation1
Figure 1 Effect of PNCs on cell viability.
Notes: (A) Viability of cultured cells after a 20-hour exposure to different concentrations of PNCs (obtained from shark blood). Row 1 (all expressed in PNCs/mL): 1.7×1011; row 2: 9.1×1010; row 3: 9.9×109; row 4: no PNCs. F98 and RG2 represent rat brain glioma cells and CTX represents rat astrocytes. (B–D) Light darkfield microscope images of rat glioma cells. (B, C) Cells before and after exposure to 7.7×1011 PNCs/mL, respectively. (D) Cells after exposure to 1.0 μM staurosporine. (E) Fluorescence photomicrograph of RG2 glioma cells exposed to 7.7×1011 PNCs/mL for 30 minutes and stained with annexin V and propidium iodide. (F) Viability of cultured RG2 after a 20-hour exposure to different concentrations of PNCs obtained from the blood of the blue shark (square), dog (circle), human (triangle), New Zealand white rabbit (inversed triangle). R2=0.95; P<0.0001. Scale bars: 40 μmol/L.Citation1 Samoylov AM, Samoylova TI, Pustovyy OM, et al. Novel metal clusters isolated from blood are lethal to cancer cells. Cells Tissues Organs. 2005;179(3):115–124. Copyright © 2005 Karger Publishers, Basel, Switzerland.
Abbreviation: PNCs, protein nucleating centers.

Synthetic copper nanoparticles have been found to be toxic to cultured cancer cells, including U937 (human histiocytic lymphoma) and HeLa cells (human cervical cancer origin) at concentrations of 1–500 μmol/L.Citation2–Citation4 This suggests cytotoxicity of other metal nanoparticles including zinc, since high concentrations of zinc ions (≈20 µmol/L) have previously shown cytotoxicity in a wide range of cultured cancer cells.Citation5–Citation11 Lethal concentrations of synthetic metal nanoparticles reported in the literature are a few orders of magnitude higher than the natural, blood-isolated metal nanoparticles;Citation1 therefore, in this work, engineered metal nanoparticles were examined to mimic properties of endogenous metal nanoparticles.
Materials and methods
Metal nanoparticle preparation
Engineered zinc and copper nanoparticles were prepared using high-voltage discharge method from bulk metal rods at alternating current (AC) 15,000 V for 1 hourCitation12,Citation13 (Supplementary materials).
Cell culture
RG2, F98 (rat brain glioma lines), and CTX TNA2 (brain, rat astrocytes transfected with SV40) were obtained from the American Type Culture Collection (ATCC) and maintained as recommended by ATCC.
Cell viability assay
3-[4,5-dimethylthiazol-2-yl]-2,5-diphenyl tetrazolium bromide (MTT) assayCitation14 was used to measure cell viability (Supplementary materials). Eight experiments with zinc nanoparticles and five experiments with copper nanoparticles and RG2 cells were performed. Sixteen control experiments with zinc and copper nanoparticles and CTX astrocytes were performed. Experiments tested cells in six conditions: untreated cells, metal nanoparticles at four different sub-nanomolar concentrations, or 1 μmol/L of staurosporine added to cells. Each condition was repeated six times. Results were presented as mean values for each condition ± standard error (SE). The concentration of metal nanoparticles is much lower than that found in the animal blood.Citation1
Atomic force microscopic images
Images of metal nanoparticles were taken by Bruker Multi-Mode 8 (Santa Barbara, CA, USA) atomic force microscope in tapping (intermittent-contact) mode. Monolayers of zinc and copper nanoparticles were prepared on a mica substrate. Size distributions of nanoparticles were measured in five independent scans over different areas (Supplementary materials).
X-ray photoelectron spectroscopy
The Kratos Axis Ultra delay-line detector instrument in hybrid mode using a monochromatic Al Kα1,2 X-ray source (hυ=1,486.6 eV) was used for X-ray photoelectron spectroscopy (XPS). Zinc and copper nanoparticles were analyzed in a water suspension on a silicon wafer (Supplementary materials).
Light microscopy
Darkfield light microscopic images were produced by a high-resolution microscope systemCitation15 (Supplementary materials).
Zeta potential
Zeta potentials of the nanoparticles in water suspension were determined with a Zetasizer Nano ZSP (Malvern Instruments, Worcestershire, UK) using the laser Doppler velocimetry technique. The results of six sequential runs were averaged (Supplementary materials).
Results
shows the physical properties of Zn and Cu nano-particles prepared by high-voltage discharge. Atomic force microscopy revealed the size distributions of Zn and Cu nanoparticles, with average diameters of 1.2±0.3 nm and 2.1±0.6 nm, respectively (). Histograms () represent distributions with relatively high peaks around average values (their standard deviations [SDs] were calculated from the bell distributions around the peaks) and tails up to 6 nm. The nanoparticles were homogenously distributed with polydispersity indexes of 0.062 and 0.082 for zinc and copper, respectively.
Figure 2 Zinc and copper metal nanoparticles.
Notes: Atomic force microscope images (A and B), size distributions (C and D), and XPS spectra (E and F) of zinc and copper nanoparticles, respectively. (A) 0.01% zinc nanoparticles on mica. A total of 270 nanoparticles with a height of above 1 nm were detected over a 2.5 μm2 area. (B) A total of 0.01% copper nanoparticles on mica; 257 nanoparticles with a height of above 0.75 nm were detected over a 2.5 μm2 area. (E and F) High-resolution XPS of Zn 2p3/2 and Cu 2p3/2 lines, showing the relative concentration of oxidized metal species.
Abbreviation: XPS, X-ray photoelectron spectroscopy.

shows XPS high-resolution 2p3/2 spectra for metal and metal oxide species: 93.9%±3.4% and 5.9%±3.7% (Zn and ZnO) and 85.8%±4.1% and 14.2%±4.1% (Cu and CuO). These data indicate that more than 85% of metal atoms were not oxidized.
Zeta potentials for zinc and copper nanoparticles in water suspension were determined as ζZn=−15.4±0.8 (SD) mV and ζCu=−17.4±1.7 (SD) mV.
Following incubation with zinc nanoparticles for 20 hours, cells displayed morphological features consistent with cell death, including a decrease in adherence, shrinking/rounding, nuclear condensation, and budding from cell bodies (). Zn-induced cell injury was similar to the effects of 1 μmol/L staurosporine, an effective apoptotic reagent.Citation16
Figure 3 Light darkfield images of RG2 glioma cells before (A) and after (B) exposure to 5 nmol/L of zinc nanoparticles (Bar, 10 μm).

Cell death was confirmed by MTT assay, showing that the relative viability of RG2 glioma cells is reduced in a dose-dependent manner when added zinc nanoparticle concentrations increased from 0.05 nmol/L to 0.3 nmol/L, reducing the cell viability by ≈60% (). This reduction in viability compares well to that of 1 μmol/L staurosporine (; treatment 5). Assuming that cell death is proportional to the number of bound metal nanoparticles, the ratio of relative number of dead (Y) and alive (1−Y) cells can be presented by the Hill presentation of binding,
Figure 4 Viability and mortality of glioma cancer cells treated with metal nanoparticles (error bars are standard deviations).
Notes: (A) Viability of glioma cells at six experimental conditions: 0, no treatment; 1–4, zinc nanoparticles (all expressed in nmol/L) 0.053, 0.106, 0.212, 0.318, respectively; 5, 1 μmol/L of staurosporine. (B) Hill presentation of glioma cell mortality caused by zinc nanoparticles. (C) Viability of glioma cells at six experimental conditions: 0, no treatment; 1–4, copper nanoparticles (all expressed in nmol/L) 0.017, 0.033, 0.066, 0.1, respectively; 5, 1 μmol/L of staurosporine. (D) Hill presentation of glioma cell mortality caused by copper nanoparticles. (E) Viability of astrocytes at six experimental conditions: 0, no treatment; 1–4, zinc nanoparticles (all expressed in nmol/L) 0.053, 0.106, 0.212, 0.318, respectively; 5, 1 μmol/L of staurosporine. (F) Viability of astrocytes at six experimental conditions: 0, no treatment; 1–4, copper nanoparticles (all expressed in nmol/L) 0.017, 0.033, 0.066, 0.1, respectively; 5, 1 µmol/L of staurosporine.


Discussion
Small, engineered metal nanoparticles of zinc and copper examined in this work were lethal to RG2 cultured cancer cells at sub-nanomolar concentrations. The results obtained are consistent with those with the natural metal nanoparticles isolated from animal blood.Citation1 Copper nanoparticles of 4–5 nm in size caused degradation of DNA molecules in U937 and HeLa cells at concentrations of 100 µmol/L–500 µmol/L. The apparent dissociation constant for copper nanoparticles, 32 μmol/L,Citation3 was approximately 25,000-fold larger than that found in the present work.Citation3 Prior studies revealed that bigger CuO nanoparticles (≈20 nm) ranging from 1 to 27 μmol/L caused HeLa cell degradationCitation4; however, synthetic copper nanoparticles (1 nm–2 nm) encapsulated in water soluble polymers also reduced HeLa cell viability by 70% at ≈1 μmol/L.Citation2 Zinc and copper nanoparticles examined in our work proved to be lethal to cancer cells at concentrations of a few orders of magnitude smaller than those found in the literature, while noncancerous cells were not affected.
The only nanoparticle that presented sub-nanomolar efficiency against cancer is iron oxide with a low Kd value of 0.2 nmol/M.Citation17
We speculate that small, uncharged nanoparticles of size 1 nm–2 nm can efficiently penetrate through the external and nuclear membranes and create aggregates with some protein or nucleic acids. Aggregates inside RG2 glioma cells increased up to ≈100 nm when the glioma cells were exposed to natural metal nanoparticles.Citation1 Large aggregates are recognized as foreign particles and expelled by exocytosis.Citation18 Perhaps when many aggregate particles leave the cell, the external cell membrane depletes causing nucleus budding and cell death. The morphological changes observed in cells exposed to metal nanoparticles are similar to those of the cells treated with staurosporine, a known apoptotic agent.Citation16 The apoptotic death of cancer cells caused by copper nanoparticles was also reported.Citation2,Citation3 It was found that surface charge is a major determinant of how metal nanoparticles impact cellular processes. The charged nanoparticles induce cell death through apoptosis and neutral nanoparticles lead to necrosis.Citation19 Zeta potentials of zinc and copper nanoparticles indicate that they are electrically charged. Therefore, we believe that the cell death in the present report has an apoptotic modality. Further research is needed to determine the exact mechanism of cell death.
Conclusion
Zinc and copper metal nanoparticles of size 1 nm–2 nm produced by the underwater electrical discharge method were lethal to the cultured RG2 glioma cancer cells, while noncancerous astrocyte cells were not affected. These nanoparticles are potentially significant as nanomedicine.
Acknowledgments
The work was funded by National Institute of Standards and Technology grant number 70 NANB14H324. The authors thank M Mansour and A David for useful discussion.
Supplementary materials
Metal nanoparticles
Metal nanoparticles were produced by the modification as per the method described.Citation1,Citation2 The system consists of a water container and a high-voltage generator connected with two metal electrodes submerged in water. By controlling the voltage and distance between the electrodes, the plasma created under water produces a very fine dispersion of metal into nanoparticles ().
Two metal electrodes (99.9999%; Alfa Aesar, Haverhill, MA, USA) of 2 mm diameter are positioned in a large Pyrex jar 1 mm below the gas–water interface at a distance of ≈0.5 cm. A total of 750 mL of liquid chromatography - mass spectrometry (LC-MS) grade water (Omnisolv, Charlotte, NC, USA) is used in this procedure (). The jar filled with nitrogen is placed in the water bath with running water to prevent overheating. An AC voltage of 15,000 volts is applied to electrodes and the electric discharge is sustained for 1 hour. The water suspension is collected in 1 L glass beaker and placed in the refrigerator for 12 hours to allow large metal particles to sediment. Then the suspended particles are separated from the sediment and subjected to centrifugation at 15,000× g for 2 hours at 25°C. After centrifugation, the pellet is discarded and the supernatant is subjected to further centrifugations to produce fractions of nanoparticles enriched in particles of particular sizes. The centrifuge speed and time to separate nanoparticles by size are estimated with Stock’s equation. The particle suspensions are analyzed similarly to that described in the work by Samoylov et al.Citation3 The total concentration of metal in suspension was measured by atomic absorption spectra (GTW Analytical Services, Memphis, TN, USA), and the size and the number of particles are determined by atomic force microscopy.
Microscopy
The illumination optical systemCitation4 with a high-aperture cardioid annular condenser has been used in this work. The system produces a highly oblique hollow cone of light (numerical aperture [NA]=1.2–1.4). The illumination system is positioned in an Olympus BX51 microscope by replacing a regular condenser. The illumination system is connected with a light source (EXFO120; Photonic Solution Ltd, Edinburgh, UK) by a liquid light guide. The objective used for this work is an infinity-corrected objective HCX PL APO 100/1.40–0.70, oil, iris from Leica. The image is magnified by a zoom intermediate lens (2×-U-CA, Olympus Corporation, Shinjuku, Tokyo City, Tokyo, Japan), a homebuilt 40× relay lens, and captured by a Sony MCC-500MD video and a Dimension 8200 Dell computer. The microscope is placed on a vibration-isolated platform (manufactured by TMC, Peabody, MA, USA).
Atomic force microscopic images
The images of metal nanoparticles were taken by Bruker MultiMode 8 (Santa Barbara, CA, USA) atomic force microscope (AFM) in tapping® (intermittent-contact) mode, using PointProbe® Plus SEIKO microscopes – Non-Contact/Tapping Mode High Force Constant (PPP-SEIH) made by Nanosensors™ (Neuchatel, Switzerland) AFM probes; the nominal values specified by the vendor for the force constant and resonance frequency of these probes are 15 N/m and 130 kHz, respectively. The AFM imaging was used to measure the size distribution of particles. Monolayers of zinc and copper nanoparticles were prepared on a mica substrate for all measurements by adding small amount of 0.01% nanoparticles solution on freshly cleaved mica surfaces.
XPS spectra
X-ray photoelectron spectroscopy (XPS) was used to make quantitative spectroscopic measurements of the elemental composition of the nanoparticles’ surfaces. The Kratos Axis Ultra delay-line detector instrument in hybrid mode using a monochromatic Al Kα1,2 X-ray source (hυ=1,486.6 eV) was used. High-resolution spectra of Zn 2p (1,017–1,057 eV) and Cu 2p (925–965 eV) were acquired using a pass energy of 40 eV with an energy resolution of 0.1 eV. Zinc and copper nanoparticles were analyzed in a water suspension on a silicon wafer.
Cell viability assay
A cell viability assayCitation5 quantitatively determined the effect of metal nanoparticles on cell viability. Cells were plated in D5648 media (Sigma 96-well polystyrene plates) at a density of 3×103 cells/well. At 24 hours after plating, the medium was replaced with Dulbecco’s Modified Eagle’s Medium (100 μL/well) containing either 1 μmol/L staurosporine or zinc or copper nanoparticles with various concentrations (0.05–0.3 nm). At 20 hours after treatment, a 20 μL aliquot of tetrazolium salt (3-[4,5-dimethylthiazol- 2-yl]-2,5-diphenyl tetrazolium bromide; MTT, 5 mg/mL in phosphate buffer solution [PBS]) was added to each well, and the cells were incubated for 4 hours at 37°C. MTT was reduced in metabolically active cells to form purple formazan crystals that were subsequently dissolved in dimethyl sulfoxide and quantified by a plate reader (Bio-Rad, Hercules, CA, USA). The dye is cleared to a colored product by the activity of NAD(P) H-dependent dehydrogenase enzymes, and this indicates the level of energy metabolism in cells. The color development (yellow to blue) is proportional to the number of metabolically active cells. The analysis was carried out with Origin: Data Analysis and Graphing Software (OriginLab).
Binding equations
The purpose of this section is to present a quantitative description of binding by using known binding equations and to describe the interaction of metal nanoparticles (M) and target molecules in cells (T) and resulting complexes in terms of binding parameters. The applicability of the binding equations to the M–T system is not trivial and special considerations are required. The reaction between M and T can be schematically presented as follows:
The association binding constant (Kb) for this reaction can be defined using the mass action law.Citation6,Citation7
If we neglect the number of target molecules bound to nonspecific molecules, then the total number of the target-molecule binding sites (CT) is composed of the free binding sites and the sites bound to the metal nanoparticles:
Combining EquationEquations S2(S1) and EquationS3
(S2) , we can determine the fraction of the target-molecule binding sites occupied by the metal nanoparticles:
The ratio of occupied and free target-molecule binding sites can be defined as follows:
Taking the logarithm of both sides, we get
A plot of the left-hand side of EquationEquation S6(S5) versus log[M] is known as a Hill plot. It gives an estimate of n from the slope and Kb from the ordinate intercept.
We can speculate that the binding of the metal nanoparticles and the target molecules in cells results in cell death. Then the concentration of the TMn complexes is directly proportional to the relative cell mortality. If we denote the number of cells at no exposure to metal nanoparticles as N and the number of dead cells at the metal nanoparticles of concentration [M] as Nd, then the relative cell mortality (D) is equal to the following:
Similarly, the relative cell viability (V) is equal to:
From EquationEquations S4(S3) , EquationS7
(S6) , and EquationS8
(S7) , it is inferred that EquationEquation S6
(S5) can be presented as follows:
Thus, the measurements of the cell viability as a function of the concentration of metal nanoparticles yield values of the association constant and the valence of the binding of metal nanoparticles and the cell target molecules.
Zeta potential
Zeta potentials were calculated with Henry’s equation:
Disclaimer
Certain commercial equipment, instruments, or materials are identified in this paper to specify the experimental procedure adequately. Such identification is not intended to imply recommendation or endorsement by the National Institute of Standards and Technology, nor is it intended to imply that the materials or equipment identified are necessarily the best available for the purpose.
References
- TokushigeMNishikioriTItoYPlasma-induced cathodic discharge electrolysis to form various metal/alloy nanoparticlesRuss J Electrochem2010466619626
- KruytHRColloid ScienceVINew YorkElsevier1952
- SamoylovAMSamoylovaTIPustovyyOMNovel metal clusters isolated from blood are lethal to cancer cellsCells Tissues Organs2005179311512415947462
- VainrubAPustovyyOVodyanoyVResolution of 90 nm (lambda/5) in an optical transmission microscope with an annular condenserOpt Lett200631192855285716969401
- BerridgeMVHerstPMTanASTetrazolium dyes as tools in cell biology: new insights into their cellular reductionBiotechnol annual review200511127152
- ConnorsKABinding Constants: The Measurements of Molecular Complex StabilityNew YorkJohn Wiley & Sons1987
- KuchelPWRalstonGBTheory and Problems of Bio-chemistryNew YorkMcGraw-Hill1988
- JachimskaBWasilewskaMAdamczykZCharacterization of Globular Protein Solutions by Dynamic Light Scattering, Electrophoretic Mobility, and Viscosity MeasurementsLangmuir2008246866687218512882
Disclosure
The authors report no conflicts of interest in this work. Certain commercial equipment, instruments, or materials are identified in this paper to specify the experimental procedure adequately. Such identification is not intended to imply recommendation or endorsement by the National Institute of Standards and Technology nor is it intended to imply that the materials or equipment identified are necessarily the best available for the purpose.
References
- SamoylovAMSamoylovaTIPustovyyOMNovel metal clusters isolated from blood are lethal to cancer cellsCells Tissues Organs2005179311512415947462
- GhoshRGoswamiUGhoshSSPaulAChattopadhyayASynergistic anticancer activity of fluorescent copper nanoclusters and cisplatin delivered through a hydrogel nanocarrierACS Appl Mater Interfaces20157120922225475566
- JoseGPSantraSMandalSKSenguptaTKSinglet oxygen mediated DNA degradation by copper nanoparticles: potential towards cytotoxic effect on cancer cellsJ Nanobiotechnol201199
- StuderAMLimbachLKVan DucLNanoparticle cytotoxicity depends on intracellular solubility: comparison of stabilized copper metal and degradable copper oxide nanoparticlesToxicol Lett2010197316917420621582
- BastowMKriedtCLBaldassareJShahMKleinCZinc is a potential therapeutic for chemoresistant ovarian cancerJ Exp Ther Oncol20119317518122070048
- KriedtCLBaldassareJShahMKleinCZinc functions as a cytotoxic agent for prostate cancer cells independent of culture and growth conditionsJ Exp Ther Oncol20108428729521222361
- ShahMRKriedtCLLentsNHDirect intra-tumoral injection of zinc-acetate halts tumor growth in a xenograft model of prostate cancerJ Exp Clin Cancer Res20092818419534805
- UzzoRGLeavisPHatchWZinc inhibits nuclear factor-kappa B activation and sensitizes prostate cancer cells to cytotoxic agentsClin Cancer Res20028113579358312429649
- BaeSNKimJLeeYSKimJDKimMYParkLOCytotoxic effect of zinc–citrate compound on choriocarcinoma cell linesPlacenta2007281223016503348
- HashemiMGhavamiSEshraghiMBooyEPLosMCytotoxic effects of intra and extracellular zinc chelation on human breast cancer cellsEur J Pharmacol2007557191917169355
- MathewMEMohanJCManzoorKNairSVTamuraHJayakumarRFolate conjugated carboxymethyl chitosan–manganese doped zinc sulphide nanoparticles for targeted drug delivery and imaging of cancer cellsCarbohyd Polym2010802442448
- KruytHRColloid ScienceVINew YorkElsevier1952
- TokushigeMNishikioriTItoYPlasma-induced cathodic discharge electrolysis to form various metal/alloy nanoparticlesRuss J Electrochem2010466619626
- BerridgeMVHerstPMTanASTetrazolium dyes as tools in cell biology: new insights into their cellular reductionBiotechnol Ann Rev20051112715216216776
- VainrubAPustovyyOVodyanoyVResolution of 90 nm (lambda/5) in an optical transmission microscope with an annular condenserOpt Lett200631192855285716969401
- XueL-YChiuS-MOleinickNLStaurosporine-induced death of MCF-7 human breast cancer cells: a distinction between caspase-3-dependent steps of apoptosis and the critical lethal lesionsExp Cell Res200328313514512581734
- NdongCTateJAKettWCTumor cell targeting by iron oxide nanoparticles is dominated by different factors in vitro versus in vivoPLoS One2015102e011563625695795
- KohaneDSMicroparticles and nanoparticles for drug deliveryBiotechnol Bioeng200796220320917191251
- SchaeublinNMBraydich-StolleLKSchrandAMSurface charge of gold nanoparticles mediates mechanism of toxicityNanoscale20113241042021229159