Abstract
Since the dawn of civilization, it has been understood that pathogenic microorganisms cause infectious conditions in humans, which at times, may prove fatal. Among the different virulent properties of microorganisms is their ability to form biofilms, which has been directly related to the development of chronic infections with increased disease severity. A problem in the elimination of such complex structures (biofilms) is resistance to the drugs that are currently used in clinical practice, and therefore, it becomes imperative to search for new compounds that have anti-biofilm activity. In this context, nanotechnology provides secure platforms for targeted delivery of drugs to treat numerous microbial infections that are caused by biofilms. Among the many applications of such nanotechnology-based drug delivery systems is their ability to enhance the bioactive potential of therapeutic agents. The present study reports the use of important nanoparticles, such as liposomes, microemulsions, cyclodextrins, solid lipid nanoparticles, polymeric nanoparticles, and metallic nanoparticles, in controlling microbial biofilms by targeted drug delivery. Such utilization of these nanosystems has led to a better understanding of their applications and their role in combating biofilms.
Introduction
The incidence of infectious diseases continues to grow at an exponential rate each year and has a direct association with high rates of morbidity and mortality.Citation1 The complex dynamics of infectious diseases has caught the attention of several scientists to investigate the possible causes that lead to the persistence and spread of acute and chronic infections.Citation2 Acute infections are triggered by microbial cells that are in their planktonic forms; however, over time such microbes develop strategies to ensure their survival and adaptation to the stressful environments. This leads to the formation of a cohesive and strong community of cells that possess intercellular communication, known as biofilm.Citation3,Citation4
Microbial biofilms may be defined as heterogeneous communities (representing species diversity, for instance, a symbiotic association of bacteria and fungi) of aggregated, organized, and functional microbial cells, that remain embedded into the matrix of extracellular polymeric substances (EPS), which allows their irreversible adhesion to biotic or abiotic surfaces.Citation5,Citation6
The EPS matrix is primarily produced by the biofilm constituting microorganisms themselves; the former is especially composed of nucleic acids, extracellular proteins, phospholipids, teichoic acid, and exopolysaccharides. Mineral crystals, silt, milk residues, and blood components or dirt may also be present in EPS matrix, depending upon the conditions and locations in which biofilms are formed.Citation7 Molecular interactions between EPS matrix and its various components and the constituents’ contribution toward the integrity of the matrix are not clearly understood yet. However, several EPS functions have been determined that have proved advantageous for biofilms.Citation8
The formation of biofilms, usually with the thickness of milli- or even micrometer order, naturally occurs around solid surfaces that are in contact with water, such as living tissues, medical devices, and water bodies/systems.Citation9 In the environment and even on the abiotic surfaces, it is very common to find microbial biofilms with other commensal species, which are, therefore, called biofilm multispecies.Citation10 Bacteria and fungi are both capable of forming biofilms on surfaces, but certain factors involved during the course of their formation differ from each other.
Studies conducted by Almeida and FrançaCitation11 and Percival et alCitation12 demonstrated that biofilms are heterogeneous structures, and these consist of discontinuous phases on the support surface; that is, there may be an area with high density of cells along with areas where there may be no colonization. Furthermore, a great diversity in the morphology of these cells has been recorded; microbes may possess different shapes, such as filamentous, spiral, or rod, or they may exist as cocci or bacilli.Citation11,Citation12
At present, the major concern of the medical research scientists working in this field is the adhesion and proliferation of the biofilm-forming microorganisms, inhibition of which may be a successful strategy to combat biofilm formation. Therefore, it is recommended to use stainless steel for manufacturing surgical devices, as this material is resistant to corrosion and fracture. All these measures are imperative owing to the capacity of biofilms to proliferate and get fixed on porous and rough surfaces.Citation13
In general, the development of a biofilm over a biotic or abiotic surface is a dynamic process that involves various steps, viz., adhesion, growth, and production of EPS matrix.Citation4 A cycle indicating the five sequential stages involved in the formation of biofilm is depicted in .
Figure 1 Stages of microbial biofilm formation over a surface.
Notes: The stages include: adherence of microbial cells (1), reversible adhesion (2), irreversible adhesion (3), maturation (4), and detachment of cells (5). The arrows explain the migration of single cells and pieces of biofilm in EPS matrix that are released after the detachment stage, and the capacity to restart the formation process.
Abbreviation: EPS, extracellular polymeric substances.
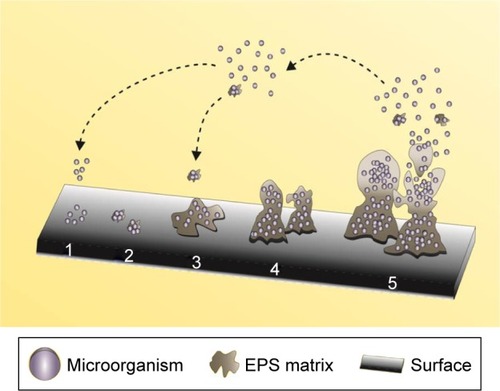
The five sequential stages are described below:
Stage 1: In this step, the deposition of free planktonic cells occurs by the arrival of microbes to the adhesion site. The presence of macromolecules, which form the constraint film as a substrate for the microbial cells, initiates biofilm formation.Citation14
Stage 2: This stage, known as the reversible adhesion phase, marks the beginning of adhesion of microbial cells and the communication process among them that is responsible for the subsequent steps. Although in small quantities, the EPS matrix can be observed in this stage.Citation15
Stage 3: Post initial adhesion to the surface, the cells that still have weak bonds with the surface consolidate the adhesion process by high EPS production that leads to an increase in their reproduction rate. The matrix also acts as a recycling center, as it is known to keep all the available lysed cell components including the DNA; thus, it may be considered as a genetic material reservoir to enable horizontal gene transfer and a source of nutrients, and provides protection against adverse conditions, such as drying, oxidation, exposure to biocides, antibiotics, certain metallic cations, and ultraviolet radiations, and immune responses. The production of the matrix represents the successful formation of biofilm communities, and propagation and survival of the cells in their local environment.Citation16,Citation17
Stage 4: The maturation step or biofilm maintenance represents the dynamics between the microbial cells and the biofilm architecture. In this step, replication of the microorganisms occurs resulting in the generation of intense communication via signaling molecules (quorum sensing, QS). Such a mechanism enables individual cells to communicate and coordinate their actions by the production and detection of extracellular signaling molecules that are called the auto-inducers, which are responsible for the uptake of the substrate into the biofilm. Further, in this step, other microorganisms may also get adhered to form a multispecies biofilm.Citation18
Stage 5: In this stage, the detachment of the inside of the biofilm cells occurs. The high population density causes the release of the packed cells in an array to the external environment. Planktonic cells are released back into the surroundings, and these cells will start the cycle again.Citation17
The actual mechanism behind the biofilm formation is still unknown and will remain a hot topic of scientific research for many years. Nevertheless, it is known that the biofilm composition and the mechanisms involved are related to the resistance and virulence of the microbes.
According to the complexity of diseases associated with microbial biofilms, in this work we propose a structured review about the impact of microbial biofilms in the infectious diseases and present the main drug delivery systems based on nanotechnology as a strategy for the control and treatment of biofilms focusing in a treatment prospection.
Bacterial biofilms: general aspects
In the last 20 years, bacterial infections have posed a considerable threat to human health. The infections may appear as an isolated disease or systemic and primarily occur in hospital environments.Citation19
Among all the microorganisms, the bacterial species are the major biofilm producers, provided that the conditions are favorable, although some may have a greater ability than others. Most of the species demonstrate elevated growth rate, great adaptability, and competence for the production of substances and extracellular structures that protect the microbes in their habitat, and as per the characteristics, make these capable of perfectly colonizing any type of surface, even in adverse conditions.Citation20
Bacteria show two survival states, namely the planktonic form (individual/free cells) and the population aggregates (biofilms). The bacterial development in the planktonic form is an important phenomenon for the propagation of biofilms. Nevertheless, the survival of a biofilm as a defense mechanism is related to its sustenance, which is dependent upon its continued life cycle, if the biofilm offers safety against adverse environmental extrinsic factors.Citation21,Citation22
There are certain advantages of bacterial biofilms, for example, the ecological participation in symbiotic relationships. The examples of this phenomenon are abundant in nature, and those exhibiting such relationships include diazotrophic prokaryotic bacteria that colonize vegetable roots and several other bacteria found in the digestive tract of the ruminants, where they promote the degradation and recycling of insoluble materials.Citation21
During the entire process of bacterial biofilm formation, there are complex factors involved, which have been poorly understood and have inspired the scientific community to analyze the whole dynamics of the complex microbial architecture. The contact of bacteria with biotic and abiotic surfaces is the first stage of biofilm formation, which is considered an important and complex process.Citation23
Considering only the abiotic surfaces, the initial attraction of planktonic bacterial cells to the surface seems to occur randomly by Brownian movement and gravitational force or in a guided way via chemotaxis and motility.Citation24
Motility is one of the extremely important characteristics since studies have shown that flagellated bacteria or the bacteria that have greater locomotion capacity form more complex and structured biofilms. Besides, the facility to migrate to other places gets increased.Citation25
Bacteria promote a variety of adhesion strategies to remain in contact with the fixation surface; after that, the microbes promote the release of EPS mixture, which increases their affinity for different types of surfaces, for example, porous, rough, and chemically heterogeneous surfaces.Citation8
The reversible adhesion occurs by nonspecific physicochemical interactions between the bacteria and the material, including the hydrodynamic forces, electrostatic interactions, van der Waals forces, and hydrophobic interactions.Citation21,Citation26 In addition, the bacteria make use of some of their proteinaceous structures, for example, pilli and fimbriae, in order to enhance the adhesion on surfaces. Besides, bacteria also have mechanisms to overcome the repulsive forces between the cell membrane and abiotic surfaces, especially in the presence of a conditioning film, which can easily be found in biomedical devices.Citation8,Citation27,Citation28
The composition of a conditioning film or an organic film is variable and depends upon the site of biofilm formation. Nevertheless, its basic constitution includes proteins, such as albumin, immunoglobulin, fibrinogen, and fibronectin.Citation29 Therefore, the film acts as a substrate for initial establishment of the biofilm. When adequate proximity and other predisposing factors are reached for appropriate elongation of biofilm formation, the bacterial cells promote enhanced production, release, and detection of self-inducing signaling molecules that regulate the biofilm formation.Citation30,Citation31
As the adhesion process progresses, the accumulation of such signaling molecules results in the induction and transcription of specific genes, which regulate various bacterial functions, such as motility, virulence, and production of the matrix containing EPS, and consequently, exacerbate the development of biofilm (formation of stronger structures with dynamic complexity). The generation of EPS matrix facilitates colonization by other species as well. It is presumed that within a multispecies biofilm, the EPS increase the stability of the other species by mediating interactions between the polymers of different species.Citation32
After the completion of reversible adhesion step, the irreversible adhesion phase occurs, which is due to the bacteria that still have weak interactions with the surface but manage to stick to the substratum due to the high production of EPS and cell signaling-based communication.Citation33
The cell-to-cell communication process, referred to as QS, is found in several pathogenic bacteria which offers benefits such as the ability of sporulation, expression of virulence genes, DNA transfer, biofilm formation, and even antibiotic production. The molecular events in bacteria are partially controlled by QS via chemical signals, and such a mechanism of intracellular communication is dependent upon the population density within a biofilm.Citation34
In bacterial biofilms, QS is a common phenomenon that favors the access to nutrients or more favorable environmental sites, thus allowing bacteria to induce defense responses against eukaryotic hosts while optimizing their ability to differentiate into most appropriate forms for their sustenance and survival in harsh environments.
The chronic bacterial infections are mainly related to the total biofilm formation cycle. Some of the major bacterial species, which are capable of triggering an infection in internal human organs, are Pseudomonas aeruginosa, the main causal agent of pneumonia and cystic fibrosis (CF),Citation35 Escherichia coli, the causative microbe of the urinary tract infections (UTIs),Citation36 and Mycobacterium tuberculosis, which causes human tuberculosis.Citation37
In dental infections caused by Streptococcus mutans (dental caries), the bacteria overcome the mechanical cleaning and antimicrobial treatmentsCitation38 by forming biofilms. Since the amount of colonized bacteria in the oral cavity is high and the renewal of biofilm mass is easily achieved, S. mutans, very peacefully, acquires resistance against the drugs.
The implantation of medical devices, such as intravenous catheters, prosthetic heart valves, joint prosthetics, peritoneal dialysis catheters, heart pacemakers, and endotracheal tubes, is a viable alternative that ensures the maintenance of a patient’s life. However, the presence of bacterial biofilms on such medical devices has been identified as the foremost cause of clinical infections. The reason is the ease of formation of biofilms due to the inflammatory responses generated by the host, which further allow bacteria to adhere to the surfaces of the devices.Citation23,Citation39 The Gram-positive species, such as Staphylococcus epidermidis and Staphylococcus aureus, colonize and form biofilms on medical devices, followed by Gram-negative species, like P. aeruginosa.Citation40
The presence of biofilms on the cardiovascular electronic devices (CEDs) is very commonly witnessed in medical routine, which poses considerable risk to host’s health leading to a compromised immune system.Citation41 The main bacterial species found in biofilms that are adhered to the CED surface belong to the genus Staphylococcus, for example, S. epidermidis and S. aureus, and these correspond to about 70% of the total infections.Citation41
Although S. aureus is the major Gram-positive bacteria that forms biofilms on CED,Citation42 the other species with same characteristics may also be related to the infections. A study by Madhavan et alCitation43 evaluated the presence of Gram-positive cocci with a coagulase-negative profile in the blood samples of 74 patients, who were having CED implants and sequentially developed bacteremia. The results demonstrated a high prevalence of Gram-positive cocci, S. aureus, among the infectious cases.
Infectious endocarditis (IE) is classified as a major disease that originates from the incidence of biofilms, primarily formed by S. aureus and P. aeruginosa. The establishment of such infection is directly related to the ability of the microorganisms to colonize in normal or abnormal valves or altered endothelial surfaces in the heart due to contamination of bloodstream of the host.Citation44
As per the published reports, the episodes of IE caused due to the contamination by P. aeruginosa are rare in comparison to those caused by Staphylococcus spp., although to a lesser extent. However, the IE caused by the former tends to be more aggressive and is associated with a higher mortality rate than the latter.Citation45 In contrast, the presence of biofilms formed by P. aeruginosa is widely observed in cases of CF.Citation46
Even though lifelong drug therapy is continued for patients, a high proportion of CF cases shows respiratory failure due to the chronic bacterial infection caused by the biofilm-induced inflammation of lungs.Citation40,Citation47
Chronic wounds (CWs) that behave as biofilm reservoirs are of major concern due to the risk of development of systemic infections. A CW is often colonized by a wide range of bacterial species that includes S. aureus, P. aeruginosa, Enterococcus faecalis, coagulase-negative Staphylococci, Proteus spp., and anaerobic bacteria.Citation48 S. aureus has been considered to be the main agent of biofilm formation in CWs; however, there are reports that define the role of other bacteria, such as P. aeruginosa, as well in CW. The infection caused by the latter reportedly reaches even the deeper layers of cells, thus aggravating the infection.Citation49
The bacterial infections of female reproductive systems, such as bacterial vaginosis, are characterized by the disturbance of the vaginal microbiota. Lactobacilli that are normally commensal inhabitants of vagina are replaced due to significant increase in the concentration of a diverse set of bacteria, like Gardnerella vaginalis, Chlamydia trachomatis, and the bacterial species belonging to the genus Mobiluncus,Citation50 which are capable of developing biofilms in the female genital tract, thereby triggering chronic infections for which effective therapies are not available.Citation51
Biofilms have also been reported in the auditory tracts causing infections, such as otitis media, which is characterized as a middle-ear infection and occurs especially in children. However, in certain cases of biofilms, particularly those formed by S. aureus, the infection may persist or reemerge frequently, and so such infections have been characterized as chronic otitis media (COM). Furthermore, in otolaryngology, the presence of biofilms in the ear canal has been associated with chronic sinusitis and COM with effusion.Citation52
Gastric infections are still of great concern in gastroenterology, and particularly, oncology, owing to the association of Gram-negative bacteria Helicobacter pylori. This bacterium forms biofilm in order to ensure its integrity and survivalCitation53 and is frequently involved in chronic gastritis, functional dyspepsia, peptic or duodenal ulcer, and gastric cancer or lymphomas. Further, H. pylori also displays a survival profile in acidic environments; it remains intact in the stomach environment and promotes the destruction of the gastric mucosa making the organ sensitive and vulnerable to ulcerative lesions. In addition, it also blocks the sterilization process of food, thus interfering in the digestion process.Citation54 The currently available therapy to eradicate this bacterium is complex and costly and presents severe side effects for the patients.Citation55
Bacterial prostatitis is a UTI that affects males of all ages. A wide spectrum of bacterial species is involved in this disease; the acute phase exhibits the presence of E. coli (67%), P. aeruginosa (13%), Klebsiella spp. (6%), Gram-positive species (5%), and others (9%).Citation56 In the chronic phase, the major aggravating factor is the presence of biofilms mainly of Gram-negative species, such as E. coli,Citation57 which is responsible for most cases of chronic UTIs (E. coli uropathogenic), thus causing about 40% of the total hospital infections. Moreover, E. coli has been directly related to biofilm formation in urinary catheters, since direct contact of the bacterial cells with the urine can carry infection to internal organs which, in turn, may trigger local and even systemic infections.Citation58
Thus, to devise novel therapeutic strategies, the scientific community has shifted focus to nanotechnologyCitation59–Citation61 for the effective prevention of biofilm formation.Citation62 In view of this, the present review draws the attention of the readers to the factors involved in adhesion of bacterial cells leading up to the establishment of biofilms.Citation18,Citation63
Fungal biofilms: general aspects
Fungal infections are a major issue for clinicians because of their high rate of incidence, especially in the immunocompromised patients. The risk factors that determine acquisition and high prevalence of fungal infections are host immunity, prolonged use of broad-spectrum antibiotics, long-term use of intravascular and urethral catheters, hemodialysis, treatment with corticosteroids, parenteral nutrition, the use of immunosuppressive anticancer drugs, and transplants among others.Citation64,Citation65
Fungi can dwell as biofilms in different body niches and subsequently induce infections. The site of infection depends on several factors, such as the amount and type of the available nutrients, host immune response, flow conditions and pH at the infection site, and substrate for cell adhesion and growth of the biofilm.Citation66
The substrate for adhesion may be considered as a major factor for the formation of fungal biofilms. The materials that prevent adhesion of microorganisms with satisfactory in vitro results are used to manufacture medical devices. However, in the case of in vivo application of such models, several precautions, for example, a sepsis, need to be taken by the medical team, as contact of the device with the host body fluids, such as urine, saliva, and blood nutrients, provides favorable conditions for the formation of biofilms.Citation67,Citation68
The flow conditions may also play an important role in the development of fungal biofilms; for instance, the majority of the Candida spp. form biofilms at different flow rates of body fluids, which can be low (salivary flow: prosthetic stomatitis), intermittent (urinary catheters and vascular circulation), and rapid (bloodstream: fungal endocarditis).Citation69,Citation70 Furthermore, the flow is directly related to the transport of oxygen and nutrients that are essential for the development of biofilms.
The nutritional composition of different body niches varies; thus, some species develop better at some places compared to others; for example, blood is rich in nutrients, sugars, and proteins, so the biofilm development is high, which is further facilitated by high vascularization.Citation66
The yeast species Candida albicans is known to frequently form biofilms and has been extensively studied by medical researchers, as it is the third most common cause of intravascular infections (catheters) among the other types.Citation68,Citation71 However, in recent years, several other biofilm-forming species of the genus Candida, Candida tropicalis, Candida krusei, Candida glabrata, and Candida parapsilosis, that affect human body have been identified.Citation72,Citation73 Further, some other types of fungi, such as Malassezia spp.,Citation74 Pneumocystis spp.,Citation75 Histoplasma capsulatum,Citation76 Cryptococcus neoformans,Citation77 and Cryptococcus gatti,Citation78 may also be associated with the infections in humans.
Yeast and the cells of filamentous fungi may naturally occur as two different phenotypes, that is, the planktonic form (free cells) or the sessile form (biofilms). According to Ramage et al,Citation79 the sessile phenotype involves the development of a group of strains on a polymeric matrix that is rich in water and allows passage of nutrients and oxygen. In addition, the polymeric matrix confers protection against the host immune response and prevents diffusion of antimicrobial drugs. Moreover, since fungi are eukaryotes and more complex than bacteria, the infections caused due to fungal biofilms remain difficult to diagnose and treat. The detailed analysis of such fungal biofilms has been done during the last years.Citation80,Citation81
Since long, the sequences of fungal biofilm formation were thought to be same as in the case of bacteria. However, some studies conducted on the dynamics of genetics and the interactions of fungi, host, and environment have changed this scenario. Even though the overall process is the same, that is, primary adhesion, irreversible adhesion, maturation, and dispersion, depending on the characteristics of fungi, the dynamics involved is different.
The genus Candida has hyphae or pseudohyphae that are associated with the proliferation and development of the biofilms.Citation65 The hyphal formation is critical for tissue invasion, as it is more resistant to phagocytosis in comparison to yeast. The virulence of C. albicans has been closely linked to the hyphae-forming ability.Citation82 The hyphae of C. tropicalis are similar in morphology to C. albicans and are related to the invasion of oral epithelium. However, the ability of C. parapsilosis to invade oral epithelium is not related to the production of pseudohyphae.Citation83 The hyphae promote the ability of the fungus to evade the host defense responses and thus serve as an essential factor for pathogenicity to form biofilms.Citation84
The models for the formation of fungal biofilms have been described by DouglasCitation69 and Harding et alCitation85 that can be studied to understand the characteristics of fungal biofilms as well. The authors, in both the studies, used C. albicans as the model organism and reported that although the biofilm formed by the fungal species has several similarities with the bacterial biofilm, the presence of hyphae and pseudohyphae is the primary difference between the two. The proposed model includes five steps: (i) adsorption of yeast cells on a surface (biotic or abiotic), (ii) adhesion to the surface, (iii) formation of microcolonies (this step occurs after the initial growth and development of hyphae followed by the formation of microcolonies in the upper layer that predominantly comprises hyphae and production of EPS matrix), (iv) maturation of biofilm, and (v) dispersion of the mature biofilm cells and restart of the cycle.
A preliminary model for the sequential development of filamentous fungi has been proposed and consists of six steps: (i) adsorption of fungal propagules, such as spores, conidia, or hyphae, on solid surfaces (the authors compare this step with reversible adhesion stage of the bacterial biofilms); (ii) promotion of adherence and attachment by release of adhesive substances by germinating spores; (iii) stage 1 of formation of microcolonies (involves apical elongation and branching of hyphae during early growth and colonization of the surface with concomitantly increased production of EPS, which enables colony growth and adhesion to the substrate); (iv) stage 2 of formation of microcolonies or beginning of maturation, which involves the formation of compact hyphal network or mycelia and the formation of water channels that enable the passage of nutrients; (v) maturation phase, which is characterized by high production of fruiting bodies, spores, and other survival structures (this is an extremely important stage since aerial growth is a predisposing factor that allows the dispersion of yeast for new colonization); and (vi) dispersion or planktonic phase, which is similar to the dispersion step in the case of bacterial biofilms, the only difference being the release of spores and fungal filaments that act as propagules to initiate the next cycle.
During the formation of fungal biofilms, QS plays a significant role in the communication between the fungal cells. QS allows the development of the cooperative relationship between the cells that leads to the coordination of different cell behaviors and secretion of signaling molecules.Citation86
However, there are certain signaling molecules involved in QS of fungal biofilms that need a special reference. A pioneering study by Hornby et alCitation87 demonstrated farnesol as the QS molecule that is responsible for inhibition of hyphae formation of C. albicans in the stationary phase. The study was important to understand the dynamics of C. albicans biofilms and inspired other researchers for further analysis.Citation88–Citation97
Chen et alCitation93 identified tyrosol to be a molecule that is involved in QS of C. albicans; thus, these two molecules (farnesol and tyrosol) became well-known regulators of QS in the fungal species.Citation98
The control of fungal biofilms has been studied in the context of various fields, such as biotechnology, mycology, and medical research. The infection is complex and results in a poor response to a therapy. The knowledge of the mechanisms that are related to the dynamics involved in the architecture of biofilms is still insufficient.
Routinely, in hospitals, it is common to observe the development of fungal biofilms on the surfaces of medical devices, such as artificial valves, pacemakers, defibrillators, endotracheal tubes, dialysis devices, prosthetic joints, catheters, urinary/intrauterine devices, and contact lenses, which is classified as extreme risk to the patient’s health.Citation94
Since long, C. albicans and Aspergillus fumigatus have been considered as prevalent fungi in infectious cases. However, in recent years, other fungal species have also been observed to cause infection in clinical practice.Citation64 Candida spp. are often found in normal human biota which facilitates their interaction with the implanted biomaterials and surfaces of the host. The treatment of infections that are caused by the fungal biofilms can be inefficient, as the infection often recurs when the therapy ends, and thus, to overcome it, the removal of the device becomes necessary.Citation95
Invasive candidiasis (IC), especially candidemias (blood infection caused by Candida spp.), is directly related to the presence of biofilms in body niches and abiotic surfaces of medical devices.Citation96 Nowadays, candidemias have been reported as the fourth most common blood infections affecting more than 0.25 million people each year and are responsible for about 50,000 deaths. According to population-based studies, candidemias occupy the seventh position in the ranking of ten most prevalent infections of the human blood that may be fatal.Citation97
The prevalence of IC in hospitals is still a major concern, and the presence of biofilms makes this infection yet more complicated. A recent observational survey conducted over 16 years by Caggiano et alCitation99 demonstrated that the infection by Candida spp. was responsible for triggering heart failure in the patients of different age groups, who visited the Southern Hospital in Italy. The authors concluded that C. albicans infection was the most prevalent among all the hospital departments. Moreover, the researchers identified that the incidence of IC caused by C. albicans has exponentially elevated over the years and the non-albicans cases have increased by 75%.
Although C. albicans is the prevalent species among the cases of candidemias, the presence of other species has been observed in clinical settings causing concern, as certain species, such as C. krusei, show high levels of resistance, which poses difficulty in the effectiveness of treatment.Citation83 Candidemias are very common in neonates. Recently, Rongpharpi et alCitation100 reported seven episodes of IC that were caused by C. krusei in neonates, and extreme measures were taken to prevent the subsequent nosocomial infections.
In a recent case, D’Acunto et alCitation10 identified C. parapsilosis to be responsible for generalized IC in two neonates. The authors reported the use of drugs, fluconazole and amphotericin B, for control of the infection, but with no success. Therefore, voriconazole was used to treat the patients. However, voriconazole is classified as a drug of choice in the cases of systemic fungal infections that are caused by the species of the genus Aspergillus.Citation101 Furthermore, the drug exhibits several side effects, such as fever, gastrointestinal symptoms, reversible visual disorders, hepatitis, jaundice, and skin reactions.
The ability of Candida spp. to develop and consequently form biofilms on mucous membranes of the human body is associated with various types of diseases, for example, vulvovaginal candidiasis (VVC). This disease is the most common cause of yeast infection in women with 80% of the cases caused by C. albicans.Citation102 However, in 20% of the cases, other non-albicans species may be involved in the infectious processes.Citation83 Moreover, the episodes of recurrent VVC are the most common cause of morbidity in women and predispose the development of cervical cancer.Citation102,Citation103 A major problem with fungal dissemination through VVC is the use of intrauterine contraceptive devices as the yeast can adhere to their abiotic surface by increasing the density of biofilms.Citation104
The respiratory tract is a perfect habitat for the formation of fungal biofilms. Respiratory fungal infections are chiefly caused by filamentous fungi. Invasive pulmonary aspergillosis (IPA) caused by airborne opportunistic fungi belonging to the Aspergillus spp. (especially A. fumigatus) is one example. IPA is characterized as severe pneumonia leading to the life-threatening invasion of the lung parenchyma and involving erosion in the vasculature leading to necrosis.Citation105
Intensive care unit (ICU) patients, especially those with chronic obstructive pulmonary disease, are at a risk of developing nosocomial IPA. Other species of Aspergillus, viz., Aspergillus flavus, Aspergillus niger, Aspergillus terreus, and Aspergillus nidulans, are also known to trigger respiratory tract diseases in humans.Citation106 A. fumigatus may also be associated with the cases of CF, although at a less frequency as compared to P. aeruginosa cases.Citation107
Another fungal respiratory disease, which is acquired by inhalation and has a high level of morbidity, is the histoplasmosis. It is one such respiratory fungal infection that accounts for about half a million cases per year only in the US.Citation108 The ability of H. capsulatum to form biofilms remained unknown for a long time until identified by Suárez-Alvarez et al.Citation109 The researchers showed that H. capsulatum yeasts could adhere to the cryosections of different organs and further provided evidence for an important mechanism of colonization and spread of the yeasts, which involves adhesion of the microorganisms to the host tissues, including the cell surface and extracellular components. Two years later, Pitangui et alCitation110 demonstrated the in vitro ability of H. capsulatum to form biofilms and evaluated its invasion potential in cell lines.
The species of the genus Pneumocystis have been known as the major cause of infections in immunocompromised individuals, especially those who have HIV infection, as it is the major cause of lethal pneumonia in such cases.Citation111 The main species associated with the development of pneumonia in humans are Pneumocystis jirovecii and Pneumocystis carinii,Citation112 and the diseases caused by these generally involve the formation of biofilms in host’s lungs as the latter are suitable for reproduction provided the immunodeficient state of the patient.
Cryptococcosis is an opportunistic fungal disease that affects individuals from different age groups and is induced by Cryptococcus, especially C. neoformans and C. gatti.Citation113 Both the species are responsible for triggering infectious processes, such as pneumonia, meningitis, and meningo-encephalitis, which often cause the death of the affected patients, especially the transplant recipients and the immu-nocompromised ones.Citation114 The progress of infection by these species involves the formation of biofilms in organs, such as the lungs and brain, that further complicates the therapy. The fungus possesses a polysaccharide capsule that completely surrounds it for the protection against phagocytosis by the cells of the immune system and has a direct relationship with the formation of biofilms.Citation77 Fungal infections that are caused by Trichosporon spp. are also considered as a serious threat to humans as their diagnosis and therapy are also complex.Citation115 Since long, the species of this genus have been detected in the cases of skin surface mycoses, such as “piedra”, which is white, and hypersensitivity pneumonitis. However, in recent decades, the medical field has associated the presence of Trichosporon spp. with the cases of systemic infections, especially in immunocompromised patients, such as those who are neutropenic and those who use central venous catheters.Citation116
The potential of certain uncommon Trichosporon spp. to form biofilms, mainly on intravenous catheters, is an issue that needs to be addressed soon. A study performed by de Pavia Fagundes Júnior et alCitation117 demonstrated emerging cases of systemic infections that were triggered by Trichosporon asahii in ICU patients with heart failure. The species belonging to Trichosporon genus have a significant adaptation as recently reported by Mattede et alCitation118 who confirmed the presence of these species in urinary catheters causing UTIs in ICU patients.
Major factors leading to the development of antimicrobial resistance to biofilms
Due to the genetic and structural dynamics of microbial biofilms, the treatment and eradication of the same seem to be complex. Certain resistance mechanisms that have been attributed to the sessile cells provide favorable conditions for survival and reproduction of microbes, making them less susceptible to elimination in contrast to the planktonic forms of the same microorganisms.Citation79 The incidence of infections caused by microbial biofilms is a problem for the entire health care system and our society. Thus, efforts need to be continued in the pharmaceutical industry to develop novel products for the treatment of drug-resistant infections as there are only limited number of agents that are effective against biofilms.Citation119
Besides, the planktonic-form microbes employ mechanisms of resistance, such as the transfer of resistance genes by QS processes, the production of specific enzymes, and the evolution of natural mutations, and certain other processes explain the increased resistance by biofilms against the antimicrobial agents presently used in clinical practice.Citation120
The sessile state of microorganisms is crucial for the formation of biofilms. The success of an antibiotic treatment against an infection depends on the drug concentration at the site of infection which must be high enough to inhibit the proliferation of pathogenic microorganisms. Thus, the antibiotics must reach their target sites, in their active forms, in order to promote efficient binding to the target and finally interfere with the target function. In bacterial biofilms, the bacteria involved in the biofilm have high resistance to the antimicrobial agents that are effective against cells of the same species in planktonic form. The minimum inhibitory concentration (MIC) for the bacteria that are in biofilms may be 10–1,000 times greater than that for their planktonic form.Citation121
The main and most studied component of biofilms is the EPS, which has been classified as a physical and chemical barrier for the prevention of the action of antibiotics and protection against attack from the host immune response. In addition, EPS matrix provides resistance to thermal stress and mechanical strength, and limits the spread of sanitizers.Citation8 The EPS matrix can react with sanitizers and promote inactivation as it is already known that certain chemical sanitizers (sodium hypochlorite) may have their action reduced or even eliminated in the presence of organic compounds, such as proteins, polysaccharides, and lipids.Citation122
The microorganisms that exist as biofilms, particularly those dwelling in the inner layers, have low rates of metabolism and growth, and the EPS matrix acts as an adsorber that reduces the amount of drug available for the interaction with the biofilm microbes. In other words, the EPS matrix is capable of reducing the physical penetration of the antimicrobial agents.Citation123 It can be understood in this way that the EPS matrix has pores that are very small and do not allow the larger drug molecules to pass through. Moreover, antibiotics may be adsorbed on the matrix itself owing to its hydrophilic and anionic nature, and an adequate amount of the antimicrobial drug does not reach the host tissues, which renders the therapy ineffective.Citation124,Citation125
Another aspect to consider is that the cells in the inner part of a biofilm often lack oxygen and nutrients, which forces the microbes to enter the stationary (or dormant) phase of growth. Thus, the microorganisms in the inner layers of biofilms become less susceptible to the action of chemotherapeutic agents since these agents require active growth of microbes.Citation126 In this regard, it has been noted that within a biofilm, there are two microbial subpopulations with different phenotypes, one being active and the other inactive, metabolically. The former is on the surface and in contact with the external environment, and thus susceptible to antibiotics, while the latter is submerged in the EPS matrix within the biofilm with a slow rate of cell division, and thus remains resistant to the drugs.Citation127,Citation128
According to Mah,Citation129 a biofilm can acquire antibiotic resistance by natural mutation or acquisition of resistance genes (for conjugation, transformation, or transduction) as well. These genes may alter the expression of antibiotic-modifying enzymes leading to the production of modified proteins that may be the targets of antibiotics. Another phenomenon that may lead to the development of resistance to antibiotics and allow sustenance of cells even during the exposure to chemotherapeutic agents is the induction of reversible phenotypic state, primarily, due to the action of the efflux pumps. The expression of efflux pumps is mainly based on the expulsion of antimicrobial agents from the cells, which has been associated with increased resistance by microbial cells in biofilms, especially the Gram-negative bacteria.Citation130 The system AcrAB-TolC, belonging to the family RND, is well characterized in E. coli and confers resistance to antibiotics, such as chloramphenicol, tetracyclines, and quinolones. However, these positively regulated genes are observed in the biofilm-forming cells; additionally, this mechanism may also be found in the strains of Salmonella typhimurium.Citation131 In P. aeruginosa, the pumps, MexAB-OprM and MexCD-OprJ, appear to be involved in the resistance to macrolides and are directly related to the formation of biofilms.Citation132,Citation133
The efflux pump mechanism is also observed in yeasts, such as C. albicans. The species has two types of efflux pumps: the first one is known as the ATP-binding cassetteCitation134 and the second type is the multidrug resistant (MDR).Citation133 The genes that encode the pumps are upregulated during the formation and maturation of biofilms. Thus, it has been recorded that during the development of biofilms of this fungus, the tolerance to fluconazole is quickly acquired and is mainly attributed to the expression of genes CDR1, CDR2, and MDR1.Citation135
Since biofilms represent the mixture of different types of microorganisms living as one community, it is likely that the resistance to multiple drugs may also occur. Not only the planktonic cells but the sessile microbes in biofilms also display specific resistance to antimicrobial agents. However, it is speculated that the classical individual mechanisms provide additional resistance to plaques, thus contributing to the overall resistance.Citation129
The currently available drugs have limitations, such as the inability to reach the biofilm microbes and even inefficiency in the cases of strains with a resistance profile. Novel alternatives have been applied for the control and eradication of biofilms. Thus, the present article intends to highlight the use of nanotechnology in drug delivery systems, which aims to enhance the pharmacological action of the bioactive compounds.
Nanotechnology
The term nanotechnology is related to the development and use of the systems and materials at the nanoscale size (1–1,000 nm) and the application of the concepts of manipulating materials at atomic, molecular, and macromolecular levels. The advantages of nanotechnology are mainly due to the unique characteristics of the materials at the nanoscale, which usually differ from the macrolevel characteristics of the materials. The change in the properties of materials is primarily due to the high surface area-to-volume ratio causing them to be highly reactive, affecting their mechanical and electrical properties. Thus, at the nanoscale, quantum effects dominate the behavior of the materials leading to interesting changes in their electromagnetic and optical properties.Citation136
Nanotechnology has played important roles in various fields of science and contributed to the progress in physics, chemistry, engineering, medicine, and pharmaceutical industries. Owing to the ability to control mechanical, magnetic, optical, and catalytic properties of materials, nanotechnology has provided numerous benefits to all areas of science. Nanotechnology has substantially contributed to various fields, such as chemical synthesis, energy supply, food production, data storage, and biotechnology, to name a few. In medicine, nanotechnology gained importance due to its applications in the prevention, diagnostics, and treatment of various diseases.Citation137
If one thinks of the disadvantages, the problem that is mostly encountered in the area of nanotechnology is the limited size of these materials, as these can penetrate any living system. Besides, enough investigations have not yet been conducted to allow commenting on the duration for which these materials may remain in the environment or the long-term consequences, which may entail accumulation of such materials in the ecological and living systems. Therefore, short-, medium-, and long-term effects of these nanomaterials are yet to be understood completely.Citation138
Ranging from the combination of materials or devices with drugs and biomolecules, adding benefits like slow and controlled drug release, promoting greater efficiency of tissue penetration to greater protection against drug degradation, nanotechnology can be used to prevent, monitor, control, and cure diseases in several ways.Citation137 At present, the main types of nanosystems used for the delivery of bioactive substances are liposomes (LIPs), microemulsions (MEs), nanoemulsions, cyclodextrins (CDs), solid lipid nanoparticles (SLNs), polymeric nanoparticles (PNs), and metallic nanoparticles (MNPs). The nanostructured systems have become promising tools for the treatment of infectious diseases, which are resistant to conventional treatments or persistent, improving the quality and expectancy of life of patients who suffer from such diseases.Citation139
Due to the resistance mechanisms and biofilm formation, which these microorganisms eventually develop, the effectiveness of conventional antimicrobial agents is gradually declining. A promising strategy to overcome bacterial resistance is nanotechnology, which makes use of nanocarriers for delivery of drugs and biomolecules for the prevention and treatment of bacterial biofilms.Citation140
The applicability of these systems in the treatment of biofilms is variable; however, nanotechnology-based drug delivery systems can facilitate drugs to directly interact with the complex structure of biofilms and exert action during the different stages of the biofilm formation.
Among the main abilities of these systems, two are predominantly studied by researchers, the direct interaction with planktonic cells (single cells) and the interaction with or denaturation of the EPS matrix. schematizes the interaction of a drug delivery system containing nanoparticles for drug release and their interaction in the biofilm formation stages.Citation141
Figure 2 Interactions of nanoparticles based in drug delivery system on biofilm formation process.
Notes: Interaction of nanoparticles based in drug delivery systems in different stages of biofilm formation (A): adherence of microbial cells (1), reversible adhesion (2), irreversible adhesion (3), maturation (4), and detachment of cells (5). Nanoparticles interaction with single cells (B) and EPS matrix (C).
Abbreviation: EPS, extracellular polymeric substances.
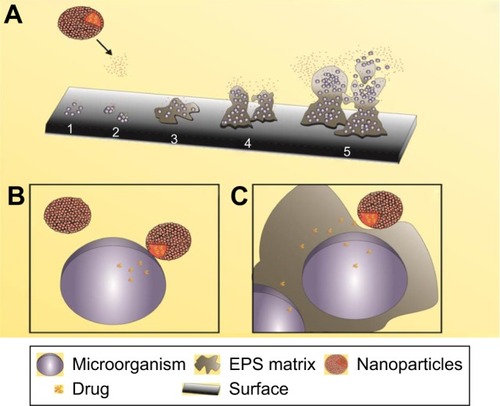
The direct interaction of nanoparticles with individual cells () that are involved in the initial event of the process of biofilm formation (primary adhesion) may prevent the continuous process of formation, since the direct interaction between the nanoparticles and membranes of the microorganisms (eg, lipid nanoparticles, LIPs, and others) facilitates entry of the drug into the intracellular medium. This type of interaction can also be attributed during the final event (dispersion), where individual cells are detached from the polymer matrix making them able to restart the formation cycle.
The interaction of nanostructured systems such as nanomulsions LIPs, SLNs, lipoproteins and micelles, and others can also exert direct action on the biofilm polymer matrix (), promoting the fusion of the nanoparticles and provoking the denaturation of the proteins and the fusion of the lipid bilayers, facilitating the entry of the nanoparticles inside the biofilm to promote contact with the microbial cells.Citation142,Citation143
Liposomes
Since the delivery of aqueous drugs through biological membranes is considered a challenge, it was devised to develop a system of similar nature to deliver such drugs. Some delivery systems, such as LIPs, were analyzed first to solve this problem.Citation144 LIPs may be described as small spherical vesicles composed of a phospholipid bilayer or sphingolipids in the membrane that are used to deliver desired drugs at the target sites in the body. presents the structure of LIPs and the difference in comparison with a micelle.
These bilayer molecules can be made from cholesterol (CHOL) or other nontoxic phospholipids, and depending upon their composition, the properties, such as rigidity or fluidity and the charge of the bilayer, can be regulated.Citation145
According to Al-Jamal et al,Citation144 LIPs are established nanometric systems that can be used to deliver antifungals, cytotoxic drugs, vaccines, and imaging agents. In addition, LIPs offer other advantages, such as biodegradability and biocompatibility, lower toxicity and doses, and the capacity of loading lipophilic and hydrophilic drugs, all of which make them more efficient than the other nanosystems. On the contrary, LIPs also exhibit certain limitations such as poor solubility, short half-life, and the possibility of oxidation and hydrolysis of phospholipids, besides their high costs.Citation145
Ahmed et alCitation146 investigated the use of PEGylated LIPs to improve the interaction of drug with the bacterial biofilms. The authors prepared LIPs that comprised lipid mixtures by vesicle extrusion method and evaluated if their adsorption to the biofilms of S. aureus would improve after changing the concentrations of lipid and phospholipid-grafted poly(ethylene)glycol (PEG). The results demonstrated the “stealth” property of PEGylated LIPs that also applies to bacterial biofilms, which showed a considerable reduction.
Robinson et alCitation147 investigated the difference between cationic and anionic LIPs, which were developed by the extrusion method, in order to deliver hydrophobic bactericide triclosan (TCS) to bacterial biofilms. Cationic LIPs with varying concentrations of dimyristoylphosphatidylcholine (DMPC), CHOL, and dimethyldioctadecylammonium bromide (DDAB) were tested against various bacteria, viz., Streptococcus oralis, Streptococcus sanguis C104, Streptococcus gordonii, Streptococcus salivarius DBD, and S. salivarius 8618. Among all, the best cationic LIP absorption was displayed by S. salivaris DBD, and the least absorption was displayed by S. sanguis C104. For the anionic LIPs, which were composed of DMPC and phosphatidylinositol (PI), the best results were observed against S. sanguis C104, while the strategy did not work against S. salivarius biofilm. The findings demonstrated the significance of electrostatic interaction for delivery of TCS, as the use of LIP formulations had a substantial effect on the mixed species of the biofilms.
Catuogno and JonesCitation148 studied the antibacterial property of the solid-supported LIPs that were produced by zinc citrate particles against the biofilms formed by the most common oral bacterium, S. oralis. The authors tested the capacity of anionic (PI and 1,2-dipalmitoyl-sn-glycero-3-phosphocholine [DPPC], 4–19 mol% PI) and cationic (DDAB, DPPC, and CHOL, 4–19 mol% DDAB) LIPs that would be able to work as carriers for drugs, such as TCS, a lipid-soluble agent, and the aqueous-soluble penicillin-G. The results showed that the adsorption of LIPs to the bacterial biofilms was dependent on the concentrations of PI and DDAB used; however, an improvement in the antimicrobial efficacy was observed, as demonstrated by the better stability of the drug-loaded LIPs. The balance between attractive hydroxyl interactions and repulsive electrostatic forces that are associated with hydrogen bonding could explain the anionic LIP–biofilm interactions, while the cationic ones may be attributed to the electrostatic forces of attraction, and hence the content of positively charged phospholipids.
Drulis-Kawa et alCitation149 developed anionic and cationic LIPs that encapsulated meropenem (MER) and compared the differences of interaction to P. aeruginosa biofilms. The authors revealed that the cationic LIPs, especially the ones that were composed of phosphatidylcholine (PC), 1,2-dioleoyloxy-3-trimethylammonium-propane (DOTAP), and CHOL (at 5:2:3 mol/mol/mol ratio) or PC, 1,2-dioleoyl-sn-glycero-3-phosphoethanolamine (DOPE), and stearylamine (SA) (at 4:4:2 mol/mol/mol ratio), had higher efficacy than the anionic ones, which may probably be explained by their ionic interactions with the bacterial cell envelope. It is also important, here, to highlight that these formulations showed MIC values that were less than the usual ones, besides demonstrating improved stability and diminished drug leakage. In order to investigate more deeply the reason why cationic LIPs presented a better interaction, further studies were conducted using 23 ATCC and clinical strains of P. aeruginosa that displayed varying susceptibility to certain antibiotics. The results demonstrated a high interaction of nanoparticles with the bacterial cells, specifically of the cationic LIPs that can be explained by the negatively charged external membrane of the microbes with the positively charged LIPs. In addition, some hydrophobic areas in the membrane may also contribute toward the enhanced interaction of the LIPs with the bacterial cell membranes.
Gubernator et alCitation150 studied in vitro antimicrobial activity of cationic LIPs (the compositions studied were PC/CHOL/DOTAP 3:4:3 and PC/DOPE/DOTAP 3:4:3) that harbored ciprofloxacin (cipro), MER, or gentamicin (GEN) against the Gram-negative clinical bacterial strains of P. aeruginosa, Klebsiella pneumoniae, and E. coli. Promising results were observed when cipro and MER were delivered using LIPs. The formulations presented the same or reduced MIC values in comparison to the free drugs. However, the liposomal GEN showed higher MIC values than the free drug against all the microorganisms, especially P. aeruginosa. The authors concluded that these differences could be due to the characteristics of the different drugs used, highlighting that the success of an antimicrobial treatment depends upon the complete formulation and not just on the delivery system employed for a drug.
Omri et alCitation151 investigated the effect of free and LIP-encapsulated polymyxin B (POLY B) against the ability of P. aeruginosa to produce pulmonary biofilms. LIPs were composed of DPPC and CHOL (2:1), and P. aeruginosa samples were obtained from the sputum of patients with CF. In general, the LIP formulations of the drug showed improved antibacterial activity, presenting reduced MIC values than the ones recorded for the free drug.Citation152–Citation154
Alhajlan et alCitation155 performed an in vivo experiment on rats that had lung infections of P. aeruginosa. The study was conducted in four treatment groups, and all administrations were done via pulmonary route: (i) group 1, treated with saline; (ii) group 2, treated with free POLY B; (iii) group 3, treated with empty LIPs; and (iv) group 4, treated with POLY B loaded into the LIP composed of DPPC:CHOL in 2:1 molar ratio. The results showed that the animals that were treated with liposomal POLY B presented significantly reduced bacterial count in the lung tissues (3.7±0.4 log10 colony-forming unit [CFU]/pair of lungs), while the animals that received POLY B alone presented 5.1±0.2 log10 CFU/pair of lungs. In addition, the group 4 rats showed better accumulation of the drug in their lungs, around five times higher than the group 2.
Alipour et alCitation156 studied the effect of liposomal POLY B against P. aeruginosa and also against several other Gram-negative bacteria, such as Bordetella bronchiseptica, E. coli, K. pneumoniae, Acinetobacter lwoffii, and Acinetobacter baumannii. The findings by the research group highlighted that all the microorganisms showed a higher sensitivity profile when the drug was used in combination with LIPs in comparison to the free drug, especially the resistant strain, P. aeruginosa (PAM13641-1).
Alipour et alCitation157 also performed an in vivo experiment in rats with pulmonary P. aeruginosa infection. After the generation of the liposomal bismuth-ethanedithiol-loaded tobramycin, the authors administered it via intratracheal route into the infected rats and compared those rats to the ones which were treated with the free drug. The results indicated lung bacterial counts of 3 and 4.7 log10 CFU/lung in the rats treated with liposomal tobramycin and free tobramycin, respectively. Since the drug did not accumulate in kidneys of the LIP-encapsulated drug recipients, the authors suggested that LIPs ensured targeted delivery of the drug, which was not the case for the administration of the free drug.
Alhajlan et alCitation155 evaluated the efficacy and safety of liposomal clarithromycin (CAM) and its effect on virulence of P. aeruginosa. The LIPs were synthesized by the dehydration–rehydration technique with different compositions: positively charged liposomal formulation was composed of DDAB, DPPC, and CHOL in a ratio of 4:2:1; the negatively charged liposomal formulation was composed of dicetyl phosphate, DPPC, and CHOL in a ratio of 4:2:1; and the uncharged liposomal formulation was composed of DPPC and CHOL in a ratio of 6:1. The strains were obtained from the lungs of patients who suffered from CF. The results revealed that LIP-encapsulated delivery of CAM led to reduced MIC of 8 mg/L in contrast to 256 mg/L MIC in the case of naked drug delivery. Further, the use of LIPs also aided in the inhibition of the bacterial growth (as observed in biofilm assay) and cytotoxicity of A549 cells by 3–4 logs.
summarizes the results of several studies related to the application of LIPs most used for control of microbial biofilms.
Table 1 LIPs used for control of microbial biofilms
MEs and nanoemulsions
MEs, as a drug delivery system, have become a highlight in the pharmaceutical research and industry. This is attributed to their potential to load a wide variety of molecules with different properties and deliver them efficiently to various parts of the body. MEs can be defined as transparent emulsions or phase-transition systems with either oil microdroplets dispersed in water or water microdroplets dispersed in oil. These are surrounded by an amphiphilic compound known as surfactant or amphiphile that is frequently associated with a suitable co-surfactant, thereby producing a thermodynamically stable system consisting of nanometric droplets in an internal phase.Citation158,Citation159 MEs are considered as reservoir systems; once the active constituent is isolated from the dissolution medium through a membrane or interface, it must be transposed for release. One of the characteristic features of MEs is their ability to separate from a dimensionally restricted environment and associate with different molecular compounds. Their versatile nature, such as low surface tension, in turn, improves the properties of the associated molecules, for example, solubility, stability, and bioavailability profile, consequently resulting in their increased absorption and permeation. When a surfactant is incapable of achieving nanometric droplets, it becomes indispensable to use a co-surfactant to enhance the surface area of droplets, thereby reducing the size of the particle and providing a better therapeutic effect.Citation160
MEs can be spontaneously generated, which makes them more advantageous than other delivery systems. In addition, MEs demonstrate an excellent thermodynamic stability, adequate appearance, and increased capacity to load drugs, which in turn result in their easy penetration through biological membranes, improved bioavailability, and reduced toxicity.Citation161 Moreover, these systems serve as potent antimicrobial agents due to the inability of microbes to survive in pure fat or oil. In addition, some studies show that the structure of MEs contributes significantly to their antimicrobial activity by especially targeting the bacterial cytoplasmic membrane.Citation162
There are many advantages that make MEs to be considered as the first choice of nanotechnology-based systems. First, they can be prepared without any kind of energy once they are considered thermodynamically stable systems that can be spontaneously formed. Also, they are able to load both hydrophilic and lipophilic drugs, improving the efficiency and thus reducing total dose and side effects. Regarding their limitations, MEs are not a good choice when high-melting substances need to be solubilized. In addition, a high amount of surfactant is needed to stabilize droplets formed during the formation, and there are some problems related to their stability, which depends on pH and temperature levels.Citation163,Citation164
Similar to MEs, nanoemulsions can be defined as heterogeneous systems in which one liquid (the inner phase) is dispersed in another (the outer phase) in the form of droplets in the presence of an emulsifying agent. The physicochemical properties of nanoemulsions are influenced by both their qualitative and quantitative compositions, and hence, they should be synthesized under strictly controlled conditions.Citation165 Certain inconsistencies exist in the literature with respect to the concept of nanoemulsions in relation to MEs. Although both systems have similar structural and visual characteristics, they differ in their thermodynamic stability. Contrary to the nanoemulsions, MEs constitute thermodynamically more stable systems. Further, ironically, the droplet size of MEs is generally smaller than the nanoemulsions (as opposed to the nomenclature).Citation166 Nevertheless, despite low thermodynamic stability, nanoemulsions are kinetically stable systems and exhibit several potentialities, such as drug delivery systems, especially for hydrophobic molecules with reduced water solubility.Citation167 schematizes the organization of a micro- and nanoemulsion (oil-in-water and water-in-oil).
Numerous studies have been conducted by various groups on MEs and nanoemulsions. Ramalingam et alCitation168 performed studies to analyze the efficacy of an oil-in-water nanoemulsion containing cetylpyridinium chloride (CPC) on dental unit waterline biofilms. The nanoemulsion was synthesized using soybean oil (25 vol%), deionized water (65 vol%), Triton X-100 (10 vol%), and CPC (1 wt%). The bacterial count was observed after exposing the formulation for 1, 6, 12, 24, 48, and 72 h to the biofilm formed by microorganisms, including Staphylococcus spp. All experiments resulted in a tremendous reduction in the number of microbial colonies, especially after 12 and 24 h (67 CFU/mL). However, the authors obtained even better results after 48 and 72 h of exposures when no visible colonies could be observed. In 2011, Ramalingam et alCitation169 tested the antimicrobial activity of a nanoemulsion on cariogenic S. mutans to control biofilm formation. The results displayed an inhibition of S. mutans grown in the concentrations ranging from 1:100 to 1:10,000. In addition, mature biofilms of the microorganism were significantly reduced after the treatment with the formulation.
In 2012, Ramalingam et alCitation168 evaluated the activity of CPC loaded onto a nanoemulsion against planktonic S. mutans, Lactobacillus casei, Actinomyces viscosus, C. albicans, and a mixed culture using microdilution technique and biofilm assay. The results demonstrated that compared to the components alone, the application of the formulation exhibited a better and enhanced activity against microorganisms, both individually and in mixed culture. Teixeira et alCitation170 studied the ability of MEs and nanoemulsions to inhibit the synthesis of biofilms by S. aureus NCTC 1803, S. typhimurium PSB 367, Listeria monocytogenes, P. aeruginosa, and E. coli O157:H7. Although both systems were capable of removing biofilms of E. coli, S. aureus, and S. typhimurium, ME was also effective against P. aeruginosa, a nosocomial agent with very high resistance to antimicrobial agents. However, L. monocytogenes was found to be resistant to both the systems.
Liu and HuangCitation171 developed curcumin (CUR)-loaded myristic acid-based MEs to study their antibacterial activity against skin infections caused by S. epidermidis BCRC-11030 (ATCC 12228). During formulation, myristic acid, a middle-chain fatty acid, was mixed dropwise with isopropanol (1:2) to improve the solubility followed by the preparation of surfactants, namely Tween® 80 and F127. The components were mixed for 10 min leading to the formation of transparent MEs. Myristic acid was chosen as one of the constituents because it demonstrated the best antibacterial activity compared with other fatty acids. Its antibacterial activity was found to be even higher than azelaic acid, commonly used to treat acne vulgaris. The results showed that before being loaded into the ME, CUR was 12 times less effective against the bacterium, but showed an MIC50 of 0.86 µg/mL after being loaded into the ME. In addition, when CUR was loaded together with myristic acid into the ME, it produced a synergistic inhibitory effect against S. epidermidis, implying that myristic acid may serve as an efficient vehicle for ME to load CUR.
Instead of encapsulating antimicrobial agents into an ME, some studies have demonstrated the use of MEs that are directly composed of components exhibiting antimicrobial properties so as to augment their effectiveness against microbial biofilms. Al-Adham et alCitation172 evaluated the effect of MEs as anti-biofilm agents after validating their effects against planktons. They formulated an ME containing 15% Tween 80, 6% pentanol, and 3% ethyl oleate in water and checked its efficiency against established P. aeruginosa biofilm. The results exhibited three log-cycle reductions in viabilities when biofilms were treated with an ME as compared to the control treatment that resulted in only one log-cycle reduction.
summarizes the results of several studies related to the application of MEs and nanoemulsions most used for control of microbial biofilms.
Table 2 MEs and nanoemulsions used for control of microbial biofilms
Cyclodextrins
CDs are a family of cyclic oligosaccharides that are obtained from starch-containing materials by enzymatic means. CDs were discovered in 1891 by the French researcher, Villiers, who noticed the ability of these molecules to form crystals.Citation173 Natural CDs are formed by the treatment of starch with amylase from Bacillus macerans, producing at least six glucose units. Among them, α-cyclodextrin (α-CD, six units), β-cyclodextrin (β-CD, seven units), and γ-cyclodextrin (γ-CD, eight units) are the most common members ().Citation174,Citation175 The external surface of the CD that offers protons (H1, H2, H4, H6) provides it a hydrophilic nature, whereas its cavity displays hydrophobic characteristics due to the presence of rings of C–H groups (H3 and H5) together with a ring of oxygen atoms forming glycosidic linkages.Citation176–Citation178 Therefore, CDs are truncated cone-shaped molecules with a relatively hydrophobic cavity and an external hydrophilic surface that can form supramolecular structures (inclusion complexes, ICs) with various molecules, mainly small molecules. The interactions, such as van der Waals, hydrophobic, and hydrogen bonding, contribute to the driving forces of IC formation, which can modify the biological, physical, and chemical properties of guest molecules.Citation179,Citation180
Due to their ability to form ICs, CDs are considered as efficient drug-carrying and drug-delivering systems. Besides, CDs are excellent solubilizers and physical and chemical stabilizers, and provide protection to the guest molecules from degradation in the gastrointestinal tract, thereby enhancing drug bioavailability.Citation181 CDs have been used as a pharmaceutical excipient, mainly to solubilize drugs with poor solubility in an aqueous environment through the synthesis of drug–CD complexes.Citation182,Citation183 The naturally occurring CDs have been used at a large scale in the evaluation and development of pharmaceutical formulations. However, their applications suffer from certain limitations; for example, β-CD exhibits a restricted solubility in water. Thus, new approaches to improve the action of CDs as drug carriers, such as the use of several chemically modified CDs,Citation183 are required. CD derivatives are classified into three groups: hydrophilic, hydrophobic, and ionizable derivatives. Each group performs a specific action. For example, in the hydro-philic group, 2,6-dimethyl-β-CD, 2,3,6,-trimethyl-β-CD, 2-hydroxypropyl-β-CD (HP-β-CD), and maltosyl-β-CD can enhance the water solubility of poorly water-soluble substances. Similarly, molecules within the hydrophobic group, such as 2,6-diethyl-β-CD, are capable of delaying the dissolution rate of poorly water-soluble drugs. Besides, the ionizable derivatives, such as O-carboxymethyl-β-CD (CM-β-CD), O-carboxymethyl-O-ethyl-β-CD, β-CD sulfate, and sulfobutyl ether β-CD, can improve the inclusion ability and decrease the localized irritation caused by drugs.Citation184,Citation185
The properties exhibited by CDs are relevant for pharmaceutical area given the fact that they can improve the solubility of water-soluble drugs, augment the chemical and physical stability, and provide protection to the guest molecules from the action of molecules and enzymes present in the gastrointestinal tract.Citation186,Citation187 However, there are limitations for parenteral formulation with CD, based on the type of CD used. For example, only α-CD can be used in parenteral formulation; β-CD affects the urinary volume and kidney and liver enzymesCitation188 and γ-CD exhibits nephrotoxicity, and hence, both these CDs are unsuitable for parenteral use.Citation189 Besides, the type and concentration of CD–drug complex also affect the encapsulation efficiencyCitation190 and the bioavailability of the drugs.Citation191 The other limitation with the native CD is the difficulty in the incorporation of large and hydrophilic substances. Thus, many chemical modifications have been performed to solve this disadvantage.Citation192
In the past years, several alternatives have been implemented and utilized to control and treat various microbial biofilms.Citation187,Citation193–Citation195 The characteristics exhibited by CDs, including lower toxicity, increased solubility of drugs, and an ability to act as stabilizers, make them eligible agents as drug carriers. These above-mentioned properties have attracted interest in the utilization of CDs to boost the action of drugs against bacterial and fungal biofilms.Citation196–Citation198
Brackman et alCitation199 developed gauzes functionalized with HP-β-CD to load vancomycin (VAN), hamamelitannin (HAM) (a natural product isolated from Hamamelis virginiana), and a quorum sensing inhibitor (QSI) of S. aureus. The authors evaluated the anti-biofilm activity of HP-β-CD complex against S. aureus and P. aeruginosa in vitro in a CW model. The functionalized HP-β-CD gauzes inhibited QS and the formation of biofilms when compared with nonfunctional-ized gauzes. The results demonstrated the utilization of HP-β-CD-functionalized gauzes containing a combination of VAN and HAM to be a promising approach against both single-species and mixed-species biofilms. Hence, HP-β-CD-functionalized gauzes may serve as an alternative to the treatment and prevention of wound infections caused by bacteria.
In a study performed by Garcia-Fernandez et al,Citation197 HP-β-CD was utilized for the loading and release of benzalkonium chloride (BzCl), for inhibiting S. aureus biofilm. The authors assessed the utilization of CDs to endow hydrogels and gauzes to increase and maintain the antimicrobial and delivery capacity for several hours. In this study, the CDs displayed an efficient delivery of BzCl, which in turn could successfully inhibit biofilm formation in the initial stage. They also decreased the number of living microbial cells in preformed biofilms in an in vitro CW biofilm model. Based on the results, the researchers concluded that BzCl-loaded functionalized HP-β-CD gauzes may be employed for the prevention and treatment of infectious wounds, although in vivo assays are necessary to confirm the findings.
Fidaleo et alCitation200 evaluated the anti-biofilm activity of TCS complexed with HP-β-CD and CM-β-CD. The two complexes (TCS–CD) were observed to exhibit anti-QS activity by interfering with cell-to-cell communication mechanisms in Chromobacterium violaceum model system. Thus, the authors suggested the use of TCS–CD complexes for producing antimicrobial hydrogels or hydrophilic coatings for medical devices to inhibit biofilm formation on the devices and promote the release of antimicrobial agents.
A recent study demonstrated the potential of HP-β-CD to clean reverse osmosis membranes contaminated and fouled by P. aeruginosa. The CD, HP-β-CD, removed the biofilm forming complex with the EPS of bacteria. Although the mechanism of removal of the biofilm by HP-β-CD was unknown, the authors attributed it to the structural distribution of HP-β-CD on the surface of the biofilm. In addition, the authors believed that there could be a destabilization of the biofilm due to the generation of free energy, making the detachment an energetically propitious process.Citation201
Iordache et alCitation198 developed a thin film consisting of β-CD complexed with usnic acid, a dibenzofuran with pharmacological properties, to improve the release of a drug into the bacterial cells. The thin film was observed to be successful in preventing the adhesion of cells and development of S. aureus biofilm at all stages. Besides, the film demonstrated good biocompatibility, an attribute that may lead it to serve as an alternative for promoting medical surfaces capable of preventing microbial colonization.
Shanmuga Priya et alCitation202 formulated a solid complex with β-CD and drug rifabutin (RFB) to increase the solubility and antimicrobial activity of the drug. The conjugation to the solid complex enhanced the effectiveness of the drug in reducing the biofilm formation by E. faecalis, S. aureus, Proteus vulgaris, and P. aeruginosa, when compared to the control. The study related the in vitro anti-biofilm action of pure RFB and its β-CD complex against both Gram-positive and Gram-negative microorganisms.
β-CDs have been used for the stable delivery of silver nanoparticles (AgNPs) due to their physicochemical properties, such as hydrophilicity, high molecular weight, the presence of a large number of hydrogen donors and acceptors, and inclusion capacities.Citation203,Citation204 A recent study involving AgNPs–β-CD complex demonstrated an excellent antibacterial activity of the complex, which inhibited the formation of biofilm by S. epidermidis, the common bacteria isolated from medical devices, such as catheters, prosthetic implants, and intrauterine devices. In addition, the AgNPs–β-CD complex was more effective than AgNPs. The authors used different concentrations of silver (Ag, 8 and 16 ppm) on biofilm of S. epidermidis; the AgNPs–β-CD complex inhibited biofilm formation to more than 90%, whereas AgNPs could inhibit only 10%–15%.Citation204
In a recent work performed by Oprea et al,Citation205 the anti-biofilm activity of zinc oxide-β-CD-cefepime (ZnO-CD-Cfp) complex against E. coli was assessed. The complex was selected and evaluated owing to its enzymatic resistance to β-lactam antibiotics. ZnO nanoparticles are used as antibacterial agents; however, the primary problem with them is their poor solubility in water. Thus, the use of CDs can enhance the solubility and bioavailability of these nanoparticles. Besides, the authors demonstrated that ZnO/CD complex displays an efficient release of Cfp, consequently leading to an improvement in the anti-biofilm activity. The results also demonstrated that the obtained coatings can prevent the biofilm formation and adhesion after 24–48 h, and the complex also demonstrated inhibitory effect against mature biofilm on the coated surfaces.
The last few years have witnessed studies involving CDs to improve the delivery of drugs to control fungal biofilms. Nava-Ortiz et alCitation206 used medical devices designed from polyethylene and polypropylene functionalized with HP-β-CD and β-CD to incorporate miconazole (MICO). Since the drug MICO is insoluble in water, CDs serve as an efficient option to assist the solubilization, thereby providing adequate bio-availability. Besides, the surface functionalization involved the grafting of glycidyl methacrylate after pre-irradiation with oxidative γ-rays. These complexes demonstrated positives results, including a reduction in microbial adhesion and prevention of the development of Candida biofilm.
In another work, a topical formulation of amphotericin B (AmB) solubilized in γ-CD (AmB–CD complex) inhibited the biofilm formation by Candida spp., C. albicans, Candida dubliniensis, C. glabrata, Candida guilliermondii, and C. parapsilosis, except for C. krusei, which was resistant to AmB–CD. The other yeasts, such as Saccharomyces and Trichosporon, were found to be sensitive to the complex. The AmB–CD, compared to AmB dissolved in dimethylsulfoxide, exhibited a high penetration through these biofilms. Despite the suggestion by the authors that the AmB–CD could be employed in catheters and prosthesis to prevent fungal infections of bloodstream, a requirement for further toxicological and clinical studies is warranted before its use in humans.Citation207
In another study, the authors elaborated gold surfaces functionalized to anidulafungin (ANF) or thymol (THY) with HP-β-CD and randomly methylated β-CD (RAMEB) complexes. Both antifungal complexes displayed similar anti-biofilm action. The results indicated that CDs improved the solubility of ANF, and together, the complexes inhibited the adhesion of C. albicans biofilm. In addition, THY–CD complex could potentially eliminate the biofilm synthesized by C. albicans. However, a considerable difference exists between THY and ANF molecules; ANF is more than seven times larger than THY and cannot be completely incorporated into the cavity of CD, while THY is a small molecule. Thus, the THY molecule loaded into RAMEB could interact efficiently with the cells and prevent the growth of C. albicans.Citation177
summarizes the results of several studies related to the application of CDs most used for control of microbial biofilms.
Table 3 CDs for control of microbial biofilms
Solid lipid nanoparticles
SLNs are colloidal drug delivery systems in which the matrix is formed by solid lipids and usually consists of physiologically well-tolerated substances; thus, the system offers low cytotoxicity. The structure of SLNs is very similar to nanoemulsions, but their core is formed by lipids that are solid at room temperature. This structural rigidity provides less mobility to the incorporated drug, which is manifested in terms of a better control during the release of drugs. The production of SLNs can be easily scaled to the level of pharmaceutical industry.Citation140 SLNs comprise one or more solid lipids, and their structure is stabilized by the presence of surfactants.
SLNs show the properties of different colloidal carriers. For instance, these are physiologically acceptable compounds like emulsions and LIPs and capable of yielding a controlled release of drugs from lipid matrices such as PNs. The mentioned advantages include the incorporation of lipophilic and hydrophilic drugs, physical stability, controlled drug release, biocompatibility, potential for site-specific drug delivery, improved drug stability, better formulation stability, ability to freeze-dry and reconstitute, high drug payload, controllable particle size, avoidance of carrier toxicity, low production cost, and easy scale-up and manufacturing.Citation208 schematizes the SLNs structure for drug delivery.
However, certain limitations are also associated with the SLN system. These include limitation of drug load due to the solubility of the drug in the solid lipid, drug expulsion phenomenon when lipid crystallizes to the stable β-form, and particle concentration in the aqueous dispersions ranging from about 1% to a maximum of only 30%.Citation209
Singh et al,Citation210 with an aim to increase antimicrobial activity against S. aureus biofilms, developed SLNs containing cefuroxime axetil (CA). The authors used stearic acid (SA) as a single lipid and SA and tristearin (TRI) as a binary lipid; the emulsification–evaporation method was used for the formation of SLNs. The nanosystem displayed a low efficiency of encapsulation. To improve this parameter, an SLN formulation (CA-SLN) was developed using binary lipid that exhibited an encapsulation efficiency of 70.62%±0.82%. The high efficiency of SLNs consisting of binary lipids was attributed to the large deformation of the crystal structure of SA in the presence of TRI, leading to the formation of cavities in its structure and efficient accommodation of drug molecules. The size of the formed nanoparticles was in the range of 279.2±28.5 nm, and the zeta potential (ZP) was −23.58 to −25.95 mV. The in vitro analysis of the release of free CA displayed a 99.68% release in just 2 h, whereas CA-SLN exhibited a 53.68% release in 2 h and 95.58% within 12 h, indicating that nanoparticles provide an additional feature of prolonged drug release owing to the diffusion of the drug through the lipid matrix of the nanoparticles. The in vitro anti-biofilm studies exhibited an inhibition zone of 9 mm for the free drug, whereas the treatment with CA-SLN created an inhibition zone of 13 mm. According to the authors, the above-mentioned observation was attributed to the enhanced diffusion and accumulation of CA-SLN in the polysaccharide matrix of the biofilm/microbial cells. The CA-SLN exhibited an MIC of 4 µg/mL, whereas free drug solution displayed an MIC of 10 mg/mL. Therefore, the authors believe that the bilipid SLNs consisting of SA and TRI meet the requirements for use as carriers of cefuroxime to inhibit S. aureus biofilm.
Nafee et alCitation211 developed SLNs with an aim to optimize the delivery of QSIs in lung tissue of patients with CF. SLNs were synthesized by hot-melt homogenization with glyceryl palmitostearate, glyceryl behenate, and TRI. The lipophilic nature of QSI facilitated its incorporation into SLNs demonstrating an encapsulation efficiency of 68%–95%. The release of QSI from different SLNs was studied in phosphate-buffered saline (PBS) buffer and simulated lung fluid (SLF). A controlled release of QSI in PBS buffer was observed with 60%–95% of drug release over 8 h. Therefore, SLF was considered to be a more relevant and a better medium for in vivo conditions; the release of QSI was prolonged in SLF showing greater than 20% burst in all SLNs. Mucus interaction assay demonstrated that the SLN could not alter physicochemical characteristics even when in contact with the highly characteristic thick mucus in patients with CF. Further, no significant aggregation was observed in maintaining its potential for carrying QSIs. The cytotoxic property of plain and loaded SLNs was checked on Calu-3 cells (human airway cell line) representing the bronchial area. The experiment demonstrated 80%–100% of cells to be viable after incubation with SLNs. Pyocyanin, the virulence factor, produced by P. aeruginosa is required for complete virulence in animal models. It promotes virulence by interfering with numerous cellular processes involved in the inhibition of pyocyanin. The inhibition of pyocyanin demonstrated that the formulation not only maintained the activity of the free QSI but was superior at all the tested concentrations. The QSI-loaded SLNs were superior to the free QSI in inhibiting pyocyanin. The authors reported an inhibition of the growth of P. aeruginosa upon treatment with SLN. According to the authors, SLNs, efficiently loaded with the novel QSIs, could be formulated with a release of the payload over more than 8 h. The hydrophilic surface of SLNs allowed the QSIs to efficiently penetrate the mucus, thereby acting as an efficient drug carrier for an effective therapy for CF. Thus, the use of SLNs reflects a new perspective in the nano-based delivery of novel anti-infectives.
Taylor et al,Citation212 with the aim of preventing and treating nosocomial infections in surgical implants, synthesized SLNs composed of SA, lauric acid (LA), and oleic acid (OA). The strategy was based on the use of SLNs to destabilize bacterial adhesion in tissues and surfaces, thus preventing the formation of biofilms. Furthermore, the antibacterial action of LA and OA stimulated the disruption of bacterial cell membrane. All SLN formulations depicted a hydrodynamic radius below 200 nm and a negative surface charge; the SLN solutions were coated with silicone polyvinyl chloride (PVC) to produce coatings with antimicrobial potential. Confocal microscopy was used to analyze the bacterial adhesion that revealed the ability of bacteria to adhere to the coating surfaces in the absence of SLN; however, after coating the surface, no bacteria could adhere to the endotracheal tube surface coated with SLN. The observation was reflected in the adhesion rate, which was 96.93% lower than in the uncoated surfaces. In the case of uncoated surfaces, the presence of bacteria and integral membrane morphologies was observed, whereas amorphous fragments and free bacterial DNA were observed on the coated surfaces, indicating a disruption of the cell wall and bacterial killing. According to the authors, potential adhesion was drastically reduced due to the presence of roughness on the surface, a decrease in the contact area, and weakening of the bacterial adhesion mechanism. Moreover, the small percentage of bacteria that achieved adhesion displayed destabilized walls due to the presence of fatty acids in the SLN with bactericidal effect. The authors postulated that it is essential to search for new alternatives, such as SLNs, which are not dependent on the antimicrobial drug action due to the multidrug resistance of microbes to antibiotics. Such SLNs may be applied as coatings of surgical implants to exert an anti-biofilm activity.
summarizes the results of several studies related to the application of SLNs most used for control of microbial biofilms.
Table 4 SLNs for control of microbial biofilms
Polymeric nanoparticles
PNs may be defined as solid colloidal particles with a size less than 1 µm and carrying bioactive molecules or substances. These can be synthesized using different methods with a feature size of 100–500 nm.Citation213
The early 1970s witnessed the introduction of methods for the preparation of nanoparticles. According to their composition and structural organization, nanoparticles can be classified as nanospheres and nanocapsules. The nanospheres do not contain oil in their composition and comprise a polymeric matrix with the active substance retained or adsorbed in the matrix. Nanocapsules consist of a polymeric shell disposed around an oily core, where the active substance can be dissolved in the core and/or adsorbed onto the polymer wall.Citation214
PNs have some advantages over other nanoparticulate systems. These include high stability in the body, long storage stability, and increased biocompatibility and biodegradability. These features allow the development of nanosystems with different degradation times and controlled drug release. The main polymers include polycaprolactone, poly lactic-co-glycolic acid (PLGA), polylactic acid, and chitosan (CS), a polysaccharide of natural origin that has been widely used due to its biodegradability and mucoadhesive capacity.Citation215,Citation216 The disadvantages of nanoparticles in relation to PNs could be attributed to the possible toxicity of the biodegradation products and a higher cost compared to other nanostructured systems, depending on the material and process used for the development of the nanosystem.Citation217 schematizes the PNs structure for drug delivery.
Numerous reports in the literature cite the utilization of PNs for controlled drug release and as a vector for a wide range of diseases; however, with the advent of bacterial resistance to antibiotics and the recent reports on the biofilm production, the data involving the use of PNs against biofilms are still scarce.
Da Silva et alCitation218 evaluated the biofilm formation in the sealant–dentin interface when treated with phosphorylated chitosan (PHCS) and chitosan connected to Rose Bengal (CSRB), which is a photo sensitizer. A combination of PHCS and CSRB (RBPH), and CS nanoparticles was incorporated into the sealant (zinc oxide–eugenol) used to explore the benefits of applying CS in endodontics. The roots of bovine teeth were used because they are physiologically and histologically very similar to human teeth and facilitate the application of the treatments due to their size. Biofilm formation was induced by applying E. faecalis and subsequent subjection to PHCS, CSRB, RBPH, and CS nanoparticles embedded in the sealant. The group submitted to CS nanoparticles incorporated into the sealant displayed a lower tendency of biofilm formation, demonstrating antimicrobial action; this action was probably due to the electrostatic interaction between the bacterial components and CS that resulted in the destabilization of the bacterial wall. However, CS nanoparticles have a positive surface charge that influences their interaction with the negatively charged dentin; this interaction hinders the adhesion of bacteria onto the tooth tissue, thereby preventing biofilm formation. Biofilm growth was also inhibited within the sealer–dentin interface when treated with PHCS, but there was a moderate inhibition when treated with CSBH followed by photodynamic therapy.
Abdelghany et alCitation219 developed a strategy to increase GEN retention in PLGA nanoparticles to verify the effectiveness of these nanoparticles in controlled drug release in vitro and in vivo against infection by P. aeruginosa. Two methodologies were employed to obtain the PNs: water-in-oil-in-water method (w/o/w) and solid-in-oil-in-water method (s/o/w). Nanoparticles generated by the w/o/w method had a size up to 251 nm, encapsulating 6.4 µg of GEN per milligram of PLGA. The authors reported that an alteration of the pH of the aqueous phase from 5.0 to 7.4 resulted in an increase of about three times in the drug-entrapping rate of PLGA nanoparticles, without significantly changing the particles diameter. An increased entrapment rate was also observed for the particles generated by s/o/w method when changing the aqueous phase pH from 5.0 to 7.4, yielding particles of 359 nm size. The release profile was similar for nanparticles obtained by both methodologies at pH 7.4 with a 50% release in 24 h and a slower and continuous release for 16 days. For the nanoparticles obtained at pH 5.0, an initial release of only 25% was observed after 24 h; however, after 7 days of release, the released drug concentration was similar to the nanoparticles prepared at pH 7.4. These differences in the release profiles resulted from the differences in the drug distribution in the polymeric network and core of the nanoparticles. With respect to bacterial growth in planktonic form, an MIC of 1.5 and 3.0 mg/mL for free and nanocoated GEN was observed, respectively, although free GEN in the planktonic form led to a more efficient inhibition of bacterial growth as compared to the nanocoated drug (on observing the GEN activity on preformed biofilm of P. aeruginosa). This offered an improved, efficient, and greater inhibition compared to the free drug, probably due to the slow and controlled release. In the in vivo studies, 96 h after the infection, the animals treated with nanoparticles exhibited a significant reduction in bacterial CFU compared to the untreated controls. Further, a significant decrease was also observed in interleukin-6 (an inflammatory mediator), indicating the severity of the injury in animals treated with polymeric PLGA nanoparticles. According to the authors, nanoparticles and microparticles harbor the potential to be utilized in aerosol delivery and may increase the therapeutic efficacy of GEN and other aminoglycosides for the treatment of lung infections.
Cai et alCitation220 developed lipid polymer nanoparticles (LPNs) in order to carry amoxicillin (AMOXIL) to enhance antimicrobial efficacy against planktonic and biofilm forms of H. pylori. According to the authors, the outer lipid bilayer of the PNs can merge easily into the polysaccharide matrix (main component of the biofilm) and then destroy this protective layer of bacterial microenvironment, leading AMOXIL directly through the free bacterial cells. The core of the PNs consists of pectin sulfate (PEC) and mixed lipids including rhamnolipids and phospholipids. The PEC inhibited H. pylori by competing with target cells, and the presence of rhamnolipids and phospholipids contributed to a second antibacterial activity due to their anti-adhesive properties, making the biofilm formation difficult. All LPNs displayed a size range from 147 to 243 nm, and AMOXILl encapsulation efficiency rate was 93.6%–96.7%. In the antibacterial activity tests, approximately 53% of live bacteria were observed in planktonic form, with no significant difference between the two treatments; however, in the assays involving biofilm, 90.2% of live bacteria were observed when treated with free AMOXIL, and when treated with LPNs, the bacterial viability dropped to a range of 18.6%–32.6%, indicating that the LPNs have a superior effect to the conventional antibiotic treatment. Fluorescence tests indicated that bacteria treated with LPNs were not able to invade AGS cells, whereas approximately 100% of AGS cells were invaded and lysed in the presence of free AMOXIL, showing that LPNs may be a promising strategy for tissue protection against H. pylori and a tool against the formation of bacterial biofilms.
Takahashi et alCitation221 developed and evaluated the antimicrobial potential of PLGA-PNs and chitosan-associated PLGA (CS-PLGA)-PNs carrying CAM in biofilms of S. epidermidis using the field emission-scanning electron microscopy (FE-SEM) technique. The PNs were prepared by emulsification–solvent diffusion method with a diameter of 252–416.5 nm and a ZP between −15.2±0.6 and 19.9±1.7 mV. The ZP of PLGA-PNs remained negative due to dissociation of the carboxyl group of PLGA in water, whereas the ZP of CS-PLGA-PNs remained positive due to the protonation of the amino group of CS. In trials conducted to evaluate the antimicrobial potential of nanoparticles on biofilms, the highest antimicrobial potential was exhibited by the PLGA-PNs carrying CAM with 62% of dead bacteria. Confocal laser scanning microscopy (CLSM) demonstrated that biofilms treated with PLGA-PNs carrying CAM displayed pores, showing an antimicrobial activity on their polysaccharide matrix. The images obtained by FE-SEM depicted that bio-films treated with CS-PLGA-PNs consisted of small pores in the polysaccharide matrix with no bacterial killing. However, the images of biofilms treated with PLGA-PNs carrying CAM exhibited a highly eroded and disturbed matrix with large pores. In addition, a decrease in the thickness of the biofilms was observed, indicating the bacterial death. The authors concluded that PNs made of PLGA may serve as effective carriers of CAM in anti-biofilm treatment. They suggested that FE-SEM could be a useful technique to obtain important information, thereby allowing the development and improvement of strategies in the medical area.
summarizes the results of several studies related to the application of PNs most used for control of microbial biofilms.
Table 5 PNs for control of microbial biofilms
Metallic nanoparticles
MNPs are metallic particles with one or more dimensions (length, width, and thickness) in the range of 1–100 nm. FaradayCitation222 recognized for the first time the presence of MNPs in solution, and Gustav Mie in 1908 gave a quantitative explanation of their color.Citation223 The principal characteristics of MNPs are large surface area-to-volume ratio as compared to their bulk equivalents, large surface energies, the transition between molecular and metallic states providing specific electronic structure (local density of states), plasmon excitation, quantum confinement, short range ordering, increased number of kinks, a large number of low-coordination sites such as corners and edges, a large number of “dangling bonds” and consequently specific chemical properties, and the ability to store excess electrons.Citation224
The major bottleneck during experimental synthesis of MNPs is obtaining stable colloidal suspensions, as MNPs have a high surface energy, favoring thermodynamically the aggregation of those ready to form metal–metal bonds.Citation225 In order to prevent the aggregation of nanoparticles, the preparation of colloidal systems is generally performed in the presence of agents known as stabilizers that are adsorbed onto the surfaces of the nanoparticles, forming a self-assembled layer, which prevents coalescence. Some of the most effective stabilizers are polymeric, for example, polyvinylpyrrolidone,Citation225 polyvinyl alcohol,Citation226 and polyacrylic acid,Citation227 which possess basic sites of Lewis with high affinity for nanoparticles, and sufficiently long organic chains that create a steric hindrance to prevent interactions among them.
The MNPs have immense potential for several technological applications and are being tremendously investigated for their antimicrobial and antifungal perspective. Zinc, titanium, silver, and gold are some of the most widely studied nanoparticles among several others.
Zinc
Khan et alCitation228 studied the effect of zinc oxide nanoparticles (ZnO-NPs) on oral cavity bacteria (S. oralis ATCC 35037). ZnO-NPs were prepared by sol–gel method and were characterized by X-ray diffraction (XRD) and transmission electron microscopy (TEM) that confirmed a polycrystalline wurtzite structure and crystalline polygonal shape, respectively. The particle showed a size distribution of 491 nm and a ZP of 16.4 mV, as determined by dynamic light scattering (DLS). The oral bacterial population was treated with ZnO-NPs, and a reduction in total bacterial counts was observed. The authors applied a concentration of 10 mg/mL of ZnO-NPs, and the CFU was reduced to be 66% and 59% on NA and MRS agar plates, respectively. At 50 mg/mL concentration, up to 92% reduction in the bacterial survival was recorded as compared to the untreated controls. However, at 100 mg/mL level, the ZnO-NPs inhibited the biofilm formation on polystyrene and glass surfaces and acrylic denture.
In another study, Khan et alCitation229 also studied the antimicrobial and anti-biofilm potential of ZnO-NPs against the oral bacterial isolates of Rothia dentocariosa (Ora-7) and Rothia mucilaginosa (Ora-16). They used the sol–gel method for obtaining the ZnO-NPs and characterized them by TEM and XRD. The ZnO-NPs showed a smooth-surface polygonal shape with an average size of 35.23 nm. The growth-inhibitory activity of ZnO-NPs against R. dentocariosa and R. mucilaginosa was determined by IC50 to be 53 and 76 µg/mL, respectively. The authors coated the artificial teeth and dental prostheses with R. dentocariosa and R. mucilaginous and conducted a study on the effect of nanoparticles on biofilm formation, and the results showed that ZnO-NPs inhibited the bacterial biofilm formation on the surfaces; the NPs were more effective against Ora-7 at all concentrations tested, while the clove oil was active at a concentration of 10 mg/mL.
Sangani et alCitation230 synthesized ZnO-NPs by a sol–gel procedure and characterized them by TEM. This method yielded particles with an average size of 20 nm. The study aimed to assess the biofilm formation by 15 clinical isolates and one reference (ATCC 9027) strain of P. aeruginosa and the antibacterial activity of ZnO-NPs. The ZnO-NPs were tested at different concentrations in the range of 0–400 µg/mL, MIC was determined by broth dilution method, and minimum bactericidal concentration (MBC) was also determined. The average MIC and MBC of ZnO-NPs for the 15 isolates were 158 and 325 µg/mL, respectively, while those for the reference strain were 150 and 325 µg/mL, respectively. The MIC50 and MIC90 of the ZnO-NPs were 150 and 175 µg/mL, respectively. The biofilms were formed in 96-well flat-bottom tissue culture plates (TCPs), and the ZnO-NPs levels were tested in the range of 50–350 µg/mL. The results showed about 75% and 94% reduction in the optical densities (ODs) at 250 and 350 µg/mL level, respectively, and the ZnO-NPs were able to eliminate preformed biofilm.
Dwivedi et alCitation231 employed a soft chemical/solution process to obtain ZnO-NPs and characterized them by TEM, atomic force microscopy, and XRD. The ZnO-NPs showed a smooth surface, spherical shape, and wurtzite phase, and the size was in the range of 10–15 nm. The disk diffusion assay was chosen to evaluate the antibacterial activity against P. aeruginosa which showed the best zone inhibition of 16 mm at 100 mg/mL of ZnO-NPs. The inhibition of the biofilm formation was also evaluated in this study, and the ZnO-NPs were able to inhibit biofilm formation at the concentrations of 50 and 100 µg/mL. The interaction of ZnO-NPs with bacterial solution produces reactive oxygen species (ROS) that is an important factor in the inhibition of biofilm. An increased production of ROS was directly proportional to the increase in antibacterial activity of ZnO-NPs; therefore, it can be presumed that the mechanism of action is related to the higher production of ROS. This study advocates the potential of ZnO-NPs usage as future nano-antibiotics.
Bacteria can stay in biomaterials such as polymers and consequently form antibiotic-resistant bacterial biofilms. Seil and WebsterCitation232 studied the effect of incorporation of ZnO nanoparticles into PVC. The ZnO-NPs of about 60 nm diameters were procured from Nanophase Technologies and were incorporated into PVC by sonication to facilitate the homogenization. The presence of ZnO-NPs on the PVC surface was confirmed via SEM. In order to evaluate the antimicrobial activity of ZnO-NPs against biofilms of S. aureus (ATCC 25923), they treated a PVC membrane with different concentrations of ZnO-NPs (0%, 2%, 10%, 25%, and 50%, w/v) to superimpose coverslips and inoculated 3×106 CFU on each coverslip. The ODs were recorded after 24 and 72 h of the inoculation and analyzed by crystal violet staining. The polymer composites containing ZnO-NPs clearly showed a reduction in the number of total bacterial count as compared to those without ZnO-NPs (control). A reduction of 24% and 28% in the biofilm formation by the composite containing ZnO-NPs at 25% and 50% (w/v) concentration was observed, respectively, over the control. The crystal violet staining analysis showed about 55% reduction in the biofilm formation on the surface of the composites containing different concentrations of ZnO-NPs against the control. The difference in the reduction in biofilm formation at the different concentrations of polymer ZnO-NPs composites was not significant (P<0.05).
Lee et alCitation233 studied the anti-biofilm activity of Zn2+ and/or ZnO-NPs against P. aeruginosa PAO1, E. coli O157:H7 (ATCC 43895), and a methicillin-sensitive S. aureus (MSSA; ATCC 6538) and a methicillin-resistant S. aureus (MRSA; ATCC BA-1707) strain. The test biofilm was formed on polystyrene plates in accordance with Pratt and Kolter.Citation234 The formation of P. aeruginosa biofilm on polystyrene surfaces was inhibited by ZnCl2 and Zn(NO3)2 in a dose-dependent manner, while ZnO (1 mM; average diameter 50 nm) reduced biofilm formation by 95%. ZnCl2 and ZnO also actively inhibited the biofilm formation on a glass surface. ZnO at 5 mM level was able to inhibit the biofilm formation of E. coli O157:H7, MSSA (ATCC 6538), and MRSA (ATCC BA-1707).
summarizes the results of several studies related to the application of ZnO-NPs most used for control of microbial biofilms.
Table 6 Zinc and ZnO-NPs for control of microbial biofilms
Titanium
Haghighi et alCitation235 evaluated the application of titanium dioxide nanoparticles (TiO2-NPs) and ethylene diamine tetra acetic acid (EDTA) as an antifungal agent against C. albicans biofilms. Titanium tetrachloride (TiCl4) was used as a precursor in TiO2-NPs synthesis. The TiO2-NPs were further characterized for their shape and size using SEM and the type of crystalline structure using XRD. Fluconazole-sensitive (ATCC 10231) and fluconazole-resistant (ATCC 76615) reference strains of C. albicans were used to form biofilms. TiO2-NPs and EDTA were tested in the range of 4–8 and 6.5–15 µg/mL, respectively, and fluconazole was used as a control. The antifungal activity of the samples was estimated via XTT reduction and ATP bioluminescence assays. The results obtained from both the methods showed that the biofilms of a fluconazole-sensitive strain of C. albicans were inhibited by TiO2-NPs (5.14 µg/mL), EDTA (8.09 µg/mL), and fluconazole (4 µg/mL). The fluconazole-resistant strain required relatively higher concentrations of these agents, that is, 5.35, 11.33, and 8 µg/mL, respectively (P<0.05). The authors proposed that the antifungal activity of TiO2-NPs is probably associated with the intracellular ROS produced by them leading to cell death by altering normal respiratory activity. In order to explain the antifungal activity of EDTA, different theories such as the inhibition of filamentation process or chelation of some constituents essential to biofilm matrix have been proposed. These findings were further verified by both XTT and ATPase assays which showed the same outcomes, and these can also be a good choice to evaluate the anti-biofilm activity of TiO2-NPs.
Ibrahem et alCitation236 carried out the biosynthesis of titanium nanoparticles (TiNPs) using vaginal Lactobacillus crispatus isolated from healthy Iraqi women. The nanoparticles were evaluated for their antibacterial and anti-adhesive properties against E. coli, K. pneumoniae, Morganella morganii, A. baumannii, and S. aureus isolated from urine samples of the Iraqi women exposed to recurrent urinary infection. The antibacterial activity was evaluated using microdilution method, and the sample concentration ranged from 4 to 128 mg/mL. The effect of these nanoparticles on bacterial biofilm was tested using Congo Red agar and tube method, and the anti-adhesive property was tested by TCP method. The TiNPs exhibited anti-adhesive activity against all clinical isolates (except for E. coli), and the highest and lowest efficiency were recorded against M. morganii (48%) and A. baumannii (6%), respectively. Tube test method also showed similar results related to the inhibitory effect on biofilm formation, as TiNPs were effective against all strains except E. coli. The Congo Red agar method showed the presence of pink or pale gray colonies of K. pneumoniae, M. morganii, and S. aureus representing a reduced slime production by these strains; however, they typically formed black colonies in this specific medium. The black colonies formed by other strains indicated that the TiNP treatment did not alter the slime-producing property. The authors concluded that TiNPs can be considered as a promising antibacterial agent in the treatment of UTIs as they are cost effective, nontoxic, and ecofriendly.
Maurer-Jones et alCitation237 evaluated several types of TiO2-NPs including acid-catalyzed TiO2-NPs synthesized in house, and Evonik Aeroxide Degussa P25 and Eusolex T-Eco (both commercially available) for their competence to inhibit Shewanella oneidensis biofilm using quartz crystal microbalance and riboflavin secretion by high-performance liquid chromatography. When compared to the controls, no differences were observed in the strain viability after an exposure to various concentrations of the three types of TiO2, but when exposed to all samples of TiO2 some sort of dose-dependent decline in the growth of strain could be observed. The changes were observed in the mass added to the crystal in order to form biofilm and viscoelastic properties irrespective of TiO2-NP exposure to the strains, although this alteration did not confer any resistance property. When the strain was exposed to high concentrations of TiO2 (all samples), an unexpected increase in riboflavin secretion was recorded. Riboflavin is a direct way to measure the bacterial growth, as it results from the molecule flavin mononucleotide, normally secreted by S. oneidensis. The authors suggested that these changes could not be considered a consequence of oxidative stress, but the altered gene expression in the bacteria due to TiO2-NPs exposure may lead to subtle changes in the bacterial function.
summarizes the results of several studies related to the application of TiNPs and TiO2-NPs most used for control of microbial biofilms.
Table 7 TiNPs and TiO2-NPs for control of microbial biofilms
Silver
Namasivayam et alCitation238 analyzed the anti-biofilm property of catheters coated with AgNPs against clinical isolates of S. aureus. AgNPs synthesized by Azadirachta indica leaf extract were applied to a catheter and were characterized by SEM, which showed the complete dispersion of the uniform spherical nanoparticles of 50–60 nm size on the surface of the catheter fiber. A differential effect on biofilm inhibition was observed in the catheter coated with nanoparticles, and the highest inhibition was observed after 72 h of incubation. In the biochemical composition, the total number of carbohydrates and the biofilm matrix proteins were greatly reduced. This study could be insightful toward the development of medical devices coated with antimicrobials against pathogenic microorganisms.
Park et alCitation239 analyzed the antimicrobial activity of AgNPs against PA01 planktonic bacteria and biofilm of P. aeruginosa, and the activity was compared with that of silver ions. The inactivation of biofilms by AgNPs was very sensitive to stirring, which caused a high AgNP bio-absorption. Although AgNPs activity against planktonic cells was approximately 10% of the activity of the silver ions, their activity against biofilm cells was comparable to that of the silver ions at the same concentration, after 90 min of agitation (about 3.5 log inactivation of biofilm cells). This study pointed out that AgNPs inactivate biofilms by bio-absorption; however, the same did not occur with the ionic silver as such.
Martinez-Gutierrez et alCitation240 explained how biofilms protect pathogenic organisms from adverse environmental conditions and can be reservoirs and sources of disease outbreaks, especially in medical equipment. The major objective of their study was to evaluate the anti-biofilm activity of AgNPs against various microorganisms of clinical interest. The antimicrobial activity of AgNPs was tested in biofilms obtained under static conditions and high fluid shear conditions in a bioreactor. After exposure to 100 mg/mL AgNPs, a 4 log reduction was recorded in the CFU of P. aeruginosa under turbulent fluid conditions in a biofilm reactor. The antibacterial activity of AgNPs against various microbial strains grown in polycarbonate membranes was well documented. It was concluded that AgNPs effectively prevent the formation of biofilms and also kill the bacteria in established biofilms, suggesting the potential role of AgNPs in the prevention and treatment of biofilm-related infections. The AgNPs at 250 mg/mL or a higher concentration were able to inhibit bacterial growth without using any antibiotics; this indicates the behavior of AgNPs as an antimicrobial agent and as a potential modifier of the bacterial cell wall. However, further research is still required to utilize AgNPs for preventive and therapeutic strategies.
The work of Gurunathan et alCitation241 focused on the application of AgNPs as antibacterial, antifungal, antiviral, anti-inflammatory, and anti-angiogenic agents due to their unique biological, physical, and chemical properties. The study aimed to investigate the antibacterial and anti-biofilm activity of isolated AgNPs alone or in combination with conventional antibiotics against various human pathogenic bacteria. The authors showed a reliable, simple, cost-efficient, and natural synthesis of AgNPs by treating silver ions with Allophylus cobbe leaf extract. The AgNPs synthesis was characterized by UV–Vis absorption spectroscopy, XRD, Fourier transform infrared spectroscopy (FTIR), X-ray photoelectron spectroscopy (XPS), DLS, and TEM. Further, the antibiotic, antibacterial, and anti-biofilm activity of AgNPs or AgNPs combined with an antibiotic was evaluated using a series of tests such as in vitro, disk diffusion, biofilm inhibition, and generation of ROS against P. aeruginosa, Shigella flexneri, S. aureus, and Streptococcus pneumoniae. A significant antimicrobial and anti-biofilm activity was observed with antibiotics in combination, with the lowest dose of AgNPs using the plant extract of A. cobbe, and in other cases sublethal doses of antibiotics. A significant synergistic effect was observed for ampicillin and vancomycin against Gram-negative and Gram-positive bacteria, respectively. The study proposed that the biogenic AgNPs in combination with antibiotics can be used for the treatment of infectious bacterial diseases. This study also showed evidence of antibacterial and anti-biofilm properties of AgNPs facilitated by A. cobbe and their resilience against various human pathogenic bacteria, which advocate the use of AgNPs as coadjutants for the treatment of infectious diseases.
Kalishwaralal et alCitation242 proposed that biofilms are formed by the bacteria that attach to the surface and aggregate to form a hydrated polymeric matrix. The formation of these sessile colonies and their inbuilt resistance to antimicrobial agents are a cause of many important and chronic bacterial infections. Such biofilms play a key role in the development of infectious eye disease in humans such as microbial keratitis. Various approaches have been attempted to avert infections related to biofilms in health institutes. These methods have their own limitations such as chemical barriers, emerging antibiotics-resistant strains, and so on. AgNPs are well known for their antimicrobial activity; therefore, in this study, biologically synthesized AgNPs showed an anti-biofilm activity, which was tested in vitro on biofilms formed by P. aeruginosa and S. epidermidis after 24 h of treatment. The treatment resulted in more than 95% inhibition of biofilm formation, and it was uniform in the test species. This study recommends the future application of AgNPs in the treatment of microbial keratitis owing to their anti-biofilm potential.
Monteiro et alCitation243 investigated the effect of AgNPs on the susceptibility of biofilm development in C. albicans and C. glabrata during middle and maturation stages. The AgNP suspensions (5 nm) were synthesized by reducing silver nitrate with sodium citrate solution and were used to treat biofilms of Candida developed after 5, 12 (intermediary phase), and 48 h (maturation phase) of growth on acrylic surfaces. Their efficacy was determined by measuring total biomass (using crystal violet staining) and the number of CFU. The AgNPs caused a significant decline in the total biomass and the number of CFU of Candida biofilms, ranging from 23% to 51.5% and 0.63 to 1.59 log10 (CFU/cm2), respectively. Thereafter, no significant differences were recorded in the total biomass of biofilm on exposure to AgNPs at different developmental stages (24 or 48 h). However, the number of CFU after 24 and 48 h of exposure of the biofilm to AgNPs was significantly different only in C. albicans strain 324LA/94. Generally, the developmental phases (transitional as well as maturation) do not affect the susceptibility of biofilms of C. albicans and C. glabrata to AgNPs. These findings are fundamental to the adoption of new therapies to prevent denture stomatitis. In the future, these nanoparticles can be incorporated into denture base resins in a safer way or may be used as a dental decontamination solution for the elderly people, especially in developing countries.
Qin et alCitation244 examined the prevention of periprosthetic infection via biofilm inhibition by AgNPs (20 nm) produced in situ and fixed on a titanium surface by silver plasma immersion ion implantation. The surface was characterized by FE-SEM and XPS. Spherical nanoparticles were homogeneously distributed on the titanium surface, and an increase in the immersion time led to an increase in the average diameter and distribution of the metallic AgNPs. The AgNPs immobilized on the titanium surface did not show any cytotoxicity against MC3T3-E1 cell line. The immobilized metallic silver particles were tested against biofilms of S. epidermidis (ATCC 35984) on the surface and analyzed by crystal violet staining, spread plate method, CLSM, and SEM, and the results showed an in vitro decrease in biofilm formation via inhibition of bacterial adhesion.
summarizes the results of several studies related to the application of AgNPs most used for control of microbial biofilms.
Table 8 AgNPs for control of microbial biofilms
Gold
Khan et alCitation245 explored gold nanoparticles (AuNPs) to enhance the efficacy of photodynamic therapy by methylene blue against resistant C. albicans and biofilm formation. The AuNPs and AuNP–methylene blue conjugates were characterized by physicochemical methods such as XRD, UV–Vis absorption spectrophotometry, cross-correlation of photons, FTIR, fluorescence spectroscopy, and electron microscopy. The properties of the conjugates were elucidated by several methods such as anti-biofilm testing, laser scanning, confocal, and electron microscopy. The microscopic analysis showed a significant reduction in biofilm formation as well as the activity of C. albicans in the presence of conjugates. Fluoro-spectroscopic analyses confirmed type I phototoxicity against the biofilms. These findings indicate that the conjugated AuNPs generated by photodynamic treatment of methylene blue can be used for the control of acquired nosocomial infection and inhibit the formation of biofilm by C. albicans.
AuNPs (10 nm) were prepared to store and release nitric oxide (NO) by the activation of their surfaces with functional polymers modified with NO donor molecules.Citation246 Initially, the copolymer chains consisting of poly (methyl methacrylate oligo-ethylene glycol ether) and poly (benzyl vinyl chloride) were synthesized via reversible addition fragmentation chain transfer polymerization. The chlorine functional groups reacted with cyclohexylamine to introduce secondary amine groups in the copolymer chains. The surface of AuNPs was coated with the copolymers by exploiting the affinity of the terminal group for gold to reach a thickness of 0.6 nm. The secondary amine functional groups were converted to N-diazenium diolate, NO donor molecules, at high pressure (5 atm). The AuNPs carrying NO were further characterized by TEM, DLS, thermal gravimetric analysis, and XPS. The nanoparticles showed the slow release of NO in biological media. TEM showed an enhancement in the stability of nanoparticles, while DLS confirmed an improved particle size and hydrodynamics. The results concluded that NO can be released slowly at pH 6.8, which can be explored for various applications such as dispersal of P. aeruginosa biofilm and destruction of cancer cells.
Boda et alCitation247 addressed the issue of bacterial resistance to drugs, particularly in relation to staphylococci biofilm. The ultrasmall AuNPs were tested for antibacterial activity against two Gram-positive bacteria, S. aureus and S. epidermidis, and two Gram-negative bacteria, E. coli and P. aeruginosa. The AuNPs, with core diameters of 0.8–1.4 nm, showed MIC and MBC of 25×10−6 m [AU]. The agar disk diffusion test demonstrated higher bactericidal activity of AuNPs and Au. The zone of inhibition showed that S. epidermidis was less susceptible to AuNPs than S. aureus, whereas the reverse was true in the case of kanamycin. However, thiol-stabilized AuNPs having a diameter of 1.9 nm (AuroVist) did not cause significant toxicity in any bacterial strain. The ultrasmall AuNPs caused about 5 log10 reductions in bacterial growth in the first 5 h of exposure and inadequate recovery after 21 h. The biofilm treated with ultrasmall AuNPs showed bubbles and lysis in the bacterial membrane. After 24 h of the treatment of mature biofilms with 50×10−6 m [AU] of ultrasmall AuNPs, a decrease of 80%–90% was recorded in bacterial cell viability. A study to understand the mechanism of antimicrobial action of ultrasmall AuNPs against Gram-positive bacteria is underway. This study demonstrated the potential activity of ultrasmall AuNPs as an effective therapeutic option against staphylococcal infections.
Sathyanarayanan et alCitation248 explained about the challenges in eliminating the microbial biofilms in implant biomaterials or devices by antibiotics. They observed that exopolymeric substances protect the microorganisms from most of the antibiotics and make the cell immune. The applications of MNPs have now been widely considered to control bacterial infections. Gold and iron oxide nanoparticles are extensively used in various medical applications; however, their potential to eradicate biofilms from biomaterial implants is still under investigation. In a recent study, the growth of S. aureus and P. aeruginosa biofilm was significantly inhibited at higher concentrations of AuNPs and iron oxide compared to the control.
summarizes the results of several studies related to the application of AuNPs most used for control of microbial biofilms.
Table 9 AuNPs for control of microbial biofilms
Final considerations
The exploration of novel approaches toward the improvement of human life is everlasting, and it is evident that the search for alternatives for the treatment and control of microbial diseases associated with biofilms is a complex path. According to the scientific reports presented in this review article, it may be concluded that the application of nanotechnology in drug delivery systems has enormous potential and can be considered as an effective alternative for the treatment of microbial biofilms in the near future. The ability of the nanoparticles to synergize the active molecules for the inhibition of biofilms is a promising characteristic as it allows the use of drugs available in clinical practice in a more efficient manner that guarantees overcoming of the constraints related to the bioavailability of the antimicrobials.
Acknowledgments
We thank São Paulo Research Foundation - FAPESP (grant #2014/24626-9 and #2016/08559-5) and the Scientific Support and Development Program of School of Pharmaceutical Sciences (UNESP). We also thank Reinier Bron for their support with the figures.
Disclosure
The authors report no conflicts of interest in this work.
References
- LeventhalGEHillALNowakMABonhoefferSEvolution and emergence of infectious diseases in theoretical and real-world networksNat Commun20156610125592476
- JonesKEPatelNGLevyMAGlobal trends in emerging infectious diseasesNature2008451718199099318288193
- FuxCACostertonJWStewartPSStoodleyPSurvival strategies of infectious biofilmsTrends Microbiol2005131344015639630
- AparnaMSYadavSBiofilms: microbes and diseaseBraz J Infect Dis200812652653019287843
- BjarnsholtTThe role of bacterial biofilms in chronic infectionsAPMIS201312113615823030626
- BurmølleMRenDBjarnsholtTSørensenSJInteractions in multispecies biofilms: do they actually matter?Trends Microbiol2014222849124440178
- CostertonJWLewandowskiZCaldwellDEKorberDRLappin-ScottHMMicrobial biofilmsAnnu Rev Microbiol1995497117458561477
- FlemmingHCWingenderJThe biofilm matrixNat Rev Microbiol20108962363320676145
- XavierJBPicioreanuCAlmeidaJSvan LoosdrechtMCMMonitorização e modelação da estrutura de biofilmes [Monitoring and modeling of biofilm structure]Bol Biotecnol2003761213 Portuguese [with English abstract]
- D’AcuntoBFrunzoLKlapperIMatteiMRModeling multispecies biofilms including new bacterial species invasionMath Biosci2015259202625447810
- AlmeidaMANDe FrançaFPBiofilm formation on brass coupons exposed to a cooling system of an oil refineryJ Ind Microbiol Biotechnol19982013944
- PercivalSLKnappJSEdyveanRGJWalesDSBiofilms, mains water and stainless steelWater Res199832721872201
- PercivalSLImportance of biofilm formation in surgical infectionBr J Surg20171042e85e9428121033
- MyszkaKCzaczykKBacterial biofilms on food contact surfaces – a reviewPol J Food Nutr Sci2011613173180
- WilkinsMHall-StoodleyLAllanRNFaustSNNew approaches to the treatment of biofilm-related infectionsJ Infect201469Suppl 1S47S5225240819
- O’TooleGKaplanHBKolterRBiofilm formation as microbial developmentAnnu Rev Microbiol2000541497911018124
- Castro-RosasJEscartínEFIncreased tolerance of Vibrio cholerae O1 to temperature, pH, or drying associated with colonization of shrimp carapacesInt J Food Microbiol2005102219520115992618
- SimõesMAntimicrobial strategies effective against infectious bacterial biofilmsCurr Med Chem201118142129214521517762
- BlackledgeMSWorthingtonRJMelanderCBiologically inspired strategies for combating bacterial biofilmsCurr Opin Pharmacol201313569970623871261
- DaviesDUnderstanding biofilm resistance to antibacterial agentsNat Rev Drug Discov20032211412212563302
- DunneWMJrBacterial adhesion: seen any good biofilms lately?Clin Microbiol Rev200215215516611932228
- FriedlanderRSVlamakisHKimPKhanMKolterRAizenbergJBacterial flagella explore microscale hummocks and hollows to increase adhesionProc Natl Acad Sci U S A2013110145624562923509269
- Hall-StoodleyLCostertonJWStoodleyPBacterial biofilms: from the natural environment to infectious diseasesNat Rev Microbiol2004229510815040259
- HarsheyRMBacterial motility on a surface: many ways to a common goalAnnu Rev Microbiol200357124927314527279
- HölscherTBartelsBLinYCMotility, chemotaxis and aerotaxis contribute to competitiveness during bacterial pellicle biofilm developmentJ Mol Biol2015427233695370826122431
- PavithraDDobleMBiofilm formation, bacterial adhesion and host response on polymeric implants – issues and preventionBiomed Mater20083303400318689922
- BurrowsLLPseudomonas aeruginosa twitching motility: type IV pili in actionAnnu Rev Microbiol201266149352022746331
- PersatANadellCDKimMKThe mechanical world of bacteriaCell2015161598899726000479
- RochfordETRichardsRGMoriartyTFInfluence of material on the development of device-associated infectionsClin Microbiol Infect201218121162116722925523
- HodgkinsonAJCarpenterEASmithCSMolanPCProsserCGAdhesion molecule expression in the bovine mammary glandVet Immunol Immunopathol20071153–420521517173979
- TrentinDDSGiordaniRBMacedoAJBiofilmes bacterianos patogênicos: aspectos gerais, importância clínica e estratégias de combate [Pathogenic bacterial biofilms: general aspects, clinical importance, and combat strategies]Rev Lib20131422113238 Portuguese [with English abstract]
- LynchASRobertsonGTBacterial and fungal biofilm infectionsAnnu Rev Med200859141542817937586
- RenduelesOGhigoJMMulti-species biofilms: how to avoid unfriendly neighborsFEMS Microbiol Rev201236597298922273363
- DickschatJSQuorum sensing and bacterial biofilmsNat Prod Rep201027334336920179876
- WonnenbergBTschernigTVossMProbenecid reduces infection and inflammation in acute Pseudomonas aeruginosa pneumoniaInt J Med Microbiol20143045–672572924938792
- UlettGCTotsikaMSchaaleKCareyAJSweetMJSchembriMAUropathogenic Escherichia coli virulence and innate immune responses during urinary tract infectionCurr Opin Microbiol201316110010723403118
- OjhaAKHatfullGFBiofilms of Mycobacterium tuberculosis: new perspectives of an old pathogenCardonaPere-JoanUnderstanding Tuberculosis – Deciphering the Secret Life of the BacilliInTech2012182192
- ChenLWenYMThe role of bacterial biofilm in persistent infections and control strategiesInt J Oral Sci201132667321485310
- MarrieTJNelliganJCostertonJWA scanning and transmission electron microscopic study of an infected endocardial pacemaker leadCirculation1982666133913417139907
- RybtkeMHultqvistLDGivskovMTolker-NielsenTPseudomonas aeruginosa biofilm infections: community structure, antimicrobial tolerance and immune responseJ Mol Biol2015427233628364526319792
- HirshDSBloomHLClinical use of antibacterial mesh envelopes in cardiovascular electronic device implantationsMed Devices (Auckl)20158717825624774
- BaddourLMEpsteinAEEricksonCCAmerican Heart Association Rheumatic Fever, Endocarditis, and Kawasaki Disease CommitteeCouncil on Cardiovascular Disease in YoungCouncil on Cardiovascular Surgery and AnesthesiaCouncil on Cardiovascular NursingCouncil on Clinical CardiologyInterdisciplinary Council on Quality of CareAmerican Heart AssociationUpdate on cardiovascular implantable electronic device infections and their management: a scientific statement from the American Heart AssociationCirculation2010121345847720048212
- MadhavanMSohailMRFriedmanPAMayo Cardiovascular Infections Study GroupOutcomes in patients with cardiovascular implantable electronic devices and bacteremia caused by Gram-positive cocci other than Staphylococcus aureusCirc Arrhythm Electrophysiol20103663964520852296
- BruunNEHabibGThunyFSogaardPCardiac imaging in infectious endocarditisEur Heart J2014351062463223900698
- LinTIHuangYFLiuPYPseudomonas aeruginosa infective endocarditis in patients who do not use intravenous drugs: analysis of risk factors and treatment outcomesJ Microbiol Immunol Infect201649451652225442867
- YoonSSHenniganRFHilliardGMPseudomonas aeruginosa anaerobic respiration in biofilms: relationships to cystic fibrosis pathogenesisDev Cell20023459360312408810
- CohenTSPrinceACystic fibrosis: a mucosal immunodeficiency syndromeNat Med201218450951922481418
- GjødsbølKChristensenJJKarlsmarkTJørgensenBKleinBMKrogfeltKAMultiple bacterial species reside in chronic wounds: a longitudinal studyInt Wound J20063322523116984578
- FazliMBjarnsholtTKirketerp-MøllerKQuantitative analysis of the cellular inflammatory response against biofilm bacteria in chronic woundsWound Repair Regen201119338739121518086
- da SilvaPBRamosMABonifácioBVNanotechnological strategies for vaginal administration of drugs–a reviewJ Biomed Nanotechnol20141092218224325992455
- GaydosCABarnesMAumakhanBChlamydia trachomatis age-specific prevalence in women who used an internet-based self-screening program compared to women who were screened in family planning clinicsSex Transm Dis2011382747821173720
- WessmanMBjarnsholtTEickhardt-SørensenSRJohansenHKHomøePMucosal biofilm detection in chronic otitis media: a study of middle ear biopsies from Greenlandic patientsEur Arch Otorhinolaryngol201527251079108524477340
- NgCGLokeMFGohKLVadiveluJHoBBiofilm formation enhances Helicobacter pylori survivability in vegetablesFood Microbiol201762687627889168
- SgourasDNTrangTTHYamaokaYPathogenesis of Helicobacter pylori infectionHelicobacter20152081626372819
- MonnoRFumarolaLCapolongoCSusceptibility of Helicobacter pylori to antibiotics including tigecyclineJ Med Microbiol Diagn2015S5005
- YoonBIKimSHanDSAcute bacterial prostatitis: how to prevent and manage chronic infection?J Infect Chemother201218444445022215226
- WagenlehnerFMPilatzABschleipferTBacterial prostatitisWorld J Urol201331471171623519458
- ZumsteinVBetschartPAlbrichWCBiofilm formation on ureteral stents – incidence, clinical impact and preventionSwiss Med Wkly2017147w1440828165539
- SinghRShivaprakashMRChakrabartiABiofilm formation by zygomycetes: quantification, structure and matrix compositionMicrobiology2011157Pt 92611261821636650
- RizzelloLCingolaniRPompaPPNanotechnology tools for antibacterial materialsNanomedicine (Lond)20138580782123656266
- ChengLZhangKWeirMDMeloMAZhouXXuHHNanotechnology strategies for antibacterial and remineralizing composites and adhesives to tackle dental cariesNanomedicine (Lond)201510462764125723095
- HabimanaOSemiãoAJCCaseyEThe role of cell-surface interactions in bacterial initial adhesion and consequent biofilm formation of nanofiltration/reverse osmosis membranesJ Membr Sci20144548296
- GarrettTRBhakooMZhangZBacterial adhesion and biofilms on surfacesProg Nat Sci200818910491056
- AntasPRBritoMMPeixotoÉPonteCGBorbaCMNeglected and emerging fungal infections: review of hyalohyphomycosis by Paecilomyces lilacinus focusing in disease burden, in vitro antifungal susceptibility and managementMicrobes Infect20121411821907304
- MayerFLWilsonDHubeBCandida albicans pathogenicity mechanismsVirulence20134211912823302789
- NettJEAndesDRFungal biofilms: in vivo models for discovery of anti-biofilm drugsGhannoumMParsekMWhiteleyMMukherjeePMicrobial Biofilms2nd ed3Washington, DCASM Press20153349
- YanagisawaNLiDQLjunghAProtein adsorption on ex vivo catheters and polymers exposed to peritoneal dialysis effluentPerit Dial Int200424326427315185775
- HerwaldSEKumamotoCACandida albicans niche specialization: features that distinguish biofilm cells from commensal cellsCurr Fungal Infect Rep20148217918424839528
- DouglasLJCandida biofilms and their role in infectionTrends Micro-biol20031113036
- KojicEMDarouicheROCandida infections of medical devicesClin Microbiol Rev200417225526715084500
- DesaiJVMitchellAPAndesDRFungal biofilms, drug resistance, and recurrent infectionCold Spring Harb Perspect Med2014410a01972925274758
- ChandraJMukherjeePKGhannoumMACandida biofilms associated with CVC and medical devicesMycoses201255Suppl 14657
- RamageGMowatEJonesBWilliamsCLopez-RibotJOur current understanding of fungal biofilmsCrit Rev Microbiol200935434035519863383
- VelegrakiACafarchiaCGaitanisGIattaRBoekhoutTMalassezia infections in humans and animals: pathophysiology, detection, and treatmentPLoS Pathog2015111e100452325569140
- CushionMTCollinsMSLinkeMJBiofilm formation by Pneumocystis sppEukaryot Cell20098219720618820078
- Carreto-BinaghiLEDamascenoLSPitangui NdeSCould Histoplasma capsulatum be related to healthcare-associated infections?Biomed Res Int2015201598242926106622
- MartinezLRCasadevallABiofilm formation by Cryptococcus neoformansGhannoumMAParsekMWhiteleyMMukherjeePKMicrobial Biofilms2nd edWashington, DCASM Press2015135147
- GibsonJFJohnstonSAImmunity to Cryptococcus neoformans and C. gattii during cryptococcosisFungal Genet Biol201578768625498576
- RamageGRajendranRSherryLWilliamsCFungal biofilm resistanceInt J Microbiol2012201252852122518145
- MiceliMHDíazJALeeSAEmerging opportunistic yeast infectionsLancet Infect Dis201111214215121272794
- MitchellKFZarnowskiRSanchezHCommunity participation in biofilm matrix assembly and functionProc Natl Acad Sci U S A2015112134092409725770218
- TsangPWBandaraHMFongWPPurpurin suppresses Candida albicans biofilm formation and hyphal developmentPLoS One2012711e5086623226409
- SilvaSNegriMHenriquesMOliveiraRWilliamsDWAzeredoJCandida glabrata, Candida parapsilosis and Candida tropicalis: biology, epidemiology, pathogenicity and antifungal resistanceFEMS Microbiol Rev201236228830521569057
- VylkovaSLorenzMCModulation of Phagosomal pH by Candida albicans promotes hyphal morphogenesis and requires Stp2p, a regulator of amino acid transportPLoS Pathog2014103e100399524626429
- HardingMWMarquesLLHowardRJOlsonMECan filamentous fungi form biofilms?Trends Microbiol2009171147548019833519
- EnjalbertBWhitewayMRelease from quorum-sensing molecules triggers hyphal formation during Candida albicans resumption of growthEukaryot Cell2005471203121016002646
- HornbyJMJensenECLisecADQuorum sensing in the dimorphic fungus Candida albicans is mediated by farnesolAppl Environ Microbiol20016772982299211425711
- SemighiniCPHornbyJMDumitruRNickersonKWHarrisSDFarnesol-induced apoptosis in Aspergillus nidulans reveals a possible mechanism for antagonistic interactions between fungiMol Microbiol200659375376416420349
- ScheperMAShirtliffMEMeillerTFPetersBMJabra-RizkMAFarnesol, a fungal quorum-sensing molecule triggers apoptosis in human oral squamous carcinoma cellsNeoplasia200810995496318714396
- DichtlKEbelFDirrFRoutierFHHeesemannJWagenerJFarnesol misplaces tiplocalized Rho proteins and inhibits cell wall integrity signalling in Aspergillus fumigatusMol Microbiol20107651191120420398212
- SharmaMPrasadRThe quorum-sensing molecule farnesol is a modulator of drug efflux mediated by ABC multidrug transporters and synergizes with drugs in Candida albicansAntimicrob Agents Chemother201155104834484321768514
- LeonhardtISpielbergSWeberMThe fungal quorum-sensing molecule farnesol activates innate immune cells but suppresses cellular adaptive immunityMBio201562e0014325784697
- ChenHFujitaMFengQClardyJFinkGRTyrosol is a quorum-sensing molecule in Candida albicansProc Natl Acad Sci U S A2004101145048505215051880
- HarriottMMLillyEARodriguezTEFidelPLNoverrMCCandida albicans forms biofilms on the vaginal mucosaMicrobiology2010156Pt 123635364420705667
- NobileCJJohnsonADCandida albicans biofilms and human diseaseAnnu Rev Microbiol2015691719226488273
- PappasPGInvasive candidiasisInfect Dis Clin North Am200620348550616984866
- CampionEWKullbergBJArendrupMCInvasive candidiasisN Engl J Med2015373151445145626444731
- Sardi JdeCPitangui NdeSRodríguez-ArellanesGTaylorMLFusco-AlmeidaAMMendes-GianniniMJHighlights in pathogenic fungal biofilmsRev Iberoam Micol2014311222924252828
- CaggianoGCorettiCBartolomeoNLoveroGDe GiglioOMontagnaMTCandida bloodstream infections in Italy: changing epidemiology during 16 years of surveillanceBiomed Res Int2015201525658026064890
- RongpharpiSRGurRDuggalSCandida krusei fungemia in 7 neonates: clonality tracked to an infusateAm J Infect Control201442111247124825444273
- SpanakisEKAperisGMylonakisENew agents for the treatment of fungal infections: clinical efficacy and gaps in coverageClin Infect Dis20064381060106816983621
- SobelJDRecurrent vulvovaginal candidiasisAm J Obstet Gynecol20162141152126164695
- GemmillJAStrattonPClearySDBallwegMLSinaiiNCancers, infections, and endocrine diseases in women with endometriosisFertil Steril20109451627163119945097
- AulerMEMorreiraDRodriguesFFBiofilm formation on intrauterine devices in patients with recurrent vulvovaginal candidiasisMed Mycol201048121121620055746
- AderFInvasive pulmonary aspergillosis in patients with chronic obstructive pulmonary disease: an emerging fungal diseaseCurr Infect Dis Rep201012640941621308548
- DimopoulosGFrantzeskakiFPoulakouGArmaganidisAInvasive aspergillosis in the intensive care unitAnn N Y Acad Sci201212721313923231712
- BakareNRickertsVBargonJJust-NüblingGPrevalence of Aspergillus fumigatus and other fungal species in the sputum of adult patients with cystic fibrosisMycoses2003461–21923
- BohseMLWoodsJPRNA interference-mediated silencing of the YPS3 gene of Histoplasma capsulatum reveals virulence defectsInfect Immun20077562811281717403872
- Suárez-AlvarezROPérez-TorresATaylorMLAdherence patterns of Histoplasma capsulatum yeasts to bat tissue sectionsMycopathologia20101702798720340046
- PitanguiNSSardiJCSilvaJFAdhesion of Histoplasma capsulatum to pneumocytes and biofilm formation on an abiotic surfaceBiofouling201228771171822784100
- Martin-GarridoICarmonaEMSpecksULimperAHPneumocystis pneumonia in patients treated with rituximabChest2013144125826523258406
- HuangLCattamanchiADavisJLInternational HIV-associated Opportunistic Pneumonias (IHOP) StudyLung HIV StudyHIV-associated Pneumocystis pneumoniaProc Am Thorac Soc20118329430021653531
- ChayakulkeereeMPerfectJRCryptococcosisInfect Dis Clin North Am2006203507544vvi16984867
- HernándezYUMonzónJFDelgado-HernándezRSoto-HernándezJLCárdenasGCryptococcoma of the brain in an immunocompetent manNatl Med J India201326421621724758445
- LiaoYLuXYangSLuoYChenQYangREpidemiology and outcome of Trichosporon fungemia: a review of 185 reported cases from 1975 to 2014Open Forum Infect Dis201524 Epub925
- Iturrieta-GonzálezIAPadovanACBizerraFCHahnRCColomboALMultiple species of Trichosporon produce biofilms highly resistant to triazoles and amphotericin BPLoS One2014910e10955325360765
- de PaviaFagundesJúniorAAde CarvalhoRTFocacciaREmergência de infecção por Trichosporon Asahii em pacientes portadores de insuficiência cardíaca em unidade de terapia intensiva cardiológica: relato de caso e revisão da literatura [Trichosporon asahii an emerging etiologic agent of fungal infection and colonization in heart failure patients in intensive care unit: case report and literature review]Rev Bras Ter Intensiva2008201106109 Portuguese [with English abstract]25306958
- MattedeMGSPirasCMattedeKDSFerrariATBaldottoLSAssbuMSZUrinary tract infections due to Trichosporon spp. in severely ill patients in an intensive care unitRev Bras Ter Intensiva201527324725126465246
- RömlingUBalsalobreCBiofilm infections, their resilience to therapy and innovative treatment strategiesJ Intern Med2012272654156123025745
- RömlingUKjellebergSNormarkSNymanLUhlinBEÅkerlundBMicrobial biofilm formation: a need to actJ Intern Med201427629811024796496
- DiciccoMNeethirajanSSinghAWeeseJSEfficacy of clarithro-mycin on biofilm formation of methicillin-resistant Staphylococcus pseudintermediusBMC Vet Res2012822523171620
- De OliveiraMMMBrugneraDFPicolliRHBiofilmes microbianos na indústria de alimentos: uma revisão [Microbial biofilms in the food industry: a review]Rev Inst Adolfo Lutz2010693277284 Portuguese [with English abstract]
- GilbertPAllisonDGMcBainAJBiofilms in vitro and in vivo: do singular mechanisms imply cross-resistance?J Appl Microbiol200292Suppl98S110S12000619
- RomaníAMFundKArtigasJSchwartzTSabaterSObstURelevance of polymeric matrix enzymes during biofilm formationMicrob Ecol200856342743618227962
- YangYLChenMGuJLCryptococcosis in kidney transplant recipients in a Chinese university hospital and a review of published casesInt J Infect Dis20142615416125063020
- HøibyNCiofuOJohansenHKThe clinical impact of bacterial biofilmsInt J Oral Sci201132556521485309
- SmithAWBiofilms and antibiotic therapy: is there a role for combating bacterial resistance by the use of novel drug delivery systems?Adv Drug Deliv Rev200557101539155015950314
- SotoSMRole of efflux pumps in the antibiotic resistance of bacteria embedded in a biofilmVirulence20134322322923380871
- MahTFBiofilm-specific antibiotic resistanceFuture Microbiol2012791061107222953707
- PodneckyNLRhodesKASchweizerHPEfflux pump-mediated drug resistance in BurkholderiaFront Microbiol2015630525926825
- SchlisselbergDBKlerEKislukGShacharDYaronSBiofilm formation ability of Salmonella enterica serovar Typhimurium acrAB mutantsInt J Antimicrob Agents201546445645926260191
- GillisRJWhiteKGChoiK-HWagnerVESchweizerHPIglewskiBHMolecular basis of azithromycin-resistant Pseudomonas aeruginosa biofilmsAntimicrob Agents Chemother20054993858386716127063
- Van AckerHVan DijckPCoenyeTMolecular mechanisms of antimicrobial tolerance and resistance in bacterial and fungal biofilmsTrends Microbiol201422632633324598086
- PrasadRGoffeauAYeast ATP-binding cassette transporters conferring multidrug resistanceAnnu Rev Microbiol2012661396322703054
- MateusCCrowSAJrAhearnDGAdherence of Candida albicans to silicone induces immediate enhanced tolerance to fluconazoleAntimicrob Agents Chemother20044893358336615328097
- TanAChawlaRGNNanotechnology and regenerative therapeutics in plastic surgery: the next frontierJ Plast Reconstr Aesthet Surg201669111326422652
- LinTCHungKHPengCHNanotechnology-based drug delivery treatments and specific targeting therapy for age-related macular degenerationJ Chin Med Assoc2015781163564126383186
- BarkalinaNCharalambousCJonesCCowardKNanotechnology in reproductive medicine: emerging applications of nanomaterialsNanomedicine201410592193824444494
- BharaliDJSiddiquiIAAdhamiVMNanoparticle delivery of natural products in the prevention and treatment of cancers: current status and future prospectsCancers (Basel)2011344024404524213123
- PelgriftRYFriedmanAJNanotechnology as a therapeutic tool to combat microbial resistanceAdv Drug Deliv Rev20136513–141803181523892192
- MartinCLowWLGuptaAStrategies for antimicrobial drug delivery to biofilmCurr Pharm Des2015211436625189862
- ForierKRaemdonckKDe SmedtSCDemeesterJCoenyeTBraeckmansKLipid and polymer nanoparticles for drug delivery to bacterial biofilmsJ Control Release201419060762324794896
- SinghRNadheSWadhwaniSShedbalkarUChopadeBANanoparticles for control of biofilms of Acinetobacter speciesMaterials (Basel)201695E38328773507
- Al-JamalWTKostarelosKLiposomes: from a clinically established drug delivery system to a nanoparticle platform for theranostic nanomedicineAcc Chem Res201144101094110421812415
- AkbarzadehARezaei-SadabadyRDavaranSLiposome: classification, preparation, and applicationsNanoscale Res Lett20138110223432972
- AhmedKMuiruriPWJonesGHScottMJJonesMNThe effect of grafted poly(ethylene glycol) on the electrophoretic properties of phospholipid liposomes and their adsorption to bacterial biofilmsColloids Surf A Physicochem Eng Asp20011941–3287296
- RobinsonAMBannisterMCreethJEJonesMNThe interaction of phospholipid liposomes with mixed bacterial biofilms and their use in the delivery of bactericideColloids Surf A Physicochem Eng Asp20011861–24353
- CatuognoCJonesMNThe antibacterial properties of solid supported liposomes on Streptococcus oralis biofilmsInt J Pharm20032571–212514012711168
- Drulis-KawaZDorotkiewicz-JachAGubernatorJGulaGBocerTDoroszkiewiczWThe interaction between Pseudomonas aeruginosa cells and cationic PC:Chol:DOTAP liposomal vesicles versus outer-membrane structure and envelope properties of bacterial cellInt J Pharm20093671–221121918952159
- GubernatorJDrulis-KawaZDorotkiewicz-JachADoroskiewieczWKozubekAIn vitro antimicrobial activity of liposomes containing ciprofloxacin, meropenem and gentamicin against gram-negative clinical bacterial strainsLett Drug Des Discov200744297304
- OmriASuntresZEShekPNEnhanced activity of liposomal polymyxin B against Pseudomonas aeruginosa in a rat model of lung infectionBiochem Pharmacol20026491407141312392822
- RukholmGMugabeCAzghaniAOOmriAAntibacterial activity of liposomal gentamicin against Pseudomonas aeruginosa: a time-kill studyInt J Antimicrob Agents200627324725216472992
- MugabeCAzghaniAOOmriALiposome-mediated gentamicin delivery: development and activity against resistant strains of Pseudomonas aeruginosa isolated from cystic fibrosis patientsJ Antimicrob Chemother200555226927115590716
- AlipourMSuntresZEOmriAImportance of DNase and alginate lyase for enhancing free and liposome encapsulated aminoglycoside activity against Pseudomonas aeruginosaJ Antimicrob Chemother200964231732519465435
- AlhajlanMAlhaririMOmriAEfficacy and safety of liposomal clarithromycin and its effect on Pseudomonas aeruginosa virulence factorsAntimicrob Agents Chemother20135762694270423545534
- AlipourMHalwaniMOmriASuntresZEAntimicrobial effectiveness of liposomal polymyxin B against resistant Gram-negative bacterial strainsInt J Pharm20083551–229329818164881
- AlipourMSuntresZELafrenieRMOmriAAttenuation of Pseudomonas aeruginosa virulence factors and biofilms by co-encapsulation of bismuth-ethanedithiol with tobramycin in liposomesJ Antimicrob Chemother201065468469320159770
- BonifácioBVSilvaPBRamosMANegriKMBauabTMChorilliMNanotechnology-based drug delivery systems and herbal medicines: a reviewInt J Nanomedicine20139111524363556
- RyanRAltriaKMcEvoyEDoneganSPowerJA review of developments in the methodology and application of microemulsion electrokinetic chromatographyElectrophoresis201334115917723161220
- LawrenceMJReesGDMicroemulsion-based media as novel drug delivery systemsAdv Drug Deliv Rev20004518912111104900
- MuzaffarFSinghUKChauhanLReview on microemulsion as futuristic drug deliveryInt J Pharm Pharm Sci2013533953
- Al-AdhamISAshourHAl-KaissiEKhalilEKieransMCollierPJStudies on the kinetics of killing and the proposed mechanism of action of microemulsions against fungiInt J Pharm2013454122623223830945
- SinghPKKashif IqubalMShuklaVKShuaibMMicroemulsions: current trends in novel drug delivery systemsJ Pharm Chem Biol Sci2014113951
- MishraAPanolaRRanaACMicroemulsions: as drug delivery systemJ Sci Innov Res201434467474
- HungCFFangCLLiaoMHFangJYThe effect of oil components on the physicochemical properties and drug delivery of emulsions: tocol emulsion versus lipid emulsionInt J Pharm20073351–219320217129692
- AntonNVandammeTFNano-emulsions and micro-emulsions: clarifications of the critical differencesPharm Res201128597898521057856
- BruxelFLauxMWildLBFragaMKoesterLSTexeiraHFNanoemulsão como sistemas de liberação parenteral de fármacos [Nanoemulsions as parenteral drug delivery systems]Quim Nova201235918271840 Portuguese [with English asbtract]
- RamalingamKAmaechiBTRalphRHLeeVAAntimicrobial activity of nanoemulsion on cariogenic planktonic and biofilm organismsArch Oral Biol2012571152221807359
- RamalingamKFrohlichNCLeeVAEffect of nanoemulsion on dental unit waterline biofilmJ Dent Sci201383333336
- TeixeiraPCLeiteGMDominguesRJSilvaJGibbsPAFerreiraJPAntimicrobial effects of a microemulsion and a nanoemulsion on enteric and other pathogens and biofilmsInt J Food Microbiol20071181151917610974
- LiuCHHuangHYAntimicrobial activity of curcumin-loaded myristic acid microemulsions against Staphylococcus epidermidisChem Pharm Bull (Tokyo)20126091118112422976319
- Al-AdhamISAl-HmoudNDKhalilEKieransMCollierPJMicroemulsions are highly effective anti-biofilm agentsLett Appl Microbiol20033629710012535129
- VilliersASur la fermentation de la fécule par l’action du ferment butyrique [The fermentation of starch by the action of butyric]Compt Rend Acad Sci1891112536538 French [with English abstract]
- ZhangJMaPXCyclodextrin-based supramolecular systems for drug delivery: recent progress and future perspectiveAdv Drug Deliv Rev20136591215123323673149
- Morin-CriniNCriniGEnvironmental applications of water-insoluble β-cyclodextrin–epichlorohydrin polymersProg Polym Sci2013382344368
- MartinsMRFMVeigaFPromotores de permeação para a liberação transdérmica de fármacos: uma nova aplicação para as ciclodextrinas [Permeation promoters for transdermal drug delivery: a new application for cyclodextrins]Rev Bras Ciênc Farm20023813354 Portuguese [with English abstract]
- GharbiAHumblotVTurpinFPradierCMImbertCBerjeaudJMElaboration of antibiofilm surfaces functionalized with antifungal-cyclodextrin inclusion complexesFEMS Immunol Med Microbiol201265225726922268719
- SzenteLSzemánJSohajdaTAnalytical characterization of cyclo-dextrins: history, official methods and recommended new techniquesJ Pharm Biomed Anal201613034736527246683
- Acuña-RougierCOlea-AzarCThermodynamic and geometric study of diasteroisomeric complexes formed by racemic flavanones and three cyclodextrins through NMRJ Incl Phenom Macrocycl Chem2013751–2119136
- Folch-CanoCYazdani-PedramMOlea-AzarCInclusion and func-tionalization of polymers with cyclodextrins: current applications and future prospectsMolecules2014199140661407925207713
- Otero-EspinarFJTorres-LabandeiraJJAlvarez-LorenzoCBlanco-MéndezJCyclodextrins in drug delivery systemsJ Drug Deliv Sci Technol2010204289301
- LakkakulaJRMaçedo KrauseRWA vision for cyclodextrin nano-particles in drug delivery systems and pharmaceutical applicationsNanomedicine (Lond)20149687789424981652
- LoftssonTDucheneDCyclodextrins and their pharmaceutical applicationsInt J Pharm20073291–211117137734
- MatsudaHArimaHCyclodextrins in transdermal and rectal deliveryAdv Drug Deliv Rev1999361819910837710
- PinhoEGrootveldMSoaresGHenriquesMCyclodextrins as encapsulation agents for plant bioactive compoundsCarbohydr Polym201410112113524299757
- LulaIDenadaiÂLResendeJMStudy of angiotensin-(1–7) vasoactive peptide and its β-cyclodextrin inclusion complexes: complete sequence-specific NMR assignments and structural studiesPeptides200728112199221017904691
- CortésMEBonillaJCSinisterraRDBiofilm formation, control and novel strategies for eradicationMendez-VilasAScience Against Microbial Pathogens: Communicating Current Research and Technological AdvancesBadajozFormatex Research Center2011896905
- HealingGSulemannTCottonPSafety data on 19 vehicles for use in 1 month oral rodent pre-clinical studies: administration of hydroxypropyl-β-cyclodextrin causes renal toxicityJ Appl Toxicol201636114015025959454
- SuvarnaVGujarPMurahariMComplexation of phytochemicals with cyclodextrin derivatives – an insightBiomed Pharmacother2017881122114428208574
- GharibRGreige-GergesHFourmentinSCharcossetCAuezovaLLiposomes incorporating cyclodextrin-drug inclusion complexes: current state of knowledgeCarbohydr Polym201512917518626050903
- HolmROlesenNEHartvigRAJørgensenEBLarsenDBWesthPEffect of cyclodextrin concentration on the oral bioavailability of danazol and cinnarizine in ratsEur J Pharm Biopharm201610191426776271
- TrottaFDianzaniCCalderaFMognettiBCavalliRThe application of nanosponges to cancer drug deliveryExpert Opin Drug Deliv201411693194124811423
- FreiresIDAMurataRMFurlettiVFCoriandrum sativum L. (Coriander) essential oil: antifungal activity and mode of action on Candida spp., and molecular targets affected in human whole-genome expressionPLoS One201496e9908624901768
- HusainFMAhmadIKhanMSSub-MICs of Mentha piperita essential oil and menthol inhibits AHL mediated quorum sensing and biofilm of Gram-negative bacteriaFront Microbiol2015642026029178
- AzzimontiBCochisABeyrouthyMEEssential oil from berries of lebanese Juniperus excelsa M. Bieb displays similar antibacterial activity to chlorhexidine but higher cytocompatibility with human oral primary cellsMolecules20152059344935726007187
- dos SantosJFTorres-LabandeiraJJMatthijsNCoenyeTConcheiroAAlvarez-LorenzoCFunctionalization of acrylic hydrogels with α-, β- or γ-cyclodextrin modulates protein adsorption and antifungal deliveryActa Biomater20106103919392620417319
- Garcia-FernandezMJBrackmanGCoenyeTConcheiroAAlvarez-LorenzoCAntiseptic cyclodextrin-functionalized hydrogels and gauzes for loading and delivery of benzalkonium chlorideBiofouling201329326127123439005
- IordacheFGrumezescuVGrumezescuAMGamma-cyclodextrin/usnic acid thin film fabricated by MAPLE for improving the resistance of medical surfaces to Staphylococcus aureus colonizationAppl Surf Sci2015336407412
- BrackmanGGarcia-FernandezMJLenoirJDressings loaded with cyclodextrin-hamamelitannin complexes increase Staphylococcus aureus susceptibility toward antibiotics both in single as well as in mixed biofilm communitiesMacromol Biosci201616685986926891369
- FidaleoMZuorroALavecchiaREnhanced antibacterial and anti-quorum sensing activities of triclosan by complexation with modified β-cyclodextrinsWorld J Microbiol Biotechnol20132991731173623536199
- AlayandeABKimLHKimISCleaning efficacy of hydroxypropyl-beta-cyclodextrin for biofouling reduction on reverse osmosis membranesBiofouling201632435937026923225
- Shanmuga PriyaASivakamavalliJVaseeharanBStalinTImprovement on dissolution rate of inclusion complex of Rifabutin drug with β-cyclodextrinInt J Biol Macromol20136247248024076034
- LoftssonTBrewsterMECyclodextrins as functional excipients: methods to enhance complexation efficiencyJ Pharm Sci201210193019303222334484
- JaiswalSBhattacharyaKMcHalePDuffyBDual effects of β-cyclodextrin-stabilised silver nanoparticles: enhanced biofilm inhibition and reduced cytotoxicityJ Mater Sci Mater Med2015261536725596861
- OpreaAEPandelLMDumitrescuAMBioactive ZnO coatings deposited by MAPLE–an appropriate strategy to produce efficient anti-biofilm surfacesMolecules2016212220
- Nava-OrtizCABBurilloGConcheiroACyclodextrin-functionalized biomaterials loaded with miconazole prevent Candida albicans biofilm formation in vitroActa Biomater2010641398140419874920
- RuizHKSerranoDRDea-AyuelaMANew amphotericin B-gamma cyclodextrin formulation for topical use with synergistic activity against diverse fungal species and Leishmania sppInt J Pharm20144731–214815724998510
- EkambaramPSathaliAAHPriyankaKSolid lipid nanoparticles: a reviewSci Rev Chem Commun20122180102
- SinghBBandyopadhyaySKapilRKatareOPNovel nanostructured lipidic drug delivery systemsPharma Rev2009742118122
- SinghBVuddandaPRMRVKumarVSaxenaPSSinghSCefu-roxime axetil loaded solid lipid nanoparticles for enhanced activity against S. aureus biofilmColloids Surf B Biointerfaces2014121929824945607
- NafeeNHusariAMaurerCKAntibiotic-free nanotherapeutics: ultra-small, mucus-penetrating solid lipid nanoparticles enhance the pulmonary delivery and anti-virulence efficacy of novel quorum sensing inhibitorsJ Control Release201419213114024997276
- TaylorENKummerKMDyondiDWebsterTJBanerjeeRMulti-scale strategy to eradicate Pseudomonas aeruginosa on surfaces using solid lipid nanoparticles loaded with free fatty acidsNanoscale20146282583224264141
- Quintanar-GuerreroDAllémannEDoelkerEFessiHPreparation and characterization of nanocapsules from preformed polymers by a new process based on emulsification-diffusion techniquePharm Res1998157105610629688060
- SchaffazickSRGuterresSSCaracterização e estabilidade físico-química de sistemas poliméricos nanoparticulados para administração de fármacos [Characterization and physico-chemical stability of nanoparticulate polymer systems for administration of drugs]Quim Nova2006265726737 Portuguese [with English abstract]
- PlapiedLDuhemNdes RieuxAPréatVFate of polymeric nanocarriers for oral drug deliveryCurr Opin Colloid Interface Sci2011163228237
- ReisCPNeufeldRJRibeiroAJVeigaFNanoencapsulation I. Methods for preparation of drug-loaded polymeric nanoparticlesNanomedicine20062182117292111
- ShafferCNanomedicine transforms drug deliveryDrug Discov Today20051023–2415811582
- Da SilvaLFinerYFriedmanSBasraniBKishenABiofilm formation within the interface of bovine root dentin treated with conjugated chitosan and sealer containing chitosan nanoparticlesJ Endod201339224925323321239
- AbdelghanySMQuinnDJIngramRJGentamicin-loaded nano-particles show improved antimicrobial effects towards Pseudomonas aeruginosa infectionInt J Nanomedicine201274053406322915848
- CaiJHuangHSongWPreparation and evaluation of lipid polymer nanoparticles for eradicating H. pylori biofilm and impairing antibacterial resistance in vitroInt J Pharm2015495272873726417849
- TakahashiCOgawaNKawashimaYYamamotoHObservation of antibacterial effect of biodegradable polymeric nanoparticles on Staphylococcus epidermidis biofilm using FE-SEM with an ionic liquidMicroscopy (Oxf)201564316918025757698
- FaradayMThe Bakerian lecture: experimental relations of gold (and other metals) to lightPhilos Trans R Soc Lond1857147145181
- HorvathHGustav Mie and the scattering and absorption of light by particles: historic developments and basicsJ Quant Spectrosc Radiat Transf200911011787799
- MeloMAJrSantosLSSGonçalves M doCNogueiraAFPreparação de nanopartículas de prata e ouro: um método simples para a introdução da nanociência em laboratório de ensino [Preparation of silver and gold nanoparticles: a simple method to introduce nanotechnology into teaching laboratories]Quím Nova201235918721878 Portuguese [with English abstract]
- KlabundeKJFrontmatter and index Nanoscale Materials in ChemistryNew YorkJohn Wiley & Sons, Inc2001ixi
- KumarMVarshneyLFrancisSRadiolytic formation of Ag clusters in aqueous polyvinyl alcohol solution and hydrogel matrixRadiat Phys Chem20057312127
- XuXLYinYDGeXWWuHKZhangZHγ-Radiation synthesis of poly(acrylic acid)–metal nanocompositesMater Lett199837354358
- KhanSTAhamedMAl-KhedhairyAMusarratJBiocidal effect of copper and zinc oxide nanoparticles on human oral microbiome and biofilm formationMater Lett2013976770
- KhanSTAhamedMMusarratJAl-KhedhairyAAAnti-biofilm and antibacterial activities of zinc oxide nanoparticles against the oral opportunistic pathogens Rothia dentocariosa and Rothia mucilaginosaEur J Oral Sci2014122639740325311638
- SanganiMHMoghaddamMNForghanifardMMInhibitory effect of zinc oxide nanoparticles on pseudomonas aeruginosa biofilm formationNanomedicine J201522121128
- DwivediSWahabRKhanFMishraYKMusarratJAl-KhedhairyAAReactive oxygen species mediated bacterial biofilm inhibition via zinc oxide nanoparticles and their statistical determinationPLoS One2014911e11128925402188
- SeilJTWebsterTJReduced Staphylococcus aureus proliferation and biofilm formation on zinc oxide nanoparticle PVC composite surfacesActa Biomater2011762579258421421087
- LeeJHKimYGChoMHLeeJZnO nanoparticles inhibit Pseudomonas aeruginosa biofilm formation and virulence factor productionMicrobiol Res20141691288889624958247
- PrattLAKolterRGenetic analysis of Escherichia coli biofilm formation: roles of flagella, motility, chemotaxis and type I piliMol Microbiol19983022852939791174
- HaghighiFMohammadiSRMohammadiPHosseinkhaniSAnti-fungal activity of TiO2 nanoparticles and EDTA on Candida albicans BiofilmsInfect Epidemiol Med2013113338
- IbrahemKHSalmanJASAliFAEffect of titanium nanoparticles biosynthesis by Lactobacillus crispatus on urease, hemolysin & biofilm forming by some bacteria causing recurrent UTI in Iraqi womenEur Sci J2014109324338
- Maurer-JonesMAGunsolusILMeyerBMChristensonCJHaynesCLImpact of TiO2 nanoparticles on growth, biofilm formation, and flavin secretion in Shewanella oneidensisAnal Chem201385125810581823701037
- NamasivayamSKRChristoBBArasuSMKKumarKAMDeepakKAnti biofilm effect of biogenic silver nanoparticles coated medical devices against biofilm of clinical isolate of Staphylococcus aureusGlob J Med Res2013133 Epub624
- ParkHParkSRohJBiofilm-inactivating activity of silver nanoparticles: a comparison with silver ionsJ Ind Eng Chem2013192614619
- Martinez-GutierrezFBoegliLAgostinhoAAnti-biofilm activity of silver nanoparticles against different microorganismsBiofouling201329665166023731460
- GurunathanSHanJWKwonDNKimJHEnhanced antibacterial and anti-biofilm activities of silver nanoparticles against Gram-negative and Gram-positive bacteriaNanoscale Res Lett20149137325136281
- KalishwaralalKBarathManiKanthSPandianSRDeepakVGurunathanSSilver nanoparticles impede the biofilm formation by Pseudomonas aeruginosa and Staphylococcus epidermidisColloids Surf B Biointerfaces201079234034420493674
- MonteiroDRTakamiyaASFeresinLPSusceptibility of Candida albicans and Candida glabrata biofilms to silver nanoparticles in intermediate and mature development phasesJ Prosthodont Res2015591424825168655
- QinHCaoHZhaoYIn vitro and in vivo anti-biofilm effects of silver nanoparticles immobilized on titaniumBiomaterials201435339114912525112937
- KhanSAlamFAzamAKhanAUGold nanoparticles enhance methylene blue-induced photodynamic therapy: a novel therapeutic approach to inhibit Candida albicans biofilmInt J Nanomedicine201273245325722802686
- DuongHTTAdnanNNMBarraudNFunctional gold nano-particles for the storage and controlled release of nitric oxide: applications in biofilm dispersal and intracellular deliveryJ Mater Chem B201423150035011
- BodaSKBrodaJSchieferFCytotoxicity of ultrasmall gold nanoparticles on planktonic and biofilm encapsulated gram-positive staphylococciSmall201511263183319325712910
- SathyanarayananMBBalachandranathRGenji SrinivasuluYKannaiyanSKSubbiahdossGThe effect of gold and iron-oxide nanoparticles on biofilm-forming pathogensISRN Microbiol2013201327208624187645