Abstract
The Heidelberg Ion Therapy Center (HIT) started clinical operation in November 2009. In this report we present the first 80 patients treated with proton and carbon ion radiotherapy and describe patient selection, treatment planning and daily treatment for different indications. Patients and methods. Between November 15, 2009 and April 15, 2010, 80 patients were treated at the Heidelberg Ion Therapy Center (HIT) with carbon ion and proton radiotherapy. Main treated indications consisted of skull base chordoma (n = 9) and chondrosarcoma (n = 18), malignant salivary gland tumors (n=29), chordomas of the sacrum (n = 5), low grade glioma (n=3), primary and recurrent malignant astrocytoma and glioblastoma (n=7) and well as osteosarcoma (n = 3). Of these patients, four pediatric patients aged under 18 years were treated. Results. All patients were treated using the intensity-modulated rasterscanning technique. Seventy six patients were treated with carbon ions (95%), and four patients were treated with protons. In all patients x-ray imaging was performed prior to each fraction. Treatment concepts were based on the initial experiences with carbon ion therapy at the Gesellschaft für Schwerionenforschung (GSI) including carbon-only treatments and carbon-boost treatments with photon-IMRT. The average time per fraction in the treatment room per patient was 29 minutes; for irradiation only, the mean time including all patients was 16 minutes. Position verification was performed prior to every treatment fraction with orthogonal x-ray imaging. Conclusion. Particle therapy could be included successfully into the clinical routine at the Department of Radiation Oncology in Heidelberg. Numerous clinical trials will subsequently be initiated to precisely define the role of proton and carbon ion radiotherapy in radiation oncology.
Since 1997, the Department of Radiation Oncology in Heidelberg has provided treatment with particle therapy within the clinical routine. The Heidelberg Ion Therapy Center (HIT) started clinical operation in November 2009, providing the capacity to treat over 1 300 patients per year with proton and carbon ion radiotherapy in three treatment rooms using active beam delivery with intensity modulated rasterscanning [Citation1]. Until 2007, about 450 patients were treated with carbon ion radiation therapy in cooperation with the Gesellschaft für Schwerionenforschung (GSI) and the German Cancer Research Center (DKFZ) as well as with the Forschungszentrum Rossendorf in Dresden, Germany. Main indications treated were chordomas and chondrosarcomas of the skull base, adenoid cystic carcinomas, meningioma, sacral chordoma and chondrosarcoma as well as prostate cancer [Citation2–6].
Particle therapy offers distinct physical and biological characteristics compared to photon radiotherapy, however, these properties differ significantly between low-linear energy transfer (LET) beams such as protons and high-LET beams such as carbon ions. The physical advantage of ions compared to photons includes a low energy deposition within the entry channel of the particle beam, with a steep dose deposition peak, which is called “Bragg Peak” named after the British physicist Sir Wilhelm Henry Bragg. The depth of this Bragg peak can be placed by defined variation of the beam energy in the accelerator and beam transport system. Thereafter, a sharp dose fall-off can achieve low energy deposition after the Bragg Peak, and normal tissue can be spared significantly behind the target volume. The lateral dose fall-off can be tailored to the individual clinical requirements by actively adjusting the size of the pencil-beam. Together with these physical characteristics, carbon ions offer an enhanced relative biological effectiveness (RBE). Several clinical and pre-clinical studies have shown the variability of RBE depending on a number of factors including cell type, dose, energy of the beam etc. [Citation7–9]. To account for this heterogeneity within the normal tissue and the target volume, biological treatment planning was developed at GSI based on the local effect model by Scholz and colleagues [Citation7,Citation8]. Using the TRiP Software developed at GSI, about 450 patients were treated with this biological plan optimization with convincing results, and therefore will be continued at HIT [Citation10,Citation11].
The HIT center is equipped with three treatment rooms, two with a horizontal beam line and one with a carbon ion and proton gantry [Citation12]. In the first step, treatment was initiated in the first horizontal treatment room. In a second step, the second horizontal treatment room will start clinical operation, and the gantry will be commissioned thereafter. The rooms are equipped with a patient couch on a robotic arm, as well as an imaging robot mounted at the ceiling of the room. Particle therapy is delivered using active raster scanning as developed by Haberer and colleagues [Citation13]. Using the scanning technique, the radiation volume is divided into virtual slices, which are subdivided into a raster of voxel points; using beam energies of up to a maximum of 221 MeV/u for Protons and 430 MeV/u for carbon ions, these slices are scanned sequentially using the focussed particle pencil beam. This is achieved through vertical and horizontal magnets, as well as adaptation of the beam energy.
In this manuscript we describe the first 80 patients treated at HIT with carbon ions and protons at a horizontal beam line including patient selection, treatment planning, time effort for treatment and setup accuracy.
Patients and methods
Patient selection
Between November 15, 2009 and April 15, 2010, 80 patients have been treated at the Heidelberg Ion Therapy Center (HIT) with carbon ion and proton radiotherapy.
The main indications included skull base chordoma (n= 9) and chondrosarcoma (n= 18), malignant salivary gland tumors of the head-and-neck region (n= 29), chordomas of the sacrum (n = 5), low grade glioma (n= 3), primary and recurrent malignant astrocytoma and glioblastoma (n= 7) and well as osteosarcoma (n= 3). Of these patients, four pediatric patients aged under 18 years were treated. summarizes the patients’ characteristics.
Table I. Patients’ characteristics of the first 80 patients treated at the Heidelberg Ion Therapy center (HIT).
Mean patient age was 53 years (range 12–78 years), 44 patients were male and 36 patients were female. In 67 patients (84%), particle therapy was performed as primary radiotherapy, and in 13 patients (16%) it was performed for recurrent tumors as re-irradiation.
Particle therapy was combined with systemic chemotherapy in five patients with glioblastoma (temozolomide) or head-and-neck tumors (cisplatinum).
Patient immobilization
Immobilization was performed using different immobilization techniques and devices depending on the clinical performance status of the patient and the location treated to minimize setup deviations or inter-and intrafractional movement as well as setup errors. Patients with intracerebral lesions or lesions of the skull base were either immobilized using Scotch Cast mask system (n=64) as published previously [Citation3,Citation14], or soft thermoplastic masks (n=6). For extracranial lesions (n=6), individual fixation devices included vacuum bags or only pillows for the head and feet. One patient treated for a chondrosarcoma of the left heel was treated with an individual fixation device constructed of thermoplastic material.
For brain and skull base tumors fixed with the Scotch Cast Mask, Stereotactic Target Point localization using the stereotactic frame as described previously was performed [Citation3,Citation14]. For all other patients, patient positioning was conducted with the laser system in the treatment room using Beekley-Spots® on the thermoplastic mask system or with three target points tattooed and marked with metal fiducials during the planning CT scan.
Treatment planning
The treatment planning system used for the treatment of patients described here is the prototype of a commercial software developed by Siemens Oncology Care Systems (Syngo PT Planning, Siemens, Germany). It features all aspects of modern 3D treatment planning and is dedicated to planning of scanned proton and ion beam treatments.
Treatment planning was performed using CT scans with and without contrast enhancement as well as contrast-enhanced MRI in all patients. Plan calculation was based on the treatment planning CT without contrast enhancement. Within the treatment planning system (Siemens PT Planning, Siemens, Erlangen, Germany) all imaging examinations required for treatment planning were fused to the three-dimensional data cube. Contouring of target volumes as well as organs at risk (OAR) was performed using the Siemens Dosimetrist and Oncologist software tools. For volume definition, ICRU criteria were set as the standard including a gross tumor volume (GTV) for any macroscopic tumor, a clinical target volume (CTV) for any microscopic spread depending on histology, and a planning target volume (PTV) for setup deviations which was defined depending on the location and the fixation device used. Individual PTV margins, especially for extracranial targets, were chosen together with the medical physicist conducting treatment planning.
The optimization of the scan control parameters for the raster scanning technique within the TPS is done with respect to the biological effective dose of the particles. For protons, a fixed value for the relative biological effectiveness (RBE) of 1.1 is used clinically. For carbon ions, the optimization is based on a radiobiological model, which takes into account the variations of RBE within the radiation quality and as a function of tissue type and fraction dose. The underlying model is the local effect model (LEM), which was developed and validated during the pilot project at GSI. This model allows the inclusion of organ and tumor specific RBE values. Within the first phase of the project, emphasis was put on late effects in normal tissues and a constant, relatively low α/β value of 2 was chosen correspondingly.
The final treatment plans have all been verified dosimetrically. This procedure is also supported by the TPS, which provides the dose distribution in a water phantom calculated from the control parameters of each treatment field. For several defined measurement positions, the dose is then determined using a set of 24 small volume ion chambers (Pinpoint chambers, PTW, Freiburg) within a water phantom. By comparison of measured with calculated dose values the correct dose calculation and beam delivery can be checked prior to the application of the treatment field to the patient.
Pre-treatment orthogonal x-ray imaging was performed prior to each treatment fraction applied. Once the imaging was completed, correlation of the planning CT DRRs with the orthogonal x-ray was performed focussing on bony landmarks. This registration process and the subsequent performance of the calculated correction vector was supervised by a radiation oncologist together with the radiation therapist. Position correction was performed using re-positioning of the treatment couch as well as using the pitch-and-roll feature of the robotic table system in some patients.
Follow-up
All patients are included in a strict radiooncological follow-up regimen started with a first visit four to six weeks after completion of radiotherapy, thereafter in three months intervals for the first year, and subsequently in individual time frames depending on the oncological guideline of the tumor entity. Follow-up examinations include a thorough clinical and neurological assessment, as well as contrast-enhanced MR-imaging. Additional examinations are schedules as needed clinically.
Results
Since the start of the clinical use of HIT the technical systems were operated routinely for about 100 days. The two-stage particle accelerator system combines a linear accelerator with a compact synchrotron to deliver libraries of scanning-ready pencil-beams to the rasterscanners in front of the treatment rooms. Daily quality assurance (QA) showed excellent stability of the beam parameters and only minor retuning of the beam position at the isocenter was needed to correct misalignments being less than 2 mm. Such a procedure for a single ion species takes some hours only to readjust the beamline settings of the range/energy library of 250 steps. The availability of the pencil-beams for the scanning-beam dose delivery was well above 96%. Resuming patient treatment after a complete power failure of all systems within HIT due to construction activities at the university hospital took five hours only including QA.
The medical-technological (medtech) equipment at the treatment rooms, i.e rasterscanners, robotic patient positioners, robot imagers, controls and operating environment and the hospital IT-interface, supported stable clinical workflows for the relevant tasks. During the dose delivery the interlock frequency was about 1.5 per field. The automized matching capability of the x-ray verification images with the CT-cube acquired prior to treatment planning including a full feed-back to the robotic patient couch proved to be a valuable tool to optimize the patient position based on bony landmarks. Daily dosimetry showed an excellent stability for protons as well as carbon ions. The standard deviation is 1.2% and field homogeneity typically better than 3%. The availability of the medtech systems was about 94% and rescheduling of patients was rarely needed.
The treatments during this first startup period have all been performed in a single treatment room equipped with a fixed horizontal beam line. Daily clinical operation starts after the daily QA procedures which took between 90–180 min. This relatively long time was due to extensive tests which were performed in order to gain confidence in the overall performance of the system.
Treatments were performed within one daily shift, five days per week. One shift per week is reserved for maintenance work of the facility. Regular time slots for periodic QA and plan verifications are scheduled twice a week and request about two shifts per week. Additional one to two shifts per week are scheduled for radiobiological and medical physics research. Within the remaining time (around 60% overall) further developments of the system is performed by Siemens in the other treatment rooms. This will continue for several months and includes developments of upgraded versions of the treatment control system, monitoring system, robotic patient positioning and imaging systems as well as dedicated solutions for the gantry treatment room. After an initial phase of four weeks of operation a scheduled yearly shutdown of ten days at the end of the year was also included.
Overall, the whole facility has been operating very reliably during the first 100 days of clinical operation with only a single day of interruption where not all patients could be treated and a few minor interruptions of only a few hours. The performance characteristics of the machine turned out to be very stable and within the tolerances, without the necessity of retuning of the machine. The overall up-time of the synchrotron during the startup-period was around 94%.
Treatment plans and indications
All patients were treated using the intensity-modulated rasterscanning technique. Seventy six patients were treated with carbon ions (95%), and four patients were treated with protons.
Particle therapy is known for superior dose distributions due to the physical characteristics of the particle beam. Several studies have shown convincing clinical results, with proton or with carbon ion radiotherapy. However, to date, hardly any randomized clinical trials have been performed to optimally define the role of particle therapy. This is a clear task for the near future. However, to demonstrate the possibilities using an ion beam for treatment of different target volumes, depict treatment plans for various anatomical locations using protons or carbon ions.
Figure 1. a, Carbon ion radiotherapy for recurrent glioblastoma using two horizontal beams. Treatment was performed up to a total dose fo 36 Gy E in 12 fractions ((A) axial, (B) sagittal and (C) coronal view). b, A–D – contrast-enhanced T1-weighted MR-imaging performed for treatment planning prior to carbon ion radiotherapy. E–H – neuroimaging performed 6 weeks after completion of carbon ion radiotherapy with 36 Gy E.
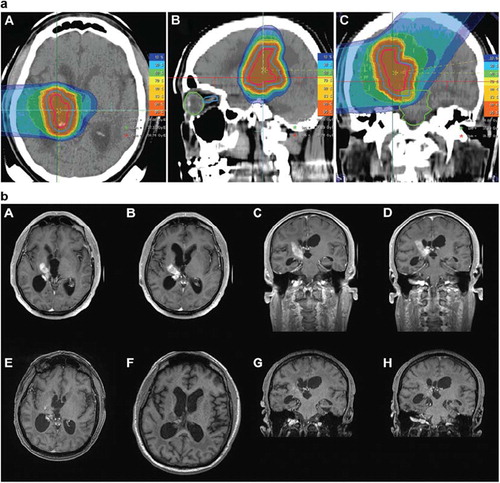
Figure 2. Carbon ion radiotherapy for histologicall confimred sacral chordoma treated with two horizontal beams up to a total dose of 60 Gy E in single fractions of 3 Gy E.
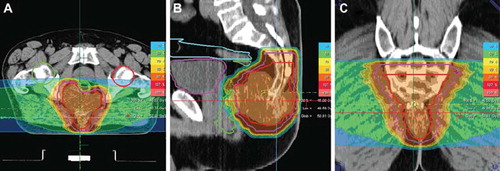
Figure 3. Typical treatment plan for a patient with a skull base chordoma treated up to a total dose of 66 Gy E with carbon ion radiotherapy.
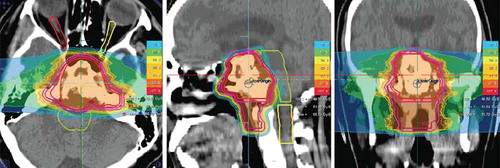
Figure 4. Proton radiotherapy applied with two hirizontal beams for a pediatric low-grade glioma clinically progressive after wait-and-see strategy; a total dose of 54 Gy E protons in single doses of 1.8 Gy E was performed.
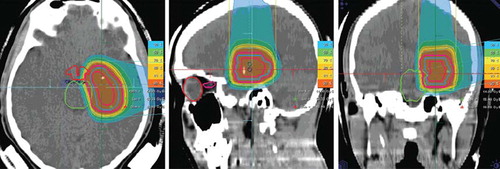
depicts a 50-year-old patient treated for a recurrent glioblastoma in the right-sided internal capsula presenting after initial neurosurgical partial resection, radio-chemotherapy with temozolomide, as well as systemic treatment for recurrence including temozolomide and cilengitide. This patient was treated with a total dose of 36 Gy E in single fractions of 3 Gy E of carbon ions. At the first follow-up visit six weeks after treatment, the tumor demonstrated a significant response to treatment as shown in , and the patient remained stable neurologically. After carbon ion radiotherapy, no additional systemic treatment had been performed.
In a patient with a sacral chordoma is shown referred to HIT by a large German surgical center for carbon ion radiotherapy. This patient was treated with a total dose of 60 Gy E of carbon ions. During follow-up, no treatment-related side effects developed, and the patient did not show any clinical symptoms.
Main indications for carbon ions treated were chordomas and chondrosarcomas of the skull base as well as malignant salivary gland tumors were carbon ion radiotherapy is a clear indication based on the previous clinical studies performed by the department. In we show a patient with a skull base chordoma treated with carbon ion radiotherapy up to a total dose of 66 Gy E in single fractions of 3.3 Gy E after partial neurosurgical resection. We treated in a two-step dose concept as published previously: 49.5 Gy E were delivered to the macroscopic tumor including any suspected subclinical disease based on surgical reports as well as histology; in all cases, this included the clivus and prevertebral muscles down to the basis of the second cervical vertebra. Thereafter, the remaining dose was prescribed to the macroscopic tumor adding a safety margin of about 2 mm.
Proton therapy was mainly performed for pediatric patients; demonstrates a 17-year-old patient with a low-grade glioma of the left-sided amygdala. Initially following wait-and-see strategy after histological classification of the lesion by a biopsy, the patient presented with increasing clinical symptoms mainly with temporal lobe seizures uncontrollable by medication.
He was treated with 54 Gy E at 1.8 Gy E single doses of proton radiotherapy in accordance with the most recent pediatric study protocol for low-grade gliomas (SIOP LGG-2004), the treatment plan is shown in .
Treatment concepts. In principle, all patients will be treated within clinical trials at HIT. For chordomas, chondrosarcomas and adnoid-cystic carcinomas treated with carbon ion radiotherapy, the treatment concepts had been established at GSI and are offered within clinical routine. For all other indications, clinical protocols have been generated and will be activated.
For skull base tumors, carbon ion radiotherapy was performed alone in a median of 20 fractions up to a median total dose of 60 Gy E. For malignant salivary gland tumors, carbon ion radiotherapy was performed as a boost treatment to any macroscopic residual and regions of R1-resection of the tumor, in combination with photon radiotherapy performed as intensity-modulated radiotherapy. The carbon ion boost was applied up to a total dose of 18 Gy E to 24 Gy E, and photon IMRT was prescribed with a median dose of 50 Gy.
For sacral chordomas, carbon ion radiotherapy alone was performed for smaller lesions, and for target volumes exceeding 1l a combination of a carbon ion boost with photon IMRT was performed.
Primary glioblastoma patients and patients with WHO Grade III primay astrocytoma were treated with a carbon ion boost of 18 Gy E in 6 fractions to the macroscopic tumor, and the clinical target volume (CTV) was treated with photon radiotherapy up to 50 Gy. In these patients, radiation was combined with temozolomide representing the treatment standard for this indication. Recurrent glioblastomas were treated with carbon ions only up to a total dose fo 36 Gy E in 12 fractions. Protons were used for low-grade gliomas (pilocytic and WHO Grade II) in conventional fractionation of 1.8 Gy.
Time effort for patient positioning, imaging and irradiation. In the first treatment room with a horizontal beam line the overall clinical performance could be optimized quickly and within two weeks 14–16 patients could be treated daily. The average time per fraction in the treatment room per patient was 29 minutes; for irradiation only, the mean time including all patients was 16 minutes. For imaging and position verification, a mean of 8.7 additional minutes can be added to the overall treatment time per fraction. This included acquisition of images, image registration and matching as well as position correction.
For skull base tumors, the mean treatment time per fraction was 28.7 minutes, whereas for tumors of the sacral region the mean treatment time was 33 minutes per fraction.
Position verification was performed prior to every treatment fraction with orthogonal x-ray imaging. For base of skull tumors fixed in individual masks imaging was performed once prior to treatment. For sacral tumors, in most cases position verification was performed prior to each treatment portal to correct possible misalignment occurring during the longer treatment times due to the larger target volumes. For all patients, a correction vector was calculated by the determined values of the correction along the x, y and z axis. The Siemens robot system for the patient couch allows an additional freedom of couch rotations (pitch and roll) which was not routinely used for every patient. For example, in skull base tumors representing the largest group of patients treated, the median correction along the axis was 2.3 mm for x, 2.7 mm for y and 0.8 mm for z, representing a median correction vector of 3.6 mm not taking into account any pitch-and-roll corrections. For head and neck patients fixed within the thermoplastic mask system the median corrections amounted to 3.6 mm for x, 1.2 mm, for y, and 2.2 mm for z and a median vector of 4.4 mm.
In patients treated for tumors of the sacrum, the median corrections were 2.5 mm for x, 4.7 mm for y and 8.7 mm for z, with a median correction vector of 10 mm.
Discussion
Particle therapy has been implemented successfully into the clinical routine at the Department of Radiation Oncology in Heidelberg, Germany. Within five months, 80 patients with different indications could be treated successfully. Main indications chosen for particle therapy were skull base chordomas and chondrosarcomas as well as adenoid-cystic carcinomas based on the experience of the department acquired over ten years with carbon ion radiotherapy at GSI [Citation2,Citation3,Citation5,Citation14]. Therefore, most patients reported in this manuscript were treated within the established clinical indications at our center. In analogy to study protocols that will be initiated shortly several other indications were treated as individual treatment concepts.
The optimal indications for protons and carbon ions alike have yet to be defined, since, although numerous clinical studies have been published on particle therapy, no randomized clinical trials have been performed as of today. For protons, the reduction of integral dose while being comparable in biological effectiveness, the necessity for clinical trials might be discussed controversially [Citation15–17]. For carbon ions, especially due to their distinct biological characteristics and their possibility to apply altered fractionation schemes with a trend to hypofractionation, warrants evaluation within clinical trials [Citation18–21].
The new center operated highly effective with 96% up-time with overall excellent stability of the system. For the treated patients safety and excellent tolerability of the treatment could be shown. With these 80 patients treated successfully in the new center a new era of radiation oncology has started. HIT is the first center in the Western World offering carbon ion radiotherapy in clinical routine. Commissioning and start-up of this center is clearly a team-effort including technical, physical and medical expertise, as well as a great portion of endurance and enthusiasm. However, facing the hurdles over the last months and years, this is now rewarded by a running center offering highly advanced oncological treatment.
Most patients were treated with carbon ion radiotherapy based on the clinical experience of the department. For primary brain tumors, especially in low-grade lesions, proton radiotherapy was chosen. For high grade tumors, the particle boost was performed with carbon ions to potentially exploit the biological characteristics of the carbon beam for tumor control, which is a concept that was performed in analogy to a clinical trial which will be initiated in due course (CLEOPATRA-Trial).
In the near future, this center will give the opportunity to initiate clinical trials in different indications to further establish and define the role of particle therapy in radiation oncology. To meet the demand for particle treatment in Europe, a number of centers in Germany as well as in other European countries are under construction and will resume clinical operation within the next years. Joint interdisciplinary effort and common approaches among the European centers are already under way. For example, European Union funded projects such as ULICE (Union of Light Ion Centers in Europe), PARTNER or ENVISION are pursuing the goal of a joint approach in basic and clinical research in the field.
The physical and biological benefits of particle therapy have been shown over the last decades. To further prove the value of particle therapy, clinical studies in different indications will be initiated in the near future. Until now, for a number of indications excellent clinical results could be published, however no randomized clinical trials comparing carbon ions to photons or protons, or comparing protons to photons, have been performed. Carbon ion radiotherapy is only available at a few centers worldwide, and most patients have been treated at Japanese facilities. For hepatocellular carcinoma, sarcomas of the bone and soft tissues, as well as for certain head-and-neck tumors or lung cancer the benefit of the carbon beam could be demonstrated by Japanese data [Citation22]. However, all Japanese centers deliver the carbon beam using passive beam delivery, and active beam delivery for carbon beams was only available in Germany at GSI, and now at HIT. With this active rasterscanning technique developed by Haberer et al., excellent dose distributions as shown in the clinical examples within this manuscript and precise dose delivery can be generated [Citation13]. This technique, based on the developments of GSI, could be implemented successfully at the Heidelberg center and is shown to be highly reliable for daily clinical routine. Clinical studies of all phases will be performed and patients can be recruited effectively. Based on preclinical results, the role of carbon ion radiotherapy will be evaluated for primary and recurrent malignant gliomas (CLEOPATRA-Trial and CINDERELLA-Trial), hepatocellular caricinomas (PROMETHEUS-01 Trial) and recurrent rectal cancer. Additionally, the unique capacity of HIT to offer protons and carbon ions allow direct comparison of both ions for chordomas and chondrosarcomas of the skull base. For several indications, radiochemotherapy is an established treatment standard. Therefore, for example in head-and-neck tumors, combination treatment of particle therapy and chemotherapy will be performed.
Within the Department of Radiation Oncology, daily availability of particle beams was integrated successfully into the clinical routine, mainly based on the experienced team of radiation oncologist, radiotherapists as well as medical physicist. Workflow from the existing department of the HIT facility was well established and patients are recruited for particle therapy from the dedicated clinics held by highly specialized radiation oncologist. Patients can be treated as out- or inpatients on three dedicated radiation oncology wards. Due to this structure, indications for treatment can be set according to the individual need of the patient and the required technique can be chosen.
At the HIT center, treatment started in one treatment room with the horizontal beamline. The second horizontal beam line as well as the carbon ion gantry will follow in due course. Overall, the technical equipment was proven to be very reliable over the last months. The in-room equipment including the robotic patient table as well as the robotic imaging system can be handled easily by the treatment team and has shown good functionality. In a next step, in room imaging will be performed by a cone beam CT. Overall treatment time still remains to be around 30 minutes including patient positioning and imaging, the actual radiation time takes up to about 50–70% of this time. Due to the rigid and reproducible fixation especially in patients with base of skull tumors, only one imaging series prior to each fraction is sufficient. For tumors of the abdomen, an additional imaging is performed prior to each treatment portal to possibly correct any positioning accuracy that might occur during the longer treatment times.
Within a close cooperation with manufacturers of medical devices, many of the developments achieved within the pilot project at GSI could be transferred into CE labelled medical products, like the biologically optimized treatment planning, the active raster scanning technique, the monitoring system as well as the control and safety software. In doing so, the experimental setting of the pilot project based at a research institution (GSI) could be successfully translated into a clinical hospital based setting. This enables us to address the important clinical questions about the role of proton and heavy ion beam therapy in clinical oncology in a much more efficient way.
The important next steps include the extension of the treatments to the second horizontal beam treatment room within 2010 and the start of the gantry room within 2011 along with an increase of the treatment time per day as well as the overall patient numbers.
In conclusion, it has been shown that ion beam therapy can be successfully translated from a research setting into a hospital based environment. The experience gained in the pilot project at GSI, however, has been a very important prerequisite in the development of techniques needed for a broader use of heavy ion beam therapy. The possibility to offer carbon ion radiotherapy has opened a new horizon and permits a whole new field of opportunities. HIT is now continuously in operation and patient numbers will be increased continuously.
Declaration of interest: The authors report no conflicts of interest. The authors alone are responsible for the content and writing of the paper.
References
- Combs SE, Jakel O, Haberer T, Debus J. Particle therapy at the Heidelberg Ion Therapy Center (HIT) – Integrated research-driven university-hospital-based radiation oncology service in Heidelberg, Germany. Radiother Oncol 2010;95: 41–4.
- Schulz-Ertner D, Didinger B, Nikoghosyan A, Jakel O, Zuna I, Wannenmacher M, . Optimization of radiation therapy for locally advanced adenoid cystic carcinomas with infiltration of the skull base using photon intensity-modulated radiation therapy (IMRT) and a carbon ion boost. Strahlenther Onkol 2003;179:345–51.
- Schulz-Ertner D, Nikoghosyan A, Hof H, Didinger B, Combs SE, Jakel O, . Carbon ion radiotherapy of skull base chondrosarcomas. Int J Radiat Oncol Biol Phys 2007; 67:171–7.
- Schulz-Ertner D, Karger CP, Feuerhake A, Nikoghosyan A, Combs SE, Jakel O, . Effectiveness of carbon ion radiotherapy in the treatment of skull-base chordomas. Int J Radiat Oncol Biol Phys 2007;68:449–57.
- Combs SE, Nikoghosyan A, Jaekel O, Karger CP, Haberer T, Munter MW, . Carbon ion radiotherapy for pediatric patients and young adults treated for tumors of the skull base. Cancer 2009;115:1348–55.
- Combs SE, Hartmann C, Nikoghosyan A, Jakel O, Karger CP, Haberer T, . Carbon ion radiation therapy for high-risk meningiomas. Radiother Oncol 2010;95:54–9.
- Scholz M, Matsufuji N, Kanai T. Test of the local effect model using clinical data: Tumour control probability for lung tumours after treatment with carbon ion beams. Radiat Prot Dosimetry 2006;122:478–9.
- Elsasser T, Kramer M, Scholz M. Accuracy of the local effect model for the prediction of biologic effects of carbon ion beams in vitro and in vivo. Int J Radiat Oncol Biol Phys 2008; 71:866–72.
- Jakel O, Schulz-Ertner D, Debus J. Specifying carbon ion doses for radiotherapy: The Heidelberg approach. J Radiat Res (Tokyo) 2007;48(Suppl A):A87–95.
- Kramer M, Jakel O, Haberer T, Rietzel E, Schardt D, Scholz M, . Treatment planning for scanned ion beams. Radiother Oncol 2004;73(Suppl 2):S80–5.
- Jakel O, Debus J, Kramer M, Scholz M, Kraft G. Treatment planning for light ions: How to take into account relative biological effectiveness (RBE). Strahlenther Onkol 1999; 175(Suppl 2):12–4.
- Haberer T, Debus J, Eickhoff H, Jakel O, Schulz-Ertner D, Weber U. The Heidelberg Ion Therapy Center. Radiother Oncol 2004;73(Suppl 2):S186–90.
- Haberer T, Becher W, Schardt D, Kraft G. Magnetic scanning system for heavy ion therapy. Nucl Instrument Meth Phys Res 1993;A330:296–305.
- Schulz-Ertner D, Karger CP, Feuerhake A, Nikoghosyan A, Combs SE, Jakel O, . Effectiveness of carbon ion radiotherapy in the treatment of skull-base chordomas. Int J Radiat Oncol Biol Phys 2007;68:449–57.
- Suit H, Goldberg S, Niemierko A, Trofimov A, Adams J, Paganetti H, . Proton beams to replace photon beams in radical dose treatments. Acta Oncol 2003;42:800–8.
- Suit H, Kooy H, Trofimov A, Farr J, Munzenrider J, DeLaney T, . Should positive phase III clinical trial data be required before proton beam therapy is more widely adopted? No. Radiother Oncol 2008;86:148–53.
- Suit H, DeLaney T, Goldberg S, Paganetti H, Clasie B, Gerweck L, . Proton vs carbon ion beams in the definitive radiation treatment of cancer patients. Radiother Oncol 2010;95:3–22.
- Pommier P, Lievens Y, Feschet F, Borras JM, Baron MH, Shtiliyanova A, . Simulating demand for innovative radiotherapies: An illustrative model based on carbon ion and proton radiotherapy. Radiother Oncol 2010.
- Pijls-Johannesma M, van Mastrigt G, Hahn SM, De Ruysscher D, Baumert BG, Lammering G, . A systematic methodology review of phase I radiation dose escalation trials. Radiother Oncol 2010;95:135–41.
- Peeters A, Grutters JP, Pijls-Johannesma M, Reimoser S, De Ruysscher D, Severens JL, . How costly is particle therapy? Cost analysis of external beam radiotherapy with carbon-ions, protons and photons. Radiother Oncol 2010;95: 45–53.
- Pijls-Johannesma M, Grutters JP, Verhaegen F, Lambin P, De Ruysscher D. Do we have enough evidence to implement particle therapy as standard treatment in lung cancer? A systematic literature review. Oncologist 2010;15:93–103.
- Schulz-Ertner D, Tsujii H. Particle radiation therapy using proton and heavier ion beams. J Clin Oncol 2007;25: 953–64.