Abstract
Advances in nanomedicine have become indispensable for targeted drug delivery, early detection, and increasingly personalized approaches to cancer treatment. Nanoparticle-based drug-delivery systems have overcome some of the limitations associated with traditional cancer-therapy administration, such as reduced drug solubility, chemoresistance, systemic toxicity, narrow therapeutic indices, and poor oral bioavailability. Advances in the field of nanomedicine include “smart” drug delivery, or multiple levels of targeting, and extended-release drug-delivery systems that provide additional methods of overcoming these limitations. More recently, the idea of combining smart drug delivery with extended-release has emerged in hopes of developing highly efficient nanoparticles with improved delivery, bioavailability, and safety profiles. Although functionalized and extended-release drug-delivery systems have been studied extensively, there remain gaps in the literature concerning their application in cancer treatment. We aim to provide an overview of smart and extended-release drug-delivery systems for the delivery of cancer therapies, as well as to introduce innovative advancements in nanoparticle design incorporating these principles. With the growing need for increasingly personalized medicine in cancer treatment, smart extended-release nanoparticles have the potential to enhance chemotherapy delivery, patient adherence, and treatment outcomes in cancer patients.
Introduction
Nanoparticles (NPs) have been successfully adopted in electronics, food and agriculture, biosensing, and some areas of nanomedicine; however, their translation to clinical oncology remains limited.Citation1 Although nanomedicine-based drug delivery has been dominating the field of cancer research over the past decade, only a dozen US Food and Drug Administration (FDA)-approved NPs are currently available.Citation2 As such, there is a growing need for novel NPs in oncology to improve drug delivery for cancer treatment, mainly through target-driven design.Citation3 Currently, poor patient outcomes are attributed in part to the low stability, drug solubility and bioavailability, poor pharmacokinetic (PK) and pharmacodynamic (PD) parameters, aspecific distribution, cytotoxicity, and chemoresistance that are characteristic of traditional chemotherapeutic agents.Citation4,Citation5 As a result, nanomedicine-based drug delivery has been of increasing research interest because NPs have been shown to substantially improve the therapeutic efficacy of chemotherapeutic agents by overcoming the various anatomical, physiological, chemical, and clinical barriers associated with intravenous drug administration.Citation4 However, the lack of efficacy in the clinic has made innovative NP-design and -delivery approaches increasingly important in the translation of these promising therapies from bench to bedside.
Recent trends in NP design suggest that there is a focus on multifunctional targeting or “smart” delivery, which incorporates multiple complementary targeting strategies, including passive, active, and stimuli-responsive targeting.Citation6,Citation7 The addition of extended drug-release properties could further improve multifunctional targeting, whereby smart extended-release NPs (SER NPs) can provide additional physiological and clinical benefits, particularly in the treatment of cancer, where drug delivery poses significant challenges.Citation8 SER NPs can be modified with active and stimuli-responsive targeting to take into account the pathophysiological characteristics of the tumor, and can be matched to the patient’s lifestyle by modifying the desired length and duration of extended release. As such, SER NPs represent a promising option in advancing the field of personalized medicine.
Despite the abundance of promising preclinical evidence for several NP formulations, there has been limited clinical advancement. This limitation is attributed to the tendency of NPs to accumulate in the liver and spleen, their low therapeutic efficiency inside tumors, and barriers associated with NP entry into the cell.Citation9,Citation10 The widespread use of NPs in cancer therapy has also been precluded due to traditional NPs exhibiting suboptimal stability in the body, slow intracellular drug release, low accumulation in tumor sites, and low cellular uptake and aspecific targeting.Citation5 Furthermore, the complexity of the multistep process required for NP preparation, safety of the components involved, and stability of the final product contribute to the barriers that limit the use of NPs in clinical practice.Citation1
This review aims to briefly summarize the physiological and clinical relevance and characteristics of SER NPs. We introduce and describe new advancements in nanodelivery systems that have either successfully combined both approaches or display the potential to do so in hopes of improving the efficacy of delivering chemotherapeutic agents to patients. Here, the particular focus is on the recently developed folic acid (FA)-functionalized nanopolymer (FA–diaminobutyric acid [DABA]–styrene-alt-maleic anhydride [SMA]), as well as injectable Pickering emulsions (IPEs), due to their novelty and particular relevance in cancer therapy. We discuss the application of SER NPs in the context of benefits and drawbacks of increasingly complex NP systems, which have the potential to advance the field of cancer treatment and delivery.
Smart nanoparticles
NP drug-delivery systems that can release the drug in response to specific physiological triggers, at the appropriate time, and at the correct target site are referred to as smart NPs.Citation11 For this review, smart NPs refer to those incorporating all three delivery strategies: passive, active, and stimuli-responsive targeting,Citation6 as summarized in . The enhanced permeability and retention (EPR) effect, or passive targeting, is the most basic targeting strategy employed by smart NPs.Citation12 The EPR effect and its limitations have been reviewed extensively elsewhere.Citation6,Citation9,Citation13–Citation18 Briefly, the EPR effect is a complex phenomenon dictated by the degree of leaky tumor vascularization and poor lymphatic draining that varies significantly between tumor types, anatomical sites, and patients.Citation14,Citation15,Citation17,Citation19 However, the high intestinal fluid pressure in tumors can prevent successful uptake and homogenous drug distribution.Citation16,Citation18 Long-circulating liposomes, polymers, and micelles are examples of NPs that take advantage of the leaky vasculature of tumors that ultimately allows for the entrapment and accumulation of NPs.Citation19–Citation21
Active targeting
The delivery of NPs can be enhanced by functionalizing NPs with a variety of targeting molecules that are commonly over-expressed on malignant cells, such as carbohydrates, antibodies, and ligands.Citation6,Citation22 Specific examples of targeting moieties used to functionalize NPs are summarized in . The efficacy of active targeting is restricted to the treatment of tumors expressing a high level of the targeted biomarker, requiring a thorough understanding of the target tumor, or patient stratification.Citation3,Citation23 There are several classes of NPs in development that employ active targeting strategies. However, apart from denileukin diftitox (an engineered protein combining IL2 and diphtheria toxin), which was approved in 2008 but clinically discontinued in 2016, there have not been any FDA-approved NPs using active targeting in the treatment of cancer.Citation20,Citation24 The lack of clinical application may be due to the reported significant dose loss due to lysosomal digestion following receptor-mediated endocytosis, aspecificity of the targeting ligand, and immunogenicity of the targeting ligand, all of which lead to blood clearance.Citation25 Therefore, it is imperative that there is ongoing research exploring methods of overcoming these limitations, as well as incorporating designs that achieve optimal biodistribution and metabolism for maximal drug delivery and efficacy.Citation25
Table 1 Active targeting strategies and potential functionalization of “smart” nanoparticles
Stimuli-responsive targeting
Smart NPs may undergo physicochemical structural changes that result in drug release at a particular time and location when exposed to external stimuli, such as heat, pH changes, light, electric/magnetic fields, and ultrasound.Citation1,Citation6,Citation11 These structural changes are a particularly important characteristic of smart NPs, as they allow for the potential to exploit the inherent characteristics of the tumor microenvironment (TME). NPs that contain pendant acidic or basic groups that accept or release protons in response to pH changes are deemed “pH-sensitive” and take advantage of the acidic pH characteristic of tumor cells (6.5–6.8), or endosomes/lysosomes (4.0–6.3).Citation11,Citation26–Citation29 pH-responsive targeting is advantageous due to its suitability for the delivery of thermolabile drugs; however, a lack of toxicity data on pH-sensitive delivery is a drawback of this targeting strategy.Citation11
Physiological importance of smart-nanoparticle drug delivery
Smart NP-delivery systems are advantageous because they have the potential to overcome some of the physiological obstacles faced by traditional chemotherapeutics, summarized in . These obstacles include, but are not limited to, renal filtration, hepatic degradation, high tumor-cell density, high interstitial fluid pressure, and drug-efflux pumps.Citation4 As previously discussed, through multiple levels of targeting, smart NPs can preferentially accumulate at the site of the tumor, achieving higher therapeutic indices.Citation58
Figure 2 Physiological benefits of “smart” and extended-release nanopolymers.
Note: Smart and extended-release nanopolymers each confer physiological benefits, with some being characteristic of both nanoparticle types.
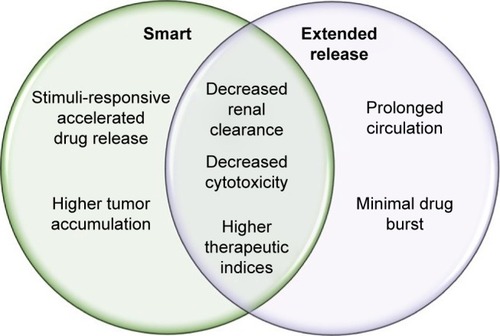
Active targeting has been shown to enhance therapeutic efficiency by minimizing off-target effects and reducing the amount of drug that must be administered to achieve a therapeutic response.Citation6 For instance, loaded FA-functionalized, pH-sensitive polymeric micelles and transferrin-conjugated NPs exhibit higher efficacy compared to their respective free drugs in drug-resistant mouse models.Citation59–Citation61 NPs employing active targeting have also been reported to enhance siRNA delivery to tumors compared to traditional NPs that lack active targeting properties.Citation62 FDA-approved nab-paclitaxel, an albumin-based formulation of paclitaxel (Ptx), has significantly enhanced the therapeutic index of solvent-based Ptx and prompted further research into albumin-based targeting strategies for other chemotherapeutics, such as docetaxel.Citation63 These examples of NPs employing active-targeting strategies will be discussed in detail under the section on the clinical importance of smart-NP drug delivery.
Owing to higher drug concentrations achieved by active targeting compared to passive targeting, active-targeting strategies have been suggested to show improved therapeutic efficacy due to improved drug penetration.Citation64 However, it has been postulated that coordinating targeting approaches may provide a solution to poor tumor penetration in instances where active targeting does not achieve effective drug accumulation.Citation65 As such, the incorporation of stimuli-responsive targeting is becoming an increasingly popular trend in NP design, as it has been shown to accelerate drug release, improve cellular binding and internalization, and lead to more efficient drug perfusion throughout the tumor volume.Citation66 Additionally, stimuli-responsive delivery is also used to achieve controlled release, which is critical for steady-state drug delivery.Citation18 Controlled drug release has been reported to reduce the toxic side effects of drugs and improve the function of insoluble drugs, such as doxorubicin.Citation67
High tumor-cell density has been an obstacle in the treatment of many cancers, due to their characteristically dense TME. Most notably, pancreatic ductal adenocarcinoma (PDAC) possesses an impenetrable stroma due to its fibrotic nature, in addition to hypovascular and hypoperfused tumor vessels, all of which contribute to increased interstitial pressure.Citation68,Citation69 This limitation has been partially overcome by nab-Ptx, which due to the functionalization of Ptx with albumin, forms a complex with the Gp60 receptor and SPARC, both commonly overexpressed on stromal fibroblasts in PDAC.Citation70 The benefits observed in the PK, PD, and side-effect profiles of currently approved anticancer NPs, such as nab-Ptx, which currently only employs passive targeting, provides a strong rationale for developing NPs with multiple levels of targeting.
Clinical importance of smart-nanoparticle drug delivery
Cancer patients often face severe cytotoxic effects, as most anticancer agents are administered at the maximum tolerated dose, leading to discontinuation of life-saving treatment.Citation71 Intravenous delivery of anticancer agents is also limited due to low drug efficacy, the need for hospitalization, frequent administration, and high cost.Citation4 Smart NPs can allow for the administration of lower doses of drugs while maintaining effective intracellular concentrations, thereby widening the therapeutic window of anticancer agents.Citation72 For instance, nanopolymers possess high drug-loading capacity, which allows them to achieve the same efficacy with smaller doses while minimizing systemic side effects. Therefore, smart-NP formulations that can increase tumor accumulation and specificity for cancer cells through coordinated targeting strategies can provide a therapeutic option that significantly reduces systemic side effects.Citation65
Tran et alCitation73 summarized the recent successes in cancer nanomedicine in the clinic. In particular, the clinical trials of irinotecan liposomal (Onivyde), which employs stimuli-responsive properties, were highlighted as a case study in the clinical success of nanomedicine in cancer therapy. Other NP “success stories” include doxorubicin liposomal (Doxil), a passive targeting FDA-approved liposomal NP that encapsulates doxorubicin for the treatment of ovarian cancer. The half-life of liposomal doxorubicin is approximately 100 times that of free doxorubicin, and the NP formulation reduces cardiotoxicity, which is significant dose-limiting toxicity associated with free doxorubicin.Citation74,Citation75 As a result, liposomal doxorubicin decreases the need for hospitalization and enables continuation of life-saving treatment.
Another pertinent example is albumin-bound Ptx, also known as nab-Ptx (Abraxane), an injectable formulation of Ptx that is used to treat breast, lung, and pancreatic cancers, among others. Nab-Ptx was developed to avoid the toxicities of polyoxyethylated castor-oil solvent (Cremophor) used for Ptx because of its poor aqueous solubility.Citation76 Cremophor has been associated with several toxicities, including hypersensitivity reactions, neutropenia, peripheral neuropathy, and liver toxicity. Nab-Ptx has also been shown to significantly increase progression-free survival of metastatic breast cancer patients compared to solvent-based Ptx.Citation77 Ptx targets metabolically active cancer cells by preventing the typical breakdown of microtubules during cell division. Nab-Ptx is a cell-cycle phase-specific drug, mainly targeting cancer cells in the G2/M phase of the cell cycle, and in combination with gemcitabine (Gem) was FDA-approved as a first-line treatment for PDAC in 2013.Citation70 Chiorean et alCitation78 examined changes in Karnofsky performance status (KPS) for patients’ well-being during treatment with nab-Ptx plus Gem vs Gem alone as first-line therapy for metastatic pancreatic cancer in the Phase III MPACT trial. The study concluded that the two treatment arms had generally comparable time to any KPS deterioration, and while baseline KPS, neutrophil:lymphocyte ratio, age, liver metastases, and region had a significant effect on time to definitive KPS deterioration, treatment arm did not. The limited efficacy of nab-Ptx is attributed to its cell cycle phase-specificity for the G2/M phase, but ongoing research on trapping cancer cells in a sensitive phase of the cell cycle may further enhance the therapeutic effects of nab-Ptx even in treatment-resistant tumors such as pancreatic cancer.Citation79
Preliminary studies from our laboratory using highly aggressive human pancreatic cancer in RAGxCγ double-mutant mice have shown that the tumor xenografts develop not only resistance to nab-Ptx treatment after 66 days, but also metastasize to the liver and lung. Tumor xenografts from the same animal model of human pancreatic cancer also developed resistance to Gem treatment after 30 days, as well as a massive liver metastatic burden. It should be noted that the toxicity profile for Gem and nab-Ptx compared with FOLFIRINOX (a combination of folinic acid, fluorouracil, irinotecan, and oxaliplatin) is similar. However, patients receiving the FOLFIRINOX regimen every 2 weeks with a 46-hour fluorouracil infusion developed higher hematological toxicities and growth-factor usage, while the nab-Ptx plus Gem weekly infusion regimen had higher rates of neuropathy.
The development of chemoresistance is a significant obstacle encountered in oncology, which may be circumvented through the use of smart NPs. One approach is to modify and optimize NPs to avoid cell-surface-pump-mediated multidrug resistance (MDR) involving Pgp-mediated resistance.Citation80 Patients who previously failed drug therapies have demonstrated clinical responses to anticancer agents encapsulated in NPs, bypassing the surface-pump-mediated resistance in humans.Citation81–Citation83 A recent smart-NP formulation incorporating redox-sensitive release of Ptx and an MMP2-triggered mitochondrion-targeting conjugate for mitochondrial delivery of Ptx demonstrated higher cellular uptake and cytotoxicity in MCF7 breast cancer cells.Citation84 A detailed description of how stimuli-responsive targeting strategies may overcome MDR has been eloquently discussed in detail in a recent review by Zhou et al.Citation85
Another approach to overcoming MDR is through the use of gene-silencing drugs. To reverse MDR in human breast cancer cells, Liu et alCitation86 designed a new carrier system loaded with an active siRNA-targeting MDR1 gene. Briefly, phospholipid (PL)-modified cationic polyamidoamine (PAMAM)–siMDR1 complexes were designed to form hybrid nanocomplexes (PL dendriplexes). This new delivery system demonstrated higher gene-silencing efficiency, enhanced cellular uptake of siMDR1 (inhibits mRNA and MDR1 protein expression), decreased Pgp expression, raised cellular accumulation of doxorubicin, and inhibited tumor-cell migration.Citation86 Additionally, when Ptx was introduced into cells after incubation with siMDR1 for 6 hours, either empty or entrapped in dendri-plexes/PL dendriplexes and transfected with the only siMDR1, flow-cytometry analyses showed that the PL dendriplex–Ptx combination resulted in a 45.2% induction of cell apoptosis. In contrast, there was 4.15%, 4.97%, and 7.45% induction of apoptosis in control, dendriplexes, and PL-dendriplex cohorts, respectively, the results of which suggest that dendriplexes and PL dendriplexes are not highly cytotoxic, but work synergistically with either siMDR1 or Ptx.
In another study, Li et alCitation87 provided compelling data showing reversal of MDR in MCF7/ADR breast cancer cells using a novel hR3–siMDR1–PAMAM complex (HSPC) in their design of a delivery system. Their complexes were designed and engineered using hR3 anti-EGFR antibody to self-assemble HSPCs via electrostatic interactions for siRNA delivery. The HSPCs were shown to have lower cytotoxicity, higher cellular uptake, and enhanced endosomal escape. It is noteworthy that HSPCs encapsulating siMDR1 reduced MDR1 gene expression by 99.4% with up to sixfold enhancement compared to siMDR1 alone, as well as increased doxorubicin accumulation, downregulated Pgp expression, and suppressed cellular migration in MCF7/ADR breast cancer cells. Furthermore, the combined effect of Ptx with siMDR1-loaded HSPCs showed synergism in reversing MDR, all of which inhibited cell growth and induced cell apoptosis. Other reports have proposed novel NP applications to reverse MDR, such as LAH4L1, an amphipathic cationic polypeptide, to form nanocomplexes via electrostatic interactions with siRNA to have high transfection efficiency in delivering siMDR1 to reverse MDR in ovarian cancer cells.Citation88
Other reports have reversed MDR using codelivery of MDR1-targeting siRNA and doxorubicin using a novel cationic poly(lactide-co-glycolic acid) (PLGA) nanoformulation,Citation89 formulating MDR1 ribozymes with N-(1-[2,3-dileoyloxy]propyl)-N,N,N-trimethylammonium methyl sulfate to form a liposomal complex,Citation90 and constructing a pDNA-iMDR1-shRNA containing a U6-RNA gene-promoter-driven expression vector encoding anti-MDR1/Pgp shRNA molecules.Citation91 Li et alCitation92 gave an essential detailed review on the recent developments on the application in PAMAM dendrimers as useful carriers for drug and genetic material (pDNA, siRNA) delivery in cancer therapy, as well as their use in hybrid NPs, and conjugated or loaded in other NP systems. They also highlighted the efficacy of PAMAM dendrimers in overcoming problems with tumor MDR. Kesharwani et alCitation93 have eloquently highlighted the challenges for effective delivery of siRNAs and oligonucleotides. The report discusses improvements in the design template, with a particular focus on describing hyperbranched PAMAM dendrimers and their unique three-dimensional architecture and nanoscale size.
The cationic surface charge of PAMAM dendrimers not only serve as siRNA-condensing agents and robust nanovectors for targeted delivery, but their functionality also permits conjugation of drugs and genes for the development of hybrid systems for combination therapy. PAMAM-dendrimer toxicity has been reviewed in detail in different models by Naha et al.Citation94 Collectively, the toxic response of PAMAM dendrimers correlated with amine-terminated residue and increased systematically with generation. Replacing the amine groups with hydroxyl (-OH) and carboxylic acid (-COOH) terminated PAMAM dendrimers, resulting in significantly less toxicity. It is noteworthy that the PK parameters, biodistribution, biodegradation, and chronic toxicity of PAMAM dendrimers are not well known.
de Jong and Borm reviewed the safety evaluation of NP formulations for drug delivery, their applications, and hazards.Citation95 The engineering and design of NPs for drug delivery must include the specificity of drug targeting, delivery, and reduced toxicity, while maintaining therapeutic effects, increased safety and biocompatibility, and increased development of new safe medicines. Drug-delivery systems should be designed with the following principles in mind: drug incorporation and release, formulation stability, shelf life, biocompatibility, biodistribution, targeting, and functionality.
One of the drawbacks of administering multiple doses of systemic siRNA carriers is their decreased fluctuation in serum. To overcome this issue, Kim and SongCitation96 developed a targetable, injectable, and noncytotoxic micelleplex hydrogel. The report highlighted the dual function of the micelleplex hydrogel carrier and provided supporting data to demonstrate its active systemic targetable siRNA-delivery carrier with an additive tunable therapeutic time by controlled release of the delivery carrier after only one injection of the hydrogel. Briefly, the micelleplex hydrogel was fabricated by mixing a functionalized folate-linked polyethylenimine-conjugated polyorganophosphazene (FPP), a biodegradable polymer, and amphiphilic forming micelles with siRNA at 4°C. After injection into the body, sol–gel transition in solution as a function of temperature occurred due to hydrophobic interactions. The hydrogel released dissociated micelleplexes, targeting the folate moiety in a time-dependent manner. It is noteworthy that the polyethylenimine moiety in the FPP formulation formed a micelleplex with anionic siRNAs by ionic interactions, which turned into a gel after subcutaneous injection, due to the properties of polyorganophosphazene. These micelleplexes were then released slowly by dissolution and degradation of the gel into the bloodstream directly or through lymphatic vessels. The released micelleplexes in circulation accumulated in the tumor region via the EPR effect, and targeted and enter only tumor cells via FA-receptor-mediated endocytosis. Gene suppression by the siRNA delivered through endocytosis process was caused by cleavage of specifically targeted mRNA only in tumor cells.
To explore current developments in siRNA-delivery systems using NPs in oncology, particularly those that encapsulate siRNA for the targeted treatment of cancer, Kim et alCitation97 reviewed the current status of clinical trials related to siRNA-based cancer therapy, elucidating the remaining issues that need to be overcome to establish a successful therapy. The report also described various promising design strategies of delivery vehicles for stable and targeted siRNA delivery, including prospects for future design. Additionally, Sarett et alCitation98 reported analyses of complicating factors relevant to the clinical adoption of local siRNA therapeutics, such as polymers, siRNA carriers, and construct types studied in basic research. Among local siRNA therapeutics in the clinic, the authors identified that systems that achieve sustained delivery are mostly absent. Clinical approaches use simple topical treatments or local-injection strategies, due to the ease of use mandated by the regulatory process. Sarett et alCitation98 recommended a balance between complicated design processes and therapeutic efficacy for controlled-release delivery systems to maintain strong gene-silencing activity without repeated doses. To this end, the report outlines the earliest to most recent clinical trials for local siRNA-delivery systems.
Classical methods of smart-drug delivery
There are three generations of delivery system that have been developed based on their degree of specific targeting.Citation99,Citation100 First-generation NPs, defined as those that lack specific targeting, include the successful FDA-approved liposomal daunorubicin, liposomal doxorubicin, and PEGylated liposomal doxorubicin.Citation65 Second-generation NPs have relied on active targeting, while third-generation NPs employ a multistage strategy or smart-drug delivery.Citation99 There is ongoing debate on the cost-versus-benefit analysis of increasingly complex drug-delivery systems, such as smart NPs.Citation1,Citation65
Recent clinical discontinuation of FDA-approved denileukin diftitox, an active-targeting NP for cutaneous T-cell lymphoma, due to production difficulties is evidence of practical limitations of developing increasingly complex NPs.Citation24 Functionalizing NPs increases the complexity of the preparation process, and is associated with increased cost and regulatory barriers that must be overcome.Citation65 To mitigate these challenges, targeting ligands or bioresponsive materials can be coupled directly with NP starting materials before NP formulation, allowing for tighter control over synthesis, uniformity, and target-ligand density.Citation65 Newer formulations of smart-drug delivery must not only improve the PK and PD parameters of free drugs, but also be relatively straightforward to produce to maximize the chances of clinical translation.
summarizes the current FDA-approved NPs and describes the type of targeting, cancer indication, advantages, and drawbacks. Other FDA-approved NPs for the treatment of cancer that are not discussed in include trastuzumab emtansine (Kadcyla) and NanoTherm (MagForce), as they are beyond the scope of smart NPs. It is noteworthy that the therapeutic efficacy of these NPs is to reduce side effects and selectively accumulate in the disease area for a prolonged period of time with high controllability.Citation101 According to a recent review article by Liu et al,Citation101 the following should be characteristic properties of NPs and the development process: clinically useful and reproducible formulations, high verifiability, precise control over the preparation process, generating nanocarriers with required features, high batch-to-batch reproducibility, design simplicity, and industrial upscaling feasibility. Therefore, future research on smart NPs for controlled drug delivery should focus on more stimulus-sensitive nanomedicine to be clinically utilized.
Table 2 Current US FDA-approved nanoparticles and type of targeting employed, indications, advantages, and drawbacks
Novel approaches to smart-nanoparticle delivery systems
Advancements in NP design have resulted in multifunctional targeting and multispecificity.Citation65 Recently, the copolymer FA-DABA-SMA was developed, which relies on the EPR effect, FA-receptor targeting, and pH sensitivity.Citation6,Citation18 This smart-delivery system incorporates all three forms of targeting. The amphiphilic polymer is characterized by a hydrophilic outer shell containing a hydrophobic core, which permits encapsulation of hydrophobic chemotherapeutic agents. The polymer exists in cylindrical conformation at neutral pH within the body, and collapses to release its cargo at an acidic pH that is typically characteristic of the TME and lysosomes,Citation18 as depicted in . Li et alCitation18 described and summarized the synthesis and characterization of FA-DABA-SMA. In brief, the SMA serves as the template for the NP. The linker, DABA, is used to improve the accessibility of the FA-DABA ligand, which is synthesized by combining FA, dicyclohexylcarbodiimide, and hydroxysuccinimide, resulting in a stable functionalized FA-DABA-SMA polymer at neutral pH and self-assembles into nanostructures in dilute concentrations.Citation18 Due to the reported anticancer effects and fluorescent capabilities of curcumin, it is used in NP studies as a hydrophobic drug load and a fluorescent marker.Citation126,Citation127 Here, the encapsulation process is chemical-free, as curcumin diffuses passively into the core of the NP, thereby minimizing undesired toxicity. This smart-delivery system is particularly advantageous due to its noninvasive delivery and drug release, because the FA-DABA-SMA polymer circulates in the bloodstream and pH changes trigger drug release at the tumor site.Citation5
Figure 3 A pH-responsive, “smart” active polymer-delivery system.
Notes: Yellow spheres represent folic acid molecules, green represents hydrophobic drugs, blue shows the hydrophilic part of the polymer, and gray is the hydrophobic part of the polymer. Reprinted from Biophys Chem, 214–215, Li X, Mctaggart M, Malardier-Jugroot C, Synthesis and characterization of a pH responsive folic acid functionalized polymeric drug delivery system, 17–26, copyright 2016, with permission from Elsevier.Citation18
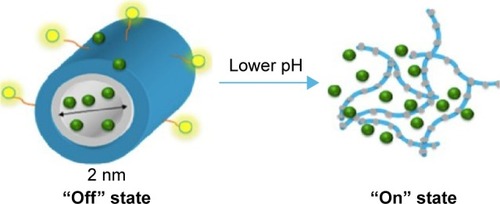
Preclinical evidence of curcumin-loaded FA-DABA-SMA polymer demonstrates significant toxicity and cell death in PANC1 pancreatic cancer cells, with the empty FA-DABA-SMA polymer being nontoxic.Citation6 Approved anticancer drugs currently used in the clinic are being tested in these NPs for their loading capacities and release profiles. Collectively, this smart FA-DABA-SMA NP shows promise for its use in a wide variety of cancer types, due to the specific overexpression of the FA receptor in pancreatic, epithelial, ovarian, cervical, breast, lung, kidney, colorectal, and brain tumors.Citation128
Other recent advances in smart-drug delivery involve the use of multiple types of functionalization and triggering stimuli for drug release. For example, doxorubicin-loaded micelles have recently been developed with reactive oxygen species and dual pH sensitivity.Citation26 Another recent advance in NP design is multispecificity, which is particularly relevant to the dynamic nature of tumor markers and is often a limiting factor of active targeting.Citation65
Stimuli-responsive targeting can be optimized by pairing nanomedicine-based drug delivery with other modalities. For example, magnetic resonance imaging-guided high-intensity focused ultrasound combines different technologies, such as image guidance for radiation treatment and triggering of smart-nanomedicine systems for delivery of drugs. In the case of thermosensitive liposomal doxorubicin, the release is triggered at the tumor site by locally increasing the temperature through high-intensity ultrasound radiation.Citation129
Despite promising advances, some improvements to current smart-NP design could be considered. To circumvent the issue of significant dose loss associated with active targeting, lysosome-escape mechanisms that rely on pH sensitivity have been developed.Citation25 Lysosomal escape aims to minimize inactivation of NPs by acid hydrolases found in endolysosomal compartments, thereby representing a critical design strategy and the focus of ongoing research efforts.Citation130 Additionally, incorporating extended-release design principles into smart NPs would lead to targeted and prolonged-action therapies with unique physiological and clinical advantages, as summarized in and .
Extended-release nanoparticle-delivery systems
Extended-release drug-delivery systems are designed to release drugs over a prolonged period employing steady-rate drug release or controlled release to achieve stable and higher therapeutic potential while minimizing adverse side effects.Citation131 Extended-release NPs used in a clinical setting hold drugs either on their surface or adsorbed in a matrix that attains sustained release.Citation132 Currently, hydrophobic biodegradable polymeric NPs are commonly used for the continuous supply of encapsulated therapeutic agents at the site of the tumour.Citation58
NPs can undergo certain cell-surface modifications to achieve more prolonged circulation. In particular, PEGylation which involves the conjugation of polyethylene glycol (PEG) to a nanopolymer, has been used extensively in nanomedicine.Citation20 It has been shown to increase drug-hydrodynamic radius, prolong plasma-retention time, decrease proteolysis, decrease renal excretion, and shield antigenic determinants from immunodetection.Citation133–Citation137 Etirinotecan pegol is an FDA-approved long-acting topoisomerase 1 inhibitor for breast cancer designed to improve the PK properties and tolerability of irinotecan whose enhanced therapeutic response has been attributed to the more prolonged circulation of PEGylated nanomaterials.Citation138
Physiological importance of extended-release drug delivery
Limiting factors in the physiological success of NPs include size and surface characteristics, as they play roles in circulation time and elimination from the body.Citation139 The optimal NP size that is correlated with more prolonged circulation, increased accumulation, and decreased renal NP clearance is 40–200 nm.Citation140 NPs that are 10–100 nm in size have been shown to increase circulation time of the drug for as long as 160 hours, with modifications in surface area and charge allowing for the ability to reach optimal loading and control of dosing.Citation141 For instance, nanocrystalline cellulose possesses an extensive negatively charged surface area that allows it to bind to large amounts of the drug.Citation142 It can be coated with cationic surfactant (cetyltrimethylammonium bromide), resulting in an altered release profile due to its ability to deliver hydrophobic anticancer agents as a result of its hydrophobic domain.Citation142 High loading capacity in combination with extended-release profiles can reduce the dosage required, thereby minimizing aspecific cytotoxicity.
Flexibility in the functionalization of nanomatrices permits the ability to obtain the desired drug-release profile. Solid-lipid NPs are particularly advantageous due to their combination of polymeric micelles and lipid-based liposomes, which allows them to deliver both hydrophobic and hydrophilic therapeutic agents, demonstrate excellent bioavailability, scalability, and physical stability.Citation131 Premature bursting and rapid removal from circulation reduce the efficacy of the treatment. However, PEGylation coating may offer an alternative option that facilitates drug release over an extended period.Citation143 An essential consideration in optimal extended-release delivery systems is minimal drug burst, with most of the drug released according to a specific release profile.Citation6,Citation18
Clinical importance of extended-release drug delivery
Extended-release NPs are attractive therapeutic options for the long-term treatment of complex chronic diseases, such as cancer, owing to drug release over a prolonged period.Citation144,Citation145 These characteristics of extended-release NPs can achieve improved patient compliance, better life-cycle management of drugs, and extended relief of symptoms, due to a reduction in fluctuating drug levels.Citation146 The clinical advantages of smart NPs are summarized in .
Challenges with patient adherence span several stages of treatment, and are primarily attributed to multiple dosing, which is standard in cancer treatment.Citation123,Citation147–Citation149 Patients typically experience challenges when entering a different phase of their treatment cycle, particularly in initiating implementation and treatment persistence.Citation150 Extended-release drug-delivery systems can overcome these challenges in patient adherence by decreasing dosing cycles. Adherence increased from 59% for multiple dosing to 80% with a single daily dose in male patients on long-term antihypertensive medication.Citation151 Conversely, a decline in adherence was observed in patients on a chemotherapy regimen of three doses/day.Citation152
The number of injections required over the course of treatment is an essential consideration in drug delivery for patients and clinicians alike. For instance, PEG–IFNα conjugates have shown clinically superior antiviral activity to free IFNα, and are approved for hepatitis C therapy.Citation153 PEG–IFNα has been useful in the treatment of melanoma and renal-cell carcinoma, and is currently being tested in other solid tumors.Citation154–Citation156
Classical methods of extended-release delivery systems
Controlled drug delivery has been an area of research interest over the past six decades, with early extended-release formulations including oral and transdermal sustained-release systems.Citation100 In oncology, FDA-approved leuprolide for injectable suspensions can achieve slow and sustained drug release for the treatment of prostate cancer.Citation157 Additionally, non-PEGylated liposomal doxorubicin for the treatment of metastatic breast cancer resembles a prolonged infusion that forms a mononuclear phagocyte-system depot capable of slow release into blood circulation.Citation158 Although promising as delivery systems, these methods of extended-release delivery have faced scrutiny, and their limitations are briefly summarized in .
Table 3 Classical methods of extended-release delivery systems and their limitations
Novel approaches to extended-release drug delivery
A number of novel NP formulations for extended drug release have been developed in recent years. For instance, PLGA polymers have been shown to attain 82% of the cumulative release of dexamethasone in 17 days.Citation130 Clinically, cancer patients undergoing chemotherapy are often given dexamethasone to counteract the adverse side effects of their antitumor treatment. The extended release of dexamethasone from PGLA polymers may overcome the long-term use of dexamethasone, but may result in thrush, bone loss, cataracts, easy bruising, or muscle weakness. PLGA is a copolymer composed of lactic and glycolic acid monomers that are used as a drug-delivery vehicle. PLGA is susceptible to hydrolytic degradation of the ester linkage on the polymer backbone, which results in the release of the encapsulated drug.Citation174 Several PLGA drug-delivery applications, such as Lupron Depot, Risperdal Consta, and Zoladex, have been approved by the FDA, European Medicines Agency, and Health Canada.Citation123 However, therapeutics demonstrating extended-term sustained release (weeks or longer) from PLGA polymers are mostly hydrophobic in nature, with molecular weights of 400–1,000 Da. The design and fabrication of extended-term, sustained release, low-molecular-weight hydrophilic drugs from PLGA has been challenging. One of the most significant challenges in drug delivery is the inherent difficulty in full encapsulation and retention, followed by long-term and targeted delivery of low-molecular-weight hydrophilic therapeutics at the tumor site.
To that end, we have recently reported that oseltamivir phosphate (OP) can prevent the tumor neovascularization, growth, and metastasis of human triple-negative breastCitation175,Citation176 and pancreaticCitation177 cancer cells in heterotopic xenografts of these tumors in RAGxCγ double-mutant mice. Findings have also included a novel signaling paradigm that regulates EGFR,Citation177 insulin receptors,Citation176,Citation178,Citation179 and Toll-like receptors,Citation180 all of which play essential roles in multistage tumorigenesis.Citation181 As such, the controlled release of hydrophilic OP from a biodegradable PLGA cylinder (PLGA-OP) implanted at the tumor site was investigated for its role in limiting tumor neovascularization, growth, and metastasis.Citation182 As shown in , PLGA-OP cylinders showed a 20%–25% release profile within 48 hours followed by a continuous metronomic low-dose release of 30%–50% OP for an additional 16 days, with all of the OP released by day 30. To show the therapeutic effect of PLGA-OP, PLGA-OP containing 20 mg OP and empty cylinders surgically implanted at the tumor site of heterotopic xenografts of human pancreatic tumors in RAGxCγ double-mutant mice impeded tumor neovascularization and growth rate and spread to the liver and lungs compared to the untreated cohort over 30 days ().Citation182
Figure 5 (A) RAGxCγ double-mutant mice bearing heterotopic xenografts of pancreatic PANC1 tumors. (B) Extended release of OP from PLGA-OP surgical implants, measurement of tumor volumes days post implantation, tumor weights at necropsy, and number of liver metastatic clusters.
Note: Copyright © 2015. Dove Medical Press. Reproduced from Hrynyk M, Ellis JP, Haxho F, et al. Therapeutic designed poly (lactic-co-glycolic acid) cylindrical oseltamivir phosphate-loaded implants impede tumor neovascularization, growth and metastasis in mouse model of human pancreatic carcinoma. Drug Des Devel Ther. 2015;9: 4573–4586.Citation182
Abbreviations: OP, oseltamivir phosphate; PLGA, poly(lactic-co-glycolic acid).
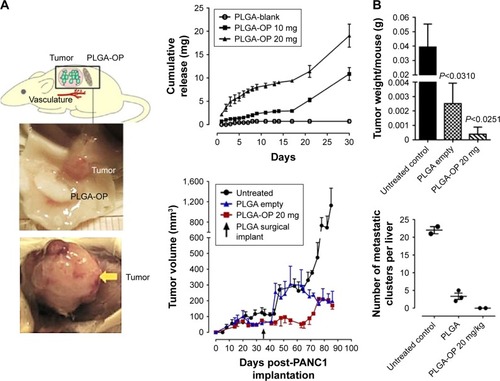
Despite difficulties with the encapsulation of small hydrophilic drugs, OP-particulate encapsulation within polymeric PLGA cylinders using the formulation method described in these studies resulted in full retention of the drug with no loss of therapeutic activity. Collectively, delivery of OP using this delivery system in the treatment of pancreatic cancer cells resulted in disabling the survival mechanism of pancreatic cancer with acquired chemoresistance to Gem.Citation183
Furthermore, the double-layered Gem- and OP-loaded PLGA cylindrical implants were designed and engineered to provide an optimal combinatorial and sequential sustained extended-release of the hydrophilic drugs for 30 days. Here, OP and Gem encapsulated in inner/outer distinct layers of implantable double-layered PLGA cylinders disabled pancreatic cancer cell survival and increased sensitivity to therapy of two low-molecular-weight hydrophilic chemotherapeutics.
The development of amphiphilic polymeric vesicles capable of encapsulating large quantities of a chemotherapeutic agent and improving its targeting efficiency and bioavailability are emerging new delivery platforms for nanomedicine in cancer research. Polymeric micelles have been modified by attaching ligand molecules to the outer shell of the micelle with specificity for biomarkers that are overly expressed on cancer cells. Such ligands as peptides, antibodies, sugars, and aptamers have enabled significant improvement in tumor selectivity and overall therapeutic efficiency of cancer treatments. However, these specific delivery vehicles are limited by low overtime stability, especially with antibodies, triggering immunogenic adverse side effects and high costs. Multiple functionalities using complex hydrophobic/hydrophilic polymeric structures prepared with highly controlled molecular weights and defined architectures are needed to enable self-assembled, stimuli-responsive regions (CO2, pH, and temperature) for triggered drug release and reactive groups for drug conjugation cross-linking and “click” chemistry. To this end, Kapishon et alCitation184 designed a specific smart chemotherapeutic delivery platform for active tumor targeting. They fabricated a new polymeric micelle-delivery system for active tumor targeting followed by micelle–drug internalization via receptor-induced endocytosis. By decorating amphiphilic block copolymeric micelles with OP at the hydrophilic end, oseltamivir–PEG methyl ether methacrylate-block-poly(methyl methacrylate) using reversible addition–fragmentation chain transfer living radical polymerization, the OP micelles had self-assembling properties to give wormlike micellar structures with a molecular weight of 80,000 g/mol. These newly designed micelles for active tumor targeting have triple functionality, such that they exert an antitumor cell effect and at the same time deliver and internalize hydrophobic chemotherapeutic agents.
IPEs are another promising novel method of extended-release NPs compared to older formulations.Citation185 In our studies, IPEs were designed and fabricated to replace our surgically implantable PGLA drug-delivery vehicle, as previously discussed, while providing similar dosing and release profiles at the tumor site to the surgical implant.Citation185 The IPE formulation is unique in that compared to previous extended-release drug-delivery systems, it has been developed to adjust the release rate to occur within a target of 30 days.Citation185 The mono-glyceride-stabilized PE (MSPE) approach is cost-effective, as it utilizes simple equipment with readily available reagents and has a formulation time of <1 hour.Citation185 In brief, emulsions consist of two liquids that remain insoluble in each other and include an emulsifier that stabilizes the mixture. The emulsion is stabilized by glycerol monostearate containing ≧ 90% glycerol monostearate (GMS). To produce a smooth emulsion from two separate mixtures, an oil phase and a water phase are prepared and subsequently mixed at a fixed temperature and mixing speed. The oil phase is prepared by mixing GMS with canola oil, resulting in a 4%-by-weight oil phase. The solutes (OP, sodium chloride, and sodium citrate) are added to distilled water to prepare a 4%-by-aqueous-weight phase. These mixtures are then warmed separately to cause melting of GMS, followed by adding the aqueous to the oil phase, producing a coarse water-in-oil emulsion of 20%:80%. This coarse emulsion is then added to a stirrer that carries out stirring and processing to produce an emulsion that is quench-cooled, resulting in a viscous final product.
Preclinical data have shown a decrease in PANC1 pancreatic cancer cell viability after these cells were exposed to MSPE at multiple time points leading up to 30 days.Citation185 This effect was unchanged when comparisons were made between PANC1 exposed to OP only, compared to OP released from the emulsion. A near-linear sustained release of OP from MSPE-encapsulated OP was observed, with >5% of the total drug loading being released over a 30-day period.Citation185 Regarding stability, the viscosity of the emulsion remains unchanged, and both phases remain stable.Citation185 These results suggest that the viscous MSPE emulsion will concentrate at the site of injection.
Future directions: the potential for smart extended-release drug-delivery nanoparticles
Smart NPs that can exhibit sustained, extended-release drug delivery would offer additional advantages from both clinical and physiological standpoints. Studies continue to highlight the importance of localizing treatment and prolonging drug release, both of which achieve therapeutic benefits with limited adverse effects, due to fewer drug interactions and reduced drug-level fluctuations.Citation186 Therefore, modifying NPs to exhibit both extended-release and targeting properties should continue to be an area of intense research focus.
One strategy of developing SER drug-delivery NPs could involve PEGylation of smart NPs to prolong circulation time, as previously discussed. PEGylation of self-assembled hyaluronic acid NPs has been shown to improve active and passive targeting in tumor-bearing mice, reduce liver uptake, and increase circulation time and NP accumulation in tumors.Citation187 Given recent advances in antibody-mediated therapy in cancer research, it is perhaps unsurprising that antibody-targeted NPs represent promising active-targeting strategies that are undergoing rigorous clinical research.Citation65 As such, smart NPs incorporating antibody-mediated targeting that also exhibit extended-release could be of great interest in cancer therapy in light of the benefits of prolonged drug release outlined herein.
Alternatively, current extended-release NPs can be modified to achieve a higher degree of targeting. Dendrimer-based stealth NPs have been used to encapsulate anastrozole, commonly used for the treatment of breast cancer, to improve its water-solubility and extended-release profile.Citation188 Extended release of encapsulated anastrozole was achieved for 40 days, and the authors suggested that the stealth NPs could be further functionalized to construct a targeted therapeutic agent for breast cancer treatment.Citation188 Similarly, IPEs can be functionalized with FA for greater specificity toward malignant cells, due to their overexpression of the FA receptor. Some NPs with targeting properties that display extended release have been in development, such as silk fibroin functionalized with the cyclic arginine–glycine–aspartic acid–phenylalanine–lysine (RGDfK) peptide, and chlorin e6 (a second-generation photosensitizer with antitumor activity when used in conjunction with irradiation), which displays both smart drug delivery and sustained release. Here, active targeting is achieved using the cyclic RGDfK peptide, which targets αVβ3 integrins.Citation50 Silk-fibroin NPs have also displayed extended-release, as only 33% of fluorouracil had been released by the end of the third day in a human gastric cancer model.Citation50 The combination of active targeting and extended-release resulted in a significant reduction in tumor burden in vivo with excellent biocompatibility and safety, with encapsulation of cisplatin, doxorubicin, and Ptx being suggested as future directions to test the efficacy of this formulation.Citation50
Due to the increased focus on patient selection, stratification, and personalized medicine, nanomedicine-based drug-delivery systems in the clinical setting need to take smart and extended-release drug delivery into consideration.Citation21,Citation189 Personalized medicine incorporates a variety of factors, including genetic, genomic, and clinical markers, as well as the patient’s age, race, and other environmental factors.Citation190 Future work looking at additional genetic and epigenetic biomarkers will assist in the development of novel targeting moieties for increasingly effective and customized therapeutic options.Citation191 Potential biomarkers could target solid tumors, tumor vasculature, and supporting cells within the TME.Citation65 Depending on the pathophysiology of the patient’s tumor, appropriate functionalization and triggers for drug release can also significantly improve efficacy.Citation192 For instance, the limitations of the EPR effect may be overcome by selecting patients with angiogenic tumors, using vasodilating agents, such as TNFα, and using imaging strategies to monitor delivery of the NPs.Citation193
Despite the benefits of SER NPs, a significant concern with increasingly complex drug-delivery systems is cost-effective upscaling manufacturing.Citation186 Maintaining the original properties of NPs during the development process is expected to be an obstacle in complex NP formulation.Citation186 The examples explained in detail here, namely FA-DABA-SMA and IPE formulations, involve straightforward preparation processes, safe intermediates, and inexpensive materials.Citation6,Citation18,Citation185 As such, they represent good candidates as potential SER NPs, and future work should explore incorporating both smart and extended-release design principles in these formulations.
Alternatively, self-assembled nanostructures may offer innovative approaches to limitations encountered in the development of NPs. Li et alCitation194 highlighted in an impressive review article cooperation principles in various self-assembled nanostructures based on the principles of molecular cooperation in self-assembled systems. The report focused on the bottom-up chemistry and material-science considerations of nanomedicine with reference to conformation change-induced cooperation in natural self-assembled nanostructures, noncovalent interactions involving the molecular basis of supramolecular cooperation, phase transition-induced cooperation in synthetic self-assembled nanostructures, the fTM molecular mechanism of supramolecular cooperation, and lastly, supramolecular cooperation in addressing the challenges in medicine. Luo et alCitation195 reported a novel nanovaccine, the mechanism of which was dependent on the STING and not on Toll-like receptors or MAVS pathways. The formulation of the nanovaccine is minimalistic, and made by mixing antigen with a synthetic polymeric NP, PC7A, which generated a strong cytotoxic T-cell response with low systemic cytokine expression. PC7A NPs achieved efficient cytosolic delivery of tumor antigens to antigen-presenting cells in draining lymph nodes, which led to increased surface presentation while simultaneously activating type I IFN-stimulated genes. This nanovaccine design produced potent inhibition of tumor growth in melanoma, highlighting nanovaccine formulation as an intense area of research interest.
Conclusion
Recent advances in NP design have led to the development of drug-delivery systems that can overcome several physiological and clinical barriers associated with the traditional administration of chemotherapeutic agents. Smart-drug delivery aims to localize treatment to tumors to reduce cytotoxicity and enhance the therapeutic index by using multifunctional targeting strategies. While older formulations have relied primarily on the EPR effect, recent advances, such as the FA-DABA-SMA polymer, employ three levels of targeting, a chemical-free process of drug encapsulation, noninvasive drug delivery and release, to achieve cytotoxicity against pancreatic cancer cells. Future work should investigate encapsulation of conventional chemotherapeutic drugs into the FA-DABA-SMA polymer, its application toward other cancers that overexpress FA, and improving its extended- release profile. IPEs represent an additional promising NP formulation for extended-drug release. Modifying IPEs to include active targeting properties could successfully combine the concepts of smart delivery with extended release to achieve more significant therapeutic responses, minimal side effects, and improved patient adherence. Therefore, combining different targeting strategies and optimizing the drug-release profile for a coordinated approach toward drug delivery is of particular importance in the drug-delivery field, but the cost and technical difficulties of achieving this complexity must also be considered. This continuously growing field allows for a novel way for clinically implementing personalized medicine in the form of NP-delivery systems, and requires a more profound understanding of the patient’s tumor pathophysiology to be successful.
Author contributions
MRS conceived the review and obtained funding. RVK, KB, BQ, and AD designed the study, carried out the searches, refined and selected the study design, extracted data, and conducted the thematic analyses. RVK, KB, and BQ led the writing of the draft as contributing first authors. MRS finalized the manuscript. All authors contributed toward data analysis, drafting and critically revising the paper and agree to be accountable for all aspects of the work. All authors approved the final manuscript.
Disclosure
This work was supported in part by grants to MRS from the Natural Sciences and Engineering Research Council of Canada (NSERC) and private-sector cancer funding from the Josefowitz family and Encyt Technologies Inc to MRS. RVK is a recipient of the Queen’s Graduate Award (QGA). BQ is a recipient of the QGA and part of the 2017 Terry Fox Research Institute Transdisciplinary Training Program in Cancer Research. The authors report no other conflicts of interest in this work.
References
- TorchilinVPMultifunctional, stimuli-sensitive nanoparticulate systems for drug deliveryNat Rev Drug Discov2014131181382725287120
- FornagueraCGarcía-CelmaMPersonalized nanomedicine: a revolution at the nanoscaleJ Pers Med201774E1229023366
- HareJILammersTAshfordMBPuriSStormGBarrySTChallenges and strategies in anti-cancer nanomedicine development: an industry perspectiveAdv Drug Deliv Rev2017108253827137110
- LammersTImproving the efficacy of combined modality anticancer therapy using HPMA copolymer-based nanomedicine formulationsAdv Drug Deliv Rev201062220323019951732
- YangMYuLGuoRDongALinCZhangJA modular coassembly approach to all-in-one multifunctional nanoplatform for synergistic codelivery of doxorubicin and curcuminNanomaterials201883E16729543780
- LiXSzewczukMMalardier-JugrootCFolic acid-conjugated amphiphilic alternating copolymer as a new active tumor targeting drug delivery platformDrug Des Devel Ther20161041014110
- GuFXKarnikRWangAZTargeted nanoparticles for cancer therapyNano Today2007231421
- RodzinskiAGuduruRLiangPTargeted and controlled anticancer drug delivery and release with magnetoelectric nanoparticlesSci Rep201662086726875783
- Pérez-HerreroEFernández-MedardeAAdvanced targeted therapies in cancer: drug nanocarriers, the future of chemotherapyEur J Pharm Biopharm201593527925813885
- BlancoEShenHFerrariMPrinciples of nanoparticle design for overcoming biological barriers to drug deliveryNat Biotechnol201533994195126348965
- HoneyPJRijoJAnjuAAnoopKRSmart polymers for the controlled delivery of drugs: a concise overviewActa Pharm Sin B20144212012726579373
- MatsumuraYMaedaHA new concept for macromolecular therapeutics in cancer chemotherapy: mechanism of tumoritropic accumulation of proteins and the antitumor agent smancsCancer Res19864612 Pt 1638763922946403
- DanhierFFeronOPréatVTo exploit the tumor microenvironment: passive and active tumor targeting of nanocarriers for anti-cancer drug deliveryJ Control Release2010148213514620797419
- BaeYHDrug targeting and tumor heterogeneityJ Control Release200913312318848589
- BaeYHParkKTargeted drug delivery to tumors: myths, reality and possibilityJ Control Release2011153319820521663778
- HeldinCHRubinKPietrasKÖstmanAHigh interstitial fluid pressure: an obstacle in cancer therapyNat Rev Cancer200441080681315510161
- JainRKStylianopoulosTDelivering nanomedicine to solid tumorsNat Rev Clin Oncol201071165366420838415
- LiXMctaggartMMalardier-JugrootCSynthesis and characterization of a pH responsive folic acid functionalized polymeric drug delivery systemBiophys Chem2016214–2151726
- WangMThanouMTargeting nanoparticles to cancerPharmacol Res2010622909920380880
- BoboDRobinsonKJIslamJThurechtKJCorrieSRNanoparticle-based medicines: a review of FDA-approved materials and clinical trials to datePharm Res201633102373238727299311
- LammersTRizzoLYStormGKiesslingFPersonalized nanomedi-cineClin Cancer Res201218184889489422829203
- ByrneJDBetancourtTBrannon-PeppasLActive targeting schemes for nanoparticle systems in cancer therapeuticsAdv Drug Deliv Rev200860151615162618840489
- RenouxBRaesFLegiganTTargeting the tumour microenvironment with an enzyme-responsive drug delivery system for the efficient therapy of breast and pancreatic cancersChem Sci2017853427343328507714
- WangZZhengQZhangHOntak-like human IL-2 fusion toxinJ Immunol Methods2017448515828551309
- ChenWCZhangAXLiSDLimitations and niches of the active targeting approach for nanoparticle drug deliveryEur J Nanomed201242–48993
- QuCXLiJZZhouYJTargeted delivery of doxorubicin via CD147-mediated ROS/pH dual-sensitive nanomicelles for the efficient therapy of hepatocellular carcinomaAAPS J20182023429476273
- AdochiteRCMoshnikovaACarlinSDTargeting breast tumors with pH (low) insertion peptidesMol Pharm20141182896290525004202
- AdochiteRCMoshnikovaAGolijaninJAndreevOAKatenkaNVReshetnyakYKComparative study of tumor targeting and biodistribution of pH (low) insertion peptides (pHLIP peptides) conjugated with different fluorescent dyesMol Imaging Biol201618568669627074841
- WeerakkodyDMoshnikovaAel-SayedNSNovel pH-sensitive cyclic peptidesSci Rep201663132227515582
- VogelSMMinshallRDPilipovićMTiruppathiCMalikABAlbumin uptake and transcytosis in endothelial cells in vivo induced by albumin-binding proteinAm J Physiol Lung Cell Mol Physiol20012816L1512L152211704548
- FreiEAlbumin binding ligands and albumin conjugate uptake by cancer cellsDiabetol Metab Syndr2011311121676260
- DesaiNPTrieuVHwangLYWuRSoon-ShiongPGradisharWJImproved effectiveness of nanoparticle albumin-bound (nab) paclitaxel versus polysorbate-based docetaxel in multiple xenografts as a function of HER2 and SPARC statusAnticancer Drugs200819989990918766004
- ChoiKYSaravanakumarGParkJHParkKHyaluronic acid-based nanocarriers for intracellular targeting: Interfacial interactions with proteins in cancerColloids Surf B Biointerfaces201299829422079699
- TaheriADinarvandRAtyabiFNouriFTargeted delivery of methotrexate to tumor cells using biotin functionalized methotrexate-human serum albumin conjugated nanoparticlesJ Biomed Nanotechnol20117674375322416572
- TaheriADinarvandRNouriFSUse of biotin targeted methotrexate-human serum albumin conjugated nanoparticles to enhance metho-trexate antitumor efficacyInt J Nanomedicine201161863187421931482
- PatilYBTotiUSKhdairAMaLPanyamJSingle-step surface functionalization of polymeric nanoparticles for targeted drug deliveryBiomaterials200930585986619019427
- ParveenSSahooSKEvaluation of cytotoxicity and mechanism of apoptosis of doxorubicin using folate-decorated chitosan nanoparticles for targeted delivery to retinoblastomaCancer Nanotechnol201011–6476226069479
- HrkachJvon HoffDAliMMPreclinical development and clinical translation of a PSMA-targeted docetaxel nanoparticle with a differentiated pharmacological profileSci Transl Med20124128128ra139
- von HoffDDMitaMMRamanathanRKPhase I study of PSMA-targeted docetaxel-containing nanoparticle BIND-014 in patients with advanced solid tumorsClin Cancer Res201622133157316326847057
- HongMZhuSJiangYNovel anti-tumor strategy: PEG-hydroxycamptothecin conjugate loaded transferrin-PEG-nanoparticlesJ Control Release20101411222919735683
- VisserCCStevanovićSVoorwindenLHValidation of the transferrin receptor for drug targeting to brain capillary endothelial cells in vitroJ Drug Target200412314515015203893
- GanCWFengSSTransferrin-conjugated nanoparticles of poly(lactide)-d-α-tocopheryl polyethylene glycol succinate diblock copolymer for targeted drug delivery across the blood–brain barrierBiomaterials201031307748775720673685
- JainAChasooGSinghSKSaxenaAKJainSKTransferrin-appended PEGylated nanoparticles for temozolomide delivery to brain: in vitro characterisationJ Microencapsul2011281212821171813
- CampERWangCLittleECTransferrin receptor targeting nanomedicine delivering wild-type p53 gene sensitizes pancreatic cancer to gemcitabine therapyCancer Gene Ther201320422222823470564
- LiuJWeiTZhaoJMultifunctional aptamer-based nanoparticles for targeted drug delivery to circumvent cancer resistanceBiomaterials201691445626994877
- SilvaCOPetersenSBReisCPEGF functionalized polymer-coated gold nanoparticles promote EGF photostability and EGFR internalization for photothermal therapyPLoS One20161110e016541927788212
- Cirstoiu-HapcaABucheggerFBossyLKosinskiMGurnyRDelieFNanomedicines for active targeting: physico-chemical characterization of paclitaxel-loaded anti-HER2 immunonanoparticles and in vitro functional studies on target cellsEur J Pharm Sci200938323023719632322
- WangZChuiWKHoPCHoPCDesign of a multifunctional PLGA nanoparticulate drug delivery system: evaluation of its physicochemical properties and anticancer activity to malignant cancer cellsPharm Res20092651162117119191012
- DanhierFVromanBLecouturierNTargeting of tumor endothelium by RGD-grafted PLGA-nanoparticles loaded with paclitaxelJ Control Release2009140216617319699245
- MaoBLiuCZhengWCyclic cRGDfk peptide and chlorin e6 functionalized silk fibroin nanoparticles for targeted drug delivery and photodynamic therapyBiomaterials201816130632029427926
- XinHJiangXGuJAngiopep-conjugated poly(ethylene glycol)-co-poly(ε-caprolactone) nanoparticles as dual-targeting drug delivery system for brain gliomaBiomaterials2011324293430521427009
- Yusuf-MakagiansarHSiahaanTJBinding and internalization of an LFA-1-derived cyclic peptide by ICAM receptors on activated lymphocyte: a potential ligand for drug targeting to ICAM-1-expressing cellsPharm Res200118332933511442273
- ChittasuphoCXieSXBaoumAYakovlevaTSiahaanTJBerklandCJICAM-1 targeting of doxorubicin-loaded PLGA nanoparticles to lung epithelial cellsEur J Pharm Sci200937214115019429421
- ObaidGChambrierICookMJRussellDACancer targeting with biomolecules: a comparative study of photodynamic therapy efficacy using antibody or lectin conjugated phthalocyanine-PEG gold nanoparticlesPhotochem Photobiol Sci201514473774725604735
- BhatRGarcíaIAznarELectin-gated and glycan functionalized mesoporous silica nanocontainers for targeting cancer cells overexpressing Lewis X antigenNanoscale2018101239249
- WuXTanYJTohHTStimuli-responsive multifunctional glyconanoparticle platforms for targeted drug delivery and cancer cell imagingChem Sci2017853980398828553540
- FrickSUDomogallaMPBaierGInterleukin-2 functionalized nanocapsules for T cell-based immunotherapyACS Nano20161092169226
- SinghRLillardJWNanoparticle-based targeted drug deliveryExp Mol Pathol200986321522319186176
- LeeESNaKBaeYHDoxorubicin loaded pH-sensitive polymeric micelles for reversal of resistant MCF-7 tumorJ Control Release2005103240541815763623
- SuzukiRTakizawaTKuwataYEffective anti-tumor activity of oxaliplatin encapsulated in transferrin–PEG-liposomeInt J Pharm20083461–214315017640835
- SahooSKMaWLabhasetwarVEfficacy of transferrin-conjugated paclitaxel-loaded nanoparticles in a murine model of prostate cancerInt J Cancer2004112233534015352049
- BartlettDWSuHHildebrandtIJWeberWADavisMEImpact of tumor-specific targeting on the biodistribution and efficacy of siRNA nanoparticles measured by multimodality in vivo imagingProc Natl Acad Sci U S A200710439155491555417875985
- GradisharWJAlbumin-bound paclitaxel: a next-generation taxaneExpert Opin Pharmacother2006781041105316722814
- PieterszGAWangXYapMLLimBPeterKTherapeutic targeting in nanomedicine: the future lies in recombinant antibodiesNanomedicine (Lond)201712151873188928703636
- ChengZal ZakiAHuiJZMuzykantovVRTsourkasAMultifunctional nanoparticles: cost versus benefit of adding targeting and imaging capabilitiesScience2012338610990391023161990
- DuJLaneLANieSStimuli-responsive nanoparticles for targeting the tumor microenvironmentJ Control Release201521920521426341694
- ZhaoXLiuPReduction-responsive core–shell–corona micelles based on triblock copolymers: novel synthetic strategy, characterization, and application as a tumor microenvironment-responsive drug delivery systemACS Appl Mater Interfaces20157116617425394962
- AdiseshaiahPPCristRMHookSSMcneilSENanomedicine strategies to overcome the pathophysiological barriers of pancreatic cancerNat Rev Clin Oncol2016131275076527531700
- NielsenMFMortensenMBDetlefsenSKey players in pancreatic cancer-stroma interaction: cancer-associated fibroblasts, endothelial and inflammatory cellsWorld J Gastroenterol20162292678270026973408
- Bhaw-LuximonAJhurryDNew avenues for improving pancreatic ductal adenocarcinoma (PDAC) treatment: selective stroma depletion combined with nano drug deliveryCancer Lett2015369226627326415628
- KennedyEJBiological drug products: development and strategiesChemMedChem201491228142815
- KalimuthuKLubinBCBazylevichAGold nanoparticles stabilize peptide-drug-conjugates for sustained targeted drug delivery to cancer cellsJ Nanobiotechnology20181613429602308
- TranSDegiovanniPJPielBRaiPCancer nanomedicine: a review of recent success in drug deliveryClin Transl Med201764429230567
- RahmanAMYusufSWEwerMSAnthracycline-induced cardio-toxicity and the cardiac-sparing effect of liposomal formulationInt J Nanomedicine20072456758318203425
- BatistGCardiac safety of liposomal anthracyclinesCardiovasc Toxicol200772727417652807
- KundrandaMNiuJAlbumin-bound paclitaxel in solid tumors: clinical development and future directionsDrug Des Devel Ther2015937673777
- UntchMJackischCSchneeweissANab-paclitaxel versus solvent-based paclitaxel in neoadjuvant chemotherapy for early breast cancer (GeparSepto – GBG 69): a randomised, phase 3 trialLancet Oncol201617334535626869049
- ChioreanEGvon HoffDWanYMargunato-DebaySBottemanMGoldsteinDPerformance status dynamics during treatment with nab-paclitaxel plus gemcitabine versus gemcitabine alone for metastatic pancreatic cancerCancer Manag Res2018101389139629910636
- HoffmanRMBouvetMNanoparticle albumin-bound-paclitaxel: a limited improvement under the current therapeutic paradigm of pancreatic cancerExpert Opin Pharmacother201516794394725887245
- MadhavanSGusevYHarrisMG-DOC: a systems medicine platform for personalized oncologyNeoplasia201113977178321969811
- LeeKChungHImSMulticenter phase II study of a Cremophor-free polymeric micelle-formulated paclitaxel in patients (pts) with metastatic breast cancer (MBC)J Clin Oncol20062418 Suppl10520
- NemunaitisJCunninghamCSenzerNPhase I study of CT-2103, a polymer-conjugated paclitaxel, and carboplatin in patients with advanced solid tumorsCancer Invest200523867167616377585
- NorthfeltDWDezubeBJThommesJAEfficacy of PEGylated-liposomal doxorubicin in the treatment of AIDS-related Kaposi’s sarcoma after failure of standard chemotherapyJ Clin Oncol19971526536599053490
- MaPChenJBiXOvercoming multidrug resistance through the GLUT1-mediated and enzyme-triggered mitochondrial targeting conjugate with redox-sensitive paclitaxel releaseACS Appl Mater Interfaces20181015123511236329569435
- ZhouLWangHLiYStimuli-responsive nanomedicines for overcoming cancer multidrug resistanceTheranostics2018841059107429463999
- LiuJLiJLiuNIn vitro studies of phospholipid-modified PAMAM-siMDR1 complexes for the reversal of multidrug resistance in human breast cancer cellsInt J Pharm20175301–229129928619457
- LiJLiuJGuoNZhangXReversal of multidrug resistance in breast cancer MCF-7/ADR cells by h-R3-siMDR1-PAMAM complexesInt J Pharm2016511143644527444552
- GuoNGaoCLiuJReversal of ovarian cancer multidrug resistance by a combination of LAH4-L1-siMDR1 nanocomplexes with chemotherapeuticsMol Pharm20181551853186129621396
- MisraRDasMSahooBSSahooSKReversal of multidrug resistance in vitro by co-delivery of MDR1 targeting siRNA and doxorubicin using a novel cationic poly(lactide-co-glycolide) nanoformulationInt J Pharm20144751–237238425178825
- MasudaYKobayashiHHollandJFOhnumaTReversal of mul-tidrug resistance by a liposome-MDR1 ribozyme complexCancer Chemother Pharmacol19984219169619752
- GuJFangXHaoJShaXReversal of P-glycoprotein-mediated multidrug resistance by CD44 antibody-targeted nanocomplexes for short hairpin RNA-encoding plasmid DNA deliveryBiomaterials2015459911425662500
- LiJLiangHLiuJWangZPoly(amidoamine) (PAMAM) dendrimer mediated delivery of drug and pDNA/siRNA for cancer therapyInt J Pharm20185461–221522529787895
- KesharwaniPBanerjeeSGuptaUPAMAM dendrimers as promising nanocarriers for RNAi therapeuticsMater Today20151810565572
- NahaPMukherjeeSByrneHToxicology of engineered nanoparticles: focus on poly(amidoamine) dendrimersInt J Environ Res Public Health2018152E33829443901
- de JongWHBormPJDrug delivery and nanoparticles: applications and hazardsInt J Nanomedicine20083213314918686775
- KimYMSongSCTargetable micelleplex hydrogel for long-term, effective, and systemic siRNA deliveryBiomaterials201435277970797724951047
- KimHJKimAMiyataKKataokaKRecent progress in development of siRNA delivery vehicles for cancer therapyAdv Drug Deliv Rev2016104617727352638
- SarettSMNelsonCEDuvallCLTechnologies for controlled, local delivery of siRNAJ Control Release20152189411326476177
- MieleESpinelliGPMieleETomaoFTomaoSAlbumin-bound formulation of paclitaxel (Abraxane ABI-007) in the treatment of breast cancerInt J Nanomedicine200949910519516888
- ParkKControlled drug delivery systems: past forward and future backJ Control Release20141903824794901
- LiuDYangFXiongFGuNThe smart drug delivery system and its clinical potentialTheranostics2016691306132327375781
- VentolaCLProgress in nanomedicine: approved and investigational nanodrugsPharm Ther20174212742755
- MontanaMDucrosCVerhaeghePTermeTVanellePRathelotPAlbumin-bound paclitaxel: the benefit of this new formulation in the treatment of various cancersJ Chemother2011232596621571619
- YanoSZhangYMiwaSSpatial–temporal FUCCI imaging of each cell in a tumor demonstrates locational dependence of cell cycle dynamics and chemoresponsivenessCell Cycle201413132110211924811200
- DouerDEfficacy and Safety of Vincristine Sulfate Liposome Injection in the Treatment of Adult Acute Lymphocytic LeukemiaOncologist201621784084727328933
- SilvermanJADeitcherSRMarqibo (vincristine sulfate liposome injection) improves the pharmacokinetics and pharmacodynamics of vincristineCancer Chemother Pharmacol201371355556423212117
- DawidczykCMRussellLMSearsonPCNanomedicines for cancer therapy: state-of-the-art and limitations to pre-clinical studies that hinder future developmentsFront Chem201426925202689
- DavisTFaragSSTreating relapsed or refractory Philadelphia chromosome-negative acute lymphoblastic leukemia: liposome-encapsulated vincristineInt J Nanomedicine201383479348824072970
- DrummondDCNobleCOGuoZHongKParkJWKirpotinDBDevelopment of a highly active nanoliposomal irinotecan using a novel intraliposomal stabilization strategyCancer Res20066663271327716540680
- LambYNScottLJLiposomal Irinotecan: a review in metastatic pancreatic adenocarcinomaDrugs201777778579228401446
- ShahNMohammadASSaralkarPInvestigational chemotherapy and novel pharmacokinetic mechanisms for the treatment of breast cancer brain metastasesPharmacol Res2018132476829604436
- RafiyathSMRasulMLeeBWeiGLambaGLiuDComparison of safety and toxicity of liposomal doxorubicin vs. conventional anthracyclines: a meta-analysisExp Hematol Oncol2012111023210520
- ForssenEACoulterDMProffittRTSelective in vivo localization of daunorubicin small unilamellar vesicles in solid tumorsCancer Res19925212325532611596882
- BulbakeUDoppalapudiSKommineniNKhanWLiposomal formulations in clinical use: an updated reviewPharmaceutics201794E1228346375
- MufamadiMSPillayVChoonaraYEA review on composite liposomal technologies for specialized drug deliveryJ Drug Deliv2011201193985121490759
- LancetJEGlUCortesJEFinal results of a phase III randomized trial of CPX-351 versus 7+3 in older patients with newly diagnosed high risk (secondary) AMLJ Clin Oncol20163415 Suppl7000
- ChenECFathiATBrunnerAMReformulating acute myeloid leukemia: liposomal cytarabine and daunorubicin (CPX-351) as an emerging therapy for secondary AMLOnco Targets Ther2018113425343429928134
- CollierMABachelderEMAinslieKMElectrosprayed myocet-like liposomes: an alternative to traditional liposome productionPharm Res201734241942627896588
- AgrahariVAgrahariVFacilitating the translation of nanomedicines to a clinical product: challenges and opportunitiesDrug Discov Today201823597499129406263
- WexJSidhuMOdeyemiIAbou-SettaAMRetsaPTombalBLeuprolide acetate 1-, 3- and 6-monthly depot formulations in androgen deprivation therapy for prostate cancer in nine European countries: evidence review and economic evaluationClinicoecon Outcomes Res2013525726923836996
- TombalBBergesREligard: advantages for optimal testosterone controlEur Urol Suppl2006518900904
- JainRAThe manufacturing techniques of various drug loaded biodegradable poly(lactide-co-glycolide) (PLGA) devicesBiomaterials200021232475249011055295
- DanhierFAnsorenaESilvaJMCocoRle BretonAPréatVPLGA-based nanoparticles: an overview of biomedical applicationsJ Control Release2012161250552222353619
- PillaiGCeballos-CoronelMLScience and technology of the emerging nanomedicines in cancer therapy: a primer for physicians and pharmacistsSage Open Med201313205031211351375926770691
- ZhangFLiuMRWanHTDiscussion about several potential drawbacks of PEGylated therapeutic proteinsBiol Pharm Bull201437333533924334536
- ShehzadALeeJHuhTLLeeYSCurcumin induces apoptosis in human colorectal carcinoma (HCT-15) cells by regulating expression of Prp4 and p53Mol Cells201335652653223686430
- KunwarABarikAMishraBRathinasamyKPandeyRPriyadarsiniKIQuantitative cellular uptake, localization and cytotoxicity of curcumin in normal and tumor cellsBiochim Biophys Acta20081780467367918178166
- CheungABaxHJJosephsDHTargeting folate receptor alpha for cancer treatmentOncotarget2016732525535257427248175
- LyonPCGriffithsLFLeeJClinical trial protocol for TAR-DOX: a phase I study to investigate the feasibility of targeted release of lyso-thermosensitive liposomal doxorubicin (ThermoDox) using focused ultrasound in patients with liver tumoursJ Ther Ultrasound201752829118984
- PanyamJZhouWZPrabhaSSahooSKLabhasetwarVRapid endo-lysosomal escape of poly(dl-lactide-co-glycolide) nanoparticles: implications for drug and gene deliveryFASEB J200216101217122612153989
- RamasamyTTranTHChoiJYLayer-by-layer coated lipid–polymer hybrid nanoparticles designed for use in anticancer drug deliveryCarbohydr Polym201410265366124507332
- WestesenKBunjesHKochMHPhysicochemical characterization of lipid nanoparticles and evaluation of their drug loading capacity and sustained release potentialJ Control Release1997482–3223236
- MolineuxGPEGylation: engineering improved biopharmaceuticals for oncologyPharmacotherapy2003238 Pt 23S8S12921216
- WangMXieFWenXTherapeutic PEG-ceramide nanomicelles synergize with salinomycin to target both liver cancer cells and cancer stem cellsNanomedicine (Lond)20171291025104228440698
- YaoXLYoshiokaYRuanGXOptimization and internalization mechanisms of PEGylated adenovirus vector with targeting peptide for cancer gene therapyBiomacromolecules20121382402240922746837
- BalalaevaIVZdobnovaTAKrutovaIVBrilkinaAALebedenkoENDeyevSMPassive and active targeting of quantum dots for whole-body fluorescence imaging of breast cancer xenograftsJ Biophotonics2012511–1286086722887708
- SykesEAChenJZhengGChanWCInvestigating the impact of nanoparticle size on active and passive tumor targeting efficiencyACS Nano2014865696570624821383
- CortésJRugoHSTwelvesCSafety and tolerability of etirinotecan pegol in advanced breast cancer: analysis of the randomized, phase 3 BEACON trialSpringerplus20165103327441152
- ChoKWangXNieSChenZShinDMTherapeutic nanoparticles for drug delivery in cancerClin Cancer Res20081451310131618316549
- LiechtyWBPeppasNAExpert opinion: Responsive polymer nanoparticles in cancer therapyEur J Pharm Biopharm201280224124621888972
- WangBJiangWYanHNovel PEG-graft-PLA nanoparticles with the potential for encapsulation and controlled release of hydrophobic and hydrophilic medications in aqueous mediumInt J Nanomedicine201161443145121796246
- JacksonJKLetchfordKWassermanBZYeLHamadWYBurtHMThe use of nanocrystalline cellulose for the binding and controlled release of drugsInt J Nanomedicine2011632133021383857
- AmoozgarZWangLBrandstoetterTWallisSSWilsonEMGoldbergMSDual-layer surface coating of PLGA-based nanoparticles provides slow-release drug delivery to achieve metronomic therapy in a paclitaxel-resistant murine ovarian cancer modelBiomacromolecules201415114187419425251833
- ProsperiDColomboMZanoniIGranucciFDrug nanocarriers to treat autoimmunity and chronic inflammatory diseasesSemin Immunol201734616728855088
- OwenARannardSConsiderations for clinically-relevant nano-medicine therapies for chronic diseasesNanomedicine (Lond)201510203103310726446297
- HoffmanAPharmacodynamic aspects of sustained release preparationsAdv Drug Deliv Rev199833318519910837659
- KumariAYadavSKYadavSCBiodegradable polymeric nanoparticles based drug delivery systemsColloids Surf B Biointerfaces201075111819782542
- SklarJJOHVMLiSCFactors affecting therapeutic compliance: a review from the patient’s perspectiveTher Clin Risk Manag20084126928618728716
- ClaxtonAJCramerJPierceCA systematic review of the associations between dose regimens and medication complianceClin Ther20012381296131011558866
- VrijensBde GeestSHughesDAA new taxonomy for describing and defining adherence to medicationsBr J Clin Pharmacol201273569170522486599
- EisenSAMillerDKWoodwardRSSpitznagelEPrzybeckTRThe effect of prescribed daily dose frequency on patient medication complianceArch Intern Med19901509188118842102668
- NoensLvan LierdeMAde BockRPrevalence, determinants, and outcomes of nonadherence to imatinib therapy in patients with chronic myeloid leukemia: the ADAGIO studyBlood2009113225401541119349618
- WangYSYoungsterSGraceMBauschJBordensRWyssDFStructural and biological characterization of PEGylated recombinant interferon alpha-2b and its therapeutic implicationsAdv Drug Deliv Rev200254454757012052714
- BukowskiRErnstoffMSGoreMEPEGylated interferon alfa-2b treatment for patients with solid tumors: a phase I/II studyJ Clin Oncol200220183841394912228203
- AtkinsMBHodiFSThompsonJAPembrolizumab (Pembro) plus ipilimumab (Ipi) or PEGylated interferon alfa-2b (PEG-IFN) for advanced melanoma or renal cell carcinoma (RCC)J Clin Oncol20163415 Suppl3013
- NunesATGreenDEkwedeIZoonKAnnunziataCMPhase 1 study of intraperitoneal infusion of autologous monocytes with PEGinter-feron alfa-2b and interferon gamma-1b in women with recurrent or refractory ovarian cancer, fallopian tube cancer, or primary peritoneal cancerJ Clin Oncol20163515 Suppl3092
- KatsukiSMatobaTKogaJNakanoKEgashiraKAnti-inflammatory nanomedicine for cardiovascular diseaseFront Cardiovasc Med201748729312961
- BatistGBartonJChaikinPSwensonCWellesLMyocet (liposome-encapsulated doxorubicin citrate): a new approach in breast cancer therapyExpert Opin Pharmacother20023121739175112472371
- DixitNMauryaSDSagarBPSustained release drug delivery systemIndian J Res Pharm Biotechnol201313305310
- TarunPVishalSGauravSSatyanandTChiragPAnilGNovel oral sustained release technology: a concise review sustained release drug disadvantagesInt J Res Dev Pharm Life Sci201322262269
- ParkKControlled drug delivery systems: past forward and future backJ Control Release20141903824794901
- MüllertzAPerrieYRadesTAnalytical Techniques in the Pharmaceutical SciencesHeidelbergSpringer2016
- XuMLiewCVHengPWEvaluation of the coat quality of sustained release pellets by individual pellet dissolution methodologyInt J Pharm2015478131832725435182
- DavidsonRSKehoeGinventorsCure Pharmaceutical, assigneeMethods for modulating dissolution, bioavailability, bioequivalence and drug delivery profile of thin film drug delivery systems, controlled-release thin film dosage formats, and methods for their manufacture and useUS patent8999372B2201547
- MccarthyCAAhernRJDontireddyRRyanKBCreanAMMes-oporous silica formulation strategies for drug dissolution enhancement: a reviewExpert Opin Drug Deliv20161319310826549623
- KidaneABhattPPinventorsSupernus Pharmaceuticals, assigneeOsmotic drug delivery systemUS patent15162829201624
- ChenJPanHYeTRecent aspects of osmotic pump systems: functionalization, clinical use and advanced imaging technologyCurr Drug Metab201617327929126467064
- ZamanMQureshiJEjazHOral controlled release drug delivery system and characterization of oral tablets: a reviewPak J Pharm Sci2016216776
- AuilerJFLiuKLynchJMGelotteCKEffect of Food on early drug exposure from extended-release stimulants: results from the Concerta, Adderall XR Food Evaluation (CAFE) studyCurr Med Res Opin200218531131612240794
- ChaudhryNCSaundersLSustained release of drugs from ion exchange resinsJ Pharm Pharmacol195681197598613368094
- SinghBNKimKHFloating drug delivery systems: an approach to oral controlled drug delivery via gastric retentionJ Control Release200063323525910601721
- KumarVPrajapatiSSoniGCSinghMKumarNSustained release matrix type drug delivery system: a reviewWorld J Pharm Pharm Sci201213934960
- PundirSBadolaASharmaDSustained release matrix technology and recent advance in matrix drug delivery system: a reviewInt J Drug Res Technol2017311220
- NairLSLaurencinCTBiodegradable polymers as biomaterialsProg Polym Sci2007328–9762798
- HaxhoFAllisonSAlghamdiFOseltamivir phosphate mono-therapy ablates tumor neovascularization, growth, and metastasis in mouse model of human triple-negative breast adenocarcinomaBreast Cancer (Dove Med Press)2014619120325525387
- HaxhoFANeufeldRJSzewczukMRNovel insulin receptor-signaling platformInt J Diabetes Clin Res20141110
- GilmourAMAbdulkhalekSChengTSA novel epidermal growth factor receptor-signaling platform and its targeted translation in pancreatic cancerCell Signal201325122587260323993964
- AlghamdiFGuoMAbdulkhalekSCrawfordNAmithSRSzewczukMRA novel insulin receptor-signaling platform and its link to insulin resistance and type 2 diabetesCell Signal20142661355136824583283
- HaxhoFHaqSSzewczukMRBiased G protein-coupled receptor agonism mediates Neu1 sialidase and matrix metalloproteinase-9 crosstalk to induce transactivation of insulin receptor signalingCell Signal201843718429277445
- AbdulkhalekSAmithSRFranchukSLNeu1 sialidase and matrix metalloproteinase-9 cross-talk is essential for Toll-like receptor activation and cellular signalingJ Biol Chem201128642365323654921873432
- HaxhoFNeufeldRJSzewczukMRNeuraminidase-1: a novel therapeutic target in multistage tumorigenesisOncotarget2016726408604088127029067
- HrynykMEllisJPHaxhoFTherapeutic designed poly (lactic-co-glycolic acid) cylindrical oseltamivir phosphate-loaded implants impede tumor neovascularization, growth and metastasis in mouse model of human pancreatic carcinomaDrug Des Devel Ther2015945734586
- O’SheaLKAbdulkhalekSAllisonSNeufeldRJSzewczukMRTherapeutic targeting of Neu1 sialidase with oseltamivir phosphate (Tamiflu) disables cancer cell survival in human pancreatic cancer with acquired chemoresistanceOnco Targets Ther2014711713424470763
- KapishonVAllisonSWhitneyRACunninghamMFSzewczukMRNeufeldRJOseltamivir-conjugated polymeric micelles prepared by RAFT living radical polymerization as a new active tumor targeting drug delivery platformBiomater Sci20164351152126788555
- WoodKSzewczukMRRousseauDNeufeldRJOseltamivir phosphate released from injectable Pickering emulsions over an extended term disables human pancreatic cancer cell survivalOncotarget2018916127541276829560107
- WakaskarRRPromising effects of nanomedicine in cancer drug deliveryJ Drug Target201826431932428875739
- ChoiKYYoonHYKimJHSmart nanocarrier based on PEGylated hyaluronic acid for cancer therapyACS Nano20115118591859921967065
- SarkarKYangHEncapsulation and extended release of anticancer anastrozole by stealth nanoparticlesDrug Deliv200815534334618763165
- TietjenGTSaltzmanWMNanomedicine gets personalSci Transl Med20157314314fs47
- MaitlandMLSchilskyRLClinical trials in the era of personalized oncologyCA Cancer J Clin201161636538122034206
- NicoliniCBragazziNPechkovaENanoproteomics enabling personalized nanomedicineAdv Drug Deliv Rev201264131522153122820526
- KyddJJadiaRVelpurisivaPGadAPaliwalSRaiPTargeting strategies for the combination treatment of cancer using drug delivery systemsPharmaceutics201794E4629036899
- LammersTKiesslingFHenninkWEStormGDrug targeting to tumors: principles, pitfalls and (pre-)clinical progressJ Control Release2012161217518721945285
- LiYWangYHuangGGaoJCooperativity principles in self-assembled nanomedicineChem Rev2018118115359539129693377
- LuoMWangHWangZA STING-activating nanovaccine for cancer immunotherapyNat Nanotechnol201712764865428436963