Abstract
The heat shock proteins (HSP) constitute a superfamily of chaperone proteins present in all cells and in all cell compartments, operating in a complex interplay with synergistic/overlapping multiplicity of functions, even though the common effect is cell protection. Several reasons explain the need for investigating HSP in prostate cancer: (1) these molecules function as chaperones of tumorigenesis accompanying the emergence of prostate cancer cells, (2) they appear as useful molecular markers associated with disease aggressiveness and with resistance to anticancer therapies including hormone therapy, radiotherapy, chemotherapy and hyperthermia, and (3) they can be used as targets for therapies. The latter can be accomplished by: (i) interrupting the interaction of HSP (mainly HSPC1) with various client proteins that are protected from degradation when chaperoned by the HSP; (ii) using the chaperone and adjuvant capabilities of certain HSP to present antigenic peptides to the immune system, so this system can recognise the prostate tumour cells as foreign to mount an effective antitumoral response; and (iii) using treatment planning models taking into account the HSP expression levels to obtain more effective therapies. In summary, the study of the HSP during tumorigenesis as well as during cancer progression, and the inclusion of treatment designs targeting HSP combined with other treatment modalities, should improve prostate cancer survival in the near future.
Introduction
Needles to say, the diagnosis of prostate cancer is very frequent in elderly men, in fact, based on pathological studies of ‘normal’ prostate glands at autopsy, almost 70% of men have prostate cancer when they reach 70 years-old Citation[1]. The difficulty is that many of these tumours are relatively indolent; they are occult causing no problems to the host, while others will grow to produce the typical aggressive cancer disease. This divergent clinical behaviour is attributed to dissimilar and largely unknown genetic and epigenetic mechanisms. Therefore, one of the main problems to be resolved is not only the early detection of the disease but also to detect those tumours with an aggressive behaviour Citation[2]. For practical purposes this is of very high importance since the local treatments (surgery and radiotherapy) are costly and can cause urinary incontinence, sexual dysfunction and others, while on the other hand, the metastatic disease can be controlled by blocking hormone levels until the disease becomes resistant and practically incurable Citation[3]. Nowadays, therefore, the area of early cancer detection using biomarkers, as well as their significance as prognostic and predictive factors, is under intensive re-evaluation and study (see an excellent review by Brawley et al. Citation[4]). Researchers are concerned about utility of ‘classic’ screening methods such as the prostate specific antigen (PSA) and about the ways to improve the identification of aggressive tumours that will progress if left untreated. For example, the Gleason score is very useful but is not perfect. Hence, several biomarkers are being evaluated and among them are the HSP (see prognosis below).
Another reason for investigating the HSP in prostate cancer is because hyperthermia is being used in some countries (e.g. Germany, Italy, Japan) alone, or more commonly in combination with other therapies to treat patients with various types of cancer, and prostate cancer is one of the main targets for this kind of therapy Citation[5–8]. Although we do not have yet a complete understanding of the molecular mechanisms by which hyperthermia facilitates the killing of cancer cells (we will see later that protein aggregation is of paramount importance), it is clear that when the temperature rises without cell killing there is induction of HSP. And the HSP have been implicated among others in the development of thermotolerance Citation[9], Citation[10]. Moreover, cancer cells need HSP to accompany many molecular events during carcinogenesis and cancer progression Citation[11], Citation[12] and for this reason several HSP are already over expressed in prostate cancer (as well as in many other cancer types) at the time of diagnosis and treatment.
The heat shock proteins
Briefly, the HSP constitute a superfamily of proteins characterised by their different molecular weight. In a recent publication the nomenclature of these proteins has been unified Citation[13] and we will use this to refer to the different HSP. summarises the new nomenclature to help reading. The HSP response is elicited by heat shock (this was historically the first stimulus found to induce the HSP synthesis giving rise to their name), but later it was found that several physiological and pathological conditions induce synthesis of the HSP; due to this the HSP are also known as stress responsive proteins Citation[14–16]. In the cells there are basal constitutive levels of HSP known as constitutive or cognate HSP, for example HSPB1 is under oestrogen regulation, and at these basal levels the HSP perform ‘house-keeping’ functions. In contrast, when the HSP are induced by stressful situations they are known as inducible HSP. The same HSP can have both roles (e.g. there are constitutive levels of HSPB1 and when a stress occurs, the same HSP is increased), while at other times the stress can induce different but closely related HSP. For example HSPA8 is the cognate/constitutive HSPA and this was previously known as HSC70 or HSP73, while HSPA1A and HSPA1B are the inducible forms and were previously known as HSP70-1 and HSP70-2 (which differ by only two amino acids)Citation[13]. In vertebrates the synthesis of these proteins is under control of at least four different heat shock factors (HSF1-4, with HSF1 as the main HSF activated by stress). Upon physiological or pathological stressors the inactive HSF1 (monomer) is quickly activated, the trimeric form undergoes various post-translational modifications (phosphorylation, acetylation and sumoylation) that regulate its interaction with heat shock responsive elements on the heat shock genesCitation[17], Citation[18]. Moreover, HSF1 is implicated in the regulation of other genes (e.g. participating in the repression of oestrogen-dependent transcription acting in combination with other molecules)Citation[19].
Table I. New nomenclature of the main HSP (complete list in Kampinga et al. Citation[13]).
The HSP work as a team having overlapping functions, they are present in all cells, and in all cell compartments. gives an overview of the complex functions of the HSP even though the common effect is cell protection.
Figure 1. Schematic representation of the HSP response and the implications of these proteins in cell functions. The numerous roles of this superfamily of proteins may explain that, during evolution, gene duplication appeared as a mode of adaptation providing the cells with the functionality of the HSP. HSFs: Heat Shock Factors.
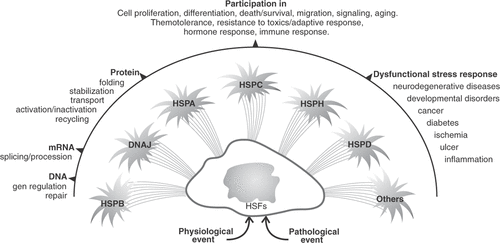
During carcinogenesis there are several situations that alter the cellular protein homeostasis that explain the de-repression of HSP genes: infection by oncogenic viruses (HPV, adenovirus, HCV), exposure to carcinogenic compounds, expression of mutated oncogenic or anti-oncogenic proteins, changes in the immune and endocrine systems, and the cell exposure to hypoxia, starvation and to endogenous and exogenous protein-damaging stresses associated with misfolded proteins. HSP are required to maintain in a stable and active conformation important signalling proteins triggered by growth signals, and they participate in inhibitory pathways of senescence and death Citation[11], Citation[12]. Moreover, anticancer treatments such as cytotoxic drugs and radiotherapy can affect HSP expression levels with important clinical consequences on cell growth and survival. All of these give rise to the development of new anticancer strategies based on HSP, and to the exploration of the utility of HSP as cancer biomarkers Citation[20], Citation[21].
Since the synthesis of HSP is triggered by heat shock it is not surprising to find that they are implicated in thermo resistance. For example, HSPA1A is one of the important HSP implicated in thermo tolerance. It has been shown in a cultured prostate cancer cell line that higher levels of HSPA1A were associated with heat resistance Citation[10]. On the other hand, very intense heat such as that applied to kill metastatic cancer foci in the liver produces necrosis; however, a mild or moderate heat (42°–45°C) produces breakage of bonds at protein level inducing protein instability, denaturation and finally collapse of protein structure, resulting in protein aggregation. This is a signal for a HSP response, they are in charge of further protein denaturation and they are in charge to restore protein conformation. If the proteins cannot be restored they are cleaved (ubiquitination) and recycled. Heat also produces double-strand break (DSB) formation via protein denaturation, and in addition, there is production of free radicals which can also induce DSBs in the DNA Citation[22]. If the cell is able to mount a competent HSP response, the HSP (HSPB1 and HSPA1A) can accumulate in the nucleus contributing to DNA polymerase-beta reactivation and stimulation, which results in thermotolerance. Takahashi et al. Citation[22] showed that thermotolerance is partially suppressed in DNA polymerase-beta (-/-) mouse fibroblasts (when compared with the wild-type cells) and also in the presence of a HSP inhibitor.
Cultured cells and animal models
Antisense design
HSPB1 is emerging as an important therapeutic target to improve the response of prostate cancer cells to different treatments, for example DU145 cells transfected with full length HSPB1 antisense cDNA showed significantly increased sensitivity to gamma radiation Citation[23]. On the other hand, the HSPB1 siRNA developed by Rocchi et al. Citation[24] inhibited HSPB1 expression and significantly reduced the in vitro growth of both the androgen independent (AI) PC-3 cells and the androgen dependent (AD) LNCaP cells. However, the HSPB1 knockdown inhibited more significantly the growth of the AI PC-3 cells than the androgen dependent LNCa-P cells, which may reflect a greater dependence on HSPB1 for the androgen independent prostate cancer cell growth. Also, an increase of caspase-3 cleavage and apoptosis was observed in both cell lines by diminishing HSPB1 levels Citation[24]. In a recent study by Andrieu et al. Citation[25] it has been found that HSPB1 confers resistance to androgen ablation and chemotherapy through eIF4E protein (eukaryotic translation initiation factor 4E). HSPB1 down-regulation decreased eIF4E protein expression in prostate cancer cells; HSPB1 co-localized and interacted with this protein decreasing eIF4E ubiquitination and proteasomal degradation. These findings illustrate the utility of silencing the anti-apoptotic HSPB1 molecule as a form of therapy in prostate cancer cells. However, the application of RNAi depends on the efficient intracellular delivery of siRNAs Citation[26]. Polyamidoamine (PAMAM) dendrimers are effective vectors for siRNA delivery to target HSPB1 and serve to protect the RNA molecules from enzymatic degradation, allowing effective siRNA delivery Citation[27]. Recently it has been documented that silencing of the HSPB1 gene through PAMAM dendrimers and siRNA delivery led to induction of caspase 3/7 dependent apoptosis and inhibition of PC-3 cell growth in vitro Citation[28]. In this manner, the siRNA delivery ensured by the dendrimers represents a great promise to further in vivo therapeutic application in cancer.
There is evidence that androgen receptor (AR) regulates HSPA1A expression in human prostatic cells. Dihydrotestosterone enhanced HSPA1A expression in AD-LNCaP cells, while this HSP was down-regulated by anti-androgens and knocking down AR Citation[29]. The apoptosis of prostate cancer cells induced by androgen ablation could be due to, at least in part, the decrease of the anti-apoptotic HSPA1 protein. The resistance to hormone therapy could be due to the re-establishment of HSPA1A levels when this protein becomes independent of AR. Therefore, the interference of HSPA1A could also be of therapeutic interest in prostate cancer.
HSPC inhibitors
Agents that target AR expression represent an attractive treatment option for prostate cancer patients with disease progression following castration (a common element of the resistance mechanism is restoration/maintenance of AR signalling). Prior to ligand binding, AR exists in a complex with HSPC proteins and with other co-chaperones. The AR-HSPC interaction maintains AR in a high-affinity ligand-binding conformation, which is necessary for efficient hormone response. Then, HSPC ‘clients’ include wild-type and mutated AR and several proteins of potential importance in mediating the progression of prostate and other cancers like HER2 and Akt Citation[30]. These data suggest that HSPC inhibitors may represent a novel strategy for the treatment of patients with prostate cancer, and clinical trials testing this hypothesis are currently ongoing (see treatment chapter below). 17-Allyamino-17-demethoxygeldanamycin (17-AAG) is an N-terminal inhibitor of the HSPC chaperone protein. Inhibition of HSPC function causes the proteasomal degradation of proteins that require this chaperone for maturation or stability. In murine models of prostate cancer, 17-AAG caused the degradation of these client proteins, at non-toxic doses, and inhibited the growth of hormone-naїve and castration-resistant tumours. Recently it has been reported a new novobiocin analogue designed to inhibit the C-terminal portion of HSPC1 (F-4) Citation[31]. This new inhibitor demonstrated improved potency and efficacy and decreased expression of client proteins in LNCaP and PC-3 cells; PSA secretion was inhibited in a dose-dependent manner that matched with the decrease of AR expression. The dietary bioflavonoid quercetin has been involved in preventing oncogenesis, specifically in prostate cancer. Quercetin treatment of prostate cancer cell lines resulted in inhibition of HSPC, decreased cell proliferation and viability, and increased apoptosis, while exerting no quantifiable effect on normal prostate epithelia cells Citation[32].
Other targets
On the other hand, a promising new strategy for prevention and/or treatment of castration-resistant prostate cancer is represented by Histone deacetylase 6 (HDAC6). Recent studies on C4-2 cells, and C4-2 xenograft tumour established in castrated but not in testes-intact nude mice, suggested that HDAC6 regulates AR hypersensitivity and nuclear localization, mainly via modulating HSPC1 acetylation Citation[33]. The knockdown of HDAC6 in C4-2 cells using short hairpin RNA resulted in ligand-independent nuclear localisation of endogenous AR and inhibited PSA expression and cell growth in the absence or presence of dihydrotestosterone. Targeting HDAC6 alone, or in combination with other therapeutic approaches, represents a promising new strategy for prevention and/or treatment of castration-resistant prostate cancer.
A mitochondria-localised HSPC chaperone, tumour necrosis factor receptor-associated protein-1 (TRAP1/HSPC5), is abundantly and ubiquitously expressed in human high-grade prostatic intraepithelial neoplasia, Gleason grades 3–5 prostatic adenocarcinomas, and metastatic prostate cancer, but largely undetectable in normal prostate or benign prostatic hyperplasia in vivo Citation[34]. Expression of HSPC5 in no transformed prostatic epithelial BPH-1 cells inhibited cell death, whereas silencing of HSPC5 in androgen-independent PC-3 or DU145 prostate cancer cells by siRNA, enhanced apoptosis. Targeting HSPC5 with a novel class of mitochondria-directed HSPC inhibitors (e.g. Gamitrinib) caused rapid and complete killing of androgen-dependent and -independent prostate cancer, but not BPH-1 cells, whereas reintroduction of HSPC5 in BPH-1 cells conferred sensitivity to Gamitrinib-induced cell death Citation[34].
The secreted mammalian chaperone clusterin (sCLU) can interact with and inhibit activated Bax, inhibiting cytochrome C release and caspase activation Citation[35]. In prostate cancer, sCLU levels have previously been correlated with Gleason grade Citation[36] and although sCLU expression is low or absent in most untreated hormone-naїve tissues, the levels increased significantly within weeks after neoadjuvant hormone therapy Citation[37]. In this study, the authors identified sCLU as over-expressed in a docetaxel-resistant PC-3 subline (PC-3dR) and reported for the first time that sCLU knockdown using sequence-specific ASO or siRNA chemo sensitised this cell line to taxane and mitoxantrone-based chemotherapy, both in vitro and in vivo Citation[38].
Immune-related HSP
Proteins identified by proteomic approaches have previously been associated with prostate cancer progression such as HSPA5, and HSPC4, among others Citation[39]. Amongst the up-regulated proteins, increased HSPA5 expression has been reported in metastatic prostate cancer in the bone Citation[40], and increased expression has been shown in metastatic LNCaP-LN3 cells compared with poorly metastatic LNCaP cells Citation[41]. Furthermore, increased HSPA5 expression has been associated with the development of androgen-independent prostate cancer Citation[42]. A possible mechanism for this may involve inhibition of apoptosis due to the ability of HSPA5 to interact with intermediate molecules of the apoptotic pathway Citation[43]. Thus, the anti-apoptotic role of HSPA5 could facilitate prostate cancer progression.
Another up-regulated HSP, HSPC4, is a glycoprotein belonging to the HSP family which has a role in protein homeostasis, cell differentiation, development, and has also been shown to play a role in eliciting antitumour immunity Citation[44], Citation[45]. In these studies HSPC4 expression has been associated with prostate cancer progression using patient's material; additionally a previous study Citation[41] has shown increased expression levels in LNCaP-LN3 cells compared with LNCaP cells. Furthermore, HSPC4 has been shown to undergo alterations in glycosylation associated with increased malignant behaviour Citation[46]. Thus, their finding of significantly increased HSPC4 immunoexpression in prostate cancer and precursor lesions suggests that, in addition to altered glycosylation, up-regulation of HSPC4 may also be involved in cancer progression, and is likely to be involved at an early stage Citation[39].
Secretion of stress protein HSPH4 promotes immune-mediated inhibition of murine prostate tumour. In a recent study it has been demonstrated that genetic modification of weakly immunogenic murine prostate tumour cells (TRAMP-C) by stable transfection with a secreteable form of endoplasmic reticulum resident chaperone HSPH4, significantly enhanced its immunogenicity in vivo, through the generation of tumour specific T-cell responses (CD8+). Furthermore, generation of systemic antitumour immunity is indicated by the growth suppression of distant parental tumours, which is associated with increased tumour infiltration Citation[47]. The systemic tumour immunity may be used to improve treatment outcomes for prostate cancer when combined with other treatment modalities. For example, adenovirally delivered nitroreductase with a prodrug that can be transformed into a cytotoxic DNA-cross-linking derivative at tumour site, induces tumour cell killing. This effect was enhanced by raising HSPA1A which caused immune stimulation Citation[48]. The use of such cytotoxic and immunomodulatory gene combinations, possibly in conjunction with other treatments such as radiotherapy and chemotherapy, warrants optimistic results.
Finally, circulating auto antibodies against HSPA5 are present at high levels in prostate cancer patients and are potential biomarkers of aggressive tumour behaviour Citation[40]. Thus, the epitope specificity and function of anti- HSPA5 antibodies produced by prostate cancer patients have been investigated in 1-LN, PC-3, DU145, and LNCaP prostate cancer cells. The results of this study showed that the anti- HSPA5 antibodies from prostate cancer patients bind to, and stimulate, proliferation of tumour cells expressing HSPA5 on their surface, and protected them from apoptosis induced by tumour necrosis factor α in a dose-dependent manner Citation[49].
Implications of HSP during prostate carcinogenesis
Carcinogenesis involves a cascade of molecular events that mediate the transformation of normal cells into cancer cells. Although prostate cancer is a malignancy with a high incidence, the events associated with its initiation remain poorly understood and there are still many enigmas about the pathophysiology of prostate cancer.
Early prostate tumorigenesis appears to be associated with a dysplasia that initiates with proliferative inflammatory atrophy (PIA), and progresses to prostatic intraepithelial neoplasia (PIN), which in some cases leads to carcinoma. Existing evidence suggests that these early lesions may be initiated by inflammation that occurs with exposure to different infectious agents and/or ingestion of carcinogens. When a premalignant lesion progresses to primary cancer, to metastatic cancer, and to androgen-independent cancer, genetic alterations continue to accumulate within the tumour cells. Moreover, normal prostate and early-stage prostate cancers cells depend on androgens for growth and survival. As the cancer advances and metastasizes, it becomes dominated by cells that proliferate and survive independently of androgens. With a practical/didactic purpose we can identify the following entities during prostate cancer progression: (1) normal prostate epithelium, (2) PIA, (3) PIN, (4) localised prostate cancer, (5) metastatic prostate cancer (all of them androgen-dependent), and (6) androgen-independent prostate cancer.
Owing to their role as molecular chaperones, HSP participate in many events related to cancer, starting from the beginning of carcinogenesis Citation[12]. During this process, the transformed cells begin to express abnormal/elevated levels of HSP, and in some cases this induction continues during tumour progression. At present there exists an important body of evidence to support the participation of this family of proteins in the initiation and progression of prostate carcinogenesis. In accordance with the above, an interesting paper of Byun et al. Citation[50] has demonstrated that during prostate tumorigenesis the expression of several sets of housekeeping genes (including HSP) are differentially expressed, suggesting that the process is driven by modulation of the expression of these genes. The expression of HSP was up-regulated during the transition of localised prostate cancer to metastatic prostate cancer, indicating that in advanced stages prostate tumour cells could be under cellular stress. Therefore, the authors suggest that during this period of cellular stress the prostate tumour may be more vulnerable and responsive to treatment. summarises the studies about the HSP expression during the different phases of prostate cancer progression. The identification and assessment of level of these genes/proteins in the prostate tumour progression will allow the best management of prostate cancer patients and to improve the treatments that have HSP as potential targets for the therapy.
Table II. Studies evaluating HSP during the tumorigenesis of prostate cancer.
Heat shock proteins in the prognosis of the disease
In a previous article our laboratory has reviewed the implications of HSP in the prognosis of cancer patients including those with prostate cancer Citation[62]. The main conclusion was that HSPB1 expression was seen in a high percentage of hormone-refractory patients and correlated with poor clinical outcome and shorter survival. The new data confirm these observations (). For example, Glaessgen et al. Citation[65] found that, although HSPB1 did not appear as an independent predictor of disease outcome, HSPB1 immunoreactivity correlated with Gleason score and predicted biochemical disease recurrence. Due to space limitations we cannot analyse each article in depth.
Table III. Studies evaluating HSP in the prognosis of prostate cancer patients.
Another molecule of interest is HSPD1, which appeared as an independent predictor of disease outcome. In the list of relevant HSP molecules to be evaluated in prostate cancer patients we can add HSPA5 as well as clusterin (a cytoprotective antiapoptotic chaperone that has been reviewed by So et al. Citation[69]) ().
shows examples of HSP expression levels in an early cancer stage and in an advanced cancer stage. It is evident that several HSP are co-expressed in advanced disease resistant to conventional treatment. A similar situation has been reported in breast cancer patients where both HSPB1 and HSPA1A have been found highly expressed in patients with locally advanced disease displaying resistance to neoadjuvant chemotherapy Citation[70]. In another article one of us (DRC) is reviewing the implications of the HSP in DNA repair mechanisms which has important implications in cancer cell survival both to endogenous and exogenous (i.e., chemotherapy) genotoxic stimuli Citation[71]. The high HSP expression levels in prostate with aggressive and therapy resistant cancer cells could be exploited to detect the problematic tumours by measuring the HSP levels in urine samples after prostate massage. The newer molecular analysis should incorporate some of the HSP (i.e., HSPB1, HSPD1, HSPA5, clusterin) to improve the diagnosis and prognosis of the disease and also to guide the antitumoural designs targeting HSP in combination to conventional treatments.
Figure 2. Examples of the expression of HSP in prostate tissues. In a patient with an early tumour (PIN in a tru-cut biopsy), the cytokeratin 7 (A) shows the basal cells in the normal gland while in the PIN (right side) these cells are lacking and there is aberrant cytokeratin 7 expression in a tumour cell clone (note the brown positive immunoreaction, diaminobenzidine). In the serial section, HSPB1 appears in the stroma and with weak immunoreactivity in the normal gland but is absent in the PIN cells (B). In contrast, HSF1 and several HSP appear in the cancer tissue from a patient with advanced disease (metastasis in a cervical lymph node). This patient failed to respond to the GnRH agonist treatment and to suffered bone metastases. (C) note the variable expression of HSPB1 in cancer cells; (D) HSPA1A and HSPA8 (these two forms are recognised by the BRM22 monoclonal antibody) appear in the nuclei and cytoplasm in the tumour cell clusters; (E) HSF1 in the nuclei of the tumour cells; (F) HSPD1 in the cytoplasm of tumour cells; and (G) HSPA5. Original magnification ×30 (A, B), ×120 (C–G). The tissues were lightly counterstained with haematoxylin to reveal nuclei.
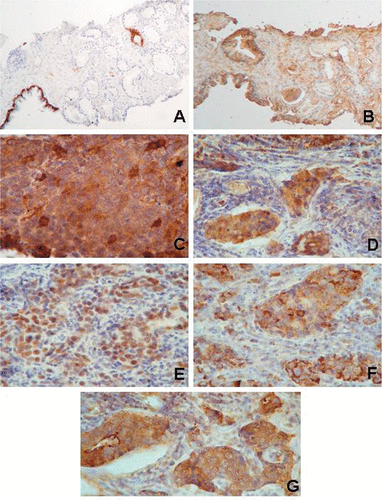
Treatment designs targeting HSP
The pre-clinical and clinical therapeutic implications of HSP in cancer have been reviewed elsewhere Citation[62]. These novel approaches still consider the HSP as key targets for cancer therapy by: (1) interrupting the interaction of HSP (mainly HSPC1) with various client proteins that are protected from degradation when chaperoned by the HSP; (2) using the chaperone and adjuvant capabilities of certain HSP to present antigenic peptides to the immune system, so this can recognise the tumour cells as foreign to mount an effective antitumoral response; and (3) using treatment planning models taking into account the HSP expression levels to obtain more effective thermal therapies. summarises the current approaches using HSP as targets for therapies. Here we can add that the genotoxic etoposide can also disrupt the AR-HSPC association interfering with the AR signalling (impeding the binding of synthetic androgen to AR) in LNCap cells Citation[87]. This is a good example that anticancer agents can act through multiple mechanisms involving among others the HSP. These combined antitumoural strategies should improve prostate cancer survival in the near future.
Table IV. Targeting HSP in prostate cancer.
Acknowledgements
The authors thank the editor and reviewers for their helpful suggestions to improve the manuscript.
Declaration of interest: This review was supported by grants from the National Research Council (CONICET, PIP2428), the National Agency for Scientific and Technological Promotion of Argentina (PICT 1047, Préstamo BID), and the Argentina Foundation for Cancer Research. The authors have no financial interest in or financial conflict with the subject matter or materials discussed in the manuscript.
References
- Delongchamps NB, Singh A, Hass GP. Epidemiology of prostate cancer in Africa: Another step in the understanding of the disease?. Curr Probl Cancer 2007; 31: 226–236
- Rubin MA. Targeted therapy of cancer: New roles for pathologists – prostate cancer. Mod Pathol 2008; 21: S44–55
- Sharifi N, Dahut WL, Figg WD. Secondary hormonal therapy for prostate cancer: What lies on the horizon?. BJU Int 2008; 101: 271–274
- Brawley OW, Ankerst DP, Thompson IM. Screening for prostate cancer. CA Cancer J Clin 2009; 59: 264–273
- Shinohara K. Thermal ablation of prostate diseases: Advantages and limitations. Int J Hyperthermia 2004; 20: 679–697
- Hurwitz MD, Kaplan ID, Hansen JL, Prokopios-Davos S, Topulos GP, Wishnow K, Manola J, Bornstein BA, Hynynen K. Hyperthermia combined with radiation treatment of locally advanced prostate cancer is associated with favourable toxicity profile. Int J Hyperthermia 2005; 21: 649–656
- Latorre M, Rinaldi C. Application of magnetic nanoparticles in medicine: Magnetic fluid hyperthermia. P R Health Sci 2009; 28: 227–238
- Baronzio G, Gramaglia A, Fiorentini G. Review. Current role and future perspectives of hyperthermia for prostate cancer treatment. In vivo 2009; 23: 143–146
- Schlesinger MJ. How the cell copes with stress and the function of heat shock proteins. Pediatr Res 1994; 36: 1–6
- Khoei S, Goliaei B, Neshasteh-Riz A, Deizadji A. The role of heat shock protein 70 in the thermoresistance of prostate cancer cell line spheroids. FEBS Lett 2004; 561: 144–148
- Calderwood SK, Khaleque MA, Sawyer DB, Ciocca DR. Heat shock proteins in cancer: Chaperones of tumorigenesis. Trends Biochem Sci 2006; 31: 164–172
- Ciocca DR, Fanelli MA, Cuello-Carrion FD, Calderwood SK. Implications of heat shock proteins in carcinogenesis and cancer progression. In: Calderwood SK, Sherman MY, Ciocca DR, editors. Heat Shock Proteins in Cancer. The Netherlands, Dordrecht: Springer; 2007. pp 31–51.
- Kampinga HH, Hageman J, Vos MJ, Kubota H, Tanguay RM, Bruford EA, Chetham ME, Chen B, Hightower LE. Guidelines for the nomenclature of the human heat shock proteins. Cell Stress Chaperones 2009; 14: 105–111
- Linquist S, Craig EA. The heat shock proteins. Ann Rev Genet 1988; 22: 631–637
- Ciocca DR, Oesterreich GC, Chamness GC, McGuire WL, Fuqua SAW. Heat shock protein 27,000 (HSP 27): Biological and clinical implications. J Natl Cancer Inst 1993; 85: 1558–1570
- Wegele H, Müller L, Buchner J. Hsp70 and Hsp90 – A relay team for protein folding. Rev Physiol Biochem Pharmacol 2004; 151: 1–44
- Sandqvist A, Björk JK, Akerfelt M, Chitikova Z, Grichine A, Vourćh C, Jolly C, Salminen TA, Nymalm Y, Sistonen L. Heterotrimerization of heat-shock factors 1 and 2 provided a transcriptional switch in response to distinct stimuli. Mol Biol Cell 2009; 20: 1340–1347
- Westerheide SD, Anckar J, Stevens SM, Jr, Sistonen L, Morimoto RI. Stress-inducible regulation of heat shock factor 1 by the deacetylase SIRT1. Science 2009; 323: 1063–1066
- Khaleque MA, Bharti A, Gong J, Gary PJ, Sachdev V, Ciocca DR, Stati A, Fanelli MA, Calderwood SK. Heat shock factor 1 represses estrogen-dependent transcription through association with MTA1. Oncogene 2008; 27: 1886–1893
- Calderwood SK, Ciocca DR, Gray PJ, Zaarur N, Lepchammer S, Sherman MY. The elevated levels of heat shock proteins in cancer: A suitable case for treatment? In: Calderwood SK, Sherman MY, Ciocca DR, editors. Heat Shock Proteins in Cancer. The Netherlands, Dordrecht: Springer. 2007. pp 351–365.
- Ciocca DR, Fanelli MA, Cuello-Carrion FD, Castro GN. Small stress proteins, biomarkers of cancer. In: Arrigo AP, Simon S, editors. Small Stress Proteins in Human Diseases. New York: Nova Science Pub. 2010 (in press).
- Takahashi A, Yamakawa N, Mori E, Ohnishi K, Yokota S, Sugo N, Aratani Y, Koyama H, Ohnishi T. Development of thermotolerance requires interaction between polymerase-beta and heat shock proteins. Cancer Sci 2008; 99: 973–978
- Teimourian S, Jalal R, Sohrabpour M, Goliaei B. Down-regulation of Hsp27 radiosensitizes prostate cancer cells. Internat J Urology 2006; 13: 1221–1225
- Rocchi P, Jugpal P, So A, Sinneman S, Ettinger S, Fazli L, Nelson C, Gleave M. Small interference RNA targeting heat shock protein 27 inhibits the growth of prostatic cell lines and induces apoptosis via caspase-3 activation in vitro. BJU International 2006; 98: 1082–1089
- Andrieu C, Taieb D, Baylot V, Ettinger S, Soubeyran P, De-Thonel A, Nelson C, Garrido C, So A, Fazli L, et al. Heat shock protein 27 confers resistance to androgen ablation and chemotherapy in prostate cancer cells through eIF4E. Oncogene 2010;29:1883–1896.
- Aagaard L, Rossi JJ. RNAi therapeutics: Principles, prospects and challenges. Adv Drug Delivery Rev 2007; 59: 75–86
- Zhou JH, Wu JY, Hafdi N, Behr J-P, Erbacher P, Peng L. PAMAM dendrimers for efficient siRNA delivery and potent gene silencing. Chem Commun 2006; 22: 2362–2364
- Liu X, Rocchi P, Qu F, Zheng S, Liang Z, Gleave M, Iovanna J, Peng L. PAMAM dendrimers mediate siRNA delivery to target Hsp27 and produce potent antiproliferative effects on prostate cancer cells. Chem Med Chem 2009; 4: 1302–1310
- Lu S, Tan Z, Wortman M, Lu S, Dong Z. Regulation of heat shock protein 70-1 expression by androgen receptor and its signaling in human prostate cancer cells. Int J Oncol 2010; 36: 459–467
- Solit DB, Scher HI, Rosen N. Hsp90 as a therapeutic target in prostate cancer. Semin Oncol 2003; 30: 709–716
- Matthews S, Vielhauer G, Manthe C, Chaguturu V, Szabla K Matts R, Donnelly A, Blagg B, Holzbeiertein J. Characterization of a novel novobiocin analogue as a putative C-teminal inhibitor of heat shock protein 90 in prostate cancer cells. Prostate 2010; 70: 27–36
- Aalinkeel R, Bindukumar B, Reynolds J, Sykes D, Mahaian S, Chadha K, Schwartz S. The dietary bioflavonoid, quercetin, selectively induces apoptosis of prostate cancer cells by down regulating the expression of heat shock protein 90. Prostate 2008; 68: 1773–1789
- Ai J, Wang Y, Dar JA, Liu J, Liu L, Nelson JB, Wang Z. HDAC6 regulates androgen receptor hypersensitivity and nuclear localization via modulating Hsp90 acetylation in castration-resistant prostate cancer. Mol Endocrinol 2009; 23: 1963–1972
- Leav I, Plescia J, Goel HL, Li J, Jiang Z, Cohen RJ, Languino LR, Altieri DC. Cytoprotective mitochondrial chaperone TRAP-1 as a novel molecular target in localized and metastatic prostate cancer. Am J Pathol 2010;176:393–401.
- Zhang H, Kim JK, Edwards CA, Xu Z, Taichman R, Wang CY. Clusterin inhibits apoptosis by interacting with activated Bax. Nat Cell Biol 2005; 7: 909–915
- Steinberg J, Oyasu R, Lang S, Sintich S, Rademaken A, Lee C, Kozlowski JM, Sensiban JA. Intracellular levels of SGP-2 (Clusterin) correlate with tumor grade in prostate cancer. Clin Cancer Res 1997; 3: 1707–1711
- July LV, Akbari M, Zellweger T, Jones EC, Goldenberg SL, Gleave ME. Clusterin expression is significantly enhanced in prostate cancer cells following androgen withdrawal therapy. Prostate 2002; 50: 179–188
- Sowery R, Hadaschik B, So A, Zoubeidi A, Fazli L, Hurtado-Coll A, Gleave M. Clusterin knockdown using the antisense oligonucleotide OGX-011 re-sensitizes docetaxel-refractory prostate cancer PC-3 cells to chemotherapy. Br J Urol Int 2008; 102: 389–397
- Glen A, Gan C, Hamdy F, Eaton C, Cross S, Catto J, Wright P, Rehman I. iTRAQ-facilitated proteomic analysis of human prostate cancer cells identifies proteins associated with progression. J Proteome Res 2008; 7: 897–907
- Mintz PJ, Kim J, Do KA, Wang X, Zinner RG, Cristofanilli M, Arap MA, Hong WK, Troncoso P, Logothetis CJ, Pasqualini R, Arap W. Fingerprinting the circulating repertoire of antibodies from cancer patients. Nat Biotechnol 2003; 21: 57–63
- Wu Y, Zhang H, Dong Y, Park YM, Ip C. Endoplasmic reticulum stress signal mediators are targets of selenium action. Cancer Res 2005; 65: 9073–9079
- Pootrakul L, Datar RH, Shi SR, Cai J, Hawes D, Groshen SG, Lee AS, Cote RJ. Expression of stress response protein Grp78 is associated with the development of castration-resistant prostate cancer. Clin Cancer Res 2006; 12: 5987–5993
- Miyake H, Hara I, Arakawa S, Kamidono S. Stress protein GRP78 prevents apoptosis induced by calcium ionophore, ionomycin, but not by glycosylation inhibitor, tunicamycin, in human prostate cancer cells. J Cell Biochem 2000; 77: 396–408
- Yang Y, Li Z. Roles of heat shock protein gp96 in the ER quality control: Redundant or unique function. Mol Cells 2005; 20: 173–182
- PeibinY, Shude Y, Changzhi H. Heat shock protein gp96 and cancer immunotherapy. Chin Med Sci J 2002; 17: 251–256
- Suriano R, Ghosh S K, Ashok BT, Mittelman A, Chen Y, Banerjee A, Tiwari RK. Differences in glycosylation patterns of heat shock protein, gp96: Implications for prostate cancer prevention. Cancer Res 2005; 65: 6466–6475
- Gao P, Sun X, Chen X, Subjeck J, Wang X. Secretion of stress protein grp170 promotes immune-mediated inhibition of murine prostate tumor. Cancer Immunol Immunother 2009; 58: 1319–1328
- Lipinski K, Pelech S, Mountain A, Irvine A, Kraaij R, Bangma C, Mills K, Todryk S. Nitroreductase-based therapy of prostate cancer, enhanced by raising expression of heat shock protein 70, acts through increased anti-tumour immunity. Cancer Immunol Immunother 2006; 55: 347–354
- Gonzalez-Gronow M, Cuchacovich M, Llanos C, Urzua C, Gawdi G, Pizzo1 S. Prostate cancer cell proliferation in vitro is modulated by antibodies against glucose-regulated protein 78 isolated from patient serum. Cancer Res 2006; 66: 11424–11431
- Byun J, Logothetis CJ, Gorlov IP. Housekeeping genes in prostate tumorigenesis. Int J Cancer 2009; 125: 2603–2608
- Ummanni R, Junker H, Zimmermann U, Venz S, Teller S, Giebel J, Scharf C, Woenckhaus C, Dombrowski F, Walther R. Prohibitin identified by proteomic analysis of prostate biopsies distinguishes hyperplasia and cancer. Cancer Lett 2008; 266: 171–185
- Lin JF, Xu J, Tian HY, Gao X, Chen QX, Gu Q, Xu GJ, Song JD, Zhao FK. Identification of candidate prostate cancer biomarkers in prostate needle biopsy specimens using proteomic analysis. Int J Cancer 2007; 121: 2596–2605
- Howard EW, Leung SC, Yuen HF, Chua CW, Lee DT, Chan KW, Wang X, Wong YC. Decreased adhesiveness, resistance to anoikis and suppression of GRP94 are integral to the survival of circulating tumor cells in prostate cancer. Clin Exp Metastasis 2008; 25: 497–508
- Elmore LW, Forsythe R, Forsythe H, Bright AT, Nasim S, Endo K, Holt SE. Overexpression of telomerase-associated chaperone proteins in prostatic intraepithelial neoplasia and carcinomas. Oncol Rep 2008; 20: 613–617
- Cardillo MR, Ippoliti F. IL-6, IL-10 and HSP-90 expression in tissue microarrays from human prostate cancer assessed by computer-assisted image analysis. Anticancer Res 2006; 26: 3409–3416
- Mori R, Wang Q, Danenberg KD, Pinski JK, Danenberg PV. Both beta-actin and GAPDH are useful reference genes for normalization of quantitative RT-PCR in human FFPE tissue samples of prostate cancer. Prostate 2008; 68: 1555–1560
- Sfar S, Saad H, Mosbah F, Chouchane L. Association of HSP70-hom genetic variant with prostate cancer risk. Mol Biol Rep 2008; 35: 459–464
- Lexander H, Palmberg C, Auer G, Hellström M, Franzén B, Jörnvall H, Egevad L. Proteomic analysis of protein expression in prostate cancer. Anal Quant Cytol Histol 2005; 27: 263–272
- Cappello F, Ribbene A, Campanella C, Czarnecka AM, Anzalone R, Bucchieri F, Palma A, Zummo G. The value of immunohistochemical research on PCNA, p53 and heat shock proteins in prostate cancer management: A review. Eur J Histochem 2006; 50: 25–34
- Zhen B, Shen Y, Zhang YM, Zhu CH, Liu ZL. Analysis of the differences in the expression of HSP27 and c-kit between benign prostatic hyperplasia and prostatic cancer tissues. Zhonghua Nan Ke Xue 2006; 12: 416–420
- Buchanan G, Ricciardelli C, Harris JM, Prescott J, Yu ZC, Jia L, Butler LM, Marshall VR, Scher HI, Gerald WL, et al. Control of androgen receptor signaling in prostate cancer by the cochaperone small glutamine rich tetratricopeptide repeat containing protein alpha. Cancer Res 2007; 67: 10087–10096
- Ciocca DR, Calderwood SK. Heat shock proteins in cancer: Diagnostic, prognostic, predictive and treatment implications. Cell Stress Chaperones 2005; 10: 86–103
- Miyake H, Muramaki M, Kurahashi T, Yamanaka K, Hara I, Fijisawa M. Enhanced expression of heat shock protein 27 following neoadjuvant hormonal therapy is associated with poor clinical outcome in patients undergoing radical prostatectomy for prostate cancer. Anticancer Res 2006; 26: 1583–1587
- Kurahashi T, Miyake H, Hara I, Fijisawa M. Expression of major heat shock proteins in prostate cancer: Correlation with clinicopathological outcomes in patients with radical prostatectomy. J Urol 2007; 177: 757–761
- Glaessgen A, Jonmarker S, Lindberg A, Nilsson B, Lewensohn R, Ekman P, Valdman A, Egevad L. Heat shock proteins 27, 60 and 70 as prognostic markers of prostate cancer. Acta Pathol Microbiol Immunol Scand 2008; 116: 888–895
- Pootrakul L, Datar RH, Shi SR, Cai J, Hawes D, Groshen SG, Lee AS, Cote RJ. Expression of stress response protein Grp78 is associated with the development of castration-resistance prostate cancer. Clin Cancer Res 2006; 12: 5987–5993
- Daneshmand S, Quek ML, Lin E, Cote RJ, Hawes D, Cai J, Groshen S, Lieskovsky G, Skinner DG, Lee AS, Pinski J. Glucose-regulated protein GRP78 is up-regulated in prostate cancer and correlates with recurrence and survival. Human Pathol 2007;38:1547–1552.
- Miyake H, Muramaki M, Kurahashi T, Takenaka A, Fujisawa M. Expression of potential molecular markers in prostate cancer: correlation with clinicopathological outcomes in patients undergoing radical prostatectomy. Urol Oncol 2010;28:145–151.
- So A, Hadaschik B, Sowery R, Gleave M. The role of stress proteins in prostate cancer. Current Genomics 2007; 8: 252–261
- Vargas-Roig LM, Gago FE, Tello O, Aznar JC, Ciocca DR. Heat shock protein expression and drug resistance in breast cancer patients treated with induction chemotherapy. Int J Cancer (Pred Oncol) 1998; 79: 468–475
- Nadin S, Ciocca DR. Participation of heat shock proteins in DNA repair mechanisms in cancer. In: Thomas AE, editor, DNA Repair: Damage, Repair Mechanisms and Aging. New York: Nova Science Publishers; 2010, (in press).
- Lattouf JB, Srinivasan R, Pinto PA, Linehan WM, Neckers L. Mechanisms of disease: The role of heat-shock protein 90 in genitourinary malignancy. Nat Clin Pract Urol 2006; 3: 590–601
- Powers MV, Workman P. Targeting of multiple signaling pathways by heat shock protein 90 molecular chaperone inhibitors. Endocr Relat Cancer 2006; 13: S125–135
- Kang BH, Altieri DC. Compartmentalized cancer drug discovery targeting mitochondrial Hsp90 chaperones. Oncogene 2009; 28: 3681–3688
- Taplin ME. Androgen receptor: Role and novel prospects in prostate cancer. Expert Rev Anticancer Ther 2008; 8: 1495–1508
- Chen Y, Sawyers CL, Scher HI. Targeting the androgen receptor pathway in prostate cancer. Curr Opin Pharmacol 2008; 8: 440–448
- Banerji U. Heat shock protein 90 as a drug target: Some like it hot. Clin Cancer Res 2009; 15: 9–14
- Rocchi P, Beraldi E, Ettinger S, Fazli L, Vessella RL, Nelson C, Gleave M. Increased Hsp27 after androgen ablation facilitates androgen-independent progression in prostate cancer via signal transducers and activators of transcription 3-mediated suppression of apoptosis. Cancer Res 2005; 65: 11083–11093
- Kang SH, Kang KW, Kim K-H, Kwon B, Kim S-K, Lee H-Y, Kong S-Y, Lee ES, Jang S-G, Yoo BC. Upregulated HSP27 in human breast cancer cells reduces Herceptin susceptibility by increasing Her2 protein stability. Br Med Council Cancer 2008; 8: 286–296
- Ronquist KG, Carlsson L, Ronquist G, Nilsson S, Larsson A. Prostasome-derived proteins capable of eliciting an immune response in prostate cancer patients. Int J Cancer 2006; 119: 847–853
- Ciocca DR, Frayssinet P, Cuello-Carrion FD. A pilot study with a therapeutic vaccine based on hydroxyapatite ceramic particles and self-antigens in cancer patients. Cell Stress Chaperones 2007; 12: 33–43
- Kottke T, Sanchez-Perez L, Diaz RM, Thompson J, Chong H, Harrington K, Calderwood SK, Pulido J, Georgopoulos N, Selby P, et al. Induction of hsp70-mediated Th17 autoimmunity can be exploited as immunotherapy for metastatic prostate cancer. Cancer Res 2007; 67: 11970–11979
- Gao P, Sun X, Chen X, Subjeck J, Wang X-Y. Secretion of stress protein grp170 promotes immune-mediated inhibition of murine prostate tumor. Cancer Immunol Immunother 2009; 58: 1319–1328
- Brusa D, Migliore E, Garetto S, Simone M, Matera L. Immunogenicity of 56°C and UVC-treated prostate cancer is associated with release of HSP70 and HMGB1 from necrotic cells. Prostate 2009; 69: 1343–1352
- Srivastava PK, Callahan MK, Mauri MM. Treating human cancers with heat shock protein-peptide complexes: The road ahead. Expert Opin Biol Ther 2009; 9: 179–186
- Rylander MN, Feng Y, Bass J, Diller KR. Heat shock protein expression and injury optimization for laser therapy designs. Lasers Surg Med 2007; 39: 731–746
- Liu S, Yamauchi H. Etoposide induces growth arrest and disrupts androgen receptor signaling in prostate cancer cells. Oncol Rep 2010; 23: 165–170