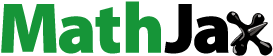
Abstract
Background and objectives
Gemcitabine (Gem) is far from satisfactory as the first-line regimen for pancreatic cancer, and the emergence of albumin nanoparticles offers new hope for the delivery of Gem. In this study, Gem-loaded human serum albumin nanoparticles (Gem-HSA-NPs) were successfully synthesized, characterized, and tested on a BxPC-3 cell line both in vitro and in vivo.
Materials and methods
4-N-myristoyl-gemcitabine (Gem-C14) was obtained first by coupling myristoyl with the 4-amino group of Gem. The Gem-HSA-NPs were then prepared by nanoparticle albumin-bound technology and characterized for particle size, zeta potential, morphology, encapsulation efficiency, drug-loading efficiency, and release characteristics. Using both in vitro and in vivo studies, Gem-C14 and Gem-HSA-NPs were tested on the human pancreatic cancer cell line BxPC-3.
Results
Gem-HSA-NPs showed an average particle size of 150±27 nm, and with an encapsulation rate of 82.99%±3.5% and a drug-loading rate of 10.42%±3.5%, they exhibited a favorable controlled- and sustained-release nature. In in vitro, Gem-C14 was equivalent in cytotoxicity to Gem. In in vivo, the Gem-HSA-NPs exhibited the strongest inhibitory effect on tumor growth but the lowest toxicity among the four groups.
Conclusion
The enhanced in vivo efficacy of Gem-HSA-NPs toward the pancreatic cancer cell line suggests their potential role for use in the clinical field.
Video abstract
Point your SmartPhone at the code above. If you have a QR code reader the video abstract will appear. Or use:
Introduction
Pancreatic cancer is one of the most lethal diseases, making it a major health concern worldwide. According to the statistics, pancreatic cancer is the fourth, fifth, and seventh leading cause of cancer-related deaths in the USA, the European Union, and People’s Republic of China, respectively.Citation1–Citation3 Although great progress has been made for other cancers, pancreatic cancer still has a poor prognosis, with a dismal 5-year survival rate <5%.Citation4 Surgery is the only effective cure, but only 20% of patients are eligible for this approach, and chemotherapy remains the standard treatment for most patients with pancreatic cancer.Citation5
Currently, gemcitabine (Gem) (2′,2′-difluoro-2′-deoxycytidine) is recommended as the first-line regimen for advanced pancreatic cancer.Citation6 However, because of its low molecular weight and high solubility in water, the clinical benefits of Gem are compromised by its short plasma half-life and relative low concentration around tumor sites.Citation7 Furthermore, in order to achieve optimal clinical efficacy, a schedule of frequent high doses is required, which in turn leads to significant side effects.Citation8,Citation9 Therefore, a search for novel therapeutic strategies is imperative. Albumin nanoparticles are increasingly being used as drug carriers for effective accumulation within tumor tissuesCitation10,Citation11 via the following two mechanisms: passive targeting via the enhanced permeability and retention (EPR) effectCitation12 and active targeting via two albumin-binding proteins,Citation13,Citation14 one is gp60 receptor, which is on the tumor endothelium,Citation15,Citation16 and the other is a secreted protein, acidic and rich in cysteine, an albumin “sticker” enriched in the tumor interstitium.Citation17,Citation18 Compared with artificial materials, albumin is nontoxic, biocompatible, biodegradable, and reproduced in the human body.Citation19,Citation20
Nanoparticle albumin-bound (nab)-paclitaxel is another emerging tool for combating late-stage pancreatic cancer. Encouraging results from a number of clinical trials have paved the way for nab-paclitaxel to be approved as the first-line option in advanced pancreatic cancer.Citation21,Citation22 Based on the nab technology, hydrophobic agents can be encapsulated into a hydrophilic exterior core, thereby becoming soluble nanoparticles.Citation23 The nanoparticles are physically stable and have a reproducible, narrow range of diameters (50–150 nm) with a mean value of 130 nm.Citation24 Their negatively charged albumin surfaces repel each other, allowing the particles to remain in homogenous suspension and avoiding flocculation.Citation25 As described earlier, nab-paclitaxel also harnesses the two albumin-binding proteins to increase the targeting efficiency of the drug to the tumor tissues. Therefore, we wondered whether using nab technology we could encapsulate Gem into albumin nanoparticles and thus optimize the delivery of Gem into pancreatic cancer cells.
We have previously reported on the use of albumin nanoparticles as drug carriers to encapsulate Gem in pancreatic cancer,Citation26–Citation28 in which two sizes of Gem-loaded albumin nanoparticles (110 nm and 406 nm) were successfully prepared. Both indicated improved antitumor cytotoxicity and prolonged drug release characteristics. However, using the desolvation–crosslinking method, these albumin nanoparticles showed poor stability, a lack of uniformity, and nonreproducibility, but with the advent of nab technology, these obstacles can be well addressed. To our knowledge, this article is the first report on the synthesis of 4-N-myristoyl-gemcitabine (Gem-C14) and also the first study report on using nab technology to encapsulate Gem-C14 into Gem-loaded human serum albumin nanoparticles (Gem-HSA-NPs). We also conducted in vitro and in vivo studies of Gem-HSA-NP in the pancreatic cancer cell line BxPC-3.
Materials and methods
Chemical and reagents
Gemcitabine (crude drug) was obtained from Eli Lilly and Company (Indianapolis, IN, USA), and the purity was >99.9%. HSA (20%, 50 mL) was purchased from Baxter AG (Vienna, Austria). Normal saline (NS) was purchased from Shanghai Baxter Healthcare Co., Ltd. (Shanghai, People’s Republic of China). Absolute ethanol and chloroform were purchased from Sinopharm Chemical Reagent Co., Ltd. (Shanghai, People’s Republic of China); deionized water was purchased from Fudan University (Shanghai, People’s Republic of China); and Roswell Park Memorial Institute (RPMI)-1640 culture medium and fetal bovine serum were obtained from Thermo Fisher Scientific (Waltham, MA, USA). Double stain Hoechst/PI kit and myristic acid (C14) were purchased from Sigma-Aldrich Co. (St Louis, MO, USA). Cell Counting Kit-8 (CCK-8) was purchased from Dojindo (Kyushu, Japan). Terminal deoxynucleotidyl transferase dUTP nick end labeling (TUNEL) kit was purchased from Hoffman-La Roche Ltd. (Basel, Switzerland). Phosphate-buffered saline (PBS) and other reagents were prepared in our laboratory. All the solvents and chemicals were of analytical grade.
Synthesis of Gem-C14
Myristic acid was dissolved in tetrahydrofuran, and 1,1′-carbonyldiimidazole was then added to the solution. The mixture was stirred at room temperature for 20 minutes to produce solution A. A solution of Gem base in anhydrous CH3CN was mixed with pyridine and trimethylsilyl chloride. The solution was stirred at room temperature for 20 minutes to produce solution B. Solution A was added dropwise to solution B. The resulting mixture was stirred for 12 hours at 60°C. Methanol and trifluoroacetic acid were added dropwise into the solution. The reaction mixture was stirred at room temperature for 10 minutes and then concentrated and dried under vacuum. The solid was dissolved in EtOAc (Ethyl acetate) and washed with brine, saturated NaHCO3 solution, and water. The organic layer was dried over MgSO4 and evaporated. The solid was purified by silica gel column flash chromatography and eluted with a mixture of methanol/chloroform (2:100) to produce the crude product, which was further recrystallized in methanol to generate the final product at a yield of 13%.
Preparation and characterization of Gem-HSA-NPs
Gem-HSA-NPs were prepared by nab technology as previously reported.Citation29 Briefly, 12 mL 20% HSA was mixed with 68 mL pure water. Separately, 100 mg Gem-C14 was dissolved in 1 mL of chloroform saturated with pure water. These two solutions were then fully mixed and homogenized (Nano DeBEE; BEE International, South Easton, MA, USA) at 20,000 psi for nine cycles. The generated colloid was rotary evaporated to remove the chloroform at 25°C for 15 minutes under vacuum. The obtained nanoparticles were then filtered through a 0.25-μm membrane syringe filter, and the solvent was removed by lyophilization for 48 hours at −80°C. The obtained Gem-HSA-NPs powder was vacuum dried for 48 hours and stored at room temperature.
The particle sizes and zeta potential were determined using Zetasizer (Malvern Instruments, Malvern, UK) at a scattering angle of 120°. The nanoparticle suspension was added dropwise onto the copper grids, which were then dried at room temperature. Transmission electron microscopy images of the nanoparticles were acquired (Hitachi H-600; Hitachi Ltd., Tokyo, Japan).
For drug-loading efficiency and encapsulation efficiency, preweighted amount of Gem-HSA-NPs was taken in distilled water and sonicated to extract Gem. The extracted Gem was analyzed by high-performance liquid chromatography–UV assay. Data were collected and calculated using the following formula:
Gemcitabine release was monitored by dispersing 2 mg of Gem-HSA-NPs in 3 mL PBS (pH 7.4). The samples were kept in temperature-controlled horizontal shaker (Ningbo Biotech Scientific Instrument Co., Ltd., Ningbo, People’s Republic of China), and samples were drawn at different time points and analyzed using ultraviolet–visible (UV-Vis) spectrophotometer.
Mice and cell culture
The BALB/c-nu/nu female mice (weighing 20±2 g) were purchased from the Charles River Laboratories (Wilmington, MA, USA) and housed in a pathogen-free animal facility. The temperature was maintained at 24°C with a humidity of 50%–60%, and the mice were subjected to a 10/14-hour light/dark cycle. All animal studies were in compliance with the approved animal protocols and the guidelines of the Institutional Animal Care and Use Committee of Fudan University. The BxPC-3 (human pancreatic cancer cell line, originally from the American Type Culture Collection) was purchased from the Shanghai Branch of the Chinese Academy of Sciences (Shanghai, People’s Republic of China). No ethics statement was required from the institutional review board for the use of these cell lines.
Cells were cultured at 37°C in the presence of 5% CO2 and 95% air with >95% humidity. Growth medium was RPMI-1640, containing 10% fetal bovine serum, 100 U/mL penicillin, and 100 mg/mL streptomycin.
Cell viability
The in vitro cytotoxic effects of Gem, Gem-C14, and Gem-HSA-NPs on BxPC-3 cells were determined using the CCK-8 assay. Briefly, cells were seeded into 96-well culture plates (10,000 cells/well) and incubated overnight at 37°C, 5% CO2. Cells were then incubated with various concentrations of Gem, Gem-C14, Gem-HSA-NPs, or sterile PBS for 48 hours. The cell viability was quantitated by reading the dye absorption at 450 nm with an automatic multiwall spectrophotometer. The cell inhibition rate (%) was calculated as follows: (1− [absorbance of the study group/absorbance of the control group]) ×100.
Apoptotic cell damages assays
The apoptosis rate was assessed using the annexin V-fluorescein isothiocyanate (FITC)/PI according to the instructions of manufacturer. The BxPC-3 cells were grouped and treated as described for the CCK-8 assay. Briefly, BxPC-3 cells were placed in a six-well culture plate (5×105 cells/well). The cells in each group were collected through centrifugation at 1,000 rpm for 5 minutes, followed by washing with PBS for three times and resuspension in binding buffer. Then, 5 μL of annexin V-FITC solution and 10 μL of PI solution were added in turn. The stained cells were incubated for 15 minutes at room temperature in the dark. Finally, the suspension was subjected to the flow cytometry analysis (BD Biosciences, San Jose, CA, USA). All the experiments were performed in triplicate.
In vivo antitumor study
An animal model was obtained by subcutaneous injection of 1×107 BxPC-3 cells suspended in 100 μL RPMI-1640 medium into the right flank of nude mice.Citation30 Studies were done 2 weeks after implantation, when the tumors had reached 5.0 mm in diameter. The mice were then randomized into four groups (n=6 per group) and treated with 100 μL of Gem, Gem-C14, Gem-HSA-NPs, and NS through tail vein injection on days 0, 3, 6, 9, and 12 (Gem or equivalent dosage: 50 mg/kg body weight). Mice body weights and tumor sizes were monitored every 2 days until day 14. Tumor volume was estimated using the formula: volume (mm3) = (length in mm) × (width in mm)2/2.
TUNEL assays
On day 14, the mice were sacrificed by cervical dislocation, and the tumor mass was harvested, photographed, and then fixed with paraformaldehyde for 48 hours and embedded in paraffin. Each section was cut into 5 μm slice and used for TUNEL staining according to the manufacturer’s instructions. Nucleus staining was performed with 4′,6-diamidino-2-phenylindole (blue) to locate the nucleus, and the percentage of apoptosis-positive (red-stained) cells were visualized under a confocal microscope (Leica DMI 4000B; Leica Microsystems, Wetzlar, Germany).
Statistical analysis
All the data are presented as the mean ± standard deviation. Significant differences between two groups were determined using Student’s t-test, while multiple groups were analyzed by one-way analysis of variance with Fisher’s least significant difference. All the statistical analyses were performed with SPSS software (Version 22.0; IBM Corporation, Armonk, NY, USA). A value of P<0.05 was considered significant.
Results
Characterization of the Gem-HSA-NPs
The Gem-HSA-NPs were prepared using nab technology, by which Gem-C14 was mixed with HSA in an aqueous solvent and passed under high pressure through a jet to form drug albumin nanoparticles. As shown in , the average size of the nanoparticles was 150±27 nm, and the polydispersity was 0.162±0.02. Overall, the nanoparticles showed a narrow size distribution, suggesting their potential role as passively targeted antitumor agent. The nanoparticles showed a negative surface potential of −10.2±3.2 mV (), indicating their potential for prolonged blood circulation; nanoparticles with a positive surface charge would interact with serum protein in human blood. The morphology of the Gem-HSA-NPs was observed by transmission electron microscopy. As shown in , under ×20,000 magnification, the Gem-HSA-NPs exhibited a nearly spherical shape with a moderate uniform particle size and even distribution. Under ×50,000 magnification (), the nanoparticles had a brighter core surrounded by a dark membrane, which confirmed the distinct layer.
Figure 1 Particle size and size distribution of Gem-HSA-NP determined using a Malvern Zetasizer (A). Zeta potential spectrum of Gem-HSA-NP in PBS solution (B). TEM image of Gem-HSA-NP under ×20,000 magnification (C). TEM image of Gem-HSA-NP under ×50,000 magnification. The nanoparticles had a brighter core surrounded by a dark membrane (arrow), which confirmed its distinct layers (D).
Abbreviations: Gem-HSA-NP, gemcitabine-loaded human serum albumin nanoparticle; PBS, phosphate-buffered saline; TEM, transmission electron microscopy.
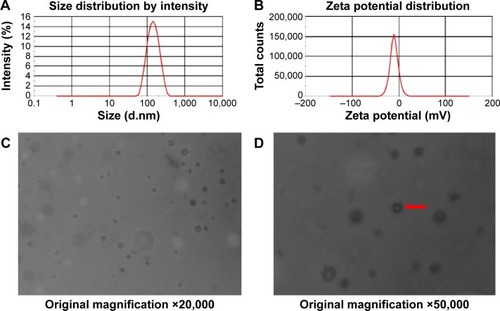
The encapsulation efficiency of Gem-HSA-NPs was 82.99%±3.5%, and the drug-loading efficiency was 10.42%±3.5%. The in vitro release profiles of Gem-HSA-NP were recorded in a cumulative release curve >5 days. As shown in , for the 1st hour, <20% of the drug was released, which shows that Gem-HSA-NPs can retain their stability in solution. Thereafter, the nanoparticles showed an accelerated release, where more than half of the Gem was released by 24 hours. By 48 hours, >70% of the Gem had been released; this was followed by a slow, sustained release. By hour 90, a relative release peak of 81.5% was observed, and this remained stable for the next 30 hours.
Figure 2 The cumulative release curve of Gem-HSA-NPs.
Notes: For the 1st hour, <20% of drugs were released and thereafter, the nanoparticles showed an accelerated release, whereas over half of Gem were released at the time of the 24th hour. For the 48th hour, >70% of Gem were released, followed by a slow sustained-release character. Until the 90th hour, a relative release peak of 81.5% was observed, and this remained stable for the next 30 hours.
Abbreviations: Gem-HSA-NP, gemcitabine-loaded human serum albumin nanoparticle; Gem, gemcitabine.
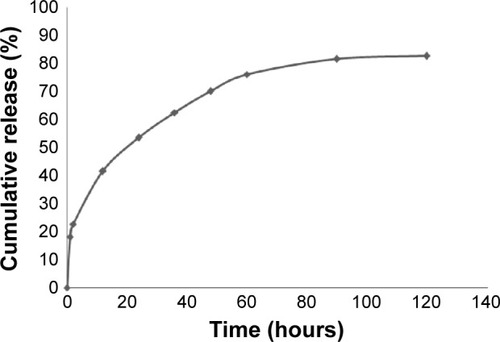
In vitro results
The in vitro cytotoxicity of Gem-HSA-NP was evaluated on the human pancreatic cancer cell line BxPC-3 via the CCK-8 assay. As shown in , the 1–8 surveying points stood for the respective concentrations of 0.01 μg/mL, 0.04 μg/mL, 0.2 μg/mL, 0.5 μg/mL, 1 μg/mL, 5 μg/mL, 10 μg/mL, and 50 μg/mL. All three drugs inhibited cell growth in a dose-dependent manner, in which Gem exhibited the highest cyto-toxicity, followed by Gem-C14 and then Gem-HSA-NPs. The IC50 of Gem iŝ0.2 μg/mL, that of Gem-C14 is 0.35 μg/mL, and that of Gem-HSA-NPs is 0.4 μg/mL.
Figure 3 In vitro cytotoxicity assay of Gem-HSA-NP on BxPC-3 cell line.
Notes: The 1–8 surveying points stood for 0.01 μg/mL, 0.04 μg/mL, 0.2 μg/mL, 0.5 μg/mL, 1 μg/mL, 5 μg/mL, 10 μg/mL, and 50 μg/mL, respectively. All three drugs inhibited cell growth in a dose-dependent manner, in which Gem exhibited the highest cytotoxicity, followed by Gem-C14, whereas the Gem-HSA-NP showed the lowest cytotoxicity level. The IC50 of Gem is ~0.2 μg/mL, that of Gem-C14 is 0.35 μg/mL, and that of Gem-HSA-NPs is 0.4 μg/mL.
Abbreviations: Gem-HSA-NP, gemcitabine-loaded human serum albumin nanoparticle; Gem, gemcitabine; Gem-C14, 4-N-myristoyl-gemcitabine.
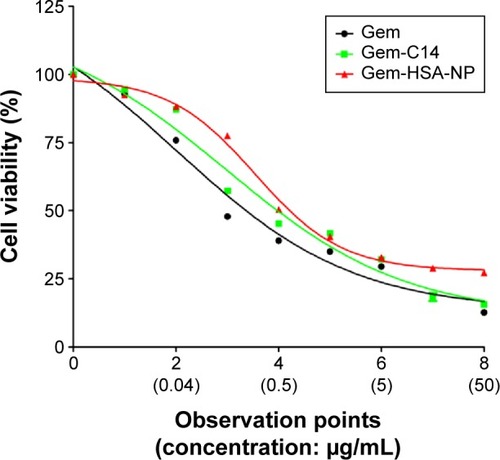
We also assessed the apoptosis assays of the three groups. As shown in , apoptosis and necrosis can be found in all treatment groups, and the total apoptosis rates (including early and late-stage apoptosis) of the three groups (Gem, Gem-C14, and Gem-HSA-NPs) were 34.94%±2.5%, 37.72%±1.8%, and 37.48%±2.6%, respectively, which were not statistically significant (P>0.05).
Figure 4 Annexin V-FITC/PI graphs of flow cytometry for Gem, Gem-C14, and Gem-HSA-NP.
Notes: The apoptotic rates of the three drugs were 34.94%±2.5%, 37.72%±1.8%, and 37.48%±2.6%, respectively. Numbers in the quadrants are percentage of cells in early apoptosis (UR), late apoptosis or necrosis (LR), and cell debris (UL).
Abbreviations: FITC, fluorescein isothiocyanate; Gem, gemcitabine; Gem-HSA-NP, gemcitabine-loaded human serum albumin nanoparticle; UR, upper right; LR, lower right; UL, upper left; PI, propidium iodide.
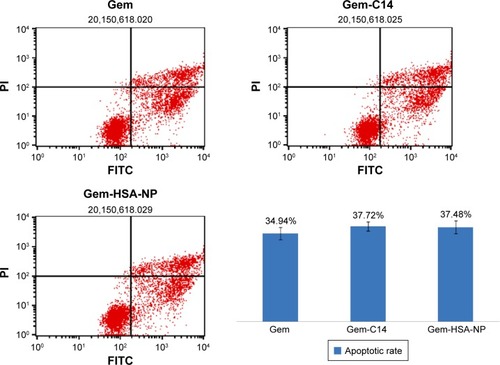
In vivo results
To evaluate the antitumor activity of Gem-HSA-NPs in a human pancreatic cancer xenograft model in vivo, we examined tumor growth and body weight changes in nude mice treated with Gem, Gem-C14, Gem-HSA-NPs, and NS. During the entire study, no mice died from any treatment-related cause. As shown in , ~2 weeks after the treatment, the tumor volume of the mice receiving the Gem formulation followed the order: Gem-HSA-NPs < Gem or Gem-C14 < NS. Tumors in mice treated with the Gem-HSA-NPs grew significantly slower than those in the other groups. shows that the average tumor volumes of mice treated with Gem-HSA-NPs were half the size of those in the Gem group and one-sixth the size of the NS group (P<0.05), whereas, in the Gem and Gem-C14 groups, the tumor volumes were almost the same (P>0.05).
Figure 5 Representative photos of tumors on mice (A). Photographs of tumors harvested from each treatment group after various treatments (B). The tumor volume profiles of mice treated with Gem, Gem-C14, Gem-HSA-NP, and NS (C). The body weight profiles of mice treated with Gem, Gem-C14, Gem-HSA-NP, and NS (D).
Notes: 1–4 represent Gem, Gem-C14, Gem-HSA-NP, and NS group, respectively.
Abbreviations: Gem, gemcitabine; Gem-C14, 4-N-myristoyl-gemcitabine; Gem-HSA-NP, gemcitabine-loaded human serum albumin nanoparticle; NS, normal saline.
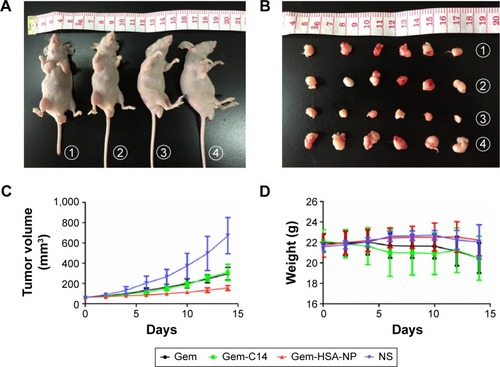
In order to evaluate the safety of the Gem-HSA-NPs, mouse body weights were monitored as a marker of overall toxicity. As shown in , the weight profiles showed a sudden loss on day 4 in both the Gem and Gem-C14 groups, whereas, in the other two groups, the weight remained stable. On day 14, mice in the Gem and Gem-C14 groups suffered a 10% body weight loss (P<0.05) compared with those in the Gem-HSA-NPs group, which indicates rapid disease progression and more severe toxicity.
As shown in , the TUNEL staining was used to label apoptotic cells, indicated by red fluorescence in the tumor section (with blue nuclei). The degree of apoptosis varied as follows: Gem-HSA-NPs > Gem-C14 > Gem > normal control, indicating that the Gem-HSA-NPs were the most effective in inducing apoptosis of tumor cells (see for the data in detail). It should be noted here, however, that the distribution of red fluorescence may also represent blood vessels; therefore, we used 4′,6-diamidino-2-phenylindole to mark the nuclei so as to differentiate the vessels from tumor cells.
Figure 6 The merged TUNEL stainings showed tumor penetration of drugs on mice bearing subcutaneous pancreatic tumors on day 14 after IV administration.
Notes: Frozen sections were examined under a confocal microscope. Nuclei were stained with DAPI (blue), while red represented apoptotic cells. The bar indicated 50 μm.
Abbreviations: TUNEL, transferase dUTP nick end labeling; IV, intravenous; DAPI, 4′,6-diamidino-2-phenylindole; NC, negative control; Gem, gemcitabine; Gem-C14, 4-N-myristoyl-gemcitabine; Gem-HSA-NP, gemcitabine-loaded human serum albumin nanoparticle.
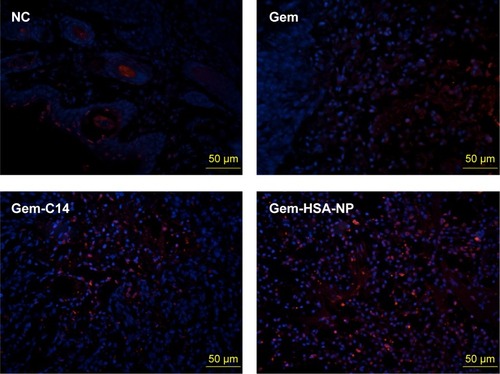
Discussion
Being a nucleoside analog, Gem is rapidly converted into its inactive metabolite dFdU (2′,2′-difluorodeoxyuridine) by cytidine deaminase after systemic administration.Citation31 In addition, Gem is too hydrophilic to cross the cell membrane, which must rely on specialized nucleoside transporters, such as human equilibrative nucleoside transporter 1 and human equilibrative nucleoside transporter 2.Citation32 Several strategies have been proposed to overcome these deficiencies:Citation7,Citation33,Citation34 1) to encapsulate Gem into nanoparticles with the advantages of an EPR effect and controlled-release characteristics; these materials can be albumin, liposome, polymeric compounds, etc and 2) to conjugate an acyl chain with the N-terminus of Gem, thereby enhancing its hydrophobicity. In this paper, we report the successful synthesis of Gem-loaded albumin nanoparticles based on the nab technology for the first time. The whole process can be divided into two steps: first, a lipophilic derivative of Gem, named Gem-C14, is obtained by coupling myristoyl with the 4-amino group of Gem and, second, under optimum circumstances, the Gem-HSA-NPs are synthesized using nab technology.
In recent years, a number of attempts have been made to encapsulate drugs into albumin nanoparticles based on the desolvation–crosslinking method.Citation35–Citation37 Previously, our research group also used a modified desolvation–crosslinking method to produce Gem-loaded albumin nanoparticles in two sizes (110 nm and 406 nm). Although both these nanoparticles showed significant inhibitory effects on human pancreatic cancer cell lines, the inhibition rate was size dependent, and we found that 406 nm nanoparticles demonstrated greater antitumor activity. Particle size and size distribution have important effects on the stability of a nanoparticle system. It is generally believed that particles up to 100–200 nm can be internalized by receptor-mediated endocytosis, whereas larger particles have to be taken up by phagocytosis.Citation38,Citation39 Nanoparticles are prone to aggregate and precipitate if the particles are large or the size distribution is not even. However, owing to the limitations of the desolvation–crosslinking method, it is difficult to control the size and size distribution of particles. With the emergence of nab-paclitaxel as the first-line therapy for advanced pancreatic cancer, more attention has been drawn to the new technology of albumin nanoparticle synthesis. This was developed by American BioScience Inc. and named nab technology, and is ideal for encapsulating lipophilic drugs into nanoparticles. Using this technology, the size of nanoparticles can be controlled in the range of 100–200 nm. In addition, the nanoparticles are stable and evenly distributed. Besides nab-paclitaxel, Kim et alCitation29 used nab technology to prepare curcumin-loaded albumin nanoparticles with a size range of 130–150 nm for intravenous administration. Similarly, in our study, the particle sizes of Gem-HSA-NPs were well controlled in the range of 130–160 nm, with an average of 150±27 nm. During antitumor therapy, this specific size can maximize the EPR effect while avoiding being taken up by the macrophages as well as the reticuloendothelial system.
Another concern may be the potential immunologic response caused by bovine serum albumin, so we used human serum albumin to encapsulate the Gem, as Gem is water soluble and must therefore be transformed into a liposoluble state in order to be encapsulated by nab technology. In our study, we increased the lipophilicity of Gem by coupling the 4-amino group with C14 linear-chain acyl derivatives. Another concern may be raised in terms of the therapeutic efficacy after derivatization of Gem. Both our in vitro and in vivo studies confirmed that Gem-C14 showed equal or even superior efficacy to Gem, and several other reports also support this conclusion.Citation2,Citation40,Citation41 This enhanced antitumor activity may be attributed to the fact that, on the one hand, Gem-C14 has the ability to cross epithelial cells and localize in tumors independent of human equilibrative nucleoside transporter 1, and on the other hand, the metabolic enzyme intercellular carboxylesterase 2 is key for transforming Gem-C14 into Gem, which hydrolyzes the prodrug and confers prodrug sensitivity to cancer cells.Citation42 Theoretically, the albumin nanoparticles have advantages over others in being nontoxic, biocompatible, and biodegradable.Citation43 Besides the EPR effect, the interplay of two albumin-binding proteins may facilitate the uptake and retention of albumin nanoparticles in tumor tissues.Citation44 Our results are also in accordance with this hypothesis. Our in vivo study showed that after five cycles of drug administration, the mice in the Gem and Gem-C14 groups lost 10% of body weight, whereas in the NS and Gem-HSA-NPs groups, the average body weights were almost unchanged. With regard to inhibition of tumor growth, the Gem-HSA-NPs exhibited the strongest inhibitory effect. Furthermore, TUNEL staining also indicated that the Gem-HSA-NPs were the most effective in inducing apoptosis of tumor cells, which were mainly distributed evenly both intratumorally and around tumor vessels. As described earlier, a combined effect of EPR and the active targeting approach of albumin nanoparticles could be the main reasons for this significant suppression of tumor growth.
Compared with the in vivo study, the in vitro results showed no significant differences among the Gem, Gem-C14, and Gem-HSA-NPs groups. There may be two reasons for this: first, with Gem-HSA-NPs, there can be no effect of EPR in vitro. Second, the Gem-HSA-NPs revealed a controlled-release nature: by hour 120, only 82.6% of Gem had been released from the nanoparticles. However, the observation period for both in vitro studies was only 48 hours.
Last but not least, the strength of this study is obvious. This is the first report on the synthesis of Gem-C14, which demonstrates an equal or superior therapeutic effect to Gem both in vitro and in vivo. Furthermore, we were the first to use nab technology to encapsulate Gem-C14 into Gem-HSA-NPs and thereby showed a significantly improved antitumor efficacy over both Gem and Gem-C14. Finally, the limitation of this study is the subcutaneous tumor model, which cannot mimic the real tumor microenvironment of the human body (hypoxia and full of stroma). Although the in vivo results so far are exciting, the delivery of drugs to pancreatic cancer cells is still a challenge in the clinic. Another drawback of current Gem-HSA-NP is its lack of tumor-targeting ability; we are now preparing to attach cyclic arginine-glycine-aspartic-conjugated peptides to it, thereby strengthening its tumor-targeting ability.
Conclusion
In summary, we have successfully synthesized Gem-HSA-NPs. Our in vivo results confirm that these prepared Gem-HSA-NPs can more efficiently inhibit tumor growth in a pancreatic cancer cell line while showing moderate toxicity. However, further studies must be performed to optimize this therapy for clinical use.
Acknowledgments
This work was financially supported by the National Natural Science Foundation of China (81201896 and 81071884) and the Research Fund for the Doctoral Program of Higher Education of China (20130071110052 and 20110071110065).
Supplementary material
Figure S1 Comparison of the average fluorescent intensity in NC, Gem, Gem-C14, and Gem-HSA-NP groups.
Notes: Image J software was used to compare the average fluorescent intensity. Data showed a significant difference during the four groups (P<0.05).
Abbreviations: SD, standard deviation; NC, negative control; Gem, gemcitabine; Gem-C14, 4-N-myristoyl-gemcitabine; Gem-HSA-NP, gemcitabine-loaded human serum albumin nanoparticle.
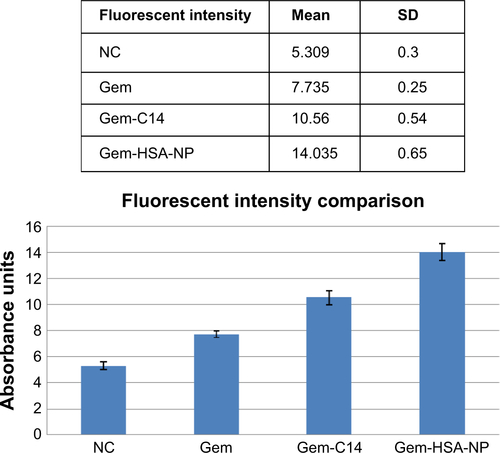
Disclosure
The authors report no conflicts of interest in this work.
References
- SiegelRMaJZouZJemalACancer statistics, 2014CA Cancer J Clin201464192924399786
- BildsteinLDubernetCMarsaudVTransmembrane diffusion of gemcitabine by a nanoparticulate squalenoyl prodrug: an original drug delivery pathwayJ Control Release2010147216317020691740
- GuoLFanLPangZTRAIL and doxorubicin combination enhances anti-glioblastoma effect based on passive tumor targeting of liposomesJ Control Release201115419310221609741
- PeixotoRDHoMRenoufDJEligibility of metastatic pancreatic cancer patients for first-line palliative intent nab-paclitaxel plus gemcitabine versus FOLFIRINOXAm J Clin Oncol Epub201541
- ConroyTDesseigneFYchouMGroupe Tumeurs Digestives of Unicancer; PRODIGE IntergroupFOLFIRINOX versus gemcitabine for metastatic pancreatic cancerN Engl J Med2011364191817182521561347
- KingRSGemcitabine. New first-line therapy for pancreatic cancerCancer Pract1996463533549128490
- MoysanEBastiatGBenoitJPGemcitabine versus modified gemcitabine: a review of several promising chemical modificationsMol Pharm201310243044422978251
- NakanoYTannoSKoizumiKGemcitabine chemoresistance and molecular markers associated with gemcitabine transport and metabolism in human pancreatic cancer cellsBr J Cancer200796345746317224927
- AnderssonRAhoUNilssonBIGemcitabine chemoresistance in pancreatic cancer: molecular mechanisms and potential solutionsScand J Gastroenterol200944778278619214867
- ShengZHuDZhengMSmart human serum albumin-indocyanine green nanoparticles generated by programmed assembly for dual-modal imaging-guided cancer synergistic phototherapyACS Nano2014812123101232225454579
- KratzFA clinical update of using albumin as a drug vehicle – a commentaryJ Control Release201419033133624637463
- MaedaHWuJSawaTMatsumuraYHoriKTumor vascular permeability and the EPR effect in macromolecular therapeutics: a reviewJ Control Release2000651–227128410699287
- GradisharWJAlbumin-bound paclitaxel: a next-generation taxaneExpert Opin Pharmacother2006781041105316722814
- SchnitzerJEOhPAlbondin-mediated capillary permeability to albumin. Differential role of receptors in endothelial transcytosis and endocytosis of native and modified albuminsJ Biol Chem19942698607260828119952
- TiruppathiCSongWBergenfeldtMSassPMalikABGp60 activation mediates albumin transcytosis in endothelial cells by tyrosine kinase-dependent pathwayJ Biol Chem19972724125968259759325331
- JohnTAVogelSMMinshallRDRidgeKTiruppathiCMalikABEvidence for the role of alveolar epithelial gp60 in active transalveolar albumin transport in the rat lungJ Physiol2001533pt 254755911389211
- NeuzilletCTijeras-RaballandACrosJFaivreSHammelPRaymondEStromal expression of SPARC in pancreatic adenocarcinomaCancer Metastasis Rev2013323–458560223690170
- HoangBErnstingMJRoyAMurakamiMUndzysELiSDDocetaxel-carboxymethylcellulose nanoparticles target cells via a SPARC and albumin dependent mechanismBiomaterials201559667625956852
- KratzFAlbumin as a drug carrier: design of prodrugs, drug conjugates and nanoparticlesJ Control Release2008132317118318582981
- ElzoghbyAOSamyWMElgindyNAAlbumin-based nanoparticles as potential controlled release drug delivery systemsJ Control Release2012157216818221839127
- Von HoffDDErvinTArenaFPIncreased survival in pancreatic cancer with nab-paclitaxel plus gemcitabineN Engl J Med2013369181691170324131140
- Von HoffDDRamanathanRKBoradMJGemcitabine plus nab-paclitaxel is an active regimen in patients with advanced pancreatic cancer: a phase I/II trialJ Clin Oncol201129344548455421969517
- LiCLiYGaoYDirect comparison of two albumin-based paclitaxel-loaded nanoparticle formulations: is the crosslinked version more advantageous?Int J Pharm20144681–2152524709221
- MonteroAJAdamsBDiaz-MonteroCMGluckSNab-paclitaxel in the treatment of metastatic breast cancer: a comprehensive reviewExpert Rev Clin Pharmacol20114332933422114779
- YardleyDAnab-Paclitaxel mechanisms of action and deliveryJ Control Release2013170336537223770008
- LiJMChenWWangHPreparation of albumin nanospheres loaded with gemcitabine and their cytotoxicity against BXPC-3 cells in vitroActa Pharmacol Sin20093091337134319730429
- JiSXuJZhangBRGD-conjugated albumin nanoparticles as a novel delivery vehicle in pancreatic cancer therapyCancer Biol Ther201213410
- LiJDiYJinCGemcitabine-loaded albumin nanospheres (GEM-ANPs) inhibit PANC-1 cells in vitro and in vivoNanoscale Res Lett20138117623594566
- KimTHJiangHHYounYSPreparation and characterization of water-soluble albumin-bound curcumin nanoparticles with improved antitumor activityInt J Pharm20114031–228529121035530
- SandovalMASloatBRLansakara-PDSEGFR-targeted stearoyl gemcitabine nanoparticles show enhanced anti-tumor activityJ Control Release2012157228729621871505
- PaolinoDCoscoDRacanicchiLGemcitabine-loaded PEGylated unilamellar liposomes vs GEMZAR: biodistribution, pharmacokinetic features and in vivo antitumor activityJ Control Release2010144214415020184929
- TaoXMWangJCWangJBEnhanced anticancer activity of gemcitabine coupling with conjugated linoleic acid against human breast cancer in vitro and in vivoEur J Pharm Biopharm201282240140922728546
- FedericoCMorittuVMBrittiDTrapassoECoscoDGemcitabine-loaded liposomes: rationale, potentialities and future perspectivesInt J Nanomedicine201275423543623139626
- WangLAnYYuanCGEM-loaded magnetic albumin nanospheres modified with cetuximab for simultaneous targeting, magnetic resonance imaging, and double-targeted thermochemotherapy of pancreatic cancer cellsInt J Nanomedicine2015102507251925848268
- ChitkaraDKumarNBSA-PLGA-based core-shell nanoparticles as carrier system for water-soluble drugsPharm Res20133092396240923756758
- YangRAnYMiaoFLiMLiuPTangQPreparation of folic acid-conjugated, doxorubicin-loaded, magnetic bovine serum albumin nanospheres and their antitumor effects in vitro and in vivoInt J Nanomedicine201494231424325228802
- DubeyRDAlamNSanejaADevelopment and evaluation of folate functionalized albumin nanoparticles for targeted delivery of gemcitabineInt J Pharm20154921–2809126165611
- Yin WinKFengS-SEffects of particle size and surface coating on cellular uptake of polymeric nanoparticles for oral delivery of anticancer drugsBiomaterials200526152713272215585275
- SykesEAChenJZhengGChanWCInvestigating the impact of nanoparticle size on active and passive tumor targeting efficiencyACS Nano2014865696570624821383
- StellaBArpiccoSRoccoFEncapsulation of gemcitabine lipophilic derivatives into polycyanoacrylate nanospheres and nanocapsulesInt J Pharm20073441–2717717651931
- FangYDuFXuYEnhanced cellular uptake and intracellular drug controlled release of VESylated gemcitabine prodrug nanocapsulesColloids Surf B Biointerfaces201512835736225746328
- PrattSEDurland-BusbiceSShepardRLHeinz-TahenyKIversenPWDantzigAHHuman carboxylesterase-2 hydrolyzes the prodrug of gemcitabine (LY2334737) and confers prodrug sensitivity to cancer cellsClin Cancer Res20131951159116823325581
- ElsadekBKratzFImpact of albumin on drug delivery – new applications on the horizonJ Control Release2012157142821959118
- MisakHEAsmatuluRGopuJSAlbumin-based nanocomposite spheres for advanced drug delivery systemsBiotechnol J20149116317024106002