Abstract
Purpose/Objective. Daily Cone-beam computed tomography (CBCT) in room imaging is used to determine tumour shrinkage during a full radiotherapy (RT) course. In addition, relative interfractional tumour and lymph node motion is determined for each RT fraction. Material and methods. From November 2009 to March 2010, 20 consecutive lung cancer patients (14 NSCLC, 6 SCLC) were followed with daily CBCT during RT. The gross tumour volume for lung tumour (GTV-t) was visible in all daily CBCT scans and was delineated at the beginning, at the tenth and the 20th fraction, and at the end of treatment. Whenever visible, the gross tumour volume for lymph nodes (GTV-n) was also delineated. The GTV-t and GTV-n volumes were determined. All patients were setup according to an online bony anatomy match. Retrospectively, matching based on the internal target volume (ITV), the GTV-t or the GTV-n was performed. Results. In eight patients, we observed a significant GTV-t shrinkage (15–40%) from the planning CT until the last CBCT. Only five patients presented a significant shrinkage (21–37%) in the GTV-n. Using the daily CBCT imaging, it was found that the mean value of the difference between a setup using the skin tattoo and an online matching using the ITV was 7.3±2.9 mm (3D vector in the direction of ITV). The mean difference between the ITV and bony anatomy matching was 3.0±1.3 mm. Finally, the mean distance between the GTV-t and the GTV-N was 2.9±1.6 mm. Conclusion. One third of all patients with lung cancer undergoing chemo-RT achieved significant tumour shrinkage from planning CT until the end of the radiotherapy. Differences in GTV-t and GTV-n motion was observed and matching using the ITV including both GTV-t and GTV-n is therefore preferable.
Locally advanced non-small-cell lung cancer (NSCLC) and limited disease small-cell lung cancer (LD-SCLC) can be cured with chemo-radiotherapy. Unfortunately it is usually associated with high local failure rate depending on the total dose of radiotherapy. However large treatment volumes including lung tumour and metastatic lymph nodes in the mediastinum make it difficult to plan and deliver high dose radiotherapy without harming the surrounding normal tissue. Especially severe and potentially fatal lung toxicity increases with higher total dose and larger volumes [Citation1,Citation2].
Lung tumour regression during chemo-radiotherapy has previously been reported. Juhler-Nøttrup et al. [Citation3] found a significant lung tumour and lymph node shrinkage during an entire RT course of 19% and 34%, respectively. However the pattern of tumour regression is very heterogeneous. In some patients a very large tumour change is observed and in others there is no change during an entire RT course [Citation4].
Daily CBCT image guidance and remote-controlled couch is essential to confirm the high geometric accuracy required for lung radiotherapy. It is significantly reducing both random and systematic positional errors for cancer patients and thereby avoiding geometrical miss of radiation therapy [Citation5–7]. Yeung et al. [Citation8] showed that soft-tissue registration to localise tumour in conventionally fractionated RT reduced the required setup margin compared to image guidance using bony anatomy. However motion analysis of mediastinal nodes and tumour in lung cancer patients showed no association between 3D primary tumour motion and node motion [Citation9]. Therefore adaptive strategies based solely on primary tumour may be misleading in locally advanced lung cancer.
Cone-beam computed tomography is a useful image-guidance technique for lung tumour because it provides good visualisation of the target and relatively high resolution soft-tissue information. At Aarhus University Hospital daily CBCT has been used for the setup of all conventionally fractionated lung cancer patients for the past six months. These novel data sets provide new clinical possibilities and the first aim in this study was therefore to determine tumour shrinkage during a RT course using CBCT.
The second aim in this study was to analyse GTV-t and GTV-n motion during a RT course using CBCT and determine the best way of matching. Four setup strategies were evaluated; no image guidance, bony anatomy matching, ITV matching and GTV-t/GVT-n matching.
Material and methods
Patients
A total of 20 lung cancer patients were treated with radical chemo-radiotherapy between November 2009 and March 2010. Fourteen of the patients were treated for NSCLC with three to four cycles of cisplatin or carboplatin and vinorelbine concomitant with 60–66 Gy in 30–33 fractions (F), 5 F/week. Six patients were treated for SCLC with four cycles of cisplatin or carboplatin and etoposide concomitant with RT. Four SCLC patients were treated with 45 Gy in 30 F, 10 F/week and two SCLC patients were treated 50 Gy in 25 F, 5 F/week. The median GTV-t volume was 35 cm3 (range 5–223 cm3) and the median GTV-n volume was 17 cm3 (range 2–118 cm3).
CT scanning
Patients underwent free breathing scanning in the supine position on a narrow cone beam helical CT scanner (Philips LB16, Philips Healthcare, Best, The Netherlands) with 3 mm slice spacing and pixel size of 1 × 1 mm2. The median time interval from the planning CT until start of RT was 14 days (range 9–20). The patients were reproducibly fixated with both arms above the head in an individual immobilisation device. The isocentre was marked on the patients with three skin tattoos and the scan range covered the complete thoracic region. Intravenous contrast was supplied 15 s before the scan.
Radiotherapy planning
All CT scans were transferred to the Eclipse treatment planning system (Varian Medical Systems, Palo Alto, CA, USA) and the gross tumour volumes of the primary tumour (GTV-t) were delineated. In 17 patients gross tumour volumes for malign lymph nodes (GTV-n) were delineated separately. One patient did only show primary tumour and in two patients GTV-t and GTV-n could not be separated. The GTV-t and GTV-n volumes were expanded to a clinical target volume (CTV) by adding 5 mm margin to the GTV. Areas of overlap with large vessels, bones and the opposite lung where subtracted. The internal target volume (ITV) was created by adding 5 mm margin to the CTV left-right (LR) and anterior-posterior (AP) and 5 to 10 mm superior-inferior (SI). A uniform 5 mm margin was added to the ITV to generate a planning target volume (PTV) that accounted for setup uncertainty.
After contouring the target and normal structures, a 3D conformal or intensity-modulated treatment plan was created, using four to six coplanar 6 MV photon beams. Inhomogeneity corrections were taken into account by the AAA algorithm. The plans were designed so that the PTV received at least 95% of the prescribed dose and the global maximum was below 107% of the prescribed dose.
Patient setup and in-room imaging
For every treatment session, the patients were aligned according to the skin tattoos by use of the in-room laser system. Thereafter, a kV cone beam CT (CBCT) scan [Citation10] was acquired with the gantry-mounted on-board imager (OBI) (Varian Medical Systems, Palo Alto, CA, USA) [Citation11]. The axial field of view was 16 cm in SI direction, which normally was sufficient to cover the full target. The CBCT scan was rigidly registered to the planning CT scan. An automatic registration of the bony anatomy was performed using a user defined region of interest (ROI) including the spinal cord. The registration was evaluated by the radiation therapists and the resulting translational shifts in three dimensions were effectuated.
Retrospective tumour volume determination
Selected CBCT scans were transferred to the treatment planning system for contouring of the tumour volumes. The GTV-t was visible at the CBCT scans for all patients. In 16 patients, GTV-t was delineated at the first CBCT scan, approximately at the tenth and the 20th scan, and at the last scan. For the remaining patients orthogonal OBI images were used as the daily imaging technique in the beginning of the treatment course. For these patients GTV-t was delineated at the first CBCT scan and at the last CBCT scan. The GTV-n was visible at the CBCT scans for 11 patients and was delineated at the same scans as the GTV-t. To avoid inter-observer variations [Citation12], the same physician performed all contouring. Intra-observer variations were minimised by copying the outlined structure from the CT scan to the selected CBCT scans, followed by a modification to reflect the variations observed. The tumour and lymph node volumes were measured for all delineated structured. The tumour volume measured at the CT scan was used as reference and the volumetric change was determined as a percentage of the CT volume for each of the contoured CBCT scans.
Retrospective CBCT registration
A total of 519 CBCT scans were obtained for the 20 patients. Four patients did not have CBCT scans at the beginning of the radiotherapy course, as they were setup according to daily orthogonal OBI images. For these patients the imaging technique was changed from daily OBI images to daily CBCT images during their treatment course. The number of acquired CBCT scans ranged from four to 33, with a median value of 29. All CBCT scans were retrospectively registered to the planning CT scans using soft tissue registration with only translational displacements being accounted for. Three different automatic soft tissue registration methods were applied: A registration including only GTV-t plus a uniform margin of 5 mm, a registration including only GTV-n (when existing) plus a uniform margin of 5 mm, and a registration including the ITV. For each registration method, the ROI was chosen to include only the GTV-t, the GTV-n and the ITV, respectively. After each automatic registration, a visual inspection of the alignment of the planning CT and the CBCT scans was performed. A proper alignment was found in all cases except for one patient where an atelectacis was markedly reduced compared to the planning CT and thus, made the inspection very difficult. This patient was rescanned and replanned after 11 fractions and thereafter, the alignment was considered acceptable.
Calculation of errors and margins
The translational couch shifts for the online and the offline registrations were collected for each patient for all CBCT scans. For the online registrations, the couch shifts were a measure of the difference between setting up using skin tattoos and setting up using the online bony registrations. For the offline soft tissue registrations using ITV, the couch shifts were calculated as the difference between the online couch shifts and the offline shifts in SI, AP, and LR direction, respectively. Finally, the couch shifts obtained using the GTV-t registrations were subtracted from the couch shifts obtained using the GTV-n registrations for each direction.
Thus, three sets of couch shifts were calculated for each patient.
The mean value, the standard deviation (SD) and the maximum deviation was calculated for each patient for each of the three datasets. From these data, the SD of the random error as calculated from the SDs of the individual patients, σ, the SD of the systematic error as given by the means per patient, Σ, and the overall mean error, M were determined [Citation13]. The random and systematic errors were used to calculate the PTV margin according to the formula: 2.5Σ + 0.7σ [Citation13]. This formula applies only to the mediastinal part of the field. In the lung tissue, a larger penumbra should be taken into account [Citation14] giving rise to a slightly smaller PTV margin. As most of targets in our patients involve both lung tumour and mediastinal lymph nodes, we have chosen to use the above mentioned formula.
The couch position obtained from the offline ITV registration was used as the reference position. From this, the PTV margins necessary for setup according to bony anatomy and skin tattoos respectively, were calculated.
The error and margin calculations were performed separately in the SI, AP, and LR direction. Furthermore, the errors and margins were calculated for the 3D vector.
Results
Tumour volume determination
The tumour volume as determined from the planning CT scan and the CBCT scans was plotted as a function of the number of treatment fractions. Tumour (GTV-t) shrinkage of more than 15% was observed in eight patients. The change in tumour volume as measured at the CBCT scans relative to the CT scan is shown in for these patients. Seven of these patients were treated for NSCLC and one patient was treated for SCLC. Minor tumour shrinkage of 5–15% was observed in six patients (three NSCLC, three SCLC). For the remaining patients no shrinkage or a minor growth was observed. The tumour volumes of these patients were shown in . Most of the lymph nodes (GTV-n) showed no or little shrinkage, with only five patients (four NSCLC, one SCLC) undergoing tumour shrinkage of more than 15% (See ). No correlation between tumour volume and shrinkage was found.
Setup by use of different match strategies
The setup error obtained by aligning to skin tattoos without image guidance in comparison to the soft tissue matching using the ITV was calculated in the SI, AP and LR directions for all CBCT scans. The mean value and standard deviation of the setup error was 2.4±4.0 mm, 1.7±2.5 mm, and 0.4±3.0 mm in the SI, AP, and LR direction, respectively. The shift is positive in the cranial, vertical and left direction. The setup error varied substantially between the patients. In one patient, a mean value of approximately 6±3 mm was found, whereas one of the other patients showed a mean value of approximately 0±2 mm. The number of errors ≥ 5 mm was 44%, 21%, and 18% in the SI, AP and LR directions, respectively. Considering errors ≥ 10 mm the numbers was 9%, 6% and 1% and the maximum errors were 16 mm, 14 mm, and 12 mm, respectively. Thus, in the SI direction, the frequency of errors larger than 5 mm is more frequent than in the other directions.
In the 3D vector of the setup error is shown for all the 519 CBCT scans. The mean value was 7.3±2.9 mm (maximum 20 mm). The overall mean, M, the systematic and the random errors for setting up using skin tattoos were shown in . The errors were approximately 3 mm in all directions. The systematic and the random errors were comparable. From these values the PTV margins in the SI, AP and LR directions were calculated and listed in the table, too. The margins were found to be 12.3 mm; 8.4 mm; and 9.3 mm; in the SI, AP and LR directions, respectively. These margins did not account for tumour position variation resulting from respiratory motion of the tumour and lymph nodes.
Figure 3. Distribution of the 3D shifts from the skin tattoos to the soft tissue matching using the ITV.
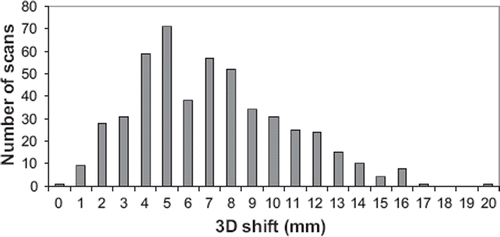
Table I. Setup errors obtained by a. aligning to skin tattoos without image guidance and b. bony anatomy matching both in comparison to the soft tissue matching using the ITV. Values are given for the overall mean, M, the systematic errors, Σ, the random errors, σ, and calculated margins.
The setup error introduced by matching on the bony anatomy compared to a soft tissue match using the ITV was calculated in the SI, AP and LR directions for all CBCT scans. The mean value and standard deviation of the setup error was 0.3±1.8 mm, 0.1±1.8 mm, and 0.4±1.4 mm in the SI, AP, and LR direction, respectively. The maximum errors were 9 mm, 5 mm, and 6 mm, respectively. The frequency of errors ≥ 5 mm was 7%, 1%, and 2% in the SI, AP, and LR direction, respectively. These errors were substantially smaller than the errors obtained by using skin tattoos. The overall mean, the systematic and the random errors were listed in along with the PTV margins. The PTV margins were seen to be reduced by more than a factor of two by using daily on line bony anatomy matching instead of no image guidance.
The 3D vector of the setup error is shown in . The mean value was 3.0±1.3 mm and the maximum deviation was 9.1 mm.
Figure 4. Distribution of the 3D shifts from the bony anatomy match to the soft tissue match using the ITV.
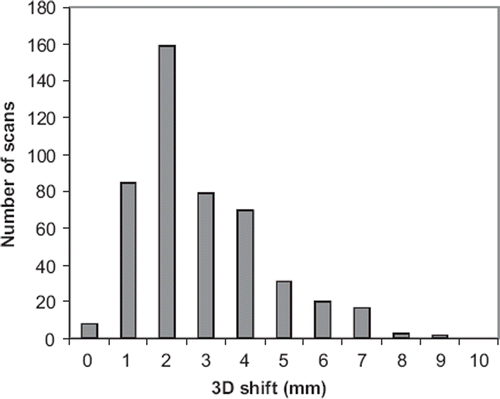
The differences in registrations performed on the GTV-t and performed on the GTV-n were plotted in for the SI, AP, and LR directions and for the 3D vector for all CBCT scans. The mean values were −0.2±2.0; −0.3±1.4 mm; and 0.0±1.4 mm in the SI, AP, and LR directions, respectively, with maximum deviations of 10 mm, 5 mm and 6 mm, respectively. The mean values varied substantially, with one patient showing mean values of 5 mm, 2 mm and 2 mm in the SI, AP, and LR directions, and another patient showing mean values of maximum 1 mm in all three directions. Therefore, in some patients rather large errors may be introduced if GTV-t is used for the online matching. The frequency of errors ≥ 5 mm was 5%, 1%, and 2% in the SI, AP, and LR directions, respectively. These errors were of the same magnitude as the errors introduced by using bone match compared to a soft tissue ITV match. The largest deviations are seen in the SI direction, which is normally direction of the largest tumour motion [Citation9].
Figure 5. Distribution of the distance between GTV-t and GTV-n for the SI, AP, and LR directions and for the 3D vector for all CBCT scans.
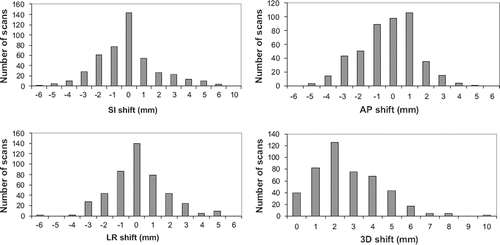
The 3D vector of the setup error is shown in . The mean value was 2.9±1.6 mm and the maximum deviation was 10.0 mm.
Discussion
The present study showed that approximately one third of all patients with lung cancer undergoing chemo-RT achieved significant tumour shrinkage from planning CT until the end of RT.
Tumour shrinkage has been studied earlier using various imaging modalities, such as electronic portal imaging, repetitive CT scanning or PET-CT scanning. The regression rates per day during an RT course reported in these studies vary between 0.6% and 2.4% [Citation14,Citation15].
Juhler-Nøttrup et al. [Citation3] analysed ten patients with locally advanced NSCLC and found a significant lung tumour reduction during an entire RT course of 19% for lung tumour and 34% for lymph node.
Woodford et al. [Citation16] analysed 17 patients with NSCLC and divided them into three groups. Group A experienced an initial period of a small tumour volume change, followed with a sharp decrease. Group B had a more gradual linear decreasing GTV and Group C experienced no clear trend toward a decrease in tumour volume. Other studies has found constant rate of decrease throughout the treatments, with no obvious initial or final plateaus. The present study did not find an obvious plateau. All other authors describing tumour shrinkage find some patients with a very large tumour change and some with minor or no change. Variations reported ranged from 12–87%. One study [Citation17] showed the lowest rate of shrinkage for the smallest lesions, and the highest rate in the largest lesions. Our study did not support that result, and we did not find a difference in tumour regression in patients with SCLC compared to NSCLC. So far it is difficult to predict the changes in GTV based on patient or tumour characteristics.
In the present study daily CBCT scans were used to localise the target for all treatment fractions. In a study of Nelson [Citation18] it was found that target localisation is more important than 4D respiratory gating, emphasising the importance of image guidance.
The CBCT images may be used for automatic image registration either using the bony anatomy as a surrogate for the position of tumour and lymph nodes or by use of a soft tissue match using the actual tumour and lymph nodes contoured on the planning CT. At the start up of daily CBCT imaging, we decided to use bony anatomy matching. This match is easily performed by the radiation therapists at the accelerator. A retrospective analysis of the data, showed nearly perfect bony anatomy matching for all 519 scans making this match technique very reliable.
In the present study, we presume that matching using the ITV as contoured at the planning CT yields the optimal match. We compared the soft tissue ITV match to a setup using skin tattoos without image guidance. We have shown that errors ≥ 5 mm occurs in the SI direction for nearly 50% of the patients. Thus daily image guidance may be used to shrink the margins. We found a margin reduction of approximately 10 mm. These findings are comparable to the findings of other groups [Citation5,Citation8,Citation19–21].
Considerable systematic tumour baseline shifts have been observed in lung cancer patients. In case of node-negative patients, several studies have shown that CBCT image-guided corrections aligning the time averaged mean target position can reduce the required PTV margins in patients treated with SBRT [Citation20]. In case of involved lymph nodes, however, differential baseline shifts between primary tumour and lymph nodes invalidates the use of couch shifts to reduce these errors. In the present study we evaluated the error achieved in the position of GTV-n by matching to GTV-t and found that in some patients rather large errors was calculated. The mean 3D vector was 2.9 mm with a maximum deviation of 10 mm. Similarly Elmpt et al. [Citation22] investigated the positional changes in primary tumour and involved lymph nodes during chemo-RT I 35 patients with NSCLC. PET-CT was performed just before and in the second week of RT. They found that in individual cases, large displacement up to 1.6 cm of primary tumour may occur that was not correlated to the lymph nodes. They concluded that position change of the primary tumour was not always predictive for lymph node change. Pantarotto et al. [Citation9] retrospectively analysed patterns of nodal and tumour motion in 41 patients with lung cancer in the 4D-planning CTs. They found that the average 3D nodal motion during quiet breathing was 0.68 cm (range, 0.17–1.64 cm) and found no association to the 3D primary tumour motion.
The data therefore support that adaptive strategies based solely on the primary tumour may be misleading and imaging of the mediastinal lymph nodes may be necessary.
Based on these findings, we used the ITV including both the GTV-t and the GTV-n structures as the reference structure for the soft tissue matching. A comparison of the soft tissue ITV matching to a bony anatomy matching showed systematic and random errors of 1 to 2 mm. From these findings a margin of approximately 4 mm (range 3.5 to 5.6 mm) was found to account for the lack of precision by using the bony anatomy match. That is, a margin reduction of 4 mm could be obtained by using soft tissue ITV matching. Only one group [Citation8] has reported on the difference between ITV and bony anatomy matching. The margin found by this group was in the range 5 to 14 mm, i.e. considerably larger. These findings are in agreement with the findings of some other groups reporting on the difference between a GTV-t and a bony anatomy match [Citation5,Citation19,Citation20]. However, matching using the GTV-t is advisable only for patients showing no malignant lymph nodes. Some lymph nodes are positioned close to the spinal cord making the deviation between the ITV and the bony anatomy match much less than for the GTV-t match. Therefore, findings of larger margins are expected when the bony anatomy match is compared to the GTV-t match. In the study of Yeung [Citation8], no description of the number of patients showing malign lymph nodes neither the position of the lymph nodes is given, which makes it difficult to make a comparison to the present study.
Matching of large targets is seldom perfect for all parts of the target, and deviations are normally seen in the other part of the target. So the margins found only apply for the central part of the target. The accepted deviation of the target as visualised on the CBCT scan compared to the planning CT scan must be defined in the match protocol. We defined a maximum deviation of 5 mm. For deviations larger than this, the patient was refixated and a new match was performed.
In one patient an atelectasis was seen to disappear after two weeks of radiation. The anatomy of the patient changed considerably, which was seen at the daily CBCT scanning and the patient was rescanned and replanned in order to deliver an optimal dose distribution. Therefore daily CBCT scanning may also be used to determine which patients may benefit of a rescanning due to anatomical changes [Citation23].
Reduction of margins is important for the treatment of NSCLC patients, as an escalation of the dose to the tumour for many patients is not possible due to a large treated volume of the lungs. Studies have shown that the mean lung dose may be reduced considerably (30–40%) by the implementation of image guidance [Citation24,Citation25] thus enabling the possibility of dose escalation.
In some patients, shrinkage of the GTV-t implies shifts in the tumour localisation. This is seen in the daily online CBCT registrations using GTV-t for registration. In one patient linear regression using the daily registrations showed shifts of 0.15 mm in the AP and SI directions. That is a shift of 5 mm from the beginning to the end of the RT course. This shift is not seen for the GTV-t, making the ITV match less correct. In other patients the shrinkage is approximately symmetrical in all directions, implying no shift in the GTV-t position. Thus, in patients with large shrinkage and shift of GTV-t it may be ideal to rescan the patients in order to create the optimal treatment plan.
Conclusion
Significant tumour shrinkage as determined from planning CT until the end of the radiotherapy was observed for one third of all patients with lung cancer undergoing chemo-RT. Daily online CBCT matching is essential for target localisation in lung cancer patients. Matching using an ITV including both GTV-t and GTV-n is preferable. The use of the GTV-t as a surrogate for GTV-t and GTV-n introduces errors in the target positioning in the order of 3 mm. A combination of adaptive planning due to tumour shrinkage and daily image guidance used for margin reduction may enable dose escalation for selected patients.
Acknowledgements
The authors acknowledge Ulrik Vindelev Elstrøm for sharing his knowledge about CBCT and Ludvig Muren for contributing to the preparation of the manuscript.
Declaration of interest: The authors report no conflicts of interest. The authors alone are responsible for the content and writing of the paper.
References
- Jin H, Tucker SL, Liu HH, Wei X, Yom SS, Wang S, . Dose-volume thresholds and smoking status for the risk of treatment-related pneumonitis in inoperable non-small cell lung cancer treated with definitive radiotherapy. Radiother Oncol 2009;91:427–32.
- Jassem J. The role of radiotherapy in lung cancer: Where is the evidence? Radiother Oncol 2007;83:203–13.
- Juhler-Nottrup T, Korreman SS, Pedersen AN, Persson GF, Aarup LR, Nystrom H, . Interfractional changes in tumour volume and position during entire radiotherapy courses for lung cancer with respiratory gating and image guidance. Acta Oncol 2008;47:1406–13.
- Fox J, Ford E, Redmond K, Zhou J, Wong J, Song DY. Quantification of tumor volume changes during radiotherapy for non-small-cell lung cancer. Int J Radiat Oncol Biol Phys 2009;74:341–8.
- Bissonnette JP, Purdie TG, Higgins JA, Li W, Bezjak A. Cone-beam computed tomographic image guidance for lung cancer radiation therapy. Int J Radiat Oncol Biol Phys 2009;73: 927–34.
- Yamashita H, Haga A, Hayakawa Y, Okuma K, Yoda K, Okano Y, . Patient set up error and day-to-day esophageal motion error analyzed by cone-beam computed tomography in radiation therapy. Acta Oncol 2010;49: 485–90.
- Nielsen M, Bertelsen A, Westberg J, Jensen HR, Brink C. Cone beam CT evaluation of patient set up accuracy as a QA tool. Acta Oncol 2009;48:271–6.
- Yeung AR, Li JG, Shi W, Newlin HE, Chvetsov A, Liu C, . Tumor localization using cone-beam CT reduces set up margins in conventionally fractionated radiotherapy for lung tumors. Int J Radiat Oncol Biol Phys 2009;74:1100–7.
- Pantarotto JR, Piet AH, Vincent A, van Sornsen de Koste JR, Senan S. Motion analysis of 100 mediastinal lymph nodes: Potential pitfalls in treatment planning and adaptive strategies. Int J Radiat Oncol Biol Phys 2009;74: 1092–9.
- Pouliot J. Megavoltage imaging, megavoltage cone beam CT and dose-guided radiation therapy. Front Radiat Ther Oncol 2007;40:132–42.
- Sorcini B, Tilikidis A. Clinical application of image-guided radiotherapy, IGRT (on the Varian OBI platform). Cancer Radiother 2006;10:252–7.
- Vorwerk H, Beckmann G, Bremer M, Degen M, Dietl B, Fietkau R, . The delineation of target volumes for radiotherapy of lung cancer patients. Radiother Oncol 2009;91: 455–60.
- van HM, Remeijer P, Rasch C, Lebesque JV. The probability of correct target dosage: Dose-population histograms for deriving treatment margins in radiotherapy. Int J Radiat Oncol Biol Phys 2000;47:1121–35.
- Sonke JJ, Belderbos J. Adaptive radiotherapy for lung cancer. Semin Radiat Oncol 2010;20:94–106.
- Bral S, Duchateau M, De RM, Everaert H, Tournel K, Schallier D, . Volumetric response analysis during chemoradiation as predictive tool for optimizing treatment strategy in locally advanced unresectable NSCLC. Radiother Oncol 2009;91:438–42.
- Woodford C, Yartsev S, Dar AR, Bauman G, Van DJ. Adaptive radiotherapy planning on decreasing gross tumor volumes as seen on megavoltage computed tomography images. Int J Radiat Oncol Biol Phys 2007;69:1316–22.
- Kupelian PA, Ramsey C, Meeks SL, Willoughby TR, Forbes A, Wagner TH, . Serial megavoltage CT imaging during external beam radiotherapy for non-small-cell lung cancer: Observations on tumor regression during treatment. Int J Radiat Oncol Biol Phys 2005;63:1024–8.
- Nelson C, Starkschall G, Balter P, Morice RC, Stevens CW, Chang JY. Assessment of lung tumor motion and set up uncertainties using implanted fiducials. Int J Radiat Oncol Biol Phys 2007;67:915–23.
- Ikushima H, Balter P, Komaki R, Hunjun S, Bucci MK, Liao Z, . Daily alignment results of in-room computed tomography-guided stereotactic body radiation therapy for lung cancer. Int J Radiat Oncol Biol Phys 2010.
- Purdie TG, Bissonnette JP, Franks K, Bezjak A, Payne D, Sie F, . Cone-beam computed tomography for on-line image guidance of lung stereotactic radiotherapy: Localization, verification, and intrafraction tumor position. Int J Radiat Oncol Biol Phys 2007;68:243–52.
- Guckenberger M, Meyer J, Wilbert J, Baier K, Mueller G, Wulf J, . Cone-beam CT based image-guidance for extracranial stereotactic radiotherapy of intrapulmonary tumors. Acta Oncol 2006;45:897–906.
- van EW, Ollers M, van HH, den HL, Vercoulen L, Wouters M, . Volume or position changes of primary lung tumor during (chemo-)radiotherapy cannot be used as a surrogate for mediastinal lymph node changes: The case for optimal mediastinal lymph node imaging during radiotherapy. Int J Radiat Oncol Biol Phys 2010.
- Tanyi JA, Fuss MH. Volumetric image-guidance: Does routine usage prompt adaptive re-planning? An institutional review. Acta Oncol 2008;47:1444–53.
- Grills IS, Yan D, Martinez AA, Vicini FA, Wong JW, Kestin LL. Potential for reduced toxicity and dose escalation in the treatment of inoperable non-small-cell lung cancer: A comparison of intensity-modulated radiation therapy (IMRT), 3D conformal radiation, and elective nodal irradiation. Int J Radiat Oncol Biol Phys 2003;57: 875–90.
- Harsolia A, Hugo GD, Kestin LL, Grills IS, Yan D. Dosimetric advantages of four-dimensional adaptive image-guided radiotherapy for lung tumors using online cone-beam computed tomography. Int J Radiat Oncol Biol Phys 2008;70: 582–9.