Abstract
Purpose. Dose painting strategies are limited by optimization algorithms in treatment planning systems and physical constraints of the beam delivery. We investigate dose conformity using the RapidArc optimizer and beam delivery technique. Furthermore, robustness of the plans with respect to positioning uncertainties are evaluated. Methods. A head & neck cancer patient underwent a [61Cu]Cu-ATSM PET/CT-scan. PET-SUVs were converted to prescribed dose with a base dose of 60 Gy, and target mean dose 90 Gy. The voxel-based prescription was converted into 3, 5, 7, 9, and 11 discrete prescription levels. Optimization was performed in Eclipse, varying the following parameters: MLC leaf width (5 mm and 2.5 mm), number of arcs (1 and 2) and collimator rotation (0, 15, 30 and 45 degrees). Dose conformity was evaluated using quality volume histograms (QVHs), and relative volumes receiving within ±5% of prescribed dose (Q0.95–1.05). Deliverability was tested using a Delta4® phantom. Robustness was tested by shifting the isocenter 1 mm and 2 mm in all directions, and recalculating the dose. Results. Good conformity was obtained using MLC leaf width 2.5 mm, two arcs, and collimators 45/315 degrees, with Q0.95–1.05=92.8%, 91.6%, 89.7% and 84.6%. Using only one arc or increasing the MLC leaf width had a small deteriorating effect of 2–5%. Small changes in collimator angle gave small changes, but large changes in collimator angle gave a larger decrease in plan conformity; for angles of 15 and 0 degrees (two arcs, 2.5 mm leaf width), Q0.95–1.05 decreased by up to 15%. Consistency between planned and delivered dose was good, with ∼90% of gamma values <1. For 1 mm shift, Q0.95–1.05 was decreased by 5–15%, while for 2 mm shift, Q0.95–1.05 was decreased to 55–60%. Conclusions. Results demonstrate feasibility of planning of prescription doses with multiple levels for dose painting using RapidArc, and plans were deliverable. Robustness to positional error was low.
For a given integral dose, it can be shown theoretically that a uniform radiation dose distribution optimizes the probability of controlling a tumor mass with a homogenous radiosensitivity [Citation1–3]. However, far from being homogenous, human tumors are characterized by large variability in micro-environment and cellular characteristics. More than 20 years ago, Brahme and Ågren [Citation4] used mathematical modelling to show that an appropriately shaped non-uniform dose-distribution could optimize the tumor control probability for a tumor with spatial variation in radiosensitivity. Advances in molecular and functional imaging are making non-invasive mapping of spatial variations in radioresponsiveness increasingly realistic. This was the background for a visionary paper published in 2000 by Ling et al. [Citation5] who proposed that molecular imaging could be used to define a discrete subvolume that would benefit from a radiation boost dose, a concept demonstrated in a case study of a hypoxia surrogate scan by Chao et al. [Citation6] the following year. A generalization of this concept, voxel-based dose prescription using a mathematical prescription function or so-called dose painting by numbers, was proposed by Bentzen in 2005 [Citation7]. Dose painting is the subject of an increasing number of – mostly theoretical and/or in silico – studies, investigating various functional imaging modalities as well as dose prescription, optimization and delivery techniques [Citation8–13]. There are a number of uncertainties and unknowns related to the imaging modalitites on which the prescription function should be based, as well as a lot of unknown factors related to the translation of the image into a prescription function for dose painting [Citation14,Citation15]. While much research is still needed on the biological basis for dose painting, clinical studies of molecular imaging based boosting of putative resistant subvolumes are in progress. From a research strategy point-of-view experience from clinical trials provides a valuable complement to the results of laboratory based research. It is therefore relevant to develop the tools for planning and delivery of dose painted treatment plans as a requisite for clinical proof-of-principle studies and this is the context of the present study.
An issue which has received little attention in the literature is the capability of existing treatment planning systems to produce treatment plans for dose painting. The optimization engines of most treatment planning systems are tailored to produce dose distributions which can be described by few dose-volume parameters with the delineation of volume structures as the only spatial guide. The challenge of the dose painting approach is that the desired dose distributions are described by the heterogeneous spatial distribution of dose, which cannot easily be described by dose-volume parameters. In the literature, several research treatment planning systems have been used with features allowing optimization of dose distributions prescribed at the voxel-level [Citation8,Citation10,Citation12,Citation16].
This paper deals with the feasibility of optimising and delivering a heterogeneous dose distribution for dose painting using currectly available commercial soft-and hardware. As a case example, a Cu-ATSM PET image for a head and neck cancer patient is used. Cu-ATSM uptake correlates with clinical outcome in several tumor types and has been shown to correlate with physiologic and endogenous markers of oxygen tension in tumours. Clinical studies have shown that hypoxia is a major cause of treatment failure after radiation therapy [Citation17,Citation18]. For the translation of the PET image into a dose prescription a simple proportional model is used, which in the case example gives rise to a dose prescription with large spatial variability.
In a study presented at AAPM 2009 annual meeting and in a separate paper in this journal issue, the feasibility of performing dose painting using a commercially available TomoTherapy treatment planning system is demonstrated [Citation19]. The present study tests the feasibility of performing dose painting using volumetric modulated arc therapy, which is a widely available technique, increasingly replacing standard IMRT in clinics equipped with gantry-based linacs. As an example of a volumetric modulated arc therapy system, RapidArc is used (Varian Medical Systems, Inc). The dependence of the plan quality on the spatial resolution of the dose objectives used for the treatment plan optimisation will be addressed, as well as the sensitivity to variations of the physical parameters of the beam configuration. Last, the robustness of the treatment plans with respect to positional variations is investigated.
Material and methods
One patient case was used for the study. A patient with a squamous cell base of tongue carcinoma with ipsilateral regional lymph node involvement underwent a pre-treatment [61Cu]Cu-ATSM PET/CT scan three hours post injection. Imaged PET uptake was segmented to include only values within the CT-based planning target volume (PTV), which required downsampling this region of interest in order to match the PET image spatial resolution. A treatment planning CT scan was also performed, and relevant strucures were delineated. For the purpose of the present study, the patient body structure from the original treatment planning system (TomoTherapy Hi Art™, TomoTherapy Incorporated, Madison, WI) was transferred to the Eclipse treatment planning system (Varian Medical Systems, Incorporated, Palo Alto, CA), and a 3D image was created in Eclipse based on this structure. The entire body contour was assigned Hounsfield Unit zero (corresponding approximately to water) in Eclipse. The actual planning CT-images of the patient were not transferred for regulatory reasons.
The Cu-ATSM PET scan image () was translated to a dose prescription based on the following relation:
Figure 1. (a) The Cu-ATSM PET image for the patient, (b) the dose prescription map, (c) the substructures corresponding to five dose prescription levels, (d) an example of the optimised dose.
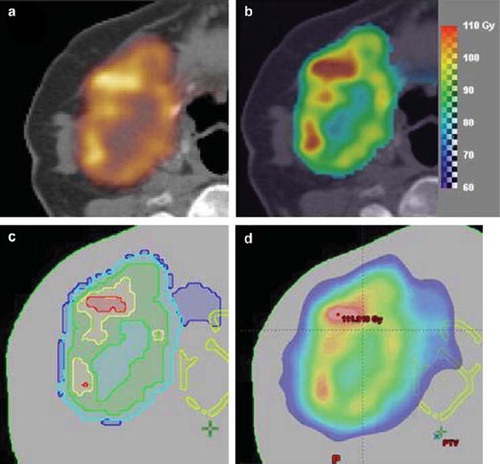
Di = 60Gy + 30Gy * PETi/<PET> (1)
such that a base dose of 60 Gy is given, and the entire target receives a mean dose of 90 Gy. In each voxel i, the dose Di is the base dose plus an escalation dose proportional to the PET SUV in that voxel normalised to the mean PET SUV. The proportionality factor of 30 Gy ensures an overall mean target dose of the base dose plus 30 Gy, i.e. a total of 90 Gy. shows the resulting voxel-based dose prescription as a prescription dose colour wash.
For optimization in the Eclipse treatment planning system, the voxel-based prescription dose was converted to prescriptions with a number of equispaced dose levels. For each dose level, a target substructure was generated corresponding to the outlines of the dose prescription level using Matlab, and the target substructures were subsequently imported into Eclipse. For each substructure, the prescription dose was defined as the mean of the voxel-based prescription dose within that substructure (). These level-based prescription doses are illustrated in c for five levels. In this study, we used level-based prescription doses with 3, 5, 7, 9 and 11 dose levels, respectively, and substructure sets were created for each of these. Additionally, an encompassing target structure was defined (and was identical) in all substructure sets.
Table I. Mean doses (in Gy) for the target substructure sets. Used as prescription doses in the level-based approach, and as target optimization objectives in Eclipse.
The physical parameters in a RapidArc treatment plan which can be varied by the user are basically the MLC width, the number of arcs, the arc rotation angles, the collimator rotation angle, the couch rotation, the isocenter position and the beam energy.
In this study, the following parameter settings, affecting the spatial resolution and the degree of modulation of an optimisation, were investigated in all combinations:
MLC widths 2.5 mm (the HD MLC installed on a Novalis TX machine) and 5 mm (the Millenium MLC installed on an iX Clinac);
One and two arcs (optimised in a single step);
Collimator rotations 45, 30, 15 and 0 degrees. When two arcs were used, the collimator rotations of the two arcs were chosen complementary, such that the collimator rotation of the second arc was 315 (45), 330 (30), 345 (15) and 0.
In all cases, 6 MV beam energy was used, couch rotation was 0 degrees, and all the arcs spanned 358 degrees of an arc rotation. A set of default parameters were chosen as two arcs with MLC width 2.5 mm and collimator rotations 45(315) degrees. This default set yields the most degrees of freedom, and is hence expected to give the best optimization results.
For the treatment plan optimisation, dose objectives were initially defined for each of the substructure target sets using the default parameter set, aiming in all cases for an overall dose distribution mimicking as closely as possible the voxel-based prescription function. For all variations of physical parameters within the same number of dose prescription levels, the same optimisation dose objectives and priorities were used.
All plans were normalised to give a mean dose of 90 Gy to the entire encompassing dose painting target volume.
The quality of a plan was quantified for each voxel as the ratio between the planned (calculated) dose and the prescribed dose in that voxel. This figure of merit was calculated for the level-based dose prescription. Quality index volume histograms were produced – analogous to dose-volume histograms – for all the substructures and for the combined total dose painting target volume, and evaluated in order to assess the quality of a treatment plan. The steeper the cumulative quality-volume histogram (QVH) is, approaching a step-function at the quality index 1, the better the treatment plan. An overall figure of merit for a plan, the Q0.95–1.05, was calculated as the proportion of voxels within a structure of interest having a quality index between 0.95 and 1.05 (dose within ±5% of the prescribed dose).
Deliverability of three selected dose painting plans for 3, 7 and 11 dose prescription levels was tested using the Delta4® (Scandidos AB) dosimetric phantom. The Delta4® is a cylindrically shaped PMMA phantom, surrounding two crossing orthogonal planes with a total of 1069 p-Si diodes. The diodes are disc shaped with a volume of 0.04 mm3, with a centre to centre distance of 0.5 cm in the central area (6 cm × 6 cm) and 1 cm in the outer area (up to 20 cm × 20 cm) of the planes.
Gamma analysis was performed to compare the calculated dose from the treatment planning system with the delivered dose (as previously described for RapidArc measurements in [Citation20]). The gamma analysis was performed with dose deviation criterion 3% and distance to agreement criterion 1 mm using the internal analysis tool of the Delta4® software. The gamma evaluation was performed for dose levels 50 to 500%, and the dose deviation of 3% was taken relative to 3 Gy for a one-fraction delivery.
Robustness towards positional variations was investigated by shifting the plan isocenter and recalculating the dose with fixed number of monitor units. This simulates in a simple way a systematic error throughout the treatment course. The resolution available for isocenter definition in Eclipse is 1 mm, and the robustness tests were carried out for 1 mm shift along all three axes and 2 mm shift along all three axes, respectively. The robustness tests were done for the default settings of 2 arcs, 2.5 mm MLC width and 45 (315) degrees collimator rotation for all numbers of dose prescription levels.
Results
Optimisation for two arcs with collimator angles 45 and 315 degrees and 2.5 mm MLC width, resulted in a plans with Q0.95–1.05 = 67.2%, 79.2%, 87.7%, 91.2% and 92.8% for 3, 5, 7 , 9 and 11 dose levels, respectively. The corresponding QVHs are shown in
Figure 2. Quality volume histograms for: (upper left) all the number of dose prescription levels used (with default settings of the optimisation paramenters); 11 levels (solid line), 9 levels (dashed line), 7 levels (dotted line), 5 levels (dash-dot line), 3 levels (thin solid line), (lower left) 11 dose prescription levels for one (dotted line) and two (solid line) arcs, (lower right) 11 dose prescription levels for MLC width 2.5 mm (solid line) and 5 mm (dotted line), (upper right) 11 dose prescription levels for collimator rotations 45(315) (solid line), 30(330) (dashed line), 15(345) (dotted line) and 0(0) (dash-dot line).
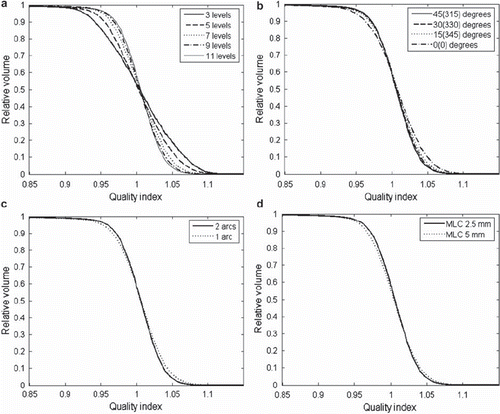
The QVHs for the 11 level plans with variations in physical parameters are shown in . In , the Q0.95–1.05 values are shown for the 11 level structure set for all variations in physical parameters (it would be too many numbers to include in tabular form all Q0.95–1.05 for all number of levels). Generally, the deterioration of plan conformity was small for increased MLC width, and for reduced number of arcs, while the effect of variation in collimator rotation angle was larger. For instance, for 11 dose levels Q0.95–1.05 (2 arcs, MLC 2.5 mm) was 92.8%, 91.6%, 89.7% and 84.6% for collimator rotations 45(315), 30(330), 15(345) and 0, while for one arc (collimator 45 degrees, MLC 2.5 mm) Q0.95–1.05 was 88.9%, and for MLC 5 mm (two arcs, 45(315) degrees) Q0.95–1.05 was 90.5%.
Table II. Tabulation of Q0.95–1.05 for combinations of physical parameters for level-based dose painting for optimisation with 11 dose levels.
Robustness to systematic positional shifts are shown in for all number of dose prescription levels. For 1 mm isocenter shift in all directions, there is a deterioration on plan conformity (Q0.95–1.05) for all plans of a magnitude of 5–15 percent points (relative decrease 10–17%). The magnitude of the deterioration tends to increase with increasing number of levels. For 2 mm isocenter shift in all directions, the deterioration is substantially worse with a magnitude of 14–34 percent points (relative 20–38%), again with a trend towards increasing magnitude for increasing number of levels.
Figure 3. Magnitudes of Q0.95–1.05 for the default settings of optimisation parameters for all numbers of dose prescription levels for no positional shift (circles), shift of 1 mm in all directions (squares), and shift of 2 mm in all directions (diamonds).
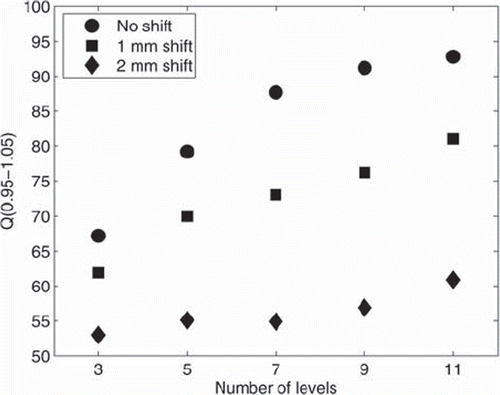
Delivery of plans for 3, 7 and 11 dose prescription levels was tested (for all three plans two arcs were used with collimator angles 45 and 315 degrees and with 2.5 mm MLC width), and the gamma analysis showed a large degree of accuracy (consistency between planned and measured dose). The number of points with a gamma value less than 1 was 89.6%, 88.5% and 92.2%, with 86.6%, 84.6% and 88.8% of the points within 3% dose deviation.
Discussion
It has been shown that high conformity can be achieved using a level-based dose prescription approach as a work-around for dose painting by numbers in a commercial treatment planning system utilising dose volume objective optimization. The quality of treatment plans was highly dependent on the number of dose levels used for the optimisation, with higher number of levels giving better conformity to prescribed dose. This trend is not surprising since the larger the number of levels, the closer does the level-based dose-volume optimisation resemble a voxel-based optimisation. However, the dose-volume based optimisation algorithms are not designed for objectives at the voxel resolution, and it is likely that there is a maximum number of levels for which better optimisations will not be reached. This may be distinguished here in the fact that the difference between using 9 and 11 levels is small.
The quality of the plans was less dependent on the settings of the physical parameters of the beam configuration related to the spatial resolution and modulation of the plans. Variation in MLC width relates to spatial resolution, and a doubling of MLC width from 2.5 mm to 5 mm gave only small change in plan conformity of ∼2–6%. The number of arcs relates to degree of modulation, and a halving of the number of arcs from two to one also gave only a small change in plan conformity in all cases of ∼2–6%.
The collimator rotation relates to both spatial resolution, degree of modulation, and available degrees of freedom. The collimator rotation giving the largest available phase space is 45 degrees, with a second arc having an orthogonal angle of 315 (or 135) degrees.
These planning results in this study are comparable to results obtained using the TomoTherapy commercially available treatment planning system, and reported in a separate paper in this journal issue. Both with respect to the dependency on the number of prescription levels used, and with respect to the overall conformity of plans are the two feasibility studies comparable.
Three test plans in the present study were deliverable with a high degree of accuracy, as measured using a diode array dosimetric phantom. The amount of measurement points in the target volume was limited, which is not ideal for treatment plans with such a high degree of modulation as seen in this case. However, an advantage of the diode array is that the measurement volumes are very small meaning that only little spatial smearing will affect the measurements. Also, for the Delta4® phantom, there is not only one array but two orthogonal arrays, and the spacing between the diodes in the central part of the beam (covering most of the target in this case) is only 5 mm. Ideally, a true 3D dose measurement should be carried out to verify the deliverability of this type of dose distribution. Gels might be a good option, and we have previously demonstrated promising results in using gels for verification of RapidArc delivery [Citation21].
Although there was very high conformity of optimised plans compared to the prescribed dose, the plans were not very robust to positional variations. For a small systematic error of 1 mm in all directions (1.7 mm vector length of error) the plan conformity was decreased substantially for all plans, but still with distinguishable better plans for higher number of optimisation levels. For 2 mm error in all directions, there was low conformity of all plans (only ∼53–60% of dose within 5% of planned dose) irrespective of original plan quality. This result points to the necessity of extremely high precision protocols in the implementation of dose painting in the clinic with extensive image guidance, and use of adaptive planning to accomodate for daily changes [Citation22].
The most important limitation of this study is that only one case has been investigated. The case was chosen among a group of head and neck cancer patients for whom Cu-ATSM PET scans were performed in a study at UW-Madison Cancer Center. The case was chosen as the one exhibiting the most complex dose prescription function based on the PET scan, and is thus expected to represent a worst-case scenario in terms of the challenge it presents to the treatment planning and delivery systems. It therefore makes a good case for a feasibility study. It is likely that most dose painting cases will be equally or less complicated, and that the treatment planning system will be able to optimise to conformal and deliverable dose patterns.
Unfortunately, it was not possible to transfer the actual patient images to Eclipse owing to regulatory reasons, and therefore tissue density and material heterogeneities were not included. This will make a small difference in the optimisations, but it is not expected that it will change the picture substantially. In the parallel TomoTherapy feasibility study, tissue heterogeneities were included, and the results were very similar to the results obtained in the present study.
Clinical guidelines for treatment of head and neck cancers often include several extended target structures treated to different dose levels, such as subclinical target volumes and elective nodes. These have not been considered in the present study. Also, dose constraints to organs at risk have not been considered. The purpose of this study was to investigate the capability of one of the most commonly available treatment planning optimisation approaches to produce the heterogeneity of dose patterns encountered in a complex dose painting example. The treatment planning systems were not designed for this purpose, but rather for using simple dose-volume constraints with structure outlines as the only geographical guides. Since we have now demonstrated the feasibility of achieving these heterogeneous dose distributions, one of the next steps will be to investigate how this can be done when additional clinical dose guidelines and restrictions are imposed.
Studies such as the present, aiming to develop and investigate methods for carrying out dose painting treatment planning and optimisation, are yet scarce in the literature, but important for facilitating the practical implementation of dose painting protocols in the clinic. Several issues need to be addressed, such as establishing the achievable spatial resolution of dose heterogeneities, establishing the appropriate settings of beam parameters, establishing appropriate use of the treatment planning systems etc. Ultimately, it should be clarified what the limiting factors in the treatment planning and delivery steps are for dose painting, and how the optimal results can be achieved in terms of delivering dose distributions as close as possible to the prescribed doses.
The important limiting factors for dose painting at the moment appear to be lying in the preparatory steps of getting from the functional images to the dose painting prescriptions in the first place. Issues especially exist in quantification of functional images, specifically with regard to uncertainties in PET images, in deciding on appropriate functional imaging modalities and tracers for functional PET imaging, and in translating the functional images into dose prescriptions [Citation23]. These limitations cannot be overcome through simple treatment planning studies such as the present. To a large extent, prospective trials will be needed in order to clarify especially those of the issues which are of a biological nature. However, in order to commence prospective trials relating to dose painting, the ability to plan and deliver dose painting must be established and verified, and the present study is an important step in the direction of achieving this.
Conclusions
Planning and delivery of a complex dose painting case was found to be feasible using volumetric modulated arc therapy – exemplified by RapidArc. A workaround employing discrete dose levels and substructures in the treatment planning system was found to be useful for generating a voxel-based dose prescription. The spatial resolution of the dose prescription discretization had a large effect on the conformity of the plan, whereas variation of the physical parameters of the beam configuration had little effect. A test plans was deliverable with a high degree of accuracy (consistency between measured and calculated dose), but generally plans were not robust towards systematic positional variations.
Acknowledgements
This project was supported by CIRRO – The Lund-beck Foundation Center for Interventional Research in Radiation Oncology and The Danish Council for Strategic Research. The project was furthermore supported by the Danish Cancer Society and by Axel Muusfeldts Foundation. The work was furthermore partially supported by grant 1UL1RR025011 from the Clinical and Translational Science Award (CTSA) program of the National Center for Research Resources (NCRR), National Institutes of Health (NIH), and in part by the NCI, grant 2P30 CA 014520-34. Stine Korreman and Silke Ulrich are involved in a research agreement with Varian Medical Systems, Inc.
Declaration of interest: This study was partially supported by a research grant from Varian Medical Systems, Inc. The authors alone are responsible for the content and writing of the paper.
References
- Webb S, Nahum AE. A model for calculating tumour control probability in radiotherapy including the effects of inhomogeneous distributions of dose and clonogenic cell density. Phys Med Biol 1993;38:653–66.
- Webb S, Evans PM, Swindell W, Deasy JO. A proof that uniform dose gives the greatest TCP for fixed integral dose in the planning target volume. Phys Med Biol 1994; 39:2091–8.
- Levin-Plotnik D, Hamilton RJ. Optimization of tumour control probability for heterogeneous tumours in fractionated radiotherapy treatment protocols. Phys Med Biol 2004; 49:407–24.
- Brahme A, Agren AK. Optimal dose distribution for eradication of heterogeneous tumours. Acta Oncol 1987;26:377–85.
- Ling CC, Humm J, Larson S, Amols H, Fuks Z, Leibel S, . Towards multidimensional radiotherapy (MD-CRT): Biological imaging and biological conformality. Int J Radiat Oncol Biol Phys 2000;47:551–60.
- Chao KS, Bosch WR, Mutic S, Lewis JS, Dehdashti F, Mintun MA, . A novel approach to overcome hypoxic tumor resistance: Cu-ATSM-guided intensity-modulated radiation therapy. Int J Radiat Oncol Biol Phys 2001;49:1171–82.
- Bentzen SM. Theragnostic imaging for radiation oncology: Dose-painting by numbers. Lancet Oncol 2005;6:112–7.
- Yang Y, Xing L. Optimization of radiotherapy dose-time fractionation with consideration of tumor specific biology. Med Phys 2005;32:3666–77.
- Malinen E, Sovik A, Hristov D, Bruland OS, Olsen DR. Adapting radiotherapy to hypoxic tumours. Phys Med Biol 2006;51:4903–21.
- Bowen SR, Flynn RT, Bentzen SM, Jeraj R. On the sensitivity of IMRT dose optimization to the mathematical form of a biological imaging-based prescription function. Phys Med Biol 2009;54:1483–501.
- Flynn RT, Bowen SR, Bentzen SM, Rockwell MT, Jeraj R. Intensity-modulated x-ray (IMXT) versus proton (IMPT) therapy for theragnostic hypoxia-based dose painting. Phys Med Biol 2008;53:4153–67.
- Vanderstraeten B, Duthoy W, De GW, De NW, Thierens H. [18F]fluoro-deoxy-glucose positron emission tomography ([18F]FDG-PET) voxel intensity-based intensity-modulated radiation therapy (IMRT) for head and neck cancer. Radiother Oncol 2006;79:249–58.
- Toma-Dasu I, Dasu A, Brahme A. Dose prescription and optimisation based on tumour hypoxia. Acta Oncol 2009;48:1181–92.
- Bentzen SM. Dose painting and theragnostic imaging: Towards the prescription, planning and delivery of biologically targeted dose distributions in external beam radiation oncology. Cancer Treat Res 2008;139:41–62.
- Busk M, Horsman MR, Overgaard J. Resolution in PET hypoxia imaging: Voxel size matters. Acta Oncol 2008;47: 1201–10.
- Thorwarth D, Soukup M, Alber M. Dose painting with IMPT, helical tomotherapy and IMXT: A dosimetric comparison. Radiother Oncol 2008;86:30–4.
- Nordsmark M, Bentzen SM, Rudat V, Brizel D, Lartigau E, Stadler P, . Prognostic value of tumor oxygenation in 397 head and neck tumors after primary radiation therapy. An international multi-center study. Radiother Oncol 2005;77:18–24.
- Koukourakis MI, Bentzen SM, Giatromanolaki A, Wilson GD, Daley FM, Saunders MI, . Endogenous markers of two separate hypoxia response pathways (hypoxia inducible factor 2 alpha and carbonic anhydrase 9) are associated with radiotherapy failure in head and neck cancer patients recruited in the CHART randomized trial. J Clin Oncol 2006;24:727–35.
- Deveau M, Bowen SR, Westerly D, Jeraj R. Feasibility study of helical TomoTherapy for dose painting. Med Phys 2009;36:2783–4.
- Korreman S, Medin J, Kjaer-Kristoffersen F. Dosimetric verification of RapidArc treatment delivery. Acta Oncol 2009;48:185–91.
- Ceberg S, Gustafson H, Korreman S, Medin J, Kjær-Kristoffersen F, Bäck S. RapidArcTM treatment verification using polymer gel dosimetry. J Phys: Confer Ser 2008;164: 012052, IOP Publishing.
- Grau C, Muren LP, Hoyer M, Lindegaard J, Overgaard J. Image-guided adaptive radiotherapy – integration of biology and technology to improve clinical outcome. Acta Oncol 2008;47:1182–5.
- Tanderup K, Olsen DR, Grau C. Dose painting: Art or science? Radiother Oncol 2006;79:245–8.