Abstract
Silver nanoparticles have been used in various fields, and several synthesis processes have been developed. The stability and dispersion of the synthesized nanoparticles is vital. The present article describes a novel approach for one-step synthesis of silver nanoparticles–embedded chitosan particles. The proposed approach was applied to simultaneously obtain and stabilize silver nanoparticles in a chitosan polymer matrix in-situ. The diameter of the synthesized chitosan composite particles ranged from 1.7 mm to 2.5 mm, and the embedded silver nanoparticles were measured to be 15±3.3 nm. Further, the analyses of ultraviolet-visible spectroscopy, energy dispersive spectroscopy, and X-ray diffraction were employed to characterize the prepared composites. The results show that the silver nanoparticles were distributed over the surface and interior of the chitosan spheres. The fabricated spheres had macroporous property, and could be used for many applications such as fungicidal agents in the future.
Keywords:
Introduction
Silver nanoparticles exhibit many unique electronic, catalytic, optical, and other physical and chemical properties that their bulk counterparts do not have.Citation1 Silver nanoparticles have been applied in many fields including photonics, micro-electronics, photocatalysis, lithography, and surface-enhanced Raman spectroscopy.Citation2,Citation3 Several types of chemical and physical technologies, such as chemical reduction,Citation4–Citation6 electrochemical reduction,Citation7–Citation9 photochemical reduction,Citation10–Citation12 and heat evaporationCitation13,Citation14 have been developed for the synthesis of silver nanoparticles. In recent years, bio- or green-synthesis of silver nanoparticles has become an emerging science. For example, using plant extracts for reducing silver nitrite to obtain nanoparticles with antimicrobial activities has emerged as a cost-effective and eco-friendly approach.Citation15,Citation16
The fabrication of silver nanoparticles has been widely studied, and their stability and dispersion are crucial. Stabilizers such as surfactants, micelles, ligands, and polymers have been widely utilized in the literature.Citation17–Citation20 Recently, incorporating metal nanoparticles into polymers has been proposed. Immobilizing metal nanoparticles on matrix polymers called nanometal polymer hybrids efficiently prevents nanoparticles’ aggregation.Citation21 In addition, the biocompatibility can thus be improved.Citation22 Polymers can act as matrix materials to help metal nanoparticles’ growth control and stabilization.Citation23 Various polymers have been employed for the synthesis of silver nanoparticles–polymer composites.Citation24 In addition, polymers [eg, chitosan, gelatin,Citation25–Citation27 liposome,Citation28 poly(lactic acid),Citation29 polymethylmethacrylate,Citation30 poly(vinyl alcohol),Citation31 polyvinylpyrrolidone,Citation32,Citation33 etc] can be used as reducing or stabilizing agents for the synthesis of silver nanoparticles, without other reducing agents.Citation34,Citation35 For example, Bankura et al proposed a simple one-step synthesis of silver nanoparticles exhibiting multiple antimicrobial activities with size range of 10–60 nm by reduction of silver nitrate (AgNO3) using aqueous dextran solution as both reducing and capping agent.Citation36 Recently, several strategies for silver nanoparticles polymer hybrids were proposed. For example, El-Rafie et al developed a green synthesis process for silver nanoparticles’ generation by using polysaccharides extracted from marine macroalgae.Citation37 Wang et al fabricated uniform polystyrene spheres coated with silver nanoparticles by simultaneous photo-induced polymerization and reduction.Citation38 Abdel-Mohsen et al synthesized core-shell structures of silver/chitosan-O-methoxy polyethylene glycol nanoparticles.Citation39
Spherical solid supports for silver nanoparticles such as microspheres are crucial for several fungicidal applications. For example, silver nanoparticles-embedded methacrylic acid copolymer microspheres were found to be effective bactericidal agents for water sterilization, because they were quite stable and were not washed away by water.Citation40 In addition, some hybrid materials with embedded silver nanoparticles were used against Candida spp., Escherichia coli, Pseudomonas sp., Aspergillus niger or Penicillium sp., and others.Citation40–Citation44 In order to apply the silver nanoparticles composite materials to industry, developing a facile and low cost method for the synthesis of silver nanoparticles polymer composite particles becomes vital.
In this study, we would like to present a facile approach to produce uniform silver nanoparticles–chitosan composite spheres, and test the fungicidal properties of the prepared hybrid spheres. The fabricated spheres would be characterized by UV-Vis spectroscopy, Fourier transform infrared spectroscopy (FTIR), scanning electron microscopy (SEM), transmission electron microscopy (TEM), and energy dispersive spectroscopy (EDS).
Materials and methods
Materials
Chitosan (molecular weight: 150,000, 1.5% w/v), AgNO3 glucose, and sodium hydroxide (NaOH) were purchased from Sigma-Aldrich Co. (St Louis, MO, USA), and used as received without further purification.
Synthesis of the chitosan spheres
Chitosan (0.2 g, dissolved in 10 mL of 1% v/v acetic acid [CH3COOH] solution) was prepared and dropped into a 20% 25 mL NaOH solution using syringes (3 mL, Terumo®) with 24 gauge needle (24G ×1″, Terumo®) and a pump. Chitosan spheres were obtained after 15 minutes. Spheres were collected and washed twice with 30 mL of dd-H2O (double distilled water) to remove residual alkali.
Synthesis of the Ag nanoparticles-chitosan composite spheres
Based on our previous studies,Citation41–Citation44 the one-step production of silver nanoparticles–chitosan composite spheres is shown in . Chitosan (0.2 g, dissolved in 10 mL of 1% v/v CH3COOH solution) and 2% 10 mL AgNO3 was mixed by constant stirring for 30 minutes, and then an AgNO3–chitosan mixture solution was obtained. The AgNO3–chitosan mixture solution was then dropped into a 20%, 30%, or 40%, 25 mL NaOH solution, respectively by using a syringe pump. After 15 minutes, yellow-brown color spheres (eg, silver nanoparticles–chitosan composite spheres) were yielded. Spheres were collected and washed twice with 30 mL of dd-H2O to remove residual alkali.
Figure 1 Schematic drawing of the silver nanoparticles–chitosan composite spheres synthesis.
Notes: Droplets were pushed out from pin tip of a pump-driven syringe and then dropped into an NaOH solution, which was used for silver nanoparticles’ reduction and chitosan solidification. Silver nanoparticles–chitosan composite spheres were formed in 15 minutes. The distance between solidifying liquid surface and the tip was one centimeter. The diameter of the needle of the syringe was 8.73 mm.
Abbreviations: AgNO3, silver nitrate; NaOH, sodium hydroxide.
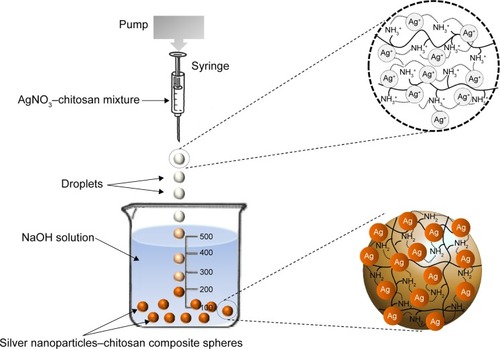
Characterization
The average diameter of the spheres, expressed as mean ± standard deviation, was obtained from the photographs taken by random sampling of approximately 50 individual particles to minimize selection bias. X-ray diffraction (XRD, D8 Advance, PANalytical X’PERT PRO) patterns were obtained at room temperature using Cu K-α radiation (λ=1.5406 Å) with a range of 2θ=20° to 80°, and a scanning rate of 0.05 s−1. The FTIR spectra were recorded with a Spectrum RXI FTIR Spectrometer, using KBr pellets, in the range of 400 to 4,000 cm−1, with a resolution of 4 cm−1. The micromorphology of the silver nanoparticles–chitosan composite spheres was analyzed using a SEM (S-2700, Hitachi Ltd., Tolyo, Japan) equipped with an energy dispersive spectrometer. The characterization of the silver nanoparticles was carried out using TEM (FEI Tecnai G2 20 S-Twin) and a UV-Vis absorbance spectrophotometer (Thermo Scientific Spectrascan UV 2700). The silver nanoparticles solution was prepared by adding 20 μL CH3COOH solution and 1 mL dd-H2O to ten silver nanoparticles–chitosan composite spheres, and then vortexing for 3–5 minutes. The silver nanoparticles–chitosan solution was dropped to grid by a micropipette for TEM analysis report.
Anti-fungal effect test of Cordyceps militaris or Antrodia cinnamomea
To test anti-fungal effect, we used C. militaris (or Cm, Bioresource Collection and Research Center (BCRC) 32219) or A. cinnamomea (or Ac, BCRC 35716) in this study. We spread Cm or Ac on BCRC agar plate (malt Extract 2%, BD 218630; peptone 0.1%, BD 211677; glucose 2%, J.T.Baker 1916-05; agarose 1.5%) until these plates were fully covered by them. The silver nanoparticles–chitosan solution was prepared by adding 20 μL CH3COOH solution and 1 mL dd-H2O to ten silver nanoparticles–chitosan composite spheres, and then vortex for 3–5 minutes. The chitosan solution was prepared by adding 20 μL CH3COOH solution and 1 mL dd-H2O to ten chitosan spheres, and then vortexing for 3–5 minutes. Then we put a round filter paper (diameter 2 cm) with dissolved chitosan sphere or silver nanocomposite chitosan sphere in the middle of the plate and calculated the diameters of the inhibition zone on Day 0, Day 9, and Day 18, respectively. The analysis graph was drawn by GraphPad Prism and data were analyzed using the two-way analysis of variance (ANOVA). A P-value less than 0.05 was considered statistically significant.
Results and discussion
Morphology of silver nanoparticles–chitosan composite spheres
shows that the silver nanoparticles–chitosan composite spheres are obtained by using various concentrations of AgNO3. The pure chitosan spheres showed milky-white (), whereas the fabricated spheres showed more gray when the concentration of AgNO3 () was lower, and more khaki when the concentration of AgNO3 was higher (). The color variation provided some clues to the formation of silver nanoparticles.Citation45 Highly coherent appearance of the prepared silver nanoparticles–chitosan composite spheres was obtained when various concentrations of AgNO3 were used. However, the diameter of the silver nanoparticles seemed to decrease with an increasing concentration of AgNO3 (). Most of the relative standard deviations of the sphere diameters observed at various concentrations of AgNO3 were less than 10%, indicating that the manufactured spheres met the typical criterion for monodispersity.Citation46 Results shown in illustrate the composite spheres fabricated with low concentrations of AgNO3 were slightly flattened in contact with the plate bottom, and thus they became slightly enlarged when measured by a top view. This phenomenon occurred because of fewer silver nanoparticles decreasing the mechanical strength of the spheres. In contrast, when the composite spheres were fabricated with high concentrations of AgNO3 (there will be more silver nanoparticles formed), the composite spheres presented a superior mechanical strength, and thus exhibited properties such as easy spheroidization and aversion to deformation and fragmentation. The literature related to the fabrication of silver nanoparticles–chitosan composite films has mentioned that silver nanoparticles were uniformly dispersed and captured by the networks of amine (-NH2) and hydroxyl (-OH) functional groups,Citation47 and thus the mechanical strength could be enhanced.Citation48,Citation49 Those results were consistent with our finding. We presume that the silver nanoparticles in silver nanoparticles–polymer composite play an identical role of cramp bar in architecture to reinforced concrete. Concrete (imagine as polymer) has relatively low tensile strength, while the presence of reinforcing bars (imagine as silver nanoparticles) shows higher tensile strength and/or ductility.
Table 1 The relationship between average diameter of fabricated silver–chitosan composite spheres and the concentration of silver nitrate (AgNO3)
Figure 2 Photographs of the synthesized spheres.
Notes: (A) Chitosan spheres. (B) Silver nanoparticles–chitosan composite spheres synthesized with 1 mM AgNO3. (C) Silver nanoparticles–chitosan composite spheres synthesized with 8 mM AgNO3. The concentration of chitosan was 2%. All scale bars are 2 mm.
Abbreviation: AgNO3, silver nitrate.

Characterization of silver nanoparticles–chitosan composite spheres
shows the UV-Vis absorbance spectra of the synthesized silver nanoparticles–chitosan composite spheres at various concentrations of NaOH. Two significant peaks were observed: the peak at 275 nm is a characteristic peak of chitosan, whereas 410 nm is a characteristic peak of nano size silver particles.Citation50 shows the TEM photo of the synthesized silver nanoparticles. The particle size was measured to be 15±3.3 nm, which was consistent with the result of UV-Vis spectroscopy. The absorption peaks of the UV-V is spectra shifted to a longer wavelength when the particle diameters were increased.Citation50 Nevertheless, the results shown in presume that there is nearly no change in the peaks (~410 nm) at the various concentrations of NaOH, indicating that the concentration of NaOH has no significant influence on the diameters of the synthesized silver particles.
Figure 3 The UV-Vis absorbance spectra of the synthesized silver–chitosan composite spheres at various concentrations of AgNO3 solution.
Abbreviations: AgNO3, silver nitrate; NaOH, sodium hydroxide; au, arbitrary unit; UV-Vis, ultraviolet-visible spectroscopy.
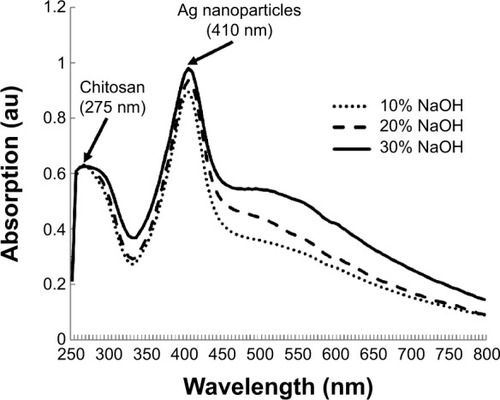
shows the FTIR spectra of the silver nanoparticles–chitosan composite spheres. The bands between 3,462 cm−1 and 3,441 cm−1 were related to the stretching vibrations of amino groups from NH-amine. The bands between 2,925 cm−1 and 2,891 cm−1 corresponded to the alkane C-H-stretching lipids. The bands between 1,632 cm−1 and 1,597 cm−1 corresponded to the amino groups of amide. The bands between 1,385 cm−1 and 1,387 cm−1 were associated with the C=C stretching of aromatic amine groups. The bands between 1,077 cm−1 and 1,075 cm−1 were related to the carbonyl stretch in proteins. The silver nanoparticles were bonded by protein, which served as a stabilizing agent, either through free amine groups or cysteine residues.Citation51,Citation52 These proteins were present as enzymes that could reduce AgNO3 ions to form silver nanoparticles.Citation52
Figure 4 Characterization of silver nanoparticles–chitosan composite spheres by FTIR and XRD.
Notes: (A) The FTIR spectrum, a represents Ag@chitosan, b represents chitosan and (B) the XRD graphs of the fabricated silver nanoparticles–chitosan composite spheres.
Abbreviations: FTIR, Fourier transform infrared spectroscopy; XRD, X-ray diffraction; au, arbitrary unit.
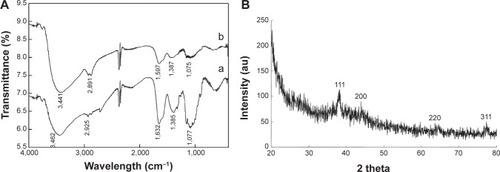
shows the XRD patterns of the silver nanoparticles–chitosan composite spheres. Four characteristic peaks of silver nanoparticles corresponding to (111), (200), (220) and (311) are consistent with those found in the Joint Committee on Powder Diffraction Standards (JCPDS) database (PDF No. 65-3107). The results show that the synthesized nanoparticles were silver nanoparticles, because the position and relative intensity of all the diffraction peaks of the samples were consistent with the crystalline pattern of silver.Citation53 Furthermore, the chitosan being used for the synthesis process did not cause the formation of silver oxides. The shape of each peak was broadened as a result of the presence of the chitosan polymer.Citation54 Line broadening in the pattern was quantitatively evaluated by using the Debye–Scherrer equation d=(kλ/βcos θ), which showed the relationship between peak broadening in the XRD and particle size.Citation55
shows the SEM photographs of the fabricated silver nanoparticles–chitosan composite spheres (using 1 mM AgNO3 and 20% NaOH). shows the SEM graphs of the surface and the “zoom-in” of their intact sphere counterparts. represent the SEM graphs of the morphology, and the “zoom-in” of their sectional sphere counterparts. The results show that the spheres possessed many irregular macro pores, particularly in their interior. shows the morphology of the chitosan spheres, which have relatively smooth structures compared with the silver nanoparticles–chitosan composite spheres. Vimala et al synthesized porous chitosan–silver nanocomposite films that had interdisciplinary applications in wound dressing, antibacterial agents, and water purification.Citation49 In our study, the silver nanoparticles–chitosan composite spheres also possessed porous structures, and could be used in multiple fields.
Figure 5 The SEM photographs of the synthesized silver nanoparticles–chitosan composite spheres.
Notes: Panel (A) is the SEM photograph of a whole sphere. Panels (B) and (C) are the “zoom-in” counterparts of (A). Panel (D) is the SEM photograph of a sectioned sphere. Panels (E) and (F) are the “zoom-in” counterparts of (D). The scale bars are 1 mm (A and D), 100 μm (B and E), and 50 μm (C and F), respectively.
Abbreviation: SEM, scanning electron microscopy.
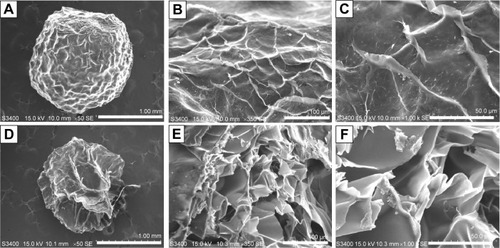
shows the EDS of the silver nanoparticles deposited on the outer surface relative to (), as well as the EDS of the inside of the synthesized silver nanoparticles–chitosan composite spheres relative to (). The intensity of the silver signal of the outer surface was higher than that inside the spheres, indicating that more silver nanoparticles were embedded in the interior of the spheres than deposited on the outer surface. The SEM-EDS mapping images presented in show the silver nanoparticles deposited on the outer surface and interior of the synthesized silver nanoparticles–chitosan composite spheres, respectively. The green dots represent the elemental map of the silver nanoparticles, which were considerably less on the outer surface than inside the spheres. The results of were consistent with those in .
Anti-fungal effect test of silver nanoparticles–chitosan composite spheres
shows the anti-fungal effect. We found that Cm is inhibited by dissolved chitosan and silver nanoparticles–chitosan composite spheres (Ag@chitosan) on Day 9 (the inhibition ring diameter of chitosan is 3.2±0.1 cm and Ag@ chitosan is 3.533±0.217 cm) and Day 18 (the inhibition ring diameter of chitosan is 2.7±0.2 cm and Ag@chitosan is 3.2±0.058 cm) but not Ac. This finding indicates that Ag@ chitosan and chitosan can both inhibit the growth of Cm but the activity of Ag@chitosan is better than chitosan (on Day 9, P<0.05; on Day 18, P<0.01). It is interesting to note that no inhibition ring is formed in Ac group, indicating that Ac is not affected by Ag@chitosan and chitosan. It is worth mentioning that on Day 9 we found not only chitosan but also Ag@chitosan show the best anti-fungal effects. We speculate that the reduction of anti-fungal effect might be caused by the degradation of chitosan and Ag@chitosan. As widely known, both chitosan and Ag@chitosan can inhibit many kinds of microorganisms. In this study, we found that Ag@chitosan or chitosan can inhibit the growth of Cm but not Ac. Taken all together, we thought chitosan and Ag@chistosan can act as a potent reagent to enhance Ac production by inhibiting other microorganism growth.
Figure 6 Ag@chitosan anti-fungal effect.
Notes: (A) Flow chart of silver nanoparticles’ anti-fungal effect. (B) and (C) the anti-fungal effect of chitosan microspheres and Ag@chitosan. *P<0.05, **P<0.01.
Abbreviations: Ag@chitosan, silver nanoparticles–chitosan composite spheres; Cm, C. militaris; Ac, A. cinnamomea; BCRC, Biore source Collection and Research Center.
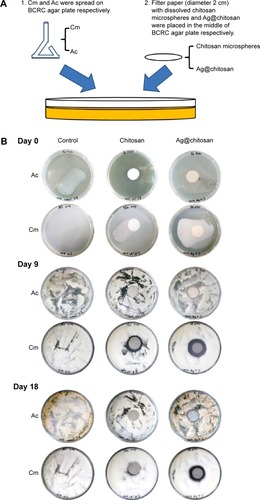
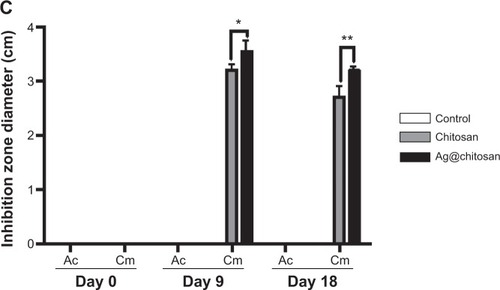
Bacterial infections still remain a major issue within health care industry and medical research. Therefore, it is urgently needed to develop novel antibiotics with high biocompatibility and without limitations of drug-resistance. Among diverse investigated antimicrobial agents, silver is one of the most promising because of its intrinsic properties of high thermal stability, low toxicity to mammalian cells and tissues,Citation56,Citation57 versatile and long-term activity.Citation58 Synthesis of silver nanoparticles or silver-based antimicrobial nanocomposite was well established by miscellaneous methods, for example employed AgNO3 as starting material to obtain silver nanoparticles by chemical reduction with some natural reductant (such as chitosan, dextran, sodium citrate, ascorbate and other reducing biomolecules like polypeptides, flavones, and alkaloids).Citation59–Citation61 A number of literatures have reported that silver nanoparticles incorporated with chitosan-based matrix present a broad spectrum of antimicrobial activity to inhibit both Gram-positive and Gram-negative bacteria including multidrug-resistant pathogens such as P. aeruginosa, ampicillin-resistant E. coli, erythromycin-resistant Streptococcus pyogenes, methicillin-resistant S. aureus (MRSA) and vancomycin-resistant S. aureus (VRSA).Citation62–Citation69 However, the application of silver nanocomposite and chitosan as antifungal effect in Cm and Ac is rare. This study shows a selective inhibition by chitosan and silver nanoparticles–chitosan composite, suggesting that the cellular wall composition or modification of Cm and Ac is distinct. With the unveiling of antifungal ability of chitosan and silver nanoparticles–chitosan composite, it could offer an alternative to conventional antibiotics and a great opportunity to develop next-generation antibiotics.
Conclusion
We proposed a facile approach for manufacturing silver nanoparticles–chitosan composite spheres in a one-step process. The diameters of the fabricated spheres ranged from 1.5 to 2.5 mm, but their particle size could be reduced by using droplet microfluidic technology. The successful formation of silver nanoparticles was evaluated using FTIR, XRD, and EDS mapping. The TEM image shows that the diameter of the fabricated silver nanoparticles was 15±3.3 nm. The UV-Vis spectroscopy shows a characteristic peak at approximately 410 nm, confirming the size of silver particle is within the nanoscale range. The peak wavelength does not substantially change even when various concentrations of NaOH are used. Compared with other approaches, the main advantages of this approach were: i) uniform-sized spheres could be continuously fabricated in a one step process, ii) silver nanoparticles could be obtained and stabilized simultaneously in a chitosan matrix, and iii) spherical shapes could have a wider range of applications compared with film structures. Because of the properties of silver nanoparticles, the synthesized spheres could become potential bactericidal agents for water disinfection, anti-pathogens, or surface plasma resonance enhancers. In addition, in this study we also found that chitosan and Ag@chitosan can inhibit the growth of Cm but not Ac, suggesting that chitosan and Ag@chitosan may be a potential reagent to enhance Ac production by preventing other microorganism growth.
Acknowledgments
This work was financially supported by a grant from the Ministry of Science and Technology, Taiwan, and Dr Lung-Shuo Wang.
Supplementary materials
Figure S1 The transmission electron microscopy photograph of the synthesized silver nanoparticles.
Notes: The silver nanoparticles–chitosan composite sphere was granulated, uniformly dispersed in water. The scale bar is 100 nm.
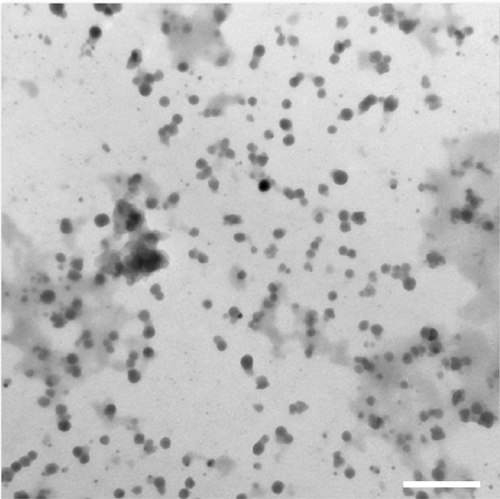
Figure S2 The transmission electron microscopy (SEM) photographs of the synthesized chitosan spheres.
Notes: Panel (A) is an SEM photograph of a whole chitosan sphere. Panels (B) and (C) are the “zoom-in” counterparts of (A). Panel (D) is an SEM photograph of a sectioned chitosan sphere. Panels (E) and (F) are the “zoom-in” counterparts of (D). The scale bars are 1 mm for (A) and (D), 100 μm for (B) and (E), and 50 μm for (C) and (F), respectively.
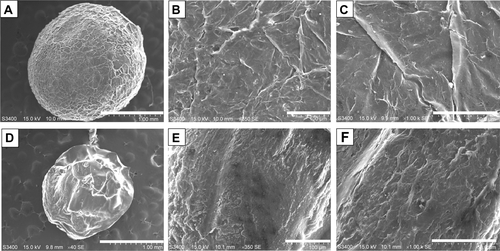
Disclosure
The authors have no conflicts of interest to disclose.
References
- SunYControlled synthesis of colloidal silver nanoparticles in organic solutions: empirical rules for nucleation engineeringChem Soc Rev20134272497251123072940
- BhuiDKBarHSarkarPSahooGPDeSPMisraASynthesis and UV–vis spectroscopic study of silver nanoparticles in aqueous SDS solutionJournal of Molecular Liquids200914513337
- StamplecoskieKGScaianoJCTiwariVSAnisHOptimal Size of Silver Nanoparticles for Surface-Enhanced Raman SpectroscopyJ Phys Chem C2011115514031409
- KhanZAl-ThabaitiSAObaidAYAl-YoubiAOPreparation and characterization of silver nanoparticles by chemical reduction methodColloids Surf B Biointerfaces201182251351721050730
- WangHQiaoXChenJDingSPreparation of silver nanoparticles by chemical reduction methodColloids and Surfaces A: Physicochemical and Engineering Aspects20052562–3111115
- DongXJiXWuHZhaoLLiJYangWShape Control of Silver Nanoparticles by Stepwise Citrate ReductionJ Phys Chem C20091131665736576
- RoldánMVPellegriNde SanctisOElectrochemical Method for Ag-PEG Nanoparticles SynthesisJournal of Nanoparticles2013201317
- TammeveskiLEriksonHSarapuuAElectrocatalytic oxygen reduction on silver nanoparticle/multi-walled carbon nanotube modified glassy carbon electrodes in alkaline solutionElectrochemistry Communications2012201518
- ZhangYChenFZhuangJSynthesis of silver nanoparticles via electrochemical reduction on compact zeolite film modified electrodesChem Commun (Camb)2002232814281512478760
- HuangHHNiXPLoyGLPhotochemical Formation of Silver Nanoparticles in Poly(N-vinylpyrrolidone)Langmuir1996124909912
- ShenZChenGChenZQuXChenYLiuRSpatially selective photochemical reduction of silver on nanoembossed ferroelectric PZT nanowiresLangmuir20112795167517021476531
- ParkH-HZhangXChoiY-JParkH-HHillRHSynthesis of Ag Nanostructures by Photochemical Reduction Using Citrate-Capped Pt SeedsJournal of Nanomaterials201120111721808638
- SmetanaABKlabundeKJSorensenCMSynthesis of spherical silver nanoparticles by digestive ripening, stabilization with various agents, and their 3-D and 2-D superlattice formationJ Colloid Interface Sci2005284252152615780291
- PyatenkoAYamaguchiMSuzukiMSynthesis of Spherical Silver Nanoparticles with Controllable Sizes in Aqueous SolutionsJ Phys Chem C20071112279107917
- KrishnarajCJaganEGRajasekarSSelvakumarPKalaichelvanPTMohanNSynthesis of silver nanoparticles using Acalypha indica leaf extracts and its antibacterial activity against water borne pathogensColloids Surf B Biointerfaces2010761505619896347
- ZargarMHamidAABakarFAGreen Synthesis and Antibacterial Effect of Silver Nanoparticles Using Vitex Negundo LMolecules20111686667667625134770
- AhmedMOLeongWKColloidal silver nanoparticles stabilized by a water-soluble triosmium clusterJournal of Organometallic Chemistry2006691510551060
- BaruahBGabrielGJAkbashevMJBooherMEFacile synthesis of silver nanoparticles stabilized by cationic polynorbornenes and their catalytic activity in 4-nitrophenol reductionLangmuir201329134225423423461821
- PintoVVFerreiraMJSilvaRLong time effect on the stability of silver nanoparticles in aqueous medium: Effect of the synthesis and storage conditionsColloids and Surfaces A: Physicochemical and Engineering Aspects20103641–31925
- ZouXBaoHGuoHMercaptoethane sulfonate protected, water-soluble gold and silver nanoparticles: Syntheses, characterization and their building multilayer films with polyaniline via ion-dipole interactionsJ Colloid Interface Sci2006295240140816413029
- ProzorovaGFPozdnyakovASKuznetsovaNPGreen synthesis of water-soluble nontoxic polymeric nanocomposites containing silver nanoparticlesInt J Nanomedicine201491883188924790430
- CapadonaJRVan Den BergOCapadonaLAA versatile approach for the processing of polymer nanocomposites with self-assembled nanofibre templatesNat Nanotechnol200721276576918654428
- LaudenslagerMJSchiffmanJDSchauerCLCarboxymethyl chitosan as a matrix material for platinum, gold, and silver nanoparticlesBiomacromolecules20089102682258518816099
- AhmadMBShlameliKDarroudiMSynthesis and Characterization of Silver/Clay/Chitosan Bionanocomposites by UV-Irradiation MethodAmerican Journal of Applied Sciences200961220302035
- RegielAIrustaSKyziołAArrueboMSantamariaJPreparation and characterization of chitosan-silver nanocomposite films and their antibacterial activity against Staphylococcus aureusNanotechnology201324101510123221018
- Bin AhmadMLimJJShameliKIbrahimNATayMYSynthesis of silver nanoparticles in chitosan, gelatin and chitosan/gelatin bionanocomposites by a chemical reducing agent and their characterizationMolecules20111697237724821869751
- FoudaMMEl-AassarMRAl-DeyabSSAntimicrobial activity of carboxymethyl chitosan/polyethylene oxide nanofibers embedded silver nanoparticlesCarbohydr Polym20139221012101723399122
- BaraniHMontazerMToliyatTSamadiNSynthesis of Ag-liposome nano compositesJ Liposome Res201020432332920131982
- FortunatiEArmentanoIZhouQMultifunctional bionanocomposite films of poly(lactic acid), cellulose nanocrystals and silver nanoparticlesCarbohydrate Polymers201287215961605
- MuzalevPAKosobudskiiIDKul’batskiiDMUshakovNMPolymer composites based on polymethylmethacrylate with silver nanoparticles, synthesis and optical propertiesInorganic Materials: Applied Research2012314043
- PenchevaDBryaskovaRLadUKaleGMKantardjievTSporocidic properties of poly(vinyl alcohol)/silver nanoparticles/TEOS thin hybrid filmsJ Biomed Nanotechnol20128346547222764416
- XuGQiaoXQiuXChenJPreparation and characterization of stable monodisperse silver nanoparticles via photoreductionColloids and Surfaces A: Physicochemical and Engineering Aspects20083201–3222226
- Slistan-GrijalvaAHerrera-UrbinaRRivas-SilvaJFSynthesis of silver nanoparticles in a polyvinylpyrrolidone (PVP) paste, and their optical properties in a film and in ethylene glycolMaterials Research Bulletin20084319096
- WeiDSunWQianWYeYMaXThe synthesis of chitosan-based silver nanoparticles and their antibacterial activityCarbohydr Res2009344172375238219800053
- TranHVBacCTDinhVHSynthesis, characterization, antibacterial and antiproliferative activities of monodisperse chitosan-based silver nanoparticlesColloids and Surfaces A: Physicochemical and Engineering Aspects20103601–33240
- BankuraKPMaityDMollickMMSynthesis, characterization and antimicrobial activity of dextran stabilized silver nanoparticles in aqueous mediumCarbohydrate Polym201289411591165
- El-RafieHMEl-RafieMHZahranMKGreen synthesis of silver nanoparticles using polysaccharides extracted from marine macro algaeCarbohydr Polym201396240341023768580
- WangCWTsengSJPengSFHwuYKLinCKFunctionalized polymer spheres via one-step photoinduced synthesis for antimicrobial activity and gene deliveryNanotechnology2012232525510322652484
- Abdel-MohsenAMAlyASHrdinaREl-ArefATA novel method for the preparation of silver/chitosan-O-methoxy polyethylene glycol core shell nanoparticlesJournal of Polymers and the Environment2011202459468
- GangadharanDHarshvardanKGnanasekarGPolymeric microspheres containing silver nanoparticles as a bactericidal agent for water disinfectionWater Res201044185481548720673945
- HuangKSWangCYYangCHSynthesis and characterization of oil-chitosan composite spheresMolecules20131855749576023681059
- WangC-YYangC-HHuangK-SElectrostatic droplets assisted in situ synthesis of superparamagnetic chitosan microparticles for magnetic-responsive controlled drug release and copper ion removalJournal of Materials Chemistry B20131162205
- YangCHWangCYHuangKSFacile synthesis of radial-like macroporous superparamagnetic chitosan spheres with in-situ co-precipitation and gelation of ferro-gelsPLoS One2012711e4932923226207
- LinYSHuangKSYangCHMicrofluidic synthesis of microfibers for magnetic-responsive controlled drug release and cell culturePLoS One201273e3318422470443
- ChenZZhangXCaoHHuangYChitosan-capped silver nanoparticles as a highly selective colorimetric probe for visual detection of aromatic ortho-trihydroxy phenolsAnalyst201313882343234923457709
- MejiaAFHePLuoDMarquezMChengZUniform discotic wax particles via electrospray emulsificationJ Colloid Interface Sci20093341222819383560
- KandileNGNasrASEnvironment friendly modified chitosan hydrogels as a matrix for adsorption of metal ions, synthesis and characterizationCarbohydrate Polymers2009784753759
- YallapuMMGuptaBKJaggiMChauhanSCFabrication of curcumin encapsulated PLGA nanoparticles for improved therapeutic effects in metastatic cancer cellsJ Colloid Interface Sci20103511192920627257
- VimalaKMohanYMSivuduKSFabrication of porous chitosan films impregnated with silver nanoparticles: a facile approach for superior antibacterial applicationColloids Surf B Biointerfaces201076124825819945827
- www.sigmaaldrich.com [homepage on the Internet]Oldenburg SJ. Silver Nanoparticles: Properties and ApplicationsSigma-Aldrich Co2013 Available from: http://www.sigmaaldrich.com/materials-science/nanomaterials/silver-nanoparticles.html#opticalAccessed January 15, 2015
- MukherjeePAhmadAMandalDFungus-Mediated Synthesis of Silver Nanoparticles and Their Immobilization in the Mycelial Matrix: A Novel Biological Approach to Nanoparticle SynthesisNano Letters2001110515519
- Mubarak AliDSasikalaMGunasekaranMThajuddinNBiosynthesis and characterization of silver nanoparticles using marine cyanobacterium, oscillatoria willei NTDM01Digest Journal of Nanomaterials and Biostructures201152385390
- StebounovaLVAdamcakova-DoddAKimJSNanosilver induces minimal lung toxicity or inflammation in a subacute murine inhalation modelPart Fibre Toxicol201181521266073
- LuLCWangCISyeWFApplications of chitosan beads and porous crab shell powder for the removal of 17 organochlorine pesticides (OCPs) in water solutionCarbohydrate Polymers201183419841989
- TranHVTranLDNguyenTNPreparation of chitosan/magnetite composite beads and their application for removal of Pb(II) and Ni(II) from aqueous solutionMaterials Science and Engineering: C2010302304310
- ChenXSchluesenerHJNanosilver: A nanoproduct in medical applicationToxicol Lett2008176111218022772
- PoonVKBurdAIn vitro cytotoxity of silver: implication for clinical wound careBurns200430214014715019121
- RaiMYadavAGadeASilver nanoparticles as a new generation of antimicrobialsBiotechnol Adv2009271768318854209
- MoritzMGeszke-MoritzMThe newest achievements in synthesis, immobilization and practical applications of antibacterial nanoparticlesChemical Engineering Journal2013228596613
- AbbasiARAkhbariKMorsaliADense coating of surface mounted CuBTC Metal-Organic Framework nanostructures on silk fibers, prepared by layer-by-layer method under ultrasound irradiation with antibacterial activityUltrason Sonochem201219484685222204978
- MontazerMShameiAAlimohammadiFStabilized nanosilver loaded nylon knitted fabric using BTCA without yellowingProgress in Organic Coatings2012741270276
- PintoRJFernandesSCFreireCSAntibacterial activity of optically transparent nanocomposite films based on chitosan or its derivatives and silver nanoparticlesCarbohydrate Research2012348778322154478
- JenaPMohantySMallickRJacobBSonawaneAToxicity and antibacterial assessment of chitosan-coated silver nanoparticles on human pathogens and macrophage cellsInt J Nanomedicine201271805181822619529
- RaiMKDeshmukhSDIngleAPGadeAKSilver nanoparticles: the powerful nanoweapon against multidrug-resistant bacteriaJ Appl Microbiol2012112584185222324439
- MoronesJRElechiguerraJLCamachoAThe bactericidal effect of silver nanoparticlesNanotechnology200516102346235320818017
- YoksanRChirachanchaiSSilver nanoparticles dispersing in chitosan solution: Preparation by γ-ray irradiation and their antimicrobial activitiesMaterials Chemistry and Physics20091151296302
- Lopez-CarballoGHiguerasLGavaraRHernández-MuñozPSilver ions release from antibacterial chitosan films containing in situ generated silver nanoparticlesJ Agric Food Chem201361126026723214988
- YoussefAMAbdel-AzizMSEl-SayedSMChitosan nanocomposite films based on Ag-NP and Au-NP biosynthesis by Bacillus Subtilis as packaging materialsInt J Biol Macromol20146918519124875320
- MohamedRRSabaaMWSynthesis and characterization of antimicrobial crosslinked carboxymethyl chitosan nanoparticles loaded with silverInt J Biol Macromol201469959924857868