Abstract
Despite recent advances in targeted therapies and immunotherapies, chemotherapy using cytotoxic agents remains an indispensable modality in cancer treatment. Recently, there has been a growing emphasis in using nanomedicine in cancer chemotherapy, and several nanomedicines have already been used clinically to treat cancers. There is evidence that formulating small molecular cancer chemotherapeutic agents into nanomedicines significantly modifies their pharmacokinetics and often improves their efficacy. Importantly, cancer cells often develop resistance to chemotherapy, and formulating anticancer drugs into nanomedicines also helps overcome chemoresistance. In this review, we briefly describe the different classes of cancer chemotherapeutic agents, their mechanisms of action and resistance, and evidence of overcoming the resistance using nanomedicines. We then emphasize on gemcitabine and our experience in discovering the unique (stearoyl) gemcitabine solid lipid nanoparticles that are effective against tumor cells resistant to gemcitabine and elucidate the underlying mechanisms. It seems that lysosomes, which are an obstacle in the delivery of many drugs, are actually beneficial for our (stearoyl) gemcitabine solid lipid nanoparticles to overcome tumor cell resistance to gemcitabine.
Nanomedicine and cancer chemotherapy
Cancer is a major public health problem worldwide and the second most common cause of death.Citation1,Citation2 Cancer chemotherapy, the treatment of cancer with one or a combination of chemotherapeutic agents, is one of the mainstream anticancer therapies.Citation3–Citation5 Nanomedicines are nanometer-sized medicinal entities. They are actively explored to diagnose, prevent, or treat cancer.Citation6 Indeed, a few nanomedicines have already been approved by the United States Food and Drug Administration for cancer treatment and more are currently in various stages of preclinical and clinical development.Citation7 Compared to conventional formulations/medicines, nanomedicines have numerous advantages; for example, they can exhibit prolonged systemic circulation time, sustained drug release kinetics, and increased tumor accumulation.Citation8,Citation9 Nanomedicines can be prepared using various materials, including liposomes, micelles, polymeric nanoparticles, solid lipid nanoparticles, inorganic nanoparticles, drug–polymer conjugates, drug–antibody conjugates, and supramolecular vesicular aggregates, etc.
Cancer chemotherapeutic agents and mechanisms of chemoresistance
The first modern cancer chemotherapeutic agent was discovered serendipitously. During World War I (1914–1918), accidental releases of mustard gas led to the discovery of the effect of nitrogen mustard on lymphoma.Citation10 Historically, anti-cancer drugs were derived from available chemical sources. Synthetic molecules from the chemical industry, in particular dyestuffs and chemical warfare agents, and natural products from plants, bacteria, and fungi are all sources of anticancer agents.Citation11 The breadth of cancer chemotherapeutic agents is vast, which is actually beneficial as most cancer patients receive multi-drug regimens. This is due to the inherent complexity of cancerCitation12 – a non-responder to one chemotherapeutic agent may respond to another. In this review, we focus on traditional cytotoxic chemotherapeutic drugs. Despite the increasing desire by cancer patients for targeted therapies and immunotherapies with reduced adverse effects, cytotoxic drugs still play an indispensable role in systemic cancer therapy, and for many cancers, targeted therapy is not available.
Tumor chemoresistance is a major clinical obstacle to successful tumor therapy.Citation13 Tumor chemoresistance can be divided into intrinsic resistance and acquired resistance.Citation14 Intrinsic resistance indicates that before receiving chemotherapy, resistance factors already pre-exist in tumor cells. Acquired resistance develops during treatment.Citation14,Citation15 Cancer cell resistance to chemotherapy is the main cause of recurrence or relapse and has gained clinical attention.Citation4 Cancer cells evade chemotherapy efficiently through a number of different mechanisms and strategies, such as decrease in drug uptake, increase in drug efflux, alteration of drug metabolism, activation of DNA repair pathways, and induction of the anti-apoptotic machinery.Citation14,Citation16,Citation17 In addition, it is increasingly recognized that the tumor microenvironment plays a critical role in tumor cell response, or lack of response, to chemotherapy.Citation18
Cytotoxic chemotherapeutic drugs can be roughly divided into alkylating agents, antimetabolites, natural products, hormones and hormone antagonists, and other miscellaneous agents.Citation10,Citation12,Citation19
Alkylating agents
Alkylating agents are commonly used as cancer chemotherapeutic agents and have a long history of clinical applications. Alkylating agents, including carmustine, lomustine, and temozolomide, can easily cross the blood–brain barrier and have thus shown the most activity against malignant glioma.Citation20 The general mode of action of alkylating agents is the in vivo formation of electron-deficient active intermediates, which are highly unstable and form covalent bonds with DNA bases. The most vulnerable to attack is the 7-N-atom of guanine.Citation21,Citation22 Moreover, alkylating agents can react with other molecules to produce extensive cellular damages.
The cytotoxicity of alkylating agents depends on DNA repair pathways, and thus enhancing DNA-repair capacity can lead to tumor resistance to alkylating agents.Citation23 Mechanisms of resistance to alkylating agents mainly involve O6-methylguanine methyltransferase (MGMT), DNA mismatch repair (MMR) pathway, and base excision repair (BER) pathway. One important mechanism of resistance to alkylating agents is mediated by the DNA repair enzyme MGMT, which repairs O6-methylguanine adducts.Citation20 MGMT covalently transfers the methyl group from O6-methylguanine to an internal cysteine residue, yielding an inactive S-alkylcysteine-modified protein and guanine.Citation24 The effects of alkylating agents on DNA can be repaired by MGMT, leading to alkylating agent resistance. DNA MMR pathway is critical for mediating the cytotoxic effect of O6-methylguanine, which is programed to correct errors in DNA base pairing, and defects in this system cause resistance to temozolomide.Citation20 Another mechanism of resistance to alkylating agents is the BER pathway that can repair N7-methylguanine and N3-methyladenine DNA adducts. Cells that are defective in MMR are generally resistant to temozolomide.Citation23
Antimetabolites
Antimetabolites are widely used for the treatment of many types of cancer. Antimetabolites have molecular structures similar to the substrates of enzymes that are involved in DNA and RNA synthesis. Inhibition of DNA or RNA synthesis ultimately destroys the structure and function of DNA or RNA and leads to tumor cell death. Antimetabolites such as 5-fluorouracil, cytarabine, methotrexate, hydroxyurea, and gemcitabine are generally analogs of the natural building blocks of DNA.Citation25,Citation26 For example, gemcitabine is a deoxycytidine analog and is widely used in the treatment of solid tumors.Citation27–Citation29 However, tumor resistance of gemcitabine often seriously limits its effect.Citation30 These drugs may interact with DNA in two ways: by acting as structural analogs of the precursors and intermediates for the synthetic pathway, and therefore interfering with the synthesis of purines and pyrimidines, or by acting as false bases in the assembly of the DNA double helix during replication and transcription.
Antimetabolites can be divided into pyrimidine analogs, purine analogs, and folic acid analogs. Research on chemoresistance to nucleoside analogs such as pyrimidine analogs and purine analogs shows that deficiency of nucleoside transporters or nucleoside kinases such as deoxycytidine kinase (dCK), increased activity of ribonucleotide reductase (RR) or cytidine deaminase (CDA), and expression of 5′-nucleotidases are related to decrease in the cytotoxicity of nucleoside analogs.Citation31–Citation33 In addition, folic acid analog resistance may result from decreased cellular influx or increased efflux of the analogs, impaired polyglutamation, increased expression and various alterations in target enzymes, and intracellular accumulation of tetrahydrofolate cofactors.Citation34,Citation35
Natural products
Natural products, molecules discovered and isolated from living organisms and possessing biological or pharmacological activity, are commonly utilized for cancer chemotherapy.Citation36,Citation37 In addition, natural products can also be synthesized, with chemical property equivalent to their natural counterparts. Many anticancer drugs such as paclitaxel, vinblastine, etoposide, and hydroxycamptothecine are all natural products. Anticancer antibiotics, produced by microorganisms, are also valuable natural products.Citation10,Citation38 These drugs tend to be cell-cycle non-specific and therefore are used in the treatment of slow-growing tumors that have a low growth fraction, including daunorubicin, doxorubicin (DOX), epirubicin, idarubicin, valrubicin, mitoxantrone, bleomycin, and mitomycin c.Citation10
Taxanes are important natural product antitumor drugs. Paclitaxel and docetaxel interfere with spindle microtubules, causing cell apoptosis. Paclitaxel enters cells and binds to β-tubulin on the inner surface of microtubules.Citation39 Paclitaxel resistance is mainly associated with the following factors: multidrug resistance caused by the overexpression of P-glycoprotein (P-gp), tubulin mutations or alterations in microtubule stability, and reduced function of significant apoptotic proteins, such as Bcl-2 and p53.Citation39–Citation41
DOX is a widely used chemotherapeutic agent. The most common mechanism of resistance to DOX is the overexpression of ATP-binding cassette (ABC) transporters such as P-gp.Citation42 In addition, alterations of the drug target, topoisomerase, and modulation of programed cell death pathways are also important contributors to DOX resistance.Citation43,Citation44
Hormones and hormone antagonists
Tumors sometimes arise from hormone-sensitive cells. Tumor grows vigorously in the presence of hormones, and even depending on these hormones. Anticancer hormone therapy exploits these features to limit the availability of the hormones to cells in different ways.Citation10 Drugs in this category include selective estrogen receptor modulators (SERMS), progestins (megestrol acetate), luteinizing hormone-releasing hormone agonists, and androgenic agonists.
Glucocorticoids (GCs) such as dexamethasone (DEX) are a class of steroid hormones frequently used as a supportive care co-medication to suppress the side effects of other chemotherapeutic agents.Citation45,Citation46 Hormones are carried into cells, where they interact with hormone receptors, regulating transcription and protein synthesis of target genes in tumor cells. Therefore, interference of hormone–hormone receptor interaction can lead to cancer cell death.Citation10 Main mechanisms of GC resistance include ligand-induced downregulation of the receptors, the dominant-negative inhibition by the β-isoform of the receptors and repression by the transcription factor NF-κB.Citation47
Tamoxifen, a SERMS, is widely used to treat estrogen receptor (ER)-positive breast tumors. However, tamoxifen therapy often fails due to de novo and acquired tamoxifen resistance.Citation48 Tamoxifen resistance is associated with altered ER expression, especially on the plasma membrane, or altered expression of microRNAs and signaling pathways that regulate epithelial–mesenchymal transition in the tumor microenvironment.Citation48,Citation49
Miscellaneous agents
This group of agents includes several cancer chemotherapeutic agents that are difficult to categorize, mainly platinum analogs and enzymes. Platinum analogs are widely used in human neoplasia therapy, alone or in combination with other agents.Citation50 Platinum adducts induce distortion of DNA double helix and cellular DNA damage. Cisplatin, carboplatin, and oxaliplatin are the main platinum analogs used for chemotherapy.Citation19 The mechanisms of cellular resistance to platinum analogs can be classified in two groups: those that limit the formation of cytotoxic platinum-DNA adducts and those that prevent cell death occurring after platinum-DNA adduct formation.Citation51,Citation52
Asparaginase, an enzyme, is an important chemotherapeutic agent for the management of acute leukemia and other blood-related cancers.Citation53 The mechanisms of asparaginase resistance include increased asparagine synthetase activity, genomic modulations and alterations, epigenetic changes, and so on.Citation54,Citation55 Other miscellaneous drugs include hydroxyurea, procarbazine, and dacarbazine.Citation10
Nanomedicine in overcoming cancer cell chemoresistance
There is evidence that nanomedicines can help overcome cancer cell resistance to all the classes of chemotherapeutic agents mentioned above. Examples of using nanomedicines to overcome tumor cell resistance to representative drugs in different classes of cancer chemotherapeutic agents are showed in .
Table 1 Examples of using nanomedicines to overcome tumor cell resistance to representative drugs in different classes of cancer chemotherapeutics
Alkylating agents
Alkylating agents are a major class of cancer chemotherapeutic drugs. Clinical chemoresistance is a common complication in alkylating agent treatment of malignant tumors.Citation56,Citation57 Studies have shown that nanomedicines can overcome tumor chemoresistance to alkylating agents.Citation58–Citation60 For example, temozolomide, a commonly used alkylating agent, is considered the gold standard for the treatment of glioblastoma.Citation61,Citation62 However, growing resistance to temozolomide remains a major clinical challenge. The DNA repair enzyme MGMT plays a critical role in primary resistance to alkylating agents such as tomozolomide.Citation20 In addition, overexpression of epidermal growth factor receptor (EGFR) and Galectin-1 by tumor cells also significantly contributes to temozolomide resistance.Citation63,Citation64 Messaoudi et al developed chitosan-grafted lipid nanocapsules to deliver both anti-EGFR and anti-Galectin-1 siRNA to tumor cells, which represents a promising strategy to overcome temozolomide resistance.Citation60,Citation65,Citation66 Patil et al synthesized multifunctional temozolomide nanoconjugates (6.5–14.8 nm) using poly(β-l-malic acid), which contained trileucine (LLL) and antibody to transferrin receptor.Citation58 It was found that temozolomide-resistant cells were sensitive to the temozolomide nanoconjugates,Citation58 clearly demonstrating the feasibility of overcoming tumor cell resistance to the alkylating agent temozolomide by formulating it into nanomedicine.
Antimetabolites
Antimetabolites are widely used cancer chemotherapeutic agents, mainly including purine analogs, pyrimidine analogs, and antifolate agents. There is evidence that chemoresistance to nucleoside analogs such as gemcitabine, cytarabine, and fluorouracil can be overcome by using nanomedicines.Citation67–Citation70 The application of nanomedicine in overcoming gemcitabine resistance will be discussed in detail later.
Methotrexate, an antifolate agent, is indicated for the treatment of rheumatic disorders and malignant tumors. However, cancer cell resistance to methotrexate limits its applications. Johar-Ahar et al conjugated methotrexate to quantum dots (QDs) and showed that methotrexate–QDs were significantly more cytotoxic than free methotrexate in methotrexate-resistant KB cells (ie, IC50 values, 12.0 vs 105.0 µg/mL).Citation71
Natural products
Nanomedicines have shown promise in combating chemoresistance to natural products such as paclitaxel, docetaxel, and vincristine.Citation72–Citation75 For example, Yuan et al reported that paclitaxel-incorporated poly(d,l-lactic-co-glycolic) acid (PLGA)-Tween 80 nanoparticles can reverse multidrug resistance to paclitaxel.Citation72 Tang et al showed that docetaxel loaded in PLGA-D-α-tocopheryl polyethylene glycol (PEG) 1000 succinate/Poloxamer 235 nanoparticles was significantly more cytotoxic to docetaxel-resistant human breast adenocarcinoma MCF-7/TXT cells than Taxotere®, a commercial docetaxel solution, in culture and in a mouse model.Citation74
DOX, an anthracycline antibiotic, is widely used in solid tumor therapy. However, tumor cell resistance to DOX reduces its therapeutic efficacy.Citation76 Many studies exploited the feasibility of using nanomedicines to reverse DOX resistance.Citation76–Citation81 For example, Wang et al showed that DOX encapsulated in mesoporous silica nanoparticles (MSNPs) can overcome MCF-7/MDR1 cell resistance to DOX.Citation79 Unsoy et al synthesized chitosan-coated magnetic nanoparticles for targeted delivery of DOX. DOX-loaded nanoparticles were efficiently taken up by DOX-resistant MCF-7 breast cancer cells (MCF-7/1 µM, MCF-7/S) and were more cytotoxic than free DOX in DOX-resistant MCF-7 cells.Citation81 Yu et al also designed DOX nanoparticles that exhibited higher cytotoxic than free DOX in DOX-resistant MCF-7/ADR cells (ie, 54.4% viability vs 66.8% for free DOX).Citation80
Hormones and hormone antagonists
In this class of chemotherapeutic agents, many researchers have reported the use of nanomedicines to overcome tamoxifen chemoresistance. Aromatase inhibitors are used to treat hormone receptor-positive, locally advanced, or metastatic breast cancer. Letrozole is a potent non-steroidal aromatase inhibitor that is indicated to treat hormone-responsive breast cancer after surgery, but some patients develop resistance to letrozole during treatment.Citation82,Citation83 Nair et al developed hyaluronic acid-bound letrozole nanoparticles (HA-Letr-NPs) and showed that the HA-Letr-NPs can restore the sensitivity of tumors to letrozole in the LTLT-Ca letrozole-resistant breast tumor model.Citation84 Cho et al developed tamoxifen-incorporated manganese superoxide dismutase (MnSOD) siRNA nanoparticles that have an siRNA/poly(amidoamine) dendriplex core and an acid-sensitive polyketal shell and showed that the tamoxifen resistance of breast cancer cells was reversed when the antagonistic MnSOD activity was silenced by the MnSOD siRNA nanoparticles both in vitro and in vivo.Citation85
Miscellaneous agents
Tumor resistance to platinum analogs is very common, and the clinical efficacy of platinum analogs is limited by intrinsic and acquired resistance.Citation86 There is an increasing interest in developing new platinum anticancer agents, but new platinum agents have been very slow to enter clinics. There is evidence that nanomedicine may offer an effective alternative to overcome the resistance. For example, Zhou et al designed canthaplatin and PP2A inhibitor (LB) encapsulated PEG-b-PLGA micelles (ie, polymeric micelles) and showed that the micelles can overcome tumor resistance to cisplatin.Citation87 In addition, micelles prepared with PCL-b-PABPA-b-POEGMEA (ie, polycaprolactone, PCL; 3-((tert-butoxycarbonyl)amino) propyl acrylate, ABPA; oligo(ethylene glycol) methyl ether acrylate, OEGMEA) and incorporated with curcumin and platinum were shown to be able to overcome tumor cell resistance to platinum.Citation88
Limitations in using nanomedicine to overcome cancer cell chemoresistance
It is exciting that formulating cancer chemotherapeutic agents into nanomedicines can help overcome cancer cell chemoresistance. However, it is worth noting that to successfully overcome cancer chemoresistance, having nanomedicine formulations that can kill resistant cancer cells is often not sufficient. In vivo, the reticuloendothelial system (RES) and other physiological barriers can significantly impede the efficient delivery of nanomedicines to tumor cells, or even tumor tissues.Citation89,Citation90 Opsonization of nanomedicines by nonspecific adsorption of plasma proteins, such as opsonins, facilitates their phagocytosis and clearance from the circulation by RES.Citation90,Citation91 Many approaches have been utilized to limit the clearance of nanomedicines, either by delaying of RES clearance or by altering the surface properties of nanomedicines.Citation92,Citation93 The physiological barriers are another obstacle that needs to be overcome for nanomedicines to effectively overcome cancer chemoresistance in vivo.Citation89 The accessibility of nanomedicines to solid tumors is determined by various mechanisms, such as the efficiency of the blood and lymphatic networks in tumor tissues, the permeability of the vascular barriers in tumors, and the constitution of the tumor stroma.Citation89,Citation94 Several strategies have been explored to enhance the delivery of nanomedicines into tumor tissues such as normalizing the tumor vasculature or reducing tumor desmoplasia.Citation89
Nucleoside analogs and gemcitabine
Nucleoside analogs are structurally similar antimetabolites that have a broad range of actions and are clinically effective in both solid tumors and hematological malignancies.Citation95 These agents may interact with DNA or RNA by inhibiting the ability of cancer cells to synthesize precursors of nucleic acids required to ensure sustained growth or by directly interfering with DNA or RNA synthesis. Nucleoside analogs have a generalized structure consisting of a purine or pyrimidine base linked to a deoxyribose sugar. Examples of purine nucleosides and related inhibitors include cladribine, fludarabine, and clofarabine; and examples of pyrimidine nucleoside analogs include the deoxycytidine analogs gemcitabine, fluorouracil, cytarabine, capecitabine.Citation10
Gemcitabine (2′,2′-difluorodeoxycytidine, dFdC) is a deoxycytidine analog with antitumor activity against a wide variety of solid tumors such as pancreatic, non-small-cell lung cancer (NSCLC), breast cancer, and ovarian cancer, alone or in combination with other chemotherapeutic agents.Citation27–Citation29,Citation96 Moreover, gemcitabine is indicated in several hematological disorders such as acute leukemia.Citation97 Furthermore, gemcitabine enhances the cytotoxicity of cisplatin by increasing the formation of cytotoxic platinum-DNA adducts and is also a potent radiosensitizer used in radiation therapy.Citation98 However, drug resistance to gemcitabine often limits its efficacy in clinics,Citation30 and overcoming gemcitabine chemoresistance remains a challenge.Citation99
Gemcitabine and its mechanisms of action
As a hydrophilic nucleoside analog, cellular uptake of gemcitabine is mediated by nucleoside transporters such as the human equilibrative nucleoside transporter 1 (hENT1).Citation100 Once taken up into cells, gemcitabine is phosphorylated by dCK to gemcitabine monophosphate (dFdCMP), gemcitabine diphosphate (dFdCDP), and gemcitabine triphosphate (dFdCTP).Citation101 The active metabolite, dFdCTP, can terminate DNA elongation by incorporating into DNA, finally leading to cell death.Citation99,Citation102,Citation103 In addition, dFdCDP can inhibit RR by binding to the large subunit (RRM1).Citation104,Citation105 RRs catalyze the conversion of nucleoside 5′-diphosphates (ie, NDPs) to their corresponding deoxynucleotides (ie, dNDPs), which are phosphorylated to dNTPs for DNA synthesis.Citation105,Citation106 Inhibition of RRs will reduce the dNTP pool, allowing dFdCTP to more effectively compete with dNTPs and inhibit DNA replication and repair.Citation104–Citation107
Mechanisms of gemcitabine resistance
The mechanisms of resistance to gemcitabine are in many aspects different from those of the other classes of cancer chemotherapeutic agents. Multiple factors, including decreased expression of nucleoside transporters,Citation108,Citation109 changes in the expression of gemcitabine-activating or degradation enzymes and target molecules,Citation33,Citation110–Citation112 some signaling molecules (eg, NF-κB, P53) affecting cells that are resistant to apoptosis,Citation113,Citation114 and the expression of efflux transporters commonly resulted in MDR,Citation115,Citation116 have been reported to cause gemcitabine resistance.
Nucleoside transporters
Gemcitabine is a hydrophilic compound and cannot readily diffuse across cell membrane. Therefore, it requires nucleoside transporters to enter cells.Citation117,Citation118 The concentrative nucleoside transporters (hCNTs) and hENTs are implicated in tumor cell uptake of gemcitabine.Citation118,Citation119 Among these transporters, hENT1 is a major transporter involved in gemcitabine cellular uptake.Citation120 In fact, hENT1 has been reported as a vital predictive marker of tumor response to gemcitabine-based therapy.Citation32,Citation121,Citation122 Clinical data showed that cancer patients with a decreased tumor expression of hENT1 have a significantly lower survival rate after gemcitabine treatment than those with tumors that express a higher level of hENT1.Citation119,Citation121–Citation124 In culture, tumor cells lacking hENT1 expression become resistant to gemcitabine-mediated cytotoxicity.Citation108 For example, in the hENT1-deficient CCRF CEM-AraC-8C cells, the IC50 value of gemcitabine was reported to be 471-fold greater than that in the parent CCRF-CEM cells.Citation68
dCK
Once gemcitabine enters cells, dCK is the rate-limiting enzyme responsible for the conversion of gemcitabine to its active metabolites.Citation125,Citation126 Studies have indicated that in vitro and in vivo, dCK deficiency is related to gemcitabine resistance to pancreatic cancer, sarcoma, lymphoma, and leukemia.Citation127–Citation131 For example, Ohmine et al showed that the attenuation of gemcitabine phosphorylation is likely a key process for the acquisition of resistance by the RPK9 human pancreatic adenocarcinoma (PDAC) cells.Citation101 Lower expression of dCK was shown to be associated with shorter overall survival in pancreatic cancer patients who received gemcitabine as an adjuvant therapy.Citation132 It is suggested that dCK expression in both protein and mRNA levels may serve as a biomarker to predict tumor cell sensitivity to nucleoside analogs such as gemcitabine.Citation133
CDA
CDA, a key enzyme involved in gemcitabine metabolism, was identified in the early 1990s.Citation134 Deamination is the main mechanism by which gemcitabine is inactivated, and it is estimated that 90% of gemcitabine is inactivated to difluorodeoxyuridine by CDA intracellularly and extracellularly.Citation96 In vitro, macrophage-induced CDA upregulation in human Panc-1 pancreatic tumor cell line has been shown to confer gemcitabine resistance.Citation111 Data from ample studies have indicated that CDA polymorphisms alter CDA enzyme activity and the pharmacokinetics of gemcitabine,Citation135–Citation138 and three functional polymorphisms of CDA (rs2072671, CDA 79A > C; rs60369023, CDA 208G > A; and rs1048977, CDA 435C > T) could predict the clinical outcomes of gemcitabine-based tumor chemotherapy.Citation33,Citation135,Citation136
RR or RNR
As mentioned above, RRs catalyze the conversion of NDPs to their corresponding dNDPs for DNA synthesis.Citation139,Citation140 In mammals, RR is a heterodimeric tetramer consisting of two large subunits (RRM1) and two small subunits (RRM2 and RRM2B).Citation141,Citation142 RRM1 catalyzes the rate-limiting step in the production of dNTPs and is an essential enzyme for DNA replication and repair.Citation139,Citation143 The catalysis activity of RRs requires the binuclear iron center and a tyrosyl-free radical located in RRM2.Citation142 RRM2B was identified as a critical p53-inducible RR subunit that can be regulated by p53 and p73 genes/proteins.Citation144,Citation145
Gemcitabine self-potentiates its own effect by directly inhibiting RRM1. Therefore, upregulation of RRM1 can lead to gemcitabine resistance.Citation110,Citation146 Conversely, RRM1 knockdown in the resistant MIA PaCa-2 pancreatic cancer cell line completely restored gemcitabine sensitivity.Citation147 The relationship between RRM2 mRNA expression and response to gemcitabine in clinical setting has been investigated in various cancers. For example, the response rate to gemcitabine is significantly higher in pancreatic cancer patients with low RRM2 mRNA expression in biopsy specimens.Citation148 Similarly, high RRM2 expression was found to be correlated with poor clinical outcome in patients with lower-risk prostate cancer.Citation112
Other mechanisms of resistance to gemcitabine
The aforementioned are the main mechanisms of gemcitabine resistance. There are other factors associated with gemcitabine resistance as well. For example, excision repair cross-complementing protein 1 can repair gemcitabine-induced strand breaks, and its overexpression is well documented in poor gemcitabine responders.Citation149,Citation150 Kozinn et al reported that microRNAs 1290, 138, let-7i, and let-7b are involved in gemcitabine resistance in bladder carcinoma cell lines.Citation151 In addition, a tumor microenvironment that favors cancer progression and metastasis can elicit drug resistance.Citation152 For example, Xu et al showed that sonic hedgehog (SHH) signaling in tumor microenvironment protects PDAC cells against gemcitabine-induced apoptosis and that overexpression of SHH in PDAC cells enhances drug resistance.Citation153 Other factors that contribute to gemcitabine resistance include NF-κB,Citation114 heat shock proteins, and the presence of highly resistant tumor stem cells.Citation154,Citation155
Overcoming gemcitabine resistance using nanomedicines
Nanomedicines have unique advantages in overcoming tumor cell resistance to gemcitabine, and various types of gemcitabine nanomedicine formulations, such as micelles,Citation156 liposomes,Citation157 supramolecular vesicular aggregates, and nanovesicles,Citation158–Citation160 have been shown to circumvent gemcitabine resistance. The general mechanisms by which gemcitabine nanomedicines overcome gemcitabine chemoresistance are discussed below.
Reducing RR expression
Accumulating evidence indicates that increased expression of RRM1 is associated with a poor response of cancer patients to gemcitabine.Citation161,Citation162 Higher levels of RRM1 were detected in tumors of various patients who respond poorly to gemcitabine.Citation139,Citation143,Citation163–Citation165 For example, in gemcitabine-treated advanced NSCLC patients, those with RRM1-positive tumors were shown to have worse overall survival and disease control than those with RRM1-negative tumors.Citation163 Similarly, in patients with advanced nasopharyngeal carcinoma (NPC) and treated with gemcitabine-based regimens, high RRM1 expression is correlated with shorter progression-free survival, compared to patients with RRM1-negative expression.Citation161 In a recent study, RRM1 siRNA was used to downregulate RRM1 expression in tumor cells, and it was shown that pre-exposure of A549 lung cancer cells to RRM1 siRNA nanoconstructs significantly decreased the IC50 value of gemcitabine in the tumor cells compared to gemcitabine alone.Citation166 Previously, we have also shown in a mouse model with TC-1 mouse lung cancer cells that overexpress RRM1 (ie, TC-1-GR cells) that treatment with RRM1 siRNA-polyethylenimine (PEI) nanocomplexes (122±5 nm) significantly increased the effect of gemcitabine against the tumors, compared to treatment with control siRNA-PEI nanocomplexes.Citation167
Increasing cellular uptake of gemcitabine
As mentioned above, gemcitabine depends on nucleoside transporters to enter cells.Citation117,Citation168 Therefore, reduced expression of nucleoside transporters on tumor cells causes tumor cell resistance to gemcitabine.Citation123,Citation169 For example, it was reported that high hENT1 expression in resected specimen of patients with PDAC who received postoperative gemcitabine therapy is correlated with increased overall survival,Citation170–Citation173 whereas low hENT1 expression was linked to gemcitabine resistance and shorter overall survival.Citation109,Citation123,Citation169 In addition, low levels of hENT1 expression were also detected in tumors in gallbladder adenocarcinoma patients who respond poorly to gemcitabine.Citation122 Nanomedicine formulations of gemcitabine can overcome gemcitabine resistance caused by reduced expression of nucleoside transporters by delivering gemcitabine into tumor cells independent of the transporters. For example, we have data showing that our previously developed stearoyl gemcitabine solid lipid nanoparticles (ie, 4-(N)-GemC18-SLNs) enter tumor cells by clathrin-mediated endocytosis.Citation174 Hung et al showed that a nanoparticle formulation of gemcitabine has significantly smaller IC50 values, compared to free gemcitabine, in ovarian cancer cells that express low levels of hCNT1,Citation175 indicating that the nanoparticle formulation can bypass nucleoside transporter defects.
Reducing deamination of gemcitabine
Stromal and cellular CDAs convert gemcitabine to an inactive metabolite.Citation96,Citation176 Preclinical and clinical studies have suggested that upregulation of CDAs increases gemcitabine resistance, while CDA deficient is associated with increased gemcitabine activity.Citation33,Citation96 To protect gemcitabine from rapid deamination, many attempts have been made by chemically modifying gemcitabine.Citation177–Citation180 For example, it was shown that conjugation of a fatty acid, such as stearic acid, to gemcitabine at the 4-N position decreases the sensitivity of the later to deaminase.Citation181,Citation182 In addition, gemcitabine–fatty acid conjugates formulated into nanoparticles also become less sensitive to deamination, as they are no longer good substrates of CDAs.Citation182 Meng et al developed a MSNP for co-delivery of gemcitabine and paclitaxel to take advantage of paclitaxel’s ability to inhibit CDA expression to increase tumor response to gemcitabine.Citation183
Enhancing distribution and/or accumulation of gemcitabine in tumor tissues
The enhanced permeability and retention (EPR) effect discovered by Matsumura and Maeda has been exploited for passive targeting of anticancer drugs into tumors.Citation184,Citation185 The discovery of EPR effect is of great significance to the design of antitumor nanomedicines.Citation186–Citation188 Nanomedicine formulations of gemcitabine can take advantage of the EPR effect to increase gemcitabine content within tumor tissues upon intravenous injection.Citation189–Citation192 Having more gemcitabine distributed into tumor tissues will provide them the chance to kill tumor cells or inhibit tumor cell growth.
Examples of using nanomedicine formulations of gemcitabine to overcome tumor cell resistance to gemcitabine
In their effort in finding a solution to overcome gemcitabine resistance, scientists showed that nanomedicine formulations of gemcitabine have promising potentials.Citation68,Citation174,Citation180,Citation193 Examples of overcoming tumor cell resistance to gemcitabine using nanomedicines are shown in .
Table 2 Examples of overcoming tumor cell resistance to gemcitabine using nanomedicines
Polymeric nanoparticles
Recognizing that defective hCNT1 contributes to gemcitabine chemoresistance in ovarian cancer, Hung et al created PLGA-b-PEG-OH nanoparticles incorporated with gemcitabine. The gemcitabine–PLGA-b-PEG-OH nanoparticles effectively delivered gemcitabine into hCNT1-decreased ES-2 and TOV-21G tumor cells and were significantly more cytotoxic to those cells than free gemcitabine.Citation175 Papa et al reported gemcitabine-loaded PLGA nanoparticles (PLGem) and evaluated their cytotoxicity to aggressive human Panc-1 cells, which are well-known to exhibit gemcitabine resistance.Citation194 The PLGem was significantly more cytotoxic than free gemcitabine to Panc-1 cells.Citation194
In another approach, gemcitabine nanoparticles were prepared by loading GemC18, a stearic acid amide derivative of gemcitabine, in PEG–poly(d,l-lactic) acid (PEG–PLA) polymeric micelles or by GemC18 self-assembling.Citation195 Both of the nanomedicines effectively reduced the viability of gemcitabine-resistant AsPC-1 cells in culture (IC50 values, 58.88 and 46.34 µM, respectively), whereas the molar equivalent free gemcitabine did not show any significant cytotoxicity to AsPC-1 cells. The GemC18 self-assembled nanoparticles showed greater in vitro cellular uptake and cytotoxicity than the GemC18-PEG-PLA polymeric micellar nanoparticles (ie, drug uptake in Panc-1 cells, 37.55%±2.21% for GemC18 self-assembled nanoparticles, 28.60%±1.85% for GemC18-PEG-PLA polymeric micelles, and 30.11%±1.98% for GemC18 in solution).Citation195
Gemcitabine nanoparticles were also prepared based on a gemcitabine–squalene conjugate (SQ-dFdC or SQ-Gem), which displayed a stronger antiproliferative and cytotoxic activity than gemcitabine. After orthotopic Panc-1 tumor-bearing mice were treated two times at a 4-day interval with either gemcitabine (20 mg/kg) or SQ-Gem (20 mg/kg), SQ-Gem was more effective than gemcitabine in inhibiting the tumor growth.Citation178 The SQ-dFdC nanoparticles were also shown to overcome gemcitabine resistance in murine and human leukemia cells (ie, L1210-10K and CEM/AraC-8C, respectively).Citation180
Liposomes
Xu et al developed pH-sensitive liposomes (PSLs) with a high content of gemcitabine. The cytotoxicity values of the various gemcitabine-PSLs developed were evaluated in the gemcitabine-resistant MIA PaCa-2 cell line.Citation67 Gemcitabine-PSLs with drug loading of 0.5% and 4.5% had similar IC50 values (ie, 1.1±0.1 versus 0.7±0.1 µM), but both were significantly smaller than that of free gemcitabine in solution and gemcitabine in non-PSLs. In an animal model, the gemcitabine-PSLs were significantly more effective than free gemcitabine in controlling the growth of gemcitabine-resistant pancreatic cancer cells.Citation67 Papa et al also reported gemcitabine-encapsulated nanoliposomes (GemPo), which were shown to be more cytotoxic than free gemcitabine in gemcitabine-resistant MDA-MB-231 breast cancer cells and sensitive 4T1 cells.Citation196
Overcoming tumor cell resistance to gemcitabine using stearoyl gemcitabine-incorporated solid lipid nanoparticles
In our effort to improve the antitumor activity of gemcitabine, we previously developed a stearoyl gemcitabine nanoparticle formulation by incorporating 4-(N)-stearoyl gemcitabine (ie, 4-(N)-GemC18) into solid lipid nanoparticles engineered from lecithin/glycerol monostearate-in-water emulsions.Citation181 In animal models (ie, C57BL/6 mice with TC-1 mouse lung cancer cells, nude mice with BxPC-3 human pancreatic cancer cells), the 4-(N)-GemC18-SLNs were significantly more effective than the molar equivalent of free gemcitabine or 4-(N)-GemC18 in a Tween 20 solution in inhibiting tumor growth.Citation181 More importantly, we discovered that the 4-(N)-GemC18-SLNs can overcome tumor cell resistance to gemcitabine.Citation68 For example, 4-(N)-GemC18-SLNs were 15-fold more cytotoxic than gemcitabine HCl in the hENT1-deficient CCRF CEM-AraC-8C cells, and ~8-fold more cytotoxic in the dCK−/− CCRF CEM-AraC-8D cells.Citation68 In the gemcitabine resistant human Panc-1 tumor cells that overexpress RRM2, 4-(N)-GemC18-SLNs were >17-fold more cytotoxic than gemcitabine HCl.Citation68 In the RRM1-overexpresssing, gemcitabine-resistant TC-1-GR cells, the IC50 value of 4-(N)-GemC18-SLNs was only about 5% of that of gemcitabine HCl.Citation68 Importantly, although both 4-(N)-GemC18-SLNs and free gemcitabine HCl can significantly inhibit the growth of the highly gemcitabine-sensitive TC-1 tumor cells in a mouse model, only 4-(N)-GemC18-SLNs, but not free gemcitbaine HCl, can significantly inhibit the growth of the gemcitabine-resistant TC-1-GR tumors.Citation68 When elucidating the mechanisms underlying the 4-(N)-GemC18-SLNs’ ability to overcome gemcitabine resistance, we discovered that the unique composition of the 4-(N)-GemC18-SLNs and the way by which the gemcitabine in the 4-(N)-GemC18-SLNs enters tumor cells are likely responsibe for their ability to more effectively kill tumor cells, especially tumor cells that are otherwise resistant to gemcitabine.Citation174 We concluded that for gemcitabine to effectively kill tumor cells, especially those resistant to gemcitaine, entering tumor cells is important, but not enough.Citation174
The unique composition of the 4-(N)-GemC18-SLNs is critical for their ability to kill tumor cells resistant to gemcitabine
The conclusion that the unique composition of the 4-(N)-GemC18-SLNs is critical for their ability to overcome gemcitabine resistance is supported by the following findings: 1) a 3′-(O)-GemC18 ester synthesized by conjugating gemcitabine in the 3′-O position with stearic acid, when incorporated into the same solid lipid nanoparticles engineered from lecithin/glyceroyl monostearate-in-water emulsions, was not significantly more effective than free gemcitabine in controlling the growth of the gemcitabine-resistant TC-1-GR tumor cells in culture and in a mouse model; 2) the same 4-(N)-GemC18 amide when incorporated into polymeric PLGA nanoparticles was not more effective than gemcitabine in inhibiting the growth of the gemcitabine-resistant TC-1-GR cells; and 3) 4-(N)-GemC8, another amide gemcitabine derivative synthesized by conjugating gemcitaine in its 4-N position with a medium chain fatty acid, caprylic acid (C8), incorporated into the same solid lipid nanoparticles engineered from lecithin/glyceroyl monostearate-in-water emulsions was not more cytotoxic than free gemcitabine against the gemcitabine-resistant TC-1-GR cells.Citation174 Therefore, it seems that the amide nature of the 4-(N)-GemC18, the long chain fatty acid (ie, stearic acid) derivative nature of the 4-(N)-GemC18, and the solid lipid nanoparticles in which the 4-(N)-GemC18 is incorporated in are all critical for the resultant 4-(N)-GemC18-SLNs to overcome tumor cell resistance to gemcitabine.
It is noted that the 4-(N)-GemC18 needs to be incorporated into the solid lipid nanoparticles, as our results showed that 4-(N)-GemC18 alone and the physical mixture of it and blank solid lipid nanoparticles were not as cytotoxic as 4-(N)-GemC18-SLNs against the gemcitabine-resistant TC-1-GR cells.Citation174 Furthermore, we showed that our 4-(N)-GemC18-SLNs, but not free gemcitabine, nor 4-(N)-GemC18, significantly downregulate RRM1 expression in the gemcitabine-resistant TC-1-GR cells in culture and in a mouse model and increased the level of dFdCTP in TC-1-GR cells in culture.
The pathway by which the 4-(N)-GemC18 in the 4-(N)-GemC18-SLNs enters tumor cells is critical for its ability to kill tumor cells resistant to gemcitabine
The exact mechanisms why our 4-(N)-GemC18-SLNs can more effectively kill the TC-1-GR tumors that overexpress RRM1 than other gemcitabine formulations, including free gemcitabine, free 4-(N)-GemC18, 3′-(O)-GemC18-SLNs, 4-(N)-GemC8-SLNs, and 4-(N)-GemC18-PLGA-nanoparticles remains unknown, but we hypothesize that it is very likely related to the pathway by which 4-(N)-GemC18-SLNs deliver the gemcitabine into tumor cells. Free gemcitabine depends on nucleoside transporters such as hENT1 to enter tumor cells, and free 4-(N)-GemC18 enters cells by passive diffusion due to its high lipophilicity. Our 4-(N)-GemC18-SLNs, however, enter cells by clathrin-mediated endocytosis.Citation174 Alkalization of lysosomes (ie, increasing pH) did not affect the uptake and intracellular degradation of 4-(N)-GemC18 when it was taken up as free 4-(N)-GemC18 in solution. However, when cells were incubated with the 4-(N)-GemC18-SLNs, alkalization of lysosomal pH significantly inhibited the intracellular degradation of 4-(N)-GemC18.Citation174 Lysosomal acidification is required for the activation of many enzymes in lysosomes, indicating that the acidic lysosomal environment and thus many pre-enzymes in lysosomes activated in acidic environment are important for the degradation of the solid lipid nanoparticles, the release of the 4-(N)-GemC18 from the nanoparticles, and the hydrolysis of 4-(N)-GemC18 to free gemcitabine, when 4-(N)-GemC18 was brought into cells by the endocytosis of the 4-(N)-GemC18-SLNs.Citation174 The 3′-(O)-GemC18-SLNs were not as effective as 4-(N)-GemC18-SLNs because the ester bond in the 3′-(O)-GemC18 was readily hydrolyzed even before the 3′-(O)-GemC18-SLNs were endocytized.Citation174 Similarly, the 4-(N)-GemC8-SLNs were not as effective as 4-(N)-GemC18-SLNs because the 4-(N)-GemC8 was readily released or leaked from the 4-(N)-GemC8-SLNs before the nanoparticles were endocytized.Citation174
Proposed mechanism by which 4-(N)-GemC18-SLNs overcome tumor cells resistant to gemcitabine
Based on the findings mentioned above, we hypothesized that the 4-(N)-GemC18-SLNs take advantage of the salvage nucleotide synthesis pathway and “channel” the 4-(N)-GemC18 into a “natural” pathway that has evolved for cells to efficiently reuse bases and nucleosides from within or outside cells. When cells take up the apoptotic bodies or foreign pathogens by endocytosis, the nucleic acids are enzymatically degraded into nucleosides and bases for reuse.Citation197 As shown in , it is likely that after our 4-(N)-GemC18-SLNs enter tumor cells by clathrin-mediated endocytosis, enzymes in lysosomes catalyze the degradation of the solid lipid nanoparticles, and the degradation facilitates the release of 4-(N)-GemC18 from the nanoparticles. Lysosomal enzymes such as cathepsin B catalyze the hydrolysis of 4-(N)-GemC18 to free gemcitabine, a nucleoside analog. Gemcitaine released into the lysosomes can then be exported out of the lysosomes to the cytoplasm by nucleoside transporters, such as the lysosome-specific hENT3,Citation198 into the proper intracellular compartment for efficient phosphorylation to its active metabolites, dFdCDP and dFdCTP. In contrast, when free 4-(N)-GemC18 diffuses into tumor cells by passive diffusion, it may be hydrolyzed to release gemcitabine intracellularly, but not in the proper intracellular compartment for efficient phosphorylation, due to its high lipophilicity. Exposing gemcitabine in the “wrong” compartment in cells will likely subject it to deamination by CDA, considering that nucleotides normally do not enter cells in the form of long chain fatty acid conjugates. Free gemcitabine enters tumor cells with the help of nucleoside transporters, but it is subjected to extensive deamination intracellularly and extracellularly before being phosphorylated ().Citation174 The small amount of dFdCTP generated may be sufficient to inhibit tumor cells that are sensitive to gemcitabine, but not against tumor cells that developed various resistant mechanisms (eg, overexpression of RRM1). Of course, more experiments will have to be carried out to generate data to fully support the hypothesized mechanism, but designing nanomedicine formulations of anticancer drugs that mimic or take advantage of a natural pathway, such as the nucleotide salvage pathway in the case of nucleoside analogs such as gemcitabine, likely represents a desirable strategy to improve the activity of the drugs and to overcome chemoresistance.
Figure 1 A schematic of the proposed mechanism by which 4-(N)-GemC18-SLNs overcome tumor cell resistance to gemcitabine.
Note: Reprinted from J Control Release. 169(1–2). Wonganan P, Lansakara PD, Zhu S, et al. Just getting into cells is not enough: mechanisms underlying 4-(N)-stearoyl gemcitabine solid lipid nanoparticle’s ability to overcome gemcitabine resistance caused by RRM1 overexpression.Citation17–Citation27. Copyright 2013, with permission from Elsevier.Citation174
Abbreviations: CDA, (deoxy)cytidine deaminase; dCK, deoxycytidine kinase; dFdC, gemcitabine; dFdCMP, gemcitabine monophosphate; dFdCDP, gemcitabine diphosphate; dFdCTP, gemcitabine triphosphate; dNDP, deoxyribonucleoside diphosphate; dNTP, deoxyribonucleoside triphosphate; hENT, human equilibrative nucleoside transporter; NDP, ribonucleoside diphosphates; RR, ribonucleotide reductase.
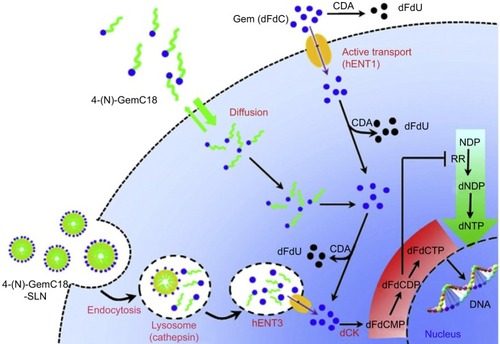
Finally, it is worth noting that the mechanism mentioned above was largely based on cell culture data.Citation174 In a mouse model with tumor cells that are resistant to gemcitabine due to the overexpression of RRM1, our 4-(N)-GemC18-SLNs significantly inhibited the tumor growth, although the molar equivalent dose of free gemcitabine did not show any significant antitumor effect.Citation68 Moreover, we have also engineered 3′-(O)-GemC18-SLNs by incorporating 3′-(O)-GemC18 into the same solid lipid nanoparticles; 3′-(O)-GemC18 was synthesized by conjugating stearic acid to gemcitabine in the 3′-O position to form an ester, which is more sensitive to hydrolysis than 4-(N)-GemC18. Therefore, 3′-(O)-GemC18-SLNs cannot as effectively as 4-(N)-GemC18-SLNs kill the RRM1-overexpressing tumor cells in culture and in vivo.Citation174 The data from our in vivo studies in a mouse model indicated that the mechanism we proposed above is also applicable in vivo. In other words, in the tumor-bearing mouse model we tested, some of our 4-(N)-GemC18-SLNs should have reached the tumor cells after intravenous injection and entered the endolysosomes of the tumor cells by endocytosis.
It is not easy for nanoparticles to evade uptake by the RES and overcome other physiological barriers to successfully reach tumor cells. For nanoparticles that reach tumor tissues intact, besides the aforementioned endocytosis by tumor cells, there are other potential mechanisms for the chemotherapeutic agents carried by the nanoparticles to enter tumor cells. One obvious mechanism is that the chemotherapeutic agents are released from the nanoparticles in the tumor tissues, diffuse to tumor cell surface, and then enter tumor cells by passive diffusion or transporter-mediated uptake. There are strategies to facilitate the release of chemotherapeutic agents from nanoparticles within tumor tissues. For example, secretory phospholipase A2 (sPLA2) are enzymes overexpressed in various tumors.Citation199–Citation201 Liposomes that are responsible for sPLA2 (SPRL) were engineered to facilitate liposomal degradation and drug release in tumor tissues.Citation202–Citation204 In a study comparing the uptakes and cytotoxicities of the SPRL encapsulated with DOX and sterically stabilized liposomes encapsulated with DOX, Moch et al suggested that the efficacy of the sPLA2 liposomes are mediated by cell-dependent mechanisms.Citation203 Recently, Hofmann et al provided evidence supporting the existence of drug delivery into cells without cellular uptake of the nanoparticles through a new “kiss-and-run” mechanism between (polymeric) nanoparticles and the cell membrane.Citation205
Conclusion
Despite recent advances in targeted therapies and immunotherapies, chemotherapy using cytotoxic agents remains an indispensable modality in cancer treatment. Formulating cancer chemotherapeutic agents into nanomedicines represents an attractive approach to modify their pharmacokinetics, efficacy, and toxicity profiles. Moreover, cancer cells often develop resistance to chemotherapeutic agents prior to or during treatment, and there is encouraging evidence that formulating cancer chemotherapeutic agents into nanomedicines may also represent a viable approach to overcome cancer cell chemoresistance. However, better nanomedicine formulations of chemotherapeutical agents are often the results of rational mechanism-based design, and occasionally by accident.
Acknowledgments
This work was supported in part by the National Natural Science Foundation of China (81660272, 81460454, 81460248, 81260457) (to YZ, ZC, and/or YS), the Inner Mongolia Natural Science Fund (2017ZD08, 2014ZD05, 2016MS0318, 2016MS0368, 2015MS0820, 2013MS1138, 2012MS1121, 2011MS1110) (to YS, ZC, and/or YZ), the National “Twelfth Five-Year” Plan for Science & Technology Support of China (2014BAI13B03) (to YS), the Inner Mongolia Science and Technology Plan (20120101, 20120402, 20110501) (to YS), and the US National Institutes of Health (CA135274, CA179362) (to ZC).
Disclosure
The authors report no conflicts of interest in this work.
References
- TorreLABrayFSiegelRLFerlayJLortet-TieulentJJemalAGlobal cancer statistics, 2012CA Cancer J Clin20156528710825651787
- SiegelRLMillerKDJemalACancer statistics, 2016CA Cancer J Clin201666173026742998
- JonckheereNSkrypekNVan SeuningenIMucins and tumor resistance to chemotherapeutic drugsBiochim Biophys Acta20141846114215124785432
- ZhaoJCancer stem cells and chemoresistance: the smartest survives the raidPharmacol Ther201616014515826899500
- DuBShimJSTargeting epithelial-mesenchymal transition (EMT) to overcome drug resistance in cancerMolecules2016217 pii:E965
- NaguibYWCuiZNanomedicine: the promise and challenges in cancer chemotherapyAdv Exp Med Biol201481120723324683034
- BoboDRobinsonKJIslamJThurechtKJCorrieSRNanoparticle-based medicines: a review of FDA-approved materials and clinical trials to datePharm Res201633102373238727299311
- WangAZGuFZhangLBiofunctionalized targeted nanoparticles for therapeutic applicationsExpert Opin Biol Ther2008881063107018613759
- ZhangLGuFXChanJMWangAZLangerRSFarokhzadOCNanoparticles in medicine: therapeutic applications and developmentsClin Pharmacol Ther200883576176917957183
- Matray-devotiJCancer DrugsNew YorkChelsea House Publications2006
- TeicherBACancer Therapeutics: Experimental and Clinical AgentsTotowa, NJHumana Press1997
- LivshitsZRaoRBSmithSWAn approach to chemotherapy-associated toxicityEmerg Med Clin North Am201432116720324275174
- KibriaGHatakeyamaHHarashimaHCancer multidrug resistance: mechanisms involved and strategies for circumvention using a drug delivery systemArch Pharm Res201437141524272889
- HolohanCVan SchaeybroeckSLongleyDBJohnstonPGCancer drug resistance: an evolving paradigmNat Rev Cancer2013131071472624060863
- DrinbergVBitcoverRRajchenbachWPeerDModulating cancer multidrug resistance by sertraline in combination with a nanomedicineCancer Lett2014354229029825173796
- GengMWangLChenXCaoRLiPThe association between chemosensitivity and Pgp, GST-pi and Topo II expression in gastric cancerDiagn Pathol2013819824326092
- TurriniEFerruzziLFimognariCNatural compounds to overcome cancer chemoresistance: toxicological and clinical issuesExpert Opin Drug Metab Toxicol201410121677169025339439
- ProvenzanoPPHingoraniSRHyaluronan, fluid pressure, and stromal resistance in pancreas cancerBr J Cancer201310811823299539
- PriestmanTCancer Chemotherapy in Clinical PracticeLondonSpringer2008
- SarkariaJNKitangeGJJamesCDMechanisms of chemoresistance to alkylating agents in malignant gliomaClin Cancer Res200814102900290818483356
- FuDCalvoJASamsonLDBalancing repair and tolerance of DNA damage caused by alkylating agentsNat Rev Cancer201212210412022237395
- BordinDLLimaMLenzGDNA alkylation damage and autophagy inductionMutat Res20137532919923872363
- SrinivasanAGoldBSmall-molecule inhibitors of DNA damage-repair pathways: an approach to overcome tumor resistance to alkylating anticancer drugsFuture Med Chem2012491093111122709253
- KainaBChristmannMNaumannSRoosWPMGMT: key node in the battle against genotoxicity, carcinogenicity and apoptosis induced by alkylating agentsDNA Repair (Amst)2007681079109917485253
- TiwariMAntimetabolites: established cancer therapyJ Cancer Res Ther20128451051923361267
- DeVitaVTJrChuEA history of cancer chemotherapyCancer Res200868218643865318974103
- TsaiSEvansDBTherapeutic advances in localized pancreatic cancerJAMA Surg2016151986286827276001
- NagourneyRAFlamMLinkJCarboplatin plus gemcitabine repeating doublet therapy in recurrent breast cancerClin Breast Cancer20088543243518952557
- PfistererJPlanteMVergoteIGemcitabine plus carboplatin compared with carboplatin in patients with platinum-sensitive recurrent ovarian cancer: an intergroup trial of the AGO-OVAR, the NCIC CTG, and the EORTC GCGJ Clin Oncol200624294699470716966687
- LongJZhangYYuXOvercoming drug resistance in pancreatic cancerExpert Opin Ther Targets201115781782821391891
- JordheimLPDumontetCReview of recent studies on resistance to cytotoxic deoxynucleoside analoguesBiochim Biophys Acta20071776213815917881132
- MarechalRBachetJBMackeyJRLevels of gemcitabine transport and metabolism proteins predict survival times of patients treated with gemcitabine for pancreatic adenocarcinomaGastroenterology20121433664674e1e622705007
- CiccoliniJDahanLAndreNCytidine deaminase residual activity in serum is a predictive marker of early severe toxicities in adults after gemcitabine-based chemotherapiesJ Clin Oncol201028116016519933910
- HagnerNJoergerMCancer chemotherapy: targeting folic acid synthesisCancer Manag Res2010229330121301589
- PurcellWTEttingerDSNovel antifolate drugsCurr Oncol Rep20035211412512583828
- SiddiquiIASannaVImpact of nanotechnology on the delivery of natural products for cancer prevention and therapyMol Nutr Food Res20166061330134126935239
- OrlikovaBLegrandNPanningJDicatoMDiederichMAnti-inflammatory and anticancer drugs from natureCancer Treat Res201415912314324114478
- AirleyRCancer Chemotherapy: Basic Science to the ClinicWest Sussex, UKWiley-Blackwell2009
- SmoterMBodnarLDuchnowskaRStecRGralaBSzczylikCThe role of Tau protein in resistance to paclitaxelCancer Chemother Pharmacol201168355355721713447
- VergaraDTinelliAIannoneAMaffiaMThe impact of proteomics in the understanding of the molecular basis of paclitaxel-resistance in ovarian tumorsCurr Cancer Drug Targets201212898799722515523
- BarbutiAMChenZSPaclitaxel through the ages of anticancer therapy: exploring its role in chemoresistance and radiation therapyCancers (Basel)2015742360237126633515
- ShengYYouYChenYDual-targeting hybrid peptide-conjugated doxorubicin for drug resistance reversal in breast cancerInt J Pharm2016512111327521706
- CoxJWeinmanSMechanisms of doxorubicin resistance in hepatocellular carcinomaHepat Oncol201631575926998221
- VatsyayanRChaudharyPLelsaniPCRole of RLIP76 in doxorubicin resistance in lung cancerInt J Oncol20093461505151119424567
- SimkoVTakacovaMDebreovaMDexamethasone downregulates expression of carbonic anhydrase IX via HIF-1alpha and NF-kappaB-dependent mechanismsInt J Oncol20164941277128827431580
- CookAMMcDonnellAMLakeRANowakAKDexamethasone co-medication in cancer patients undergoing chemotherapy causes substantial immunomodulatory effects with implications for chemo-immunotherapy strategiesOncoimmunology201653e106606227141331
- SchaafMJMCidlowskiJAMolecular mechanisms of glucocorticoid action and resistanceJ Steroid Biochem Mol Biol2002831–5374812650700
- Rondon-LagosMVillegasVERangelNSanchezMCZaphiropoulosPGTamoxifen resistance: emerging molecular targetsInt J Mol Sci2016178 pii:E1357
- Viedma-RodriguezRBaiza-GutmanLSalamanca-GomezFMechanisms associated with resistance to tamoxifen in estrogen receptor-positive breast cancer (review)Oncol Rep201432131524841429
- MacerelliMGanzinelliMGouedardCCan the response to a platinum-based therapy be predicted by the DNA repair status in non-small cell lung cancer?Cancer Treat Rev20164881927262017
- TapiaGDiaz-PadillaIMolecular mechanisms of platinum resistance in ovarian cancerDiaz-PadillaIOvarian Cancer – A Clinical and Translational UpdateInTechOpen2013205223
- RabikCADolanMEMolecular mechanisms of resistance and toxicity associated with platinating agentsCancer Treat Rev200733192317084534
- ShrivastavaAKhanAAKhurshidMKalamMAJainSKSinghalPKRecent developments in L-asparaginase discovery and its potential as anticancer agentCrit Rev Oncol Hematol201610011025630663
- AndradeAFBorgesKSSilveiraVSUpdate on the use of l-asparaginase in infants and adolescent patients with acute lymphoblastic leukemiaClin Med Insights Oncol201489510025210485
- ChenSHAsparaginase therapy in pediatric acute lymphoblastic leukemia: a focus on the mode of drug resistancePediatr Neonatol201556528729325603726
- KwaFACole-SinclairMKapuscinskiMChlorambucil-sensitive and -resistant lymphoid cells display different responses to the histone deacetylase inhibitor, sodium butyrateBiochem Biophys Res Commun20104033–428829221075074
- XiaoSYangZQiuXmiR-29c contribute to glioma cells temozolomide sensitivity by targeting O6-methylguanine-DNA methyltransferases indirectlyOncotarget2016731502295023827384876
- PatilRPortilla-AriasJDingHTemozolomide delivery to tumor cells by a multifunctional nano vehicle based on poly(beta-L-malic acid)Pharm Res201027112317232920387095
- OrzaASoritauOTomuleasaCReversing chemoresistance of malignant glioma stem cells using gold nanoparticlesInt J Nanomedicine2013868970223467447
- DanhierFMessaoudiKLemaireLBenoitJPLagarceFCombined anti-galectin-1 and anti-EGFR siRNA-loaded chitosan-lipid nanocapsules decrease temozolomide resistance in glioblastoma: in vivo evaluationInt J Pharm20154811–215416125644286
- Balca-SilvaJMatiasDdo CarmoATamoxifen in combination with temozolomide induce a synergistic inhibition of PKC-pan in GBM cell linesBiochim Biophys Acta20151850472273225554223
- YoshimotoKMizoguchiMHataNComplex DNA repair pathways as possible therapeutic targets to overcome temozolomide resistance in glioblastomaFront Oncol2012218623227453
- YangRYYangKSPikeLJMarshallGRTargeting the dimerization of epidermal growth factor receptors with small-molecule inhibitorsChem Biol Drug Des20107611920456371
- LefrancFSadeghiNCambyIMetensTDewitteOKissRPresent and potential future issues in glioblastoma treatmentExpert Rev Anticancer Ther20066571973216759163
- MessaoudiKSaulnierPBoesenKBenoitJPLagarceFAnti-epidermal growth factor receptor siRNA carried by chitosan-transacylated lipid nanocapsules increases sensitivity of glioblastoma cells to temozolomideInt J Nanomedicine201491479149024711698
- MessaoudiKClavreulADanhierFSaulnierPBenoitJPLagarceFCombined silencing expression of MGMT with EGFR or galectin-1 enhances the sensitivity of glioblastoma to temozolomideEur J Nanomed20157297107
- XuHPaxtonJWWuZDevelopment of long-circulating pH-sensitive liposomes to circumvent gemcitabine resistance in pancreatic cancer cellsPharm Res20163371628163726964546
- ChungWGSandovalMASloatBRLansakaraPDCuiZStearoyl gemcitabine nanoparticles overcome resistance related to the overexpression of ribonucleotide reductase subunit M1J Control Release2012157113214021851843
- WangJYinCTangGLinXWuQGlucose-functionalized multidrug-conjugating nanoparticles based on amphiphilic terpolymer with enhanced anti-tumorous cell cytotoxicityInt J Pharm20134411–229129823194888
- ChengMGaoXWangYSynthesis of glycyrrhetinic acid-modified chitosan 5-fluorouracil nanoparticles and its inhibition of liver cancer characteristics in vitro and in vivoMar Drugs20131193517353624048270
- Johari-AharMBararJAlizadehAMDavaranSOmidiYRashidiMRMethotrexate-conjugated quantum dots: synthesis, characterisation and cytotoxicity in drug resistant cancer cellsJ Drug Target201624212013326176269
- YuanXJiWChenSA novel paclitaxel-loaded poly(d,l-lactide-co-glycolide)-Tween 80 copolymer nanoparticle overcoming multi-drug resistance for lung cancer treatmentInt J Nanomedicine2016112119213127307727
- WangLJiaEOvarian cancer targeted hyaluronic acid-based nanoparticle system for paclitaxel delivery to overcome drug resistanceDrug Deliv20162351810181726530693
- TangXLiangYFengXZhangRJinXSunLCo-delivery of docetaxel and Poloxamer 235 by PLGA-TPGS nanoparticles for breast cancer treatmentMater Sci Eng C Mater Biol Appl20154934835525686959
- SongXRZhengYHeGDevelopment of PLGA nanoparticles simultaneously loaded with vincristine and verapamil for treatment of hepatocellular carcinomaJ Pharm Sci201099124874487920821385
- TacarOSriamornsakPDassCRDoxorubicin: an update on anticancer molecular action, toxicity and novel drug delivery systemsJ Pharm Pharmacol201365215717023278683
- KopeckaJPortoSLusaSZoledronic acid-encapsulating self-assembling nanoparticles and doxorubicin: a combinatorial approach to overcome simultaneously chemoresistance and immunoresistance in breast tumorsOncotarget2016715207532077226980746
- YangHDengLLiTMultifunctional PLGA nanobubbles as theranostic agents: combining doxorubicin and P-gp siRNA co-delivery into human breast cancer cells and ultrasound cellular imagingJ Biomed Nanotechnol201511122124213626510307
- WangXTengZWangHIncreasing the cytotoxicity of doxorubicin in breast cancer MCF-7 cells with multidrug resistance using a mesoporous silica nanoparticle drug delivery systemInt J Clin Exp Pathol2014741337134724817930
- YuCZhouMZhangXWeiWChenXZhangXSmart doxorubicin nanoparticles with high drug payload for enhanced chemotherapy against drug resistance and cancer diagnosisNanoscale20157135683569025740312
- UnsoyGKhodadustRYalcinSMutluPGunduzUSynthesis of doxorubicin loaded magnetic chitosan nanoparticles for pH responsive targeted drug deliveryEur J Pharm Sci20146224325024931189
- GeneraliDBerrutiACappellettiMREffect of primary letrozole treatment on tumor expression of mTOR and HIF-1alpha and relation to clinical responseJ Natl Cancer Inst Monogr2015201551646626063890
- HoeflichKPGuanJEdgarKAThe PI3K inhibitor taselisib overcomes letrozole resistance in a breast cancer model expressing aromataseGenes Cancer201673–4738527382432
- NairHBHuffmanSVeerapaneniPHyaluronic acid-bound letrozole nanoparticles restore sensitivity to letrozole-resistant xenograft tumors in miceJ Nanosci Nanotechnol20111153789379921780370
- ChoSKPedramALevinERKwonYJAcid-degradable core-shell nanoparticles for reversed tamoxifen-resistance in breast cancer by silencing manganese superoxide dismutase (MnSOD)Biomaterials20133438102281023724055523
- DilrubaSKalaydaGVPlatinum-based drugs: past, present and futureCancer Chemother Pharmacol20167761103112426886018
- ZhouDCongYQiYOvercoming tumor resistance to cisplatin through micelle-mediated combination chemotherapyBiomater Sci20153118219126214201
- ScaranoWde SouzaPStenzelMHDual-drug delivery of curcumin and platinum drugs in polymeric micelles enhances the synergistic effects: a double act for the treatment of multidrug-resistant cancerBiomater Sci20153116317426214199
- JainRKStylianopoulosTDelivering nanomedicine to solid tumorsNat Rev Clin Oncol201071165366420838415
- OwensDE3rdPeppasNAOpsonization, biodistribution, and pharmacokinetics of polymeric nanoparticlesInt J Pharm200630719310216303268
- GaoHHeQThe interaction of nanoparticles with plasma proteins and the consequent influence on nanoparticles behaviorExpert Opin Drug Deliv201411340942024397260
- RattanRBhattacharjeeSZongHNanoparticle-macrophage interactions: a balance between clearance and cell-specific targetingBioorg Med Chem201725164487449628705434
- ChrastinaAMasseyKASchnitzerJEOvercoming in vivo barriers to targeted nanodeliveryWiley Interdiscip Rev Nanomed Nanobiotechnol20113442143721538941
- StylianopoulosTIntelligent drug delivery systems for the treatment of solid tumorsEur J Nanomed201681916
- EwaldBSampathDPlunkettWNucleoside analogs: molecular mechanisms signaling cell deathOncogene200827506522653718955977
- WongASooRAYongWPInnocentiFClinical pharmacology and pharmacogenetics of gemcitabineDrug Metab Rev2009412778819514966
- KimTMKimSAhnYOLeeSHKimDWHeoDSAnti-cancer activity of gemcitabine against natural killer cell leukemia/lymphomaLeuk Lymphoma201455494094323772641
- EdwardCPhysicians’ Cancer Chemotherapy Drug Manual 2015Burlington, MAJones and Bartlett Publishers2015
- FanPLiuLYinYMicroRNA-101-3p reverses gemcitabine resistance by inhibition of ribonucleotide reductase M1 in pancreatic cancerCancer Lett2016373113013726828016
- OrlandiACalegariMAMartiniMGemcitabine versus FOLFIRINOX in patients with advanced pancreatic adenocarcinoma hENT1-positive: everything was not too bad back when everything seemed worseClin Transl Oncol2016181098899526742940
- OhmineKKawaguchiKOhtsukiSAttenuation of phosphorylation by deoxycytidine kinase is key to acquired gemcitabine resistance in a pancreatic cancer cell line: targeted proteomic and metabolomic analyses in PK9 cellsPharm Res20122972006201622419259
- SongYBabaTMukaidaNGemcitabine induces cell senescence in human pancreatic cancer cell linesBiochem Biophys Res Commun2016477351551927311854
- HuangPChubbSHertelLWGrindeyGBPlunkettWAction of 2′,2′-difluorodeoxycytidine on DNA synthesisCancer Res19915122611061171718594
- ElnaggarMGiovannettiEPetersGJMolecular targets of gemcitabine action: rationale for development of novel drugs and drug combinationsCurr Pharm Des201218192811282922390765
- ShaoJLiuXZhuLYenYTargeting ribonucleotide reductase for cancer therapyExpert Opin Ther Targets201317121423143724083455
- GestoDSCerqueiraNMFernandesPARamosMJGemcitabine: a critical nucleoside for cancer therapyCurr Med Chem20121971076108722257063
- CiccoliniJSerdjebiCPetersGJGiovannettiEPharmacokinetics and pharmacogenetics of Gemcitabine as a mainstay in adult and pediatric oncology: an EORTC-PAMM perspectiveCancer Chemother Pharmacol201678111227007129
- MoriRIshikawaTIchikawaYHuman equilibrative nucleoside transporter 1 is associated with the chemosensitivity of gemcitabine in human pancreatic adenocarcinoma and biliary tract carcinoma cellsOncol Rep20071751201120517390066
- FarrellJJElsalehHGarciaMHuman equilibrative nucleoside transporter 1 levels predict response to gemcitabine in patients with pancreatic cancerGastroenterology2009136118719518992248
- WangCZhangWFuMYangAHuangHXieJEstablishment of human pancreatic cancer gemcitabine-resistant cell line with ribonucleotide reductase overexpressionOncol Rep201433138339025394408
- WeizmanNKrelinYShabtay-OrbachAMacrophages mediate gemcitabine resistance of pancreatic adenocarcinoma by upregulating cytidine deaminaseOncogene201433293812381923995783
- HuangYLiuXWangYHThe prognostic value of ribonucleotide reductase small subunit M2 in predicting recurrence for prostate cancersUrol Oncol201432151.e919
- GalmariniCMClarkeMLFaletteNPuisieuxAMackeyJRDumontetCExpression of a non-functional p53 affects the sensitivity of cancer cells to gemcitabineInt J Cancer200297443944511802204
- SkrypekNDucheneBHebbarMLeteurtreEvan SeuningenIJonckheereNThe MUC4 mucin mediates gemcitabine resistance of human pancreatic cancer cells via the concentrative nucleoside transporter familyOncogene201332131714172322580602
- RudinDLiLNiuNGemcitabine cytotoxicity: interaction of efflux and deaminationJ Drug Metab Toxicol2011210711021804948
- WattanawongdonWHahnvajanawongCNamwatNEstablishment and characterization of gemcitabine-resistant human cholangiocarcinoma cell lines with multidrug resistance and enhanced invasivenessInt J Oncol201547139841025998688
- MackeyJRYaoSYSmithKMGemcitabine transport in xenopus oocytes expressing recombinant plasma membrane mammalian nucleoside transportersJ Natl Cancer Inst199991211876188110547395
- MackeyJRManiRSSelnerMFunctional nucleoside transporters are required for gemcitabine influx and manifestation of toxicity in cancer cell linesCancer Res19985819434943579766663
- SpratlinJSanghaRGlubrechtDThe absence of human equilibrative nucleoside transporter 1 is associated with reduced survival in patients with gemcitabine-treated pancreas adenocarcinomaClin Cancer Res200410206956696115501974
- BrandiGDesertiMVasuriFMembrane localization of human equilibrative nucleoside transporter 1 in tumor cells may predict response to adjuvant gemcitabine in resected cholangiocarcinoma patientsOncologist201621560060727032872
- LiuZQHanYCZhangXPrognostic value of human equilibrative nucleoside transporter1 in pancreatic cancer receiving gemcitabin-based chemotherapy: a meta-analysisPLoS One201491e8710324475233
- EspinozaJAGarciaPBizamaCLow expression of equilibrative nucleoside transporter 1 is associated with poor prognosis in chemotherapy-naive pT2 gallbladder adenocarcinoma patientsHistopathology201668572272826266900
- GiovannettiEDel TaccaMMeyVTranscription analysis of human equilibrative nucleoside transporter-1 predicts survival in pancreas cancer patients treated with gemcitabineCancer Res20066673928393516585222
- BirdNTElmasryMJonesRImmunohistochemical hENT1 expression as a prognostic biomarker in patients with resected pancreatic ductal adenocarcinoma undergoing adjuvant gemcitabine-based chemotherapyBr J Surg2017104432833628199010
- NakanoTSaikiYKudoCAcquisition of chemoresistance to gemcitabine is induced by a loss-of-function missense mutation of DCKBiochem Biophys Res Commun201546441084108926196746
- ShimadaYOkumuraTSekineSClinicopathological significance of deoxycytidine kinase expression in esophageal squamous cell carcinomaMol Clin Oncol20131471672024649234
- JordheimLPCrosEGouyMHCharacterization of a gemcitabine-resistant murine leukemic cell line: reversion of in vitro resistance by a mononucleotide prodrugClin Cancer Res200410165614562115328204
- GalmariniCMClarkeMLJordheimLResistance to gemcitabine in a human follicular lymphoma cell line is due to partial deletion of the deoxycytidine kinase geneBMC Pharmacol20044815157282
- JordheimLPGalmariniCMDumontetCGemcitabine resistance due to deoxycytidine kinase deficiency can be reverted by fruitfly deoxynucleoside kinase, DmdNK, in human uterine sarcoma cellsCancer Chemother Pharmacol200658454755416463058
- KazunoHSakamotoKFujiokaAFukushimaMMatsudaASasakiTPossible antitumor activity of 1-(3-C-ethynyl-beta-D-ribo-pentofuranosyl)cytosine (ECyd, TAS-106) against an established gemcitabine (dFdCyd)-resistant human pancreatic cancer cell lineCancer Sci200596529530215904471
- KroepJRLovesWJvan der WiltCLPretreatment deoxycytidine kinase levels predict in vivo gemcitabine sensitivityMol Cancer Ther20021637137612477049
- SebastianiVRicciFRubio-ViqueiraBImmunohistochemical and genetic evaluation of deoxycytidine kinase in pancreatic cancer: relationship to molecular mechanisms of gemcitabine resistance and survivalClin Cancer Res20061282492249716638857
- FujitaHOhuchidaKMizumotoKGene expression levels as predictive markers of outcome in pancreatic cancer after gemcitabine-based adjuvant chemotherapyNeoplasia2010121080780820927319
- BouffardDYLaliberteJMomparlerRLKinetic studies on 2′,2′-difluorodeoxycytidine (gemcitabine) with purified human deoxycytidine kinase and cytidine deaminaseBiochem Pharmacol1993459185718618494545
- YoonKAWooSMHongEKCytidine deaminase as a molecular predictor of gemcitabine response in patients with biliary tract cancerOncology201589634535026418006
- OkazakiTJavleMTanakaMAbbruzzeseJLLiDSingle nucleotide polymorphisms of gemcitabine metabolic genes and pancreatic cancer survival and drug toxicityClin Cancer Res201016132032920028759
- TibaldiCGiovannettiEVasileECorrelation of CDA, ERCC1, and XPD polymorphisms with response and survival in gemcitabine/cisplatin-treated advanced non-small cell lung cancer patientsClin Cancer Res20081461797180318347182
- GilbertJASalavaggioneOEJiYGemcitabine pharmacogenomics: cytidine deaminase and deoxycytidylate deaminase gene resequencing and functional genomicsClin Cancer Res20061261794180316551864
- JordheimLPSèvePTrédanODumontetCThe ribonucleotide reductase large subunit (RRM1) as a predictive factor in patients with cancerLancet Oncol201112769370221163702
- IwamotoKNakashiroKTanakaHTokuzenNHamakawaHRibonucleotide reductase M2 is a promising molecular target for the treatment of oral squamous cell carcinomaInt J Oncol20154651971197725738429
- WangXZhenchukAWimanKGAlbertioniFRegulation of p53R2 and its role as potential target for cancer therapyCancer Lett200927611718760875
- NordlundNReichardPRibonucleotide reductasesAnnu Rev Biochem20067568170616756507
- ZengCFanWZhangXRRM1 expression is associated with the outcome of gemcitabine-based treatment of non-small cell lung cancer patients – a short reportCell Oncol (Dordr)201538431932526092210
- NakanoKBálintEAshcroftMVousdenKHA ribonucleotide reductase gene is a transcriptional target of p53 and p73Oncogene200019374283428910980602
- ChoECKuoMLLiuXYTumor suppressor FOXO3 regulates ribonucleotide reductase subunit RRM2B and impacts on survival of cancer patientsOncotarget20145134834484424947616
- LeccaPMethods of biological network inference for reverse engineering cancer chemoresistance mechanismsDrug Discov Today201419215116324211413
- NakahiraSNakamoriSTsujieMInvolvement of ribonucleotide reductase M1 subunit overexpression in gemcitabine resistance of human pancreatic cancerInt J Cancer200712061355136317131328
- ItoiTSofuniAFukushimaNRibonucleotide reductase subunit M2 mRNA expression in pretreatment biopsies obtained from unresectable pancreatic carcinomasJ Gastroenterol200742538939417530364
- AkitaHZhengZTakedaYSignificance of RRM1 and ERCC1 expression in resectable pancreatic adenocarcinomaOncogene200928322903290919543324
- CeppiPVolanteMNovelloSERCC1 and RRM1 gene expressions but not EGFR are predictive of shorter survival in advanced non-small-cell lung cancer treated with cisplatin and gemcitabineAnn Oncol200617121818182516980606
- KozinnSIHartyNJDelongJMMicroRNA Profile to Predict Gemcitabine Resistance in Bladder Carcinoma Cell LinesGenes Cancer201341–2616923946872
- NeesseAMichlPFreseKKStromal biology and therapy in pancreatic cancerGut201160686186820966025
- XuMLiLLiuZABCB2 (TAP1) as the downstream target of SHH signaling enhances pancreatic ductal adenocarcinoma drug resistanceCancer Lett2013333215215823340176
- KuramitsuYTabaKRyozawaSIdentification of up- and down-regulated proteins in gemcitabine-resistant pancreatic cancer cells using two-dimensional gel electrophoresis and mass spectrometryAnticancer Res20103093367337220944110
- FeigCGopinathanANeesseAChanDSCookNTuvesonDAThe pancreas cancer microenvironmentClin Cancer Res201218164266427622896693
- MittalAChitkaraDBehrmanSWMahatoRIEfficacy of gemcitabine conjugated and miRNA-205 complexed micelles for treatment of advanced pancreatic cancerBiomaterials201435257077708724836307
- MengHZhaoYDongJTwo-wave nanotherapy to target the stroma and optimize gemcitabine delivery to a human pancreatic cancer model in miceACS Nano2013711100481006524143858
- LicciardiMPaolinoDCeliaCGiammonaGCavallaroGFrestaMFolate-targeted supramolecular vesicular aggregates based on polyaspartyl-hydrazide copolymers for the selective delivery of antitumoral drugsBiomaterials201031287340735420609469
- VenturaCACannavaCStancanelliRGemcitabine-loaded chitosan microspheres. Characterization and biological in vitro evaluationBiomed Microdevices201113579980721607718
- JiaLPreparation, physicochemical characterization and cytotoxicityin vitroof gemcitabine-loaded PEG-PDLLA nanovesiclesWorld J Gastroenterol2010168100820180242
- ZhaoLPXueCZhangJWExpression of RRM1 and its association with resistancy to gemcitabine-based chemotherapy in advanced nasopharyngeal carcinomaChin J Cancer2012311047648322692073
- XieHJiangWJiangJPredictive and prognostic roles of ribonucleotide reductase M1 in resectable pancreatic adenocarcinomaCancer2013119117318122736490
- LeeJJMaengCHBaekSKThe immunohistochemical overexpression of ribonucleotide reductase regulatory subunit M1 (RRM1) protein is a predictor of shorter survival to gemcitabine-based chemotherapy in advanced non-small cell lung cancer (NSCLC)Lung Cancer201070220521020223551
- UlkerMDumanBBSahinBGumurduluDERCC1 and RRM1 as a predictive parameter for non-small cell lung, ovarian or pancreas cancer treated with cisplatin and/or gemcitabineContemp Oncol (Pozn)201519320721326557761
- GongWZhangXWuJRRM1 expression and clinical outcome of gemcitabine-containing chemotherapy for advanced non-small-cell lung cancer: a meta-analysisLung Cancer201275337438021889227
- KhatriNRathiMBaradiaDMisraAcRGD grafted siRNA nano-constructs for chemosensitization of gemcitabine hydrochloride in lung cancer treatmentPharm Res201532380681824792823
- WongananPChungWGZhuSKiguchiKDigiovanniJCuiZSilencing of ribonucleotide reductase subunit M1 potentiates the antitumor activity of gemcitabine in resistant cancer cellsCancer Biol Ther2012131090891422785206
- DamarajuVLDamarajuSYoungJDNucleoside anticancer drugs: the role of nucleoside transporters in resistance to cancer chemotherapyOncogene200322477524753614576856
- MarechalRMackeyJRLaiRHuman equilibrative nucleoside transporter 1 and human concentrative nucleoside transporter 3 predict survival after adjuvant gemcitabine therapy in resected pancreatic adenocarcinomaClin Cancer Res20091582913291919318496
- GreenhalfWGhanehPNeoptolemosJPPancreatic cancer hENT1 expression and survival from gemcitabine in patients from the ESPAC-3 trialJ Natl Cancer Inst20141061djt34724301456
- NakagawaNMurakamiYUemuraKCombined analysis of intratumoral human equilibrative nucleoside transporter 1 (hENT1) and ribonucleotide reductase regulatory subunit M1 (RRM1) expression is a powerful predictor of survival in patients with pancreatic carcinoma treated with adjuvant gemcitabine-based chemotherapy after operative resectionSurgery2013153456557523253379
- MorinagaSNakamuraYWatanabeTImmunohistochemical analysis of human equilibrative nucleoside transporter-1 (hENT1) predicts survival in resected pancreatic cancer patients treated with adjuvant gemcitabine monotherapyAnn Surg Oncol201219Suppl 3S558S56421913012
- KondoNMurakamiYUemuraKCombined analysis of dihydropyrimidine dehydrogenase and human equilibrative nucleoside transporter 1 expression predicts survival of pancreatic carcinoma patients treated with adjuvant gemcitabine plus S-1 chemotherapy after surgical resectionAnn Surg Oncol201219Suppl 3S646S65522086444
- WongananPLansakaraPDZhuSJust getting into cells is not enough: mechanisms underlying 4-(N)-stearoyl gemcitabine solid lipid nanoparticle’s ability to overcome gemcitabine resistance caused by RRM1 overexpressionJ Control Release20131691–2172723570983
- HungSWMarracheSCumminsSDefective hCNT1 transport contributes to gemcitabine chemoresistance in ovarian cancer subtypes: overcoming transport defects using a nanoparticle approachCancer Lett2015359223324025600708
- FreseKKNeesseACookNnab-Paclitaxel potentiates gemcitabine activity by reducing cytidine deaminase levels in a mouse model of pancreatic cancerCancer Discov20122326026922585996
- CouvreurPStellaBReddyLHSqualenoyl nanomedicines as potential therapeuticsNano Lett20066112544254817090088
- RejibaSReddyLHBigandCParmentierCCouvreurPHajriASqualenoyl gemcitabine nanomedicine overcomes the low efficacy of gemcitabine therapy in pancreatic cancerNanomedicine20117684184921419876
- ReddyLHFerreiraHDubernetCSqualenoyl nanomedicine of gemcitabine is more potent after oral administration in leukemia-bearing rats: study of mechanismsAnticancer Drugs20081910999100618827565
- ReddyLHDubernetCMouelhiSLMarquePEDesmaeleDCouvreurPA new nanomedicine of gemcitabine displays enhanced anticancer activity in sensitive and resistant leukemia typesJ Control Release20071241–2202717878060
- SloatBRSandovalMALiDIn vitro and in vivo anti-tumor activities of a gemcitabine derivative carried by nanoparticlesInt J Pharm20114091–227828821371545
- LansakaraPDRodriguezBLCuiZSynthesis and in vitro evaluation of novel lipophilic monophosphorylated gemcitabine derivatives and their nanoparticlesInt J Pharm20124291–212313422425885
- MengHWangMLiuHUse of a lipid-coated mesoporous silica nanoparticle platform for synergistic gemcitabine and paclitaxel delivery to human pancreatic cancer in miceACS Nano2015943540355725776964
- IyerAKKhaledGFangJMaedaHExploiting the enhanced permeability and retention effect for tumor targetingDrug Discov Today20061117–1881281816935749
- MatsumuraYMaedaHA new concept for macromolecular therapeutics in cancer chemotherapy: mechanism of tumoritropic accumulation of proteins and the antitumor agent smancsCancer Res19864612 Pt 1638763922946403
- AcharyaSSahooSKPLGA nanoparticles containing various anti-cancer agents and tumour delivery by EPR effectAdv Drug Deliv Rev201163317018320965219
- MaedaHNakamuraHFangJThe EPR effect for macromolecular drug delivery to solid tumors: Improvement of tumor uptake, lowering of systemic toxicity, and distinct tumor imaging in vivoAdv Drug Deliv Rev2013651717923088862
- FangJNakamuraHMaedaHThe EPR effect: unique features of tumor blood vessels for drug delivery, factors involved, and limitations and augmentation of the effectAdv Drug Deliv Rev201163313615120441782
- XuYMengHDuFPreparation of intravenous injection nanoformulation of VESylated gemcitabine by co-assembly with TPGS and its anti-tumor activity in pancreatic tumor-bearing miceInt J Pharm2015495279279726410754
- SobotDMuraSRouquetteMCirculating Lipoproteins: A Trojan Horse Guiding Squalenoylated Drugs to LDL-Accumulating Cancer CellsMol Ther20172571596160528606375
- MoysanEBastiatGBenoitJPGemcitabine versus modified gemcitabine: a review of several promising chemical modificationsMol Pharm201310243044422978251
- FedericoCMorittuVMBrittiDTrapassoECoscoDGemcitabine-loaded liposomes: rationale, potentialities and future perspectivesInt J Nanomedicine201275423543623139626
- ZhuSWongananPLansakaraPDO’MaryHLLiYCuiZThe effect of the acid-sensitivity of 4-(N)-stearoyl gemcitabine-loaded micelles on drug resistance caused by RRM1 overexpressionBiomaterials20133492327233923261218
- PapaALBasuSSenguptaPBanerjeeDSenguptaSHarfoucheRMechanistic studies of Gemcitabine-loaded nanoplatforms in resistant pancreatic cancer cellsBMC cancer20121241922998550
- DamanZOstadSAminiMGilaniKPreparation, optimization and in vitro characterization of stearoyl-gemcitabine polymeric micelles: a comparison with its self-assembled nanoparticlesInt J Pharm20144681–214215124731731
- PapaALSidiquiABalasubramanianSUPEGylated liposomal gemcitabine: insights into a potential breast cancer therapeuticCell Oncol (Dordr)201336644945724081907
- NyhanWNucleotide Synthesis Via Salvage PathwayeLSChichesterJohn Wiley & Sons Ltd2005
- KangNJunAHBhutiaYDKannanNUnadkatJDGovindarajanRHuman equilibrative nucleoside transporter-3 (hENT3) spectrum disorder mutations impair nucleoside transport, protein localization, and stabilityJ Biol Chem201028536283432835220595384
- JiangJNeubauerBLGraffJRExpression of group IIA secretory phospholipase A2 is elevated in prostatic intraepithelial neoplasia and adenocarcinomaAm J Pathol2002160266767111839587
- AbeTSakamotoKKamoharaHHiranoYKuwaharaNOgawaMGroup II phospholipase A2 is increased in peritoneal and pleural effusions in patients with various types of cancerInt J Cancer19977432452509221799
- OkaYOgawaMMatsudaYSerum immunoreactive pancreatic phospholipase A2 in patients with various malignant tumorsEnzyme199043280881702053
- QuachNDMockJNScholpaNERole of the phospholipase A2 receptor in liposome drug delivery in prostate cancer cellsMol Pharm201411103443345125189995
- MockJNCostynLJWildingSLArnoldRDCummingsBSEvidence for distinct mechanisms of uptake and antitumor activity of secretory phospholipase A2 responsive liposome in prostate cancerIntegr Biol (Camb)20135117218222890797
- ZhuGMockJNAljuffaliICummingsBSArnoldRDSecretory phospholipase A(2) responsive liposomesJ Pharm Sci201110083146315921455978
- HofmannDMesserschmidtCBannwarthMBLandfesterKMailanderVDrug delivery without nanoparticle uptake: delivery by a kiss-and-run mechanism on the cell membraneChem Commun (Camb)201450111369137124346146
- MarkmanJLRekechenetskiyAHollerELjubimovaJYNanomedicine therapeutic approaches to overcome cancer drug resistanceAdv Drug Deliv Rev20136513–141866187924120656
- CoscoDRoccoFCerutiMVonoMFrestaMPaolinoDSelf-assembled squalenoyl-cytarabine nanostructures as a potent nanomedicine for treatment of leukemic diseasesInt J Nanomedicine201272535254622679366
- AryaGVandanaMAcharyaSSahooSKEnhanced antiproliferative activity of herceptin (HER2)-conjugated gemcitabine-loaded chitosan nanoparticle in pancreatic cancer therapyNanomedicine20117685987021550422
- LiJDiYJinCGemcitabine-loaded albumin nanospheres (GEM-ANPs) inhibit PANC-1 cells in vitro and in vivoNanoscale Res Lett20138117623594566
- MaksimenkoACaronJMouginJDesmaeleDCouvreurPGemcitabine-based therapy for pancreatic cancer using the squalenoyl nucleoside monophosphate nanoassembliesInt J Pharm20154821–2384625448549
- XuHTPaxtonJWWuZMDevelopment of long-circulating pH-sensitive liposomes to circumvent gemcitabine resistance in pancreatic cancer cellsPharm Res-Dordr201633716281637
- ChitkaraDMittalABehrmanSWKumarNMahatoRISelf-assembling, amphiphilic polymer-gemcitabine conjugate shows enhanced antitumor efficacy against human pancreatic adenocarcinomaBioconjug Chem20132471161117323758084