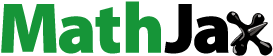
Abstract
Background
Renal epithelial cell injury facilitates crystal adhesion to cell surface and serves as a key step in renal stone formation. However, the effects of cell injury on the adhesion of nano-calcium oxalate crystals and the nano-crystal-induced reinjury risk of injured cells remain unclear.
Methods
African green monkey renal epithelial (Vero) cells were injured with H2O2 to establish a cell injury model. Cell viability, superoxide dismutase (SOD) activity, malonaldehyde (MDA) content, propidium iodide staining, hematoxylin–eosin staining, reactive oxygen species production, and mitochondrial membrane potential (Δψm) were determined to examine cell injury during adhesion. Changes in the surface structure of H2O2-injured cells were assessed through atomic force microscopy. The altered expression of hyaluronan during adhesion was examined through laser scanning confocal microscopy. The adhesion of nano-calcium oxalate monohydrate (COM) and calcium oxalate dihydrate (COD) crystals to Vero cells was observed through scanning electron microscopy. Nano-COM and COD binding was quantitatively determined through inductively coupled plasma emission spectrometry.
Results
The expression of hyaluronan on the cell surface was increased during wound healing because of Vero cell injury. The structure and function of the cell membrane were also altered by cell injury; thus, nano-crystal adhesion occurred. The ability of nano-COM to adhere to the injured Vero cells was higher than that of nano-COD crystals. The cell viability, SOD activity, and Δψm decreased when nano-crystals attached to the cell surface. By contrast, the MDA content, reactive oxygen species production, and cell death rate increased.
Conclusion
Cell injury contributes to crystal adhesion to Vero cell surface. The attached nano-COM and COD crystals can aggravate Vero cell injury. As a consequence, crystal adhesion and aggregation are enhanced. These findings provide further insights into kidney stone formation.
Introduction
Crystal formation and retention lead to kidney stone development.Citation1 Crystal retention can be caused by the adherence of crystals to renal tubular epithelial cells.Citation2 Thus, stone development occurs when crystals bind to the epithelial cell surface.Citation3 Epithelial cell injury induces crystals to attach to the site of injury.Citation4,Citation5 Cell injury is also required for crystal–cell adhesion.Citation6–Citation8
Exposure to calcium oxalate (CaOx) crystals can induce oxidative stress in renal epithelial cells; oxidative stress occurs during the attachment of crystals to renal tubular cells.Citation9 CaOx crystals can generate toxicity in renal epithelial cells via several approaches; for instance, crystals can change the composition and function of membrane surfaces, disrupt membrane potential, increase lipid peroxidation, promote mitochondrial dysfunction, induce reactive oxygen species (ROS) production, and cause an imbalance in the antioxidant mechanism;Citation10 as a result, renal epithelial cells become injured. Injured cells express crystal adhesion molecules, such as hyaluronic acid (HA) and OPN, which promote crystal adhesion and retention.
Approximately 80% of kidney stones are composed of CaOx.Citation11 The most common forms are calcium oxalate monohydrate (COM) and calcium oxalate dihydrate (COD).Citation12 An important number of COM stones are developed as a consequence of the transformation of thermodynamically unstable COD crystals into stable COM crystals.Citation13 This is a liquid (urine)-mediated process, but takes place once the stone is generated, and is a slow process. This means that the first generated crystals were COD, not COM crystals, and in spite of this transformation, the calculus can be considered a COD calculus. In fact, the etiology of such calculi is exactly the same as the COD calculi.
Urinary crystals in healthy persons and lithogenic patients are frequently diverse in terms of crystal size and phase.Citation14,Citation15 Laser-scattering spectroscopy has revealed that the particle size distribution of nano-crystallites in healthy urine is relatively uniform; conversely, this distribution is nonuniform in lithogenic urine, and particle size broadly increases from 100 nm to more than 1,000 nm.Citation16 The nonuniform nano-particles are easily aggregated; as a consequence, stone formation may occur.
Studies have demonstrated the adhesion of COM and COD to renal tubular epithelial cells; however, studies have focused on micron-graded crystals.Citation17,Citation18 Although these micron crystals are usually formed from nano-crystals,Citation19 especially urinary crystals that require a short residence time to flow through renal tubules, numerous nano-crystals are also formed. Therefore, the adhesion of nano-COM and COD to renal epithelial cells, especially injured renal epithelial cells, should be further investigated. Nano-sized crystals cause more serious injury than micron-sized crystals in normal epithelial cells.Citation20 Our study evaluated the differences in the ability of nano-COM and COD to adhere to injured Vero cells and provided the relationship between cell injury and nano-crystal adhesion. Our results helped reveal the mechanisms of the formation of COM papillary renal stones or COD stones associated with tubular Randall’s plugs.
Materials and methods
Materials and equipment
African green monkey kidney epithelial (Vero) cells were purchased from Shanghai Institute of Cell Bank (Shanghai, People’s Republic of China). Other materials included the Dulbecco’s Modified Eagle’s Medium (HyClone, Logan, UT, USA), fetal bovine serum (Hangzhou Evergreen Biological Engineering Materials Co., Ltd., Hangzhou, People’s Republic of China), and penicillin and streptomycin (Beijing probe biological technology Co., Ltd, Beijing, People’s Republic of China). Cell culture plates were purchased from Wuxi Nest Bio-Tech Co., Ltd. (Wuxi, People’s Republic of China).
Cell proliferation assay kit (Cell Counting Kit-8 [CCK-8]) was purchased from Dojindo Laboratory, Kumamoto, Japan. Superoxide dismutase (SOD) kit and malonaldehyde (MDA) kit were purchased from Nanjing Jiancheng Institute of Biotechnology (Nanjing, People’s Republic of China). Propidium iodide (PI), anti-quencher, hematoxylin–eosin (H&E) dye, 2′,7′-dichlorofluorescein diacetate dye (DCFH-DA) and 5,5′,6,6′-tetrachloro-1,1′,3,3′-tetraethyl-imidacarbocyanine iodide were purchased from Shanghai Beyotime Bio-Tech Co., Ltd. (Shanghai, People’s Republic of China). bHABP was purchased from Merck Corporation (Darmstadt, Germany), Fluorescein isothiocyanate–avidin was purchased from Wuhan Boster Biological Engineering Co., Ltd (Wuhan, People’s Republic of China). Other conventional reagents were analytically pure and purchased from Guangzhou Chemical Reagent Factory (Guangzhou, People’s Republic of China).
Apparatus included X-L type environmental scanning electron microscope (Philips, Eindhoven, the Netherlands), D/max2400X X-ray powder diffractometer (Rigaku Corporation, Tokyo, Japan), laser scanning confocal microscope (LSM510 META Duo Scan, Carl Zeiss Meditec AG, Jena, Germany), inverted fluorescence microscope (IX51, Olympus Corporation, Tokyo, Japan), flow cytometer (FACS Aria, BD Biosciences, San Jose, CA, USA), microplate reader (Safire2, Tecan, Mannedorf, Switzerland), inductively coupled plasma atomic emission spectrometer (Optima 2000DV, PerkinElmer Inc., Waltham, MA, USA), and atomic force microscope (AutoProbe CP, Thermomicroscopes, Veeco, Plainview, NY, USA).
Experimental methods
Synthesis and characterization of nano-COM, COD crystals
According to our previous research,Citation21 nano-COM and COD crystals were synthesized. For synthesis of nano-COM, CaCl2 (300 mmol/L) and K2Ox (300 mmol/L) solutions were prepared in water. A 50 mL aliquot of each solution was mixed directly at 25°C reaction temperature. The reaction mixture was stirred with a magnetic stirrer (1,250 rpm) followed by washing twice with anhydrous ethanol under ultrasonication. The crystals were collected by suction filtration and dried in drying oven for 24 hours. For synthesis of nano-COD, CaCl2 solution (192 mmol/L) was added to buffer solution (pH =6.8), before adding K2Ox solution (50 mmol/L) to reaction mixture at 25°C. The reaction mixture was stirred with a magnetic stirrer (1,250 rpm) followed by washing twice with anhydrous ethanol under ultrasonication. The crystals were collected by suction filtration and dried in drying oven for 24 hours. The morphology and crystal phase of the prepared sample were characterized by scanning electron microscopy (SEM) and X-ray powder diffraction.
Cell culture
African green monkey kidney epithelial (Vero) cells in our experiments were obtained from Shanghai Institute of Cell Bank (Shanghai, People’s Republic of China). As no in vivo experiments were performed in this study, ethical consent to use these cell lines was not needed, as approved by the ethics committee of Jinan University, Guangzhou, People’s Republic of China. Vero cells were cultured in Dulbecco’s Modified Eagle’s Medium-F12 culture medium supplemented with 10% fetal bovine serum. Cells were incubated in an atmosphere of 5% CO2 air at37°C and saturated humidity. After the cells were trypsinized, 100 μL of cell suspension (1×105 cells/mL) was plated in 96-well plates and cultured for 24 hours. The culture medium was removed by suction and the cells were washed twice with phosphate-buffered saline (PBS). The experimental model was divided into three groups: a) normal control group: only serum-free medium was added; b) injury control group: cells were exposed to a serum-free medium containing 0.3, 0.5, and 1.0 mmol/L H2O2 for 1 hour; c) crystal-treated group: cells were treated with 0.3, 0.5, and 1.0 mmol/L H2O2 for 1 hour, after which the culture medium was removed by suction, washed twice with PBS, then incubated with 100 μg/mL serum-free medium containing nano-COM or COD for 6 hours.
We selected 6 hours of incubation in the following cell experiments because the transit time of urinary crystals across the kidney is only several minutes;Citation1 this period is too short for crystals to become large enough to be trapped. After the crystals become fixed on the surface of renal tubular epithelial cells, the free urinary crystals can grow and form stones. These adhered crystals could be fixed on the epithelial cell surface for a long time and could even grow large enough to block renal tubules. The treatment time of 6 hours in this study is consistent with that described in previous studies on kidney stones.Citation22,Citation23
We selected the concentrations of H2O2 and crystals based on the following reasons. The commonly used H2O2 concentration to induce cell injury ranged from 10 to 1,000 μmol/L.Citation24,Citation25 We also set a series of crystal concentrations (50, 100, 200, and 400 μg/mL) in our preexperiment; among these concentrations, 100 μg/mL yielded moderate results. This concentration did not induce severe cellular damage or minor cell injury; this status could be easily compared in terms of the differences in adhesion behavior and reinjury ability. The CaOx concentration utilized in several studies is also 100 μg/mL, which is a frequently used dose.Citation26,Citation27
Cell viability, SOD activity, and MDA content detection
An amount of 100 μL of cell suspension (1×105 cells/mL) was plated per well in 96-well plates, and incubated in Dulbecco’s Modified Eagle’s Medium culture medium containing 10% fetal bovine serum for 24 hours. After that, the cells were divided into three groups as per the “Cell culture” section, and then cell viability assay was carried out using the CCK-8 test method. Simultaneously, SOD activity and MDA content were measured using the SOD and MDA kits, respectively.
PI staining
PI staining assay was performed on cells of each group as per the “Cell culture” section. After the treatment time, the supernatant was removed by suction and cells were washed three times with PBS, and then 5 μL PI staining solution was added and the cells were incubated at 4°C for 20–30 minutes. The cells were washed with PBS three times again, followed by observing the dead cells under the fluorescence microscope.
PI quantitative analysis: 100 μL of cell suspension was inoculated in 96-well plates with the concentration of 1×105 cells/mL, PI fluorescence intensity was measured directly in accordance with the earlier operation using a microplate reader.
H&E staining
An amount of 1 mL cell suspension (1×105 cells/mL) was seeded per well in 12-well plates and grouped as discussed in the “Cell culture” section. After the treatment time, the supernatant was removed by suction and washed three times with PBS. Afterwards, the cells were fixed with 4% paraformaldehyde for 15 minutes at room temperature. Cells were washed thrice with PBS. After fixation, the cells were stained with hematoxylin and incubated for 15 minutes. Then cells were washed with distilled water for 2 minutes to remove excess stain. After that, the cells were stained with eosin staining solution for 5 minutes. The cells were washed with distilled water for 2 minutes to remove excess eosin. After treatment, the cells were observed under the microscope, cell nucleus was stained purple or blue, cytoplasm was stained pink or red.
Atomic force microscopy analysis
An amount of 1 mL cell suspension with a cell density of 1×105 cells/mL was plated per well in 12-well plates. Afterward, the cells were treated with 0, 0.3, and 1.0 mmol/L H2O2 and incubated for 1 hour. Then, atomic force microscopy (AFM) was used to observe the morphology of cell and structural change of cell surface. The values of cell surface roughness, mean height, and distribution of particle size on cell surface were obtained automatically by AFM with software IP 2.1. (Thermomicroscopes, Veeco, Plainview, NY, USA) Scanning range of nuclear area was 5×5 μm. Histogram of the cell surface particles was also obtained automatically by AFM. Root-mean-square roughness and average roughness of cell surface were obtained by the following equations:
ROS generation
ROS production of each group was measured according to our previous research.Citation19 In brief, 2 mL of cell suspension with a cell concentration of 1×105 cells/mL was inoculated per well in six-well plates. After synchronization, the cells were divided into three groups as per the “Cell culture” section. The cells were resuspended by adding 500 mL PBS in a microcentrifuge tube. The samples were then stained with 2′,7′-dichlorofluorescein diacetate. ROS distribution was observed under fluorescent microscope; the fluorescence intensity of intracellular ROS was quantitatively detected by microplate reader.
Measurement of mitochondrial membrane potential (Δψm)
The cell suspension (2 mL) with a concentration of 1×105 cells/mL was inoculated per well in six-well plates for 24 hours. After synchronization, the cells were divided into three groups as per the “Cell culture” section. After 6 hours of incubation with nano-COD and COM crystals at the concentration of 100 μg/mL, the supernatant was aspirated and the cells were washed twice with PBS and digested with 0.25% trypsin. The cells were suspended by pipetting, followed by centrifugation (1,000 rpm, 5 minutes). The supernatant was aspirated and the cells were washed with PBS and centrifuged again to obtain a cell pellet. The cells were resuspended by adding and thoroughly mixing 500 μL of PBS in a microcentrifuge tube. Finally, the samples were stained with 5,5′,6,6′-tetrachloro-1,1′,3,3′-tetraethyl-imidacarbocyanine iodide and then quantitatively detected by flow cytometer.
Hyaluronic acid (HA) detection
HA detection was analyzed in the media as described previously.Citation28 Briefly, 1 mL of cell suspension with a cell concentration of 1×105 cells/mL was inoculated per well in 12-well plates. After synchronization, the cells were grouped; 0.3, 0.5, and 1.0 mmol/L H2O2 were used to damage Vero cells. Then 100 μg/mL nano-COM or COD crystals were added to the injured cells. After 6 hours’ incubation, the supernatant was aspirated and the cells were washed twice with PBS. Afterward, the cells were fixed with fixative (composed of 5% glacial acetic acid, 10% formalin, and 70% ethyl alcohol) and washed with PBS three times. An amount of 100 μL of 5 mg/mL bHABP solution was then added to the cells and incubated at 4°C overnight. After washing thrice with PBS, 100 μL of fluorescein isothiocyanate–avidin was added to the cells and they were incubated for 1 hour. The prepared samples were mounted with anti-fade fluorescence mounting medium and observed using a confocal microscope.
Quantitative analysis: HA fluorescence intensity was analyzed using Axiovision software (Carl Zeiss Meditec AG). HA expressions in 100 cells were quantitatively detected for each group.
SEM observation of crystal adhesion to cell surface
Cells were grouped as per the “Cell culture” section; after incubating with 100 μg/mL nano-COM and COD crystals for 6 hours, the supernatant was removed by suction and cells were washed three times with PBS, fixed in 2.5% glutaraldehyde at 4°C for 24 hours, then fixed with 1% OsO4, washed again three times with PBS, dehydrated in gradient ethanol (30%, 50%, 70%, 90%, and 100%, respectively), dried under the critical point of CO2, treated with gold sputtering, and finally observed under SEM.
Quantitative determination of crystal adhesion
An amount of 1 mL of cell suspension with a cell concentration of 1×105 cells/mL was inoculated per well in 12-well plates and incubated for 12 hours. Cells were grouped as per the “Cell culture” section. After 6 hours’ incubation, the supernatant was aspirated and the cells were washed three times with PBS to remove unbound crystals. The samples were then transferred to a 25 mL beaker and mixed with 4.0 mL concentrated HNO3 and 1.0 mL HClO4 solution for digestion. A separate HClO4 solution was heated until smoke appeared; the remaining heat was used to dry the solution. After cooling, 3 mL of 2% HNO3 was added. An inductively coupled plasma atomic emission spectrometer was used to measure the concentration of Ca2+ ions, which was then converted to determine the amount of crystal adhesion. The control group was treated using the same method to determine the interference of intracellular Ca2+ in Vero cells.
Statistical analysis
Experimental data were expressed as the mean ± standard deviation. The experimental results were analyzed statistically using SPSS 13.0 software (SPSS Inc., Chicago, IL, USA).
Results
Characterization of nano-COM and COD
The COM and COD crystals of approximately 100 nm were prepared in accordance with our previous research.Citation21 The morphological characteristics, crystal size, and crystal phase were detected through SEM and X-ray powder diffraction; all of the prepared crystals were confirmed as target products (). The prepared crystals presented a nearly spherical morphology with some irregularities. The crystal phase was detected through X-ray powder diffraction. The prepared samples were pure-phase COM or COD crystals, and no other impure diffraction peaks were observed.
Figure 1 Characterization of nano-COM and COD crystals.
Notes: SEM images (A, B) and XRD spectra (C, D) of nano-COM and COD crystals. (A, C) COM crystal; (B, D) COD crystal. Scale bars: 200 nm.
Abbreviations: SEM, scanning electron microscopy; XRD, X-ray powder diffraction; COM, calcium oxalate monohydrate; COD, calcium oxalate dihydrate; au, arbitrary units.

Changes in cell viability, SOD activity, and MDA content
The extent of oxidative stress in Vero cells after treatment with H2O2 and further treatment with nano-COM and COD crystals is indicated in . The decreased cell viability () and antioxidant activity (SOD; ), as well as the increased lipid peroxidation products (MDA content; ), reflect oxidative stress and cell injury. When Vero cells were treated with H2O2, the cell viability and SOD activity were decreased, whereas the MDA content was increased. Moreover, the c(H2O2) range of 0–1.0 mmol/L exhibited a concentration-dependent effect on all parameters. Nano-COM or COD crystals aggravated cell injury, which further decreased cell viability and SOD activity while increasing the MDA content. The reinjury effect of nano-COM was stronger than that of nano-COD on cells under the same conditions.
Figure 2 Cell viability, SOD activity, and MDA content detection.
Notes: Changes of cell viability (A), SOD activity (B), and MDA content (C) of injured Vero cells after exposure to 100 μg/mL nano-COM or COD crystals for 6 hours. The injured Vero cells were obtained by different concentrations of H2O2 treatment (0 mmol/L, 0.3 mmol/L, 0.5 mmol/L, 1.0 mmol/L). Data were expressed as mean ± standard deviation from three independent experiments.
Abbreviations: COM, calcium oxalate monohydrate; COD, calcium oxalate dihydrate; SOD, superoxide dismutase; MDA, malonaldehyde.

Cell death detection by PI staining
The results of PI staining in Vero cells at different injury levels and after interaction with nano-COM and COD for 6 hours are shown in . When c(H2O2) was increased from 0.3 mmol/L to 0.5 mmol/L and 1.0 mmol/L, the number of PI-stained nuclei and the relative fluorescence intensity () in the control group, nano-COM, and nano-COD groups were all gradually increased, thereby indicating that the overall cell death rate was gradually increased.Citation29 Nano-COM and COD crystals can reinjure the injured Vero cells, which further increases the number of PI-stained nuclei. More nuclei were stained by PI, and the relative fluorescence intensity was stronger in the nano-COM group than in the nano-COD group (), thereby indicating that the nano-COM crystals induced higher cell death than the nano-COD crystals.
Figure 3 PI staining detection of injured Vero cells after exposure to nano-COM and COD crystals.
Notes: PI staining detection by fluorescence microscope (A) and relative fluorescence intensity by microplate reader (B) of injured Vero cells after exposure to 100 μg/mL nano-COM or COD crystals for 6 hours. The injured Vero cells were obtained by different concentrations of H2O2 treatment (0, 0.3, 0.5, 1.0 mmol/L). Data were expressed as mean ± standard deviation from three independent experiments. Magnification: ×600.
Abbreviations: COM, calcium oxalate monohydrate; COD, calcium oxalate dihydrate; PI, propidium iodide.

Cell morphological observation by H&E staining
To observe the injury in Vero cells, the cells were stained with H&E, which demonstrates the overall morphology of cells. Hematoxylin is an alkaline dye that stains chromatin in the nucleus with blue–purple. While eosin is an acidic dye which stains cytoplasm red or pink.Citation30,Citation31
With the increasing H2O2 concentration, the degree of injury of cells increases, which is mainly manifested in the reduced cell number and disordered cell morphology (). The number of cells in the nano-COM- and COD-treated group was less than the cells in the control group, which was only treated with H2O2. The cell nuclei of the crystal-treated group were shrunk and stained blue–black. Simultaneously, the cells were loosely arranged, and the intercellular connections were further destroyed. After treatment with 0.5 mmol/L and 1.0 mmol/L of H2O2, the morphological disorder of cells in the nano-COM treatment group was exceptionally obvious.
Figure 4 Morphology observation by H&E staining of injured Vero cells after exposure to 100 μg/mL nano-COM or COD crystals for 6 hours.
Notes: The nucleus was stained blue or purple, and the cytoplasm was pink or red. Injury time of H2O2: 1 hour; magnification: ×400.
Abbreviations: COM, calcium oxalate monohydrate; COD, calcium oxalate dihydrate; H&E, hematoxylin–eosin.

AFM detection of cell morphology and cell membrane surface structure
The cell membrane is a barrier that prevents extracellular substances from freely entering cells. After cell injury, the composition and properties of the cell membrane are changed. Consequently, we investigated the changes in the surface structure of normal and injured Vero cells treated with 0.3 mmol/L and 1.0 mmol/L H2O2 by AFM (). This approach determines the surface roughness, mean height of cell, particle size on the cell surface (), and the ultrastructure of the cell surface ().
Table 1 Comparison of the parameters of Vero cells with different degrees of damage
Figure 5 AFM detection of cell morphology and cell membrane surface structure.
Notes: AFM images of Vero cells (A–C) and the size distribution of particles on cell surface (D–F) before and after cell damage. (A, D) Normal cells; (B, D) cells treated with 0.3 mmol/L H2O2; (C, F) cells treated with 1.0 mmol/L H2O2. Thumbnails in (A–C) were the nucleus area of corresponding cells. Scanning range was 5×5 μm.
Abbreviation: AFM, atomic force microscopy.

The surface of normal Vero cells was smooth (), with less roughness (11.3 nm); the cell periphery had no protrusions, and the particle size of the cell surface was ~20–80 nm (). When the cells were treated with 0.3 mmol/L H2O2, the cell surface roughness increased to 39.3 nm and became more complex (); the particle size was distributed at 50–250 nm. Therefore, the cell surface secreted more proteins, fats, sugars, and other substances, and particulate matter covered the surface.Citation32 In addition, the cells began to shrink; the cell edges appeared fragmented in , and the average height of the cells increased to 166.1 nm (). When Vero cells were treated with 1.0 mmol/L H2O2, the cell shrinkage was increased (). Likewise, the cell surface roughness was increased to 73.36 nm, and the particle diameter was increased to ~200–500 nm (). Therefore, the amount of cell surface protein, fat, sugar, and other substances increased on the cell surface when Vero cells have been seriously injured.
Intracellular ROS generation after cell injury
ROS can rapidly react with cellular macromolecules, thereby damaging normal cell function and eventually causing cell death; the oxidation during cell injury can promote the deposition of CaOx crystals in kidneys.Citation33 The ROS produced by COD and COM crystals were measured with a 2′,7′-dichlorofluorescein diacetate fluorescence probe. Compared with the control group, H2O2 and COM or COD nanocrystals could stimulate cells to produce ROS (). The higher c(H2O2) produced a stronger green fluorescence intensity from the intracellular ROS. After nano-COD and COM crystals were added, the fluorescence intensity of intracellular ROS was further enhanced. The fluorescence intensity of the COM-treated group was slightly higher than that of the COD-treated group (), which indicated that COM produced higher cytotoxicity and generated more ROS.
Figure 6 ROS level of injured Vero cells after exposure to 100 μg/mL nano-COM or COD crystals for 6 hours.
Notes: ROS distribution was observed under fluorescent microscope (A); the fluorescence intensity of intracellular ROS was quantitatively detected by microplate reader (B). Injury time of H2O2: 1 hour; crystal concentration: 200 μg/mL; scale bars: 50 μm; magnification: ×400.
Abbreviations: COM, calcium oxalate monohydrate; COD, calcium oxalate dihydrate; ROS, reactive oxygen species.

Decreased mitochondrial membrane potential caused by nano-COM and COD
Apoptosis and necrosis are often preceded by mitochondrial dysfunction, specifically the loss of mitochondrial membrane potential (Δψm). 5,5′,6,6′-Tetrachloro-1,1′,3,3′-tetraethyl-imidacarbocyanine iodide differentially labels mitochondria with high and low Δψm by forming J-aggregates or monomers that emit orange–red or green light, respectively.Citation34
We analyzed the changes in Δψm of injured cells treated with nano-COM and COD crystals with a mitochondrial membrane potential assay kit (). Compared with the control group, H2O2 and nano-COM or COD crystals caused Δψm to decrease. The higher H2O2 concentration caused a greater Δψm decrease in Vero cells. After adding nano-COD and COM crystals, the degree of Δψm decrease was further enhanced. The decrease of Δψm in the COM-treated group was slightly higher than in the COD-treated group, thereby indicating that COM produced higher cytotoxicity and caused more mitochondrial dysfunction.
Figure 7 Effects of nano-COM or COD exposure on mitochondrial membrane potential (Δψm) in Vero cells injured by H2O2.
Notes: (A) Dot plots of Δψm after incubation with nano-COM or COD; (B) quantitative histogram of Δψm. Injury time of H2O2: 1 hour; crystal concentration: 100 μg/mL.
Abbreviations: COM, calcium oxalate monohydrate; COD, calcium oxalate dihydrate; JC-1, 5,5′,6,6′-tetrachloro-1,1′,3,3′-tetraethyl-imidacarbocyanine iodide.

Hyaluronic acid (HA) expression on the injured cell surface
HA acts as a crystal adhesion molecule that is expressed after cell injury. Thus, the HA expression can indirectly reflect the condition of injured cells.Citation35 Accordingly, we investigated the crystal adhesion mediated by the altered HA expression after cell injury.
As shown in , treatment with nano-COM and COD for 6 hours at different damage levels increased the HA expression (green color) on the cell surface in a concentration-dependent manner, as seen under a confocal microscope. With the increasing c(H2O2), the green fluorescence was gradually enhanced, thereby indicating that the HA expression was gradually increased. The green fluorescence intensity caused by nano-COM crystals was obviously stronger compared with that of the nano-COD crystals. Consequently, higher HA expression was induced by nano-COM, and the degree of cell injury was higher.
Figure 8 HA expression of injured Vero cells after exposure to 100 μg/mL nano-COM or COD crystals for 6 hours.
Notes: (A) HA expression observation by laser confocal microscope and (B) histogram of quantitative fluorescence intensity. Crystal concentration: 100 μg/mL; injury time of H2O2: 1 hour. Scale bars: 20 μm. Magnification: ×10,000.
Abbreviations: COM, calcium oxalate monohydrate; COD, calcium oxalate dihydrate; HA, hyaluronic acid.

SEM of crystal binding
The SEM micrographs in and show the different degrees of Vero cell injury after incubation for 6 hours with nano-COM and COD crystals, respectively. Results demonstrated that the crystal binding was gradually increased on the cell surface with the increasing degree of Vero injury. When the cells were injured by 0.5 mmol/L and 1.0 mmol/L H2O2, the entire cell surface was almost covered by crystals. After 6 hours of exposure to nano-crystals, the nano-COM crystals could bind to the Vero cells much more readily than nano-COD under the same conditions. Our results also revealed that the injured cells have the ability to promote the crystal aggregation. As the degree of cell injury increased, the aggregation of adhered crystals also increased, especially the nano-COM crystals.
Figure 9 SEM observation of nano-COM crystal adhesion to H2O2-treated Vero cell surface.
Notes: Vero cells were treated with (A) 0 mmol/L (B) 0.3 mmol/L (C) 0.5 mmol/L, or (D) 1.0 mmol/L H2O2 for 1 hour and then incubated with 100 μg/mL nano-COM for 6 hours. Scale bars: 5 μm.
Abbreviations: COM, calcium oxalate monohydrate; SEM, scanning electron microscopy.

Figure 10 SEM observation of nano-COD crystal adhesion to H2O2-treated Vero cell surface.
Notes: Vero cells were treated with (A) 0 mmol/L (B) 0.3mmol/L (C) 0.5 mmol/L, or (D) 1.0 mmol/L H2O2 for 1 hour and then incubated with 100 μg/mL nano-COD for 6 hours. Scale bars: 5 μm.
Abbreviations: COD, calcium oxalate dihydrate; SEM, scanning electron microscopy.

Quantitative determination of crystal binding by inductively coupled plasma emission spectrometry
The quantitative determination of the amount of crystal adhesion on each set of cell surface conditions by inductively coupled plasma emission spectrometry is shown in . Treatment with different concentrations of H2O2 increased the degree of Vero cell injury, which enhanced the amount of nano-COM and COD crystals that adhered to the cell surface. The adhesion amount was positively related to the degree of injury caused by H2O2. Under the same conditions, the amount of crystal adhesion was greater in nano-COM crystals than in nano-COD.
Figure 11 Degree of adhesion of nano-COM, COD crystals to H2O2-treated Vero cells determined by inductively coupled plasma emission spectrometry.
Notes: Crystal concentration: 100 μg/mL; injury time: 1 hour; adhesion time: 6 hours.
Abbreviations: COM, calcium oxalate monohydrate; COD, calcium oxalate dihydrate.

Discussion
The present study demonstrated that nano-CaOx crystals induce oxidative injury in renal epithelial cells. This injury could contribute to CaOx crystal adhesion and the development of kidney stones. In this study, we used the H2O2-induced oxidative injury model of Vero cells.
H2O2 is an important ROS because it is produced in abundant amounts in several tissues; a high level of oxidants have been detected at inflammatory sites.Citation36 H2O2 causes various changes in renal epithelial cells, such as the increased free radical generation, increased lipid peroxidation, and the decreased cellular antioxidant status, which induces cell injury and eventual cell death.Citation37,Citation38 H2O2 could cause oxidative stress by reacting with transition metals, thereby damaging cell components, such as proteins, lipids, and DNA, and leading to cell death.Citation39 The toxic effect of H2O2 has been suggested to occur via hydroxyl radicals, which are generated by the Fenton or Haber–Weiss reactions. These free radicals seem to induce changes in the cell membrane integrity and function via the peroxidation reaction.Citation40,Citation41
When Vero cells were treated with different concentrations of H2O2 in this study, the cell viability ( and ), SOD activity (), and intracellular Δψm were decreased while the MDA content was simultaneously increased () with the concentration of H2O2, thereby indicating that the H2O2 decreased antioxidant capacity, increased lipid peroxidation, and damaged the cells. Lipid peroxidation represents oxidative damage by free radicals resulting in structural alteration to cell membrane (),Citation40 which has been proven by the results of AFM analysis (). H2O2 can cause membrane lesions through lipid peroxidation, promote alterations in several amino acids, and lead to the inactivation of enzymes.Citation42 Several antioxidant enzymes such as SOD, GPx, and catalase limit the extent of cell damage; however, exposure to a high concentration of H2O2 can give rise to the generation of ROS (), which decreases the antioxidant enzyme activity resulting in the cellular injury.
COM is the major crystalline component that is most frequently observed in the majority of CaOx renal stones; its crystals have been shown to exert toxic effects on renal tubular epithelial cells as a result of oxidative stress. Previous studies reported the cytotoxic effects of COM in proximal tubule cells,Citation14 human renal epithelial HK-2 cells,Citation43,Citation44 canine distal renal tubular MDCK cells,Citation45,Citation46 and LLC-PK1 cells.Citation8 COM crystals could modify the membrane structure and function, activate ROS, cause oxidant/antioxidant imbalance, lead to mitochondrial dysfunction, and increase lipid peroxidation,Citation19 which induces apoptotic or necrotic cell death in renal epithelial cells.Citation9,Citation47–Citation49 COD is the second most common component of CaOx renal stones, although existing studies on the toxic effect of COD crystals on renal epithelial cells are inadequate. Our previous study reported that nano-COD induced higher toxicity on Vero cells compared with the micro-sized crystals. The nano-COD crystals also increased the LDH release, ROS level, apoptosis rate, and the expression of adhesion molecules.Citation28 Consequently, renal epithelial cell injury occurs during the adhesion of CaOx crystals.Citation9
The cell viability (), SOD activity (), MDA content (), PI staining (), and intracellular Δψm () demonstrated that the nano-COM and COD crystals injured the Vero cells by producing oxidative stress. The generation of free radicals during the interaction between crystals and Vero cells has been evidenced by ROS production (). ROS generation was higher when the cells were exposed to higher amounts of nano-COM and COD crystals. The nano-sized crystals have a larger specific surface area and more active sites than larger crystals;Citation21 these active sites could capture oxygen molecules and produce superoxide radicals, which disrupted the antioxidant system and enhanced the lipid peroxidation in Vero cells.Citation50 H&E staining demonstrated that when cells were treated with nano-CaOx crystals, the cell-to-cell contacts appeared stretched and the intercellular spaces widened, whereas the number of cells was decreased compared with the normal control group (). Therefore, nano-CaOx crystals can injure renal epithelial cells. Nano-COM and COD could be internalized by Vero cells because of their small size. The internalized nano-crystals can directly affect mitochondria, thereby disturbing the metabolic balance and leading to mitochondrial membrane depolarization and Δψm decrease (). In addition, HA expression increased with the concentration of nano-COM and COD crystals (). HA expression on the cell surface during wound healing also indirectly reflected the extent of cell damage.Citation35 The cell injury and cell death induced by nano-COM crystals was higher than that of nano-COD crystals because nano-COM caused the higher production of ROS compared with nano-COD.
Several researchers have reported that renal tubular cell injury is associated with the crystal–cell interaction process. CaOx crystals can cause cell injury and alter the cell composition, which can affect crystal adhesion. On the other hand, another study suggested that when renal epithelial cells are damaged by any other form of injury, the adhesion of crystals is increased.Citation51
Our results identified two distinct reasons for nano-crystal attachment to Vero cells after cell injury. First, the expression of adhesion molecules on the cell surface, as a result of cell injury, facilitates crystal attachment. When Vero cells were injured by different concentrations of H2O2, cells secreted HA () to repair the epithelial wound.Citation52 The expression of HA by the injured tubular epithelial cells was most likely aimed at restoring renal function; however, HA contains a carboxyl group and a large number of negative charges, thereby turning the non-crystal-binding epithelium into a crystal-binding site.Citation47 These trends may be attributed to the fact that the degree of injury increased with the increasing c(H2O2), thereby resulting in the adhesion of COM and COD crystals on the cell surface ( and ). Additionally, the adhered crystals continuously increased the active sites to which the crystals were subsequently attached. Therefore, the number of crystals that adhered to the cell surface gradually increased (). This trend significantly increased the risk of forming kidney stones. The adherence of crystals to the Vero cell surface is illustrated in the SEM micrographs ( and ). A previous study also showed that the mechanism of injury increased the adherence of crystals after injury and during wound repair.Citation51 Another experiment suggested that the crystal binding sites are exposed on the cell surface when cells are injured and/or during regeneration after injury.Citation53
Second, AFM observation revealed that Vero cell injury by H2O2 led to cell membrane rupture and released intracellular organic substances (), thereby increasing the crystal binding sites on the cell surface. These sites can form ionic bonds, hydrogen bonds, or electrostatic forces with the CaOx crystals, thereby promoting crystal adhesion. The attached crystals caused further membrane damage, which increased CaOx crystal binding. The AFM results provide the surface roughness, mean height, and particle size of the cell surface; these parameters can explain structural changes in the cell membrane after H2O2 treatment (). The cell surface of normal cells was relatively smooth, with low adhesion force. By contrast, in Vero cells treated with H2O2, the cell surface was disrupted as visualized by the cell membrane ultrastructure (). In addition, the secreted intracellular organic substances increased the particle size on the cell surface (). Simultaneously, these secreted molecules increased the adhesion force and allowed for crystal–cell adhesion. Previous investigations with AFM have confirmed that the renal cell surface mediated the adhesion force in specific faces of COM crystals.Citation54 The SEM observation of COD crystals’ adhesion also produced similar results.Citation55 The adherent crystals on the apical cell surface could serve as sites for the aggregation of more crystals.Citation56
Furthermore, the cell injury induced by H2O2 and CaOx crystals can destroy the tight junctions and polarity of the cell membrane, which translocates the basolateral or tight junction region components to the apical surface of the cell.Citation53,Citation57 These components, such as phosphatidylserine,Citation53 and Na+/K+-ATPase-α1 protein,Citation57 contain acidic groups with a high affinity for CaOx crystals, especially for COM. Consequently, the exposure of these molecules on the membrane surface facilitates crystal adhesion to injured cells. A previous study suggested that injured cells induced the loss of membrane lipid asymmetry, which also led to phosphatidylserine exposure on the cell surface, thereby promoting crystal attachment.Citation58
Our results recognized that the degree of attachment to the cell surface was greater for nano-COM than for nano-COD (). The COM crystal surface has a higher positive charge density than COD;Citation59 thus, the negatively charged molecules of the injured cell surface showed stronger interaction with COM than COD. Therefore, the electrical charge could mediate the elevated adhesion of COM crystals to cells. A previous study also suggested that the COM may have a higher affinity for the renal tubular cell surface than COD.Citation60 Furthermore, toxicity results confirmed that the nano-COM crystals induced higher injury effects on Vero cells compared with COD, which increased the adhesion and aggregation of nano-COM (). A study by Wesson et al supported our results;Citation61 their group confirmed that the adhesion to renal tubular cells in culture was higher for COM compared with COD.
Conclusion
Our study demonstrated that Vero cell injury enhanced the cell surface expression of the crystal binding molecule HA and altered the structure and function of the cell membrane. These mechanisms facilitated the attachment of nano-CaOx crystals to the cell surface. The attached nano-COM and COD crystals elicited toxic effects on epithelial cells. Simultaneously, the attached crystals further damaged the injured Vero cells. Thus, crystal adhesion and aggregation were exacerbated. This study compared the different levels of nano-crystal adhesion with various degrees of injury in renal epithelial cells to reveal the different morbidity rates of kidney stones in healthy subjects and in patients suffering from renal trauma.
Disclosure
The authors report no conflicts of interest in this work.
Acknowledgments
We thank Jinan University analytical and testing center for technical assistance. This work was supported by the National Natural Science Foundation of China (grant no 21371077).
References
- FinlaysonBReidFThe expectation of free and fixed particles in urinary stone diseaseInvest Urol1978156442448649291
- AsselmanMVerkoelenCFCrystal-cell interaction in the pathogenesis of kidney stone diseaseCurr Opin Urol200212427127612072645
- ThamilselvanVMenonMThamilselvanSOxalate at physiological urine concentrations induces oxidative injury in renal epithelial cells: effect of alpha-tocopherol and ascorbic acidBJU Int2014114114015024460843
- KhanSRCockrellCAFinlaysonBHackettRLCrystal retention by injured urothelium of the rat urinary bladderJ Urol198413211531576726949
- GillWBRuggieroKStrausFH2ndCrystallization studies in a urothelial-lined living test tube (the catheterized female rat bladder). I. Calcium oxalate crystal adhesion to the chemically injured rat bladderInvest Urol1979173257261500327
- WiessnerJHHungLYMandelNSCrystal attachment to injured renal collecting duct cells: influence of urine proteins and pHKidney Int20036341313132012631348
- FarellGHuangEKimSYHorstkorteRLieskeJCModulation of proliferating renal epithelial cell affinity for calcium oxalate monohydrate crystalsJ Am Soc Nephrol200415123052306215579508
- ThamilselvanSKhanSRMenonMOxalate and calcium oxalate mediated free radical toxicity in renal epithelial cells: effect of antioxidantsUrol Res20033113912624656
- KhanSRCrystal-induced inflammation of the kidneys: results from human studies, animal models, and tissue-culture studiesClin Exp Nephrol200482758815235923
- JonassenJACaoLCHoneymanTScheidCRIntracellular events in the initiation of calcium oxalate stonesNephron Exp Nephrol2004982e61e6415499209
- MoeOWKidney stones: pathophysiology and medical managementLancet2006367950733334416443041
- MandelNSMandelGSUrinary tract stone disease in the United States veteran population. II. Geographical analysis of variations in compositionJ Urol19891426151615212585627
- ContiCCasatiMColomboCRealiniMBrambillaLZerbiGPhase transformation of calcium oxalate dihydrate–monohydrate: effects of relative humidity and new spectroscopic dataSpectrochim Acta A Mol Biomol Spectrosc201412841341924682057
- GuoCGMcMartinKEThe cytotoxicity of oxalate, metabolite of ethylene glycol, is due to calcium oxalate monohydrate formationToxicology2005208334735515695020
- ShapurNKUvarovVPopovIcrystallite size-is it a new predictor for renal stone burden?Urology201280598098522990052
- HeJYDengSPOuyangJMMorphology, particle size distribution, aggregation, and crystal phase of nanocrystallites in the urine of healthy persons and lithogenic patientsIEEE Trans Nanobioscience20109215616320423812
- KhaskhaliMHByerKJKhanSRThe effect of calcium on calcium oxalate monohydrate crystal-induced renal epithelial injuryUrol Res20093711619005647
- WangTThurgoodLAGroverPKRyallRLA comparison of the binding of urinary calcium oxalate monohydrate and dihydrate crystals to human kidney cells in urineBJU Int2010106111768177420230382
- PengHOuyangJMYaoXQYangREInteraction between submicron COD crystals and renal epithelial cellsInt J Nanomed2012747274737
- SunXYOuyangJMLiYBWenXLMechanism of cytotoxicity of micron/nano calcium oxalate monohydrate and dihydrate crystals on renal epithelial cellsRSC Advances20155564539345406
- SunXYOuyangJMLiuAJDingYMGanQZPreparation, characterization, and in vitro cytotoxicity of COM and COD crystals with various sizesMater Sci Eng C Mater Biol Appl20155714715626354249
- SchepersMSDuimRAAsselmanMRomijnJCSchröderFHVerkoelenCFInternalization of calcium oxalate crystals by renal tubular cells: a nephron segment-specific process?Kidney Int200364249350012846744
- HovdaKEGuoCAustinRMcMartinKERenal toxicity of ethylene glycol results from internalization of calcium oxalate crystals by proximal tubule cellsToxicol Lett2010192336537219931368
- WangFLiuQWangWLiXZhangJA polysaccharide isolated from Cynomorium songaricum Rupr. protects PC12 cells against H2O2-induced injuryInt J Biol Macromol20168722222826853824
- DuanZZLiYHLiYYDanhong injection protects cardio-myocytes against hypoxia/reoxygenation- and H2O2-induced injury by inhibiting mitochondrial permeability transition pore openingJ Ethnopharmacol201517561762526320687
- MulaySRKulkarniOPRupanagudiKVCalcium oxalate crystals induce renal inflammation by NLRP3-mediated IL-1β secretionJ Clin Invest2013123123624623221343
- TsujihataMMiyakeOYoshimuraKKakimotoKTakaharaSOkuyamaAFibronectin as a potent inhibitor of calcium oxalate urolithiasisJ Urol200016451718172311025758
- SunXYOuyangJMZhuWYLiYBGanQZSize-dependent toxicity and interactions of calcium oxalate dihydrate crystals on Vero renal epithelial cellsJ Mater Chem B20153918641878
- HezelMEbrahimiFKochMDehghaniFPropidium iodide staining: a new application in fluorescence microscopy for analysis of cytoarchitecture in adult and developing rodent brainMicron201243101031103822579654
- LiYSunLJinMSize-dependent cytotoxicity of amorphous silica nanoparticles in human hepatoma HepG2 cellsToxicol In Vitro20112571343135221575712
- KongWHParkKLeeMYLeeHSungDKHahnSKCationic solid lipid nanoparticles derived from apolipoprotein-free LDLs for target specific systemic treatment of liver fibrosisBiomaterials201334254255123092863
- RotschCRadmacherMDrug-induced changes of cytoskeletal structure and mechanics in fibroblasts: an atomic force microscopy studyBiophys J200078152053510620315
- SanpuiPChattopadhyayAGhoshSSInduction of apoptosis in cancer cells at low silver nanoparticle concentrations using chitosan nanocarrierACS Appl Mater Interfaces20113221822821280584
- SalvioliSArdizzoniAFranceschiCCossarizzaAJC-1, but not DiOC6(3) or rhodamine 123, is a reliable fluorescent probe to assess delta psi changes in intact cells: implications for studies on mitochondrial functionality during apoptosisFEBS Lett1997411177829247146
- HongSHLeeHJSohnEJAnti-nephrolithic potential of resveratrol via inhibition of ROS, MCP-1, hyaluronan and osteopontin in vitro and in vivoPharmacol Rep201365497097924145091
- FantoneJCWardPARole of oxygen-derived free radicals and metabolites in leukocyte-dependent inflammatory reactionsAm J Pathol198210733954186282132
- OuyangJMYaoXQTanJWangFXRenal epithelial cell injury and its promoting role in formation of calcium oxalate monohydrateJ Biol Inorg Chem201116340541621127923
- WhittemoreERLooDTWattJACotmanCWA detailed analysis of hydrogen peroxide-induced cell death in primary neuronal cultureNeuroscience19956749219327675214
- MenghiniRGenotoxicity of active oxygen species in mammalian cellsMutat Res198819532152303283541
- ComportiMLipid peroxidation and cellular damage in toxic liver injuryLab Invest19855365996233906270
- BurtonKPMorrisACMasseyKDBujaLMHaglerHKFree radicals alter ionic calcium levels and membrane phospholipids in cultured rat ventricular myocytesJ Mol Cell Cardiol1990229103510472126294
- FarrSBKogomaTOxidative stress responses in Escherichia coli and Salmonella typhimuriumMicrobiol Rev19915545615851779927
- KhanSRHyperoxaluria-induced oxidative stress and antioxidants for renal protectionUrol Res200533534935716292585
- WangBWuBLiuJAnalysis of altered microRNA expression profiles in proximal renal tubular cells in response to calcium oxalate monohydrate crystal adhesion: implications for kidney stone diseasePLoS One201497e10130624983625
- HackettRLShevockPNKhanSRMadin-Darby canine kidney cells are injured by exposure to oxalate and to calcium oxalate crystalsUrol Res19942241972037871629
- ThongboonkerdVSemangoenTSinchaikulSChenSTProteomic analysis of calcium oxalate monohydrate crystal-induced cytotoxicity in distal renal tubular cellsJ Proteome Res20087114689470018850734
- AsselmanMVerhulstADe BroeMEVerkoelenCFCalcium oxalate crystal adherence to hyaluronan-, osteopontin-, and CD44-expressing injured/regenerating tubular epithelial cells in rat kidneysJ Am Soc Nephrol200314123155316614638914
- SchepersMSvan BallegooijenESBangmaCHVerkoelenCFOxalate is toxic to renal tubular cells only at supraphysiologic concentrationsKidney Int20056841660166916164643
- SchepersMSvan BallegooijenESBangmaCHVerkoelenCFCrystals cause acute necrotic cell death in renal proximal tubule cells, but not in collecting tubule cellsKidney Int20056841543155316164631
- NelAXiaTMadlerLLiNToxic potential of materials at the nanolevelScience2006311576162262716456071
- VerkoelenCFvan der BoomBGHoutsmullerABSchroderFHRomijnJCIncreased calcium oxalate monohydrate crystal binding to injured renal tubular epithelial cells in cultureAm J Physiol19982745 Pt 2F958F9659612335
- AsselmanMVerhulstAVan BallegooijenESBangmaCHVerkoelenCFDe BroeMEHyaluronan is apically secreted and expressed by proliferating or regenerating renal tubular cellsKidney Int2005681718315954897
- RieseRJMandelNSWiessnerJHMandelGSBeckerCGKleinmanJGCell polarity and calcium oxalate crystal adherence to cultured collecting duct cellsAm J Physiol19922622 Pt 2F177F1841539682
- ShengXWardMDWessonJAAdhesion between molecules and calcium oxalate crystals: Critical interactions in kidney stone formationJ Am Chem Soc2003125102854285512617634
- LieskeJCTobackFGDeganelloSFace-selective adhesion of calcium oxalate dihydrate crystals to renal epithelial cellsCalcif Tissue Int19965831952008852576
- LieskeJCNorrisRSwiftHTobackFGAdhesion, internalization and metabolism of calcium oxalate monohydrate crystals by renal epithelial cellsKidney Int1997525129113019350652
- PeerapenPThongboonkerdVEffects of calcium oxalate monohydrate crystals on expression and function of tight junction of renal tubular epithelial cellsLab Invest20119119710520856225
- BigelowMWWiessnerJHKleinmanJGMandelNSSurface exposure of phosphatidylserine increases calcium oxalate crystal attachment to IMCD cellsAm J Physiol19972721 Pt 2F55F629039049
- TunikLFüredi-MilhoferHGartiNAdsorption of sodium diisooctyl sulfosuccinate onto calcium oxalate crystalsLangmuir1998141233513355
- MandelNCrystal-membrane interaction in kidney stone diseaseJ Am Soc Nephrol199455 Suppl 1S37S457873743
- WessonJAWorcesterEMWiessnerJHMandelNSKleinmanJGControl of calcium oxalate crystal structure and cell adherence by urinary macromoleculesKidney Int19985349529579551403