Abstract
Background. To investigate whether a standard Cone beam CT (CBCT) scan can be used to determined the intra- and inter-fractional tumour motion for lung tumours that have infiltrated the mediastinum. Material and methods. This study includes 23 patients with non small cell lung cancer (NSCLC). The intra-fractional tumour motion was analysed for each patient on a 4D-CT scan as well as on three 4D-CBCT (fraction 3, 10 and 20). The 4D-CBCT was reconstructed from a standard 3D-CBCT using in-house developed software. The tumour (GTV) was delineated in the first phase of the 4D-CT. Registration of phase one from the 4D-CT and 4D-CBCT was used to copy the GTV to the CBCT scans. Hereafter the motion of the outlined GTV was tracked in the planning 4D-CT and the three 4D-CBCT using Pinnacle® version 8.1w (research version). Additionally, the inter-fractional tumour movement, relative to the bony structure, was obtained from the difference in tumour position between the 3D-CT and the standard 3D-CBCT. Results. It is possible to track a lung tumour with mediastinal infiltration in the 4D-CBCT scan based on a standard 3D-CBCT. The respiration motion in the 4D-CBCT is not significantly different from the result found from the initial 4D-CT. Likewise, no differences in respiration motion was found between fractions 3, 10 and 20. Conclusion. This study shows that it is possible to track tumour motion for NSCLC patients with mediastinal infiltration using a standard 3D-CBCT. No change in the intra-fractional tumour motion of clinically relevance was observed during the fractionated treatment course. The inter-fractional tumour motion found underlines the importance of using daily IGRT with online match on soft tissue in order to be able to reduce treatment margins.
Respiration induced geometric uncertainties associated with external beam radiotherapy of patients with lung cancer have been addressed in many studies [Citation1–4]. Several respiratory-correlated acquisition techniques have been developed to construct a four dimensional (4D) CT data set to reduce the uncertainties and artefacts induced by respiratory motion during CT [Citation5–8]. This gives a measure of the induced tumour motion that can be used to improve the treatment planning. However, the measured tumour motion during the CT might not be representative for the motion during the entire treatment course. Hence it would be of interest to investigate the respiratory induced tumour motion during treatment to verify not only the size of the inter-fractional respiration induced motion but also the respiration induced intra-fractional motion. Sonke et al. [Citation9] has already demonstrated that it is possible to track a lung tumour without mediastinal infiltration using a slow gantry speed 4D-Cone beam CT (4D-CBCT). Many patients with lung tumours do have mediastinal infiltration thus it would be beneficial to extend the study by Sonke et al. [Citation9] to a group of patient for which the main fraction do have mediastinal involvement. Furthermore, it would be beneficial if a standard speed 3D-CBCT could be used to create the 4D-CBCT due mainly to two factors: 1) Patients will move slightly on the treatment couch during a treatment. Thus, the less time needed for the treatment the less additional uncertainty is introduced. 2) Use of a slow rotating gantry will increase the time used for image acquisition which affects the normal clinical workflow at the machines for these patients.
Therefore this study is related to the measure of intra- and inter-fractional tumour motion for a group of patients, for which the main fractions did have mediastinal involvement, by the use of a standard speed CBCT. Furthermore the focus of this study was to investigate whether the intra- and inter-fractional tumour motion was unchanged during the entire treatment course.
Material and methods
Patients
This study included 23 patients (one patient with two individual tumours) with non small cell lung cancer (NSCLC). These patients had a visible diaphragm in their CBCT scans so it is possible to reconstruct the respiratory motion. Four tumours were in the lung, one was attached to the thoracic wall and 19 were attached to mediastinum (a component of the tumour in the mediastinum and not an individual lymph node in the mediastinum). The patients were included in this study if they had been CBCT scanned and fulfilled the criteria described above. All patients were scanned in a head-first supine position, with the arms raised above the head. The patients were immobilised using a VacFix vacuum bag with a thermo plastic mask. The patients did not receive any training or coaching for regular breathing before or during treatment.
4D-CT acquisition
The patients were 4D-CT scanned (Siemens Somatom 4) according to standard clinical procedures. Simultaneously, the respiration signal was obtained using a thermocouple placed in a facial mask [Citation8]. The 4D-CT scans were divided in ten time-equidistant phases (phase binning) consisting of 108 slices with a width of 2.5 mm by in-house developed software. The tumour was manually contoured in the 3D-CT scan representing the first phase of the respiration. The contoured tumour was automatically projected to the remaining nine respiration phases by translational rigid registration based on matching of the CT values in the tumour region. Based on the tumour registrations the tumour trajectory and the tumour's time weighted mean position, the mid-ventilation point, was obtained. Based on the mid-ventilation point it was possible to find the respiration phase which was most representative for the mean tumour position, the mid-ventilation phase, as the respiration phase for which the tumour is closest to the mid-ventilation point [Citation8].
4D-CBCT acquisition
All patients were CBCT scanned (Elekta Synergy accelerator with XVI software v 4.2) according to standard clinical procedure as listed in . For this study 3D-CBCT clinical scans (standard gantry rotation speed) from the patient's 3rd, 10th and 20th treatment were used to create 4D-CBCTs. The projection images from the 3D-CBCT scan were sorted in ten equidistant phases according to the breathing phase by use of in-house developed software. The breathing signal, required for sorting, was obtained using an algorithm [Citation10,Citation11] to track the position of the diaphragm motion from the series of projection images (eliminating the need for additional respiratory monitoring systems). Hence only patients where the tumour was located such that the diaphragm was visible in the 3D-CBCT were used. For a given respiration phase a 3D-CBCT was constructed on the XVI system based only on the respiration sorted projection images related to that specific respiration phase. This procedure was repeated ten times thereby creating a 4D-CBCT. All reconstruction was performed with the presets resolution “High Resolution” and collimator “M20”.
Table I. Standard settings for the CBCT scans.
The newest released version of XVI software (version 4.5) makes it possible to use different gantry speeds when performing the CBCT scan (slow gantry rotations produces more images) and to create 4D-CBCT. In the new software as well as in the study by Sonke et al. [Citation9] the default scan time for 3D-CBCT is increased from one minute to four minutes for an CBCT arc of 200 degrees, which is to be compared to the “standard” gantry speed of 200 degrees per minute which is the speed used in the versions of XVI prior to version 4.5 (which is what is referred to as standard 3D-CBCT in this study). As mentioned previously it would be an advantage to use a standard speed gantry rotation to create the 4D-CBCT. It was therefore investigated in the present study whether using a standard gantry speed acquiring the 3D-CBCT scan can be used to track lung tumour motion using an arc of 360 degrees. A side-effect of using a standard speed gantry rotation, which produces less projection images, is that the imaging dose delivered to the patient will be lower compared to the slow gantry speed.
Inter-fractional tumour motion
The inter-fractional tumour motion investigated in this study was the change of mean tumour position relative to bony structures between each treatment session (measured at the planning CT and the three CBCT scans). This is also often referred to as the baseline shift. To find this movement a 3D-CT (reconstructed from all the data used to produce the 4D-CT) and the 3D-CBCT for the patient's 3rd, 10th and 20th treatment were imported into Pinnacle® v 8.1w (research version). A common reference system between the above mentioned 3D-CT and 3D-CBCT scans was obtained by bony anatomy registration of the whole scan and using a cross correlation algorithm with rigid translation and rotation. Hereafter a registration of the tumour was performed using a defined tumour region. For this procedure only rigid translation was used. All the registrations were visually evaluated before a match was accepted.
Intra-fractional tumour motion
To investigate the intra-fractional tumour motion for the patients involved in this study a total number of 920 scans (23 patients × 10 phases × 4 scans) were transferred into the planning system. A common reference system between the different 4D-CT and 4D-CBCT scans for each patient were obtained by registration of the patient anatomy between the scans as previously described. After the co-registration the delineation of the tumour (GTV) was propagated as a solid object from the first phase of the 4D-CT to the first phase of the 4D-CBCTs. Subsequently the tumour motion were tracked in Pinnacle by propagating the GTV from the first phase to the remaining nine phases of the 4D-CT and the three 4D-CBCTs. The displacement of the centre of mass of the GTV between the different phases of each scan was used to describe the tumour motion. Each registration was visually inspected before it was accepted. Data from these registrations were used for the analysis of the tumour motion for all patients’ 4D-CT scan and their 3rd, 10th and 20th treatment 4D-CBCT scans.
Results
For the patients in this study a standard speed 3D-CBCT has 622 projections on average, thus approximately 60 projections in each respiration phase for the created 4D-CBCT. In an example of a patient with a tumour that has infiltrated the mediastinum, is shown. It is seen that the visual image quality is reduced from the 4D-CT to the 3D-CBCT and further reduced for one phase of the 4D-CBCT. In the results of tumour motion tracking is shown for the same patient. From the data for this patient it is seen that despite the reduced image quality it is possible to find the tumour motion in the CBCT. Furthermore it is seen that the extraordinary motion in the left-right (LR) direction found in the planning 4D-CT is the same motion seen in the 4D-CBCTs taken before treatment.
Figure 1. Coronal view of one phase in the 4D-CT, the 3D-CBCT and one phase of the 4D-CBCT for a patient with a lung tumour attached to mediastinum. The visual image quality is clearly reduced from 4D-CT to 4D-CBCT.

Figure 2. Tumour position for the same patient as in figure 1. The solid line is the CT scan and the dashed line 3rd is the CBCT scan.
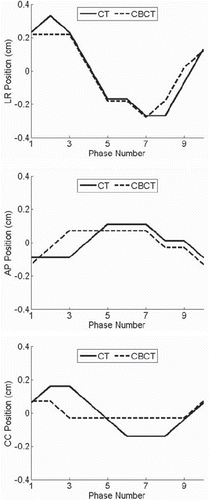
For all 23 patients the standard deviation (SD) for the intra-fractional tumour position (which is the measure of the motion in this study) is seen in . The line in shows identical motion in the two scans. Thus, deviation from the line shows changes in tumour motion. A Wilcoxon Signed Ranks test (2 tailed) was used to test the similarity between the different scans. No statistically significant deviation (p<0.05) was observed in the cranial-caudal (CC) direction. However, in the anterior-posterior (AP) direction there is a small statistically significant shift in the analysis between the 4D-CT and all the three 4D-CBCT scans at fraction 3, 10 and 20. In the LR direction a small shift is detected between the 4D-CT and the 3rd 4D-CBCT. On the other hand there is no significant result among the CBCT scans themselves.
Figure 3. SD for the intra-fractional tumour position (the measure of motion) in the 4D-CT against the SD of the 3rd, 10th and 20th fraction 4D-CBCT scans for all 23 patients in the CC, AP and LR directions.
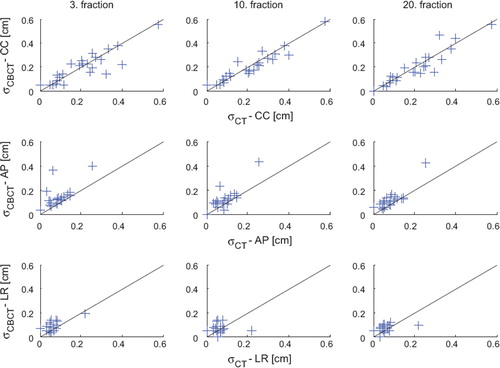
shows the values of the SD for intra- and inter-fractional tumour position (the measure of the motion) averaged over the patients. SD for the intra-fractional position is based on all 960 measured positions (4 scans, 10 phases and 24 tumour locations) while the SD for the inter-fractional position which is the SD of the tumour in the mid-position is based on 96 measured positions (4 scans and 24 tumour locations). The reason for showing the SD and not the peak-to-peak amplitude here is that it is the SD that is used in margin formulas [Citation12–14]. In the present data the ratio between peak-to-peak amplitude and the SD values was found to be approximately 2.5. It is seen from the table that the two components of respiration induced movements are approximately the same magnitude. The largest motion, is as expected, seen in the CC direction.
Table II. Standard deviation of the tumour positions (mm). The numbers are population based SDs.
A linear regression between tumour motions and tumour location within the lung has been performed in this study for the 19 tumours infiltrating the mediastinum. Only the correlation between motion in the CC direction and the position in the CC directions showed to be statistically significant (p< 0.05). A scatter plot of these data is shown in . In the figure the curve found in the study of stereotactic NSCLC patients by Jensen et al. [Citation15] is also plotted.
Figure 4. Tumour motion as a function of relative longitudinal tumour position in the lung (zero is apex of lung and one is base of lung) of the 19 tumours attached to the mediastinum. The solid line is a linear fit to this data. The Pearson correlation coefficient is 0.581 (p=0.009). The uncertainty on the slope coefficient and on the constant of the fit is 0.132 and 0.067 (1SD), respectively. The dotted line shows the result found in Jensen et al. [Citation15] for stereotactic lung patients with a free lung tumour.
![Figure 4. Tumour motion as a function of relative longitudinal tumour position in the lung (zero is apex of lung and one is base of lung) of the 19 tumours attached to the mediastinum. The solid line is a linear fit to this data. The Pearson correlation coefficient is 0.581 (p=0.009). The uncertainty on the slope coefficient and on the constant of the fit is 0.132 and 0.067 (1SD), respectively. The dotted line shows the result found in Jensen et al. [Citation15] for stereotactic lung patients with a free lung tumour.](/cms/asset/d3cb2d2e-6266-41f2-8364-88d06f827478/ionc_a_498834_f0004_b.gif)
Discussion
The results from this study show that it was possible to extract a respiration signal, create a 4D-CBCT and track tumour position using the standard clinical setup. It has previously been shown [Citation16] that a reduction in the number of projection images in a CBCT does not compromise the accuracy of the image registration in XVI. The clinical data in the present study supports this result since a good correlation was found between the 4D-CT and 4D-CBCT data. Hence this study supports the use of a standard full rotation XVI setup with a scan time of two minutes to create 4D-CBCT. This makes it possible to introduce 4D-CBCT into daily clinical practice without increasing the patient's time on the couch. This is of importance in order not to increase the probability of patient movement during treatment. Furthermore, the use of a standard 3D-CBCT allows retrospective studies to be performed on all previously acquired 3D-CBCT data if the projection images are available. The new XVI v 4.5 release has software to produce a 4D-CBCT. By default the scan is predefined by the vendor to a 200 degree rotation with a scan time of four minutes. This study shows that it is possible to decrease the scan time by a factor of two which is now used in our daily clinical procedure for lung cancers using XVI v 4.5.
The correlation seen in showed the motion measured in the 4D-CT planning scan to be representative for the tumour motion in the entire treatment course. This finding has previously been demonstrated by Sonke et al. [Citation9] for tumours situated freely in the lungs but this study shows that the same conclusion is also valid for tumours attached to the mediastinum. A few outliers are seen in . A number of these are related to patients who had anatomical changes during the treatment course (e.g. increased atelectasis). It is therefore important to monitor the CBCT scans during the treatment course in order to detect anatomical changes which might warrant the need of re-planning.
As mentioned in the results section a small deviation between the tumour motion measured in the AP and LR direction is observed for some of the CBCT fractions. However due to the small difference this effect will have no clinical impact on the margins needed for the treatment planning. The reason for the observed small difference is not clear. In this study no patients has been excluded due to tumour shrinkage or atelectasis. Such anatomical changes might induce a change in the respiration pattern. However, such anatomical changes would be expected to occur for the remaining CBCT fractions which are not what is observed in the data. Furthermore, if anatomical changes would be the main reason for a changed respiration pattern, it seems likely that such a change would also influence movements in the CC direction. Neither is the case. Another possible reason for the small deviations found could be that there is a difference in size of the voxels between the 4D-CT and 4D-CBCTs. These are in the CC direction 2.5 and 1.5 mm for 4D-CT and 4D-CBCT, respectively and in the AP and LR direction 0.98 mm and 0.75 mm for 4D-CT and 4D-CBCT, respectively. Whether the smaller voxels size in the non CC direction might facilitate detection of small differences is not clear presently, but seem as a possible explanation since no differences is observed between the individual CBCT scans. However, the small differences in SD in the non-CC direction do not influence the treatment margins needed since the margin related to the lateral respiration motion is very small compared to other uncertainties.
The uncertainty of the measured SD of the intra-fractional movement measured in the three 4D-CBCT scans can be estimated if it is assumed that they represent the same respiration pattern for each patient. If this is the case, the fluctuation in the measured SD is related to the uncertainty of the measured value. For this study it gives a value of uncertainty of the SD in the 4D-CBCT scan that is approximately 0.5 mm which is considerably smaller than the motion measured in the CC direction. Knowing the uncertainty of the SD values in the 4D-CBCT scans an estimate of the uncertainty in the 4D-CT scans can be obtained likewise. It is important to notice that the fluctuation between SD values measured in 4D-CBCT and 4D-CT is a combination of the uncertainty in 4D-CBCT and in the 4D-CT scan. For this study it gives a value of the uncertainty of the SD measured in the 4D-CT scans that is approximately 0.2 mm. The reason for the larger uncertainty for the 4D-CBCT compared to the 4D-CT are of course due to the reduced image quality. The image quality could be improved by using slower gantry speed which gives more image projections. However, the current uncertainty values are sufficient for calculation of treatment margins and verification of tumour position. The image quality could also be improved by use of more advanced reconstruction algorithms; however such more advanced algorithms are currently too slow to be applicable for on-line image verification.
It might be noted that the conclusion in this study would not be very clear if a systematic difference had been detected between the 4D-CT and 4D-CBCT results, since it would not be clear whether the course of the difference would be due to a change in the patients breathing pattern or the inability to measure the respiration pattern correctly using a standard 3D-CBCT scan. One could argue that the same problem exists even in the situation of a high degree of correlation between the two signals, since in theory a change in breathing pattern could be compensated by an error of the measured movement in 4D-CBCT. However, even if such a cancellation of errors could occur for a single patient it is highly unlikely that it would occur for a group of patient.
It has previously been demonstrated that respiration induced longitudinal tumour movement correlates with longitudinal tumour position, hence the closer the tumour is located to the diaphragm, the larger the tumour motion [Citation15,Citation17]. As it was demonstrated in the same finding is observed in this study. The relation between movement and tumour position is very similar to the result from Jensen et al. [Citation15] although that relation was based on a quite different group of patients. Thus also for patients with tumour infiltration of the mediastinum the tumour movement can be estimated based on the longitudinal position of the tumour.
It is seen in that the inter-fractional motion, also named baseline shift, is approximately the same size as the intra-fractional changes. This is the reason why daily IGRT registration not on bony structures but on tumour tissue is needed in order to substantially reduce the treatment margin. If only the margins related to the intra-fractional motion is minimised there will still be a systematic uncertainty related to the baseline shift. This uncertainty would limit the possible reduction in margin dramatically due to its systematic nature. Thus both of these uncertainties need to be addressed in order to minimise the treatment margin. Furthermore, the size of the inter-fractional motion imply that the introduction of gating without use of daily IGRT performed on soft tissue will not warrant a large reduction of the treatment margins.
Conclusion
In this study it is shown that 3D-CBCT of lung cancer patients can be used to create 4D-CBCT without introducing slower gantry speed. By use of such a 4D-CBCT it is possible to track lung tumours attached to the mediastinum. Furthermore, the tumour motion seen at the 4D-CT in the planning phase is representative of the tumour motion during the entire treatment course. Hence respiration induced intra-fractional motion is not changing significantly during the radiotherapy course. The inter-fractional tumour motion found underlines the importance of using daily IGRT on soft tissue in order to be able to reduce treatment margins.
Acknowledgements
This work is supported by CIRRO – The Lundbeck Foundation Center for Interventional Research in Radiation Oncology and The Danish Council for Strategic Research.
Declaration of interest: The authors report no conflicts of interest. The authors alone are responsible for the content and writing of the paper.
References
- Chen GTY, Kung JH, Beaudette KP. Artifacts in computed tomography scanning of moving objects. Semin Radiat Oncol 2004;14:19–26.
- Keall PJ, Mageras GS, Balter JM, Emery RS, Forster KM, Jiang SB, . The management of respiratory motion in radiation oncology report of AAPM Task Group 76. Med Phys 2006;33:3874–900.
- Persson GF, Nygaard DE, Brink C, Jahn JW, af Rosenschöld PM, Specht L, . Deviations in delineated GTV caused by artefacts in 4DCT. Radiother Oncol 2010 (in press).
- Slotman BJ, Lagerwaard FJ, Senan S. 4D imaging for target definition in stereotactic radiotherapy for lung cancer. Acta Oncol 2006;45:966–72.
- Keall P. 4-dimensional computed tomography imaging and treatment planning. Semin Radiat Oncol 2004;14:81–90.
- Østergaard Noe K, Senneville BDD, Elstrøm UV, Tanderup K, Sørensen TS. Acceleration and validation of optical flow based deformable registration for image-guided radiotherapy. Acta Oncol 2008;47:1286–93.
- Rietzel E, Pan T, Chen GTY. Four-dimensional computed tomography: Image formation and clinical protocol. Med Phys 2005;32:874–89.
- Wolthaus JWH, Schneider C, Sonke JJ, van Herk M, Belderbos JSA, Rossi MMG, . Mid-ventilation CT scan construction from four-dimensional respiration-correlated CT scans for radiotherapy planning of lung cancer patients. Int J Radiat Oncol Biol Phys 2006;65:1560–71.
- Sonke JJ, Lebesque J, van Herk M. Variability of four-dimensional computed tomography patient models. Int J Radiat Oncol Biol Phys 2008;70:590–8.
- Sonke JJ, Zijp L, Remeijer P, van Herk M. Respiratory correlated cone beam CT. Med Phys 2005;32:1176–86.
- Zijp L, Sonke JJ, van Herk M. Extraction of the respiratory signal from sequential thorax Cone-Beam X-ray images. Proceedings of the 14th ICCR Conference; 2004; Seoul, Korea. 507–9.
- Antolak JA, Rosen II. Planning target volumes for radiotherapy: How much margin is needed? Int J Radiat Oncol Biol Phys 1999;44:1165–70.
- van Herk M, Remeijer P, Rasch C, Lebesque JV. The probability of correct target dosage: Dose-population histograms for deriving treatment margins in radiotherapy. Int J Radiat Oncol Biol Phys 2000;47:1121–35.
- van Herk M. Errors and margins in radiotherapy. Semin Radiat Oncol 2004;14:52–64.
- Jensen HR, Hansen O, Hjelm-Hansen M, Brink C. Inter- and intra-fractional movement of the tumour in extracranial stereotactic radiotherapy of NSCLC. Acta Oncol 2008;47:1432–7.
- Westberg J, Jensen HR, Bertelsen A, Brink C. Reduction of Cone-Beam CT scan time without compromising the accuracy of the image registration in IGRT. Acta Oncol 2010;49: 225–9.
- Onimaru R, Shirato H, Fujino M, Suzuki K, Yamazaki K, Nishimura M, . The effect of tumor location and respiratory function on tumor movement estimated by real-time tracking radiotherapy (RTRT) system. Int J Radiat Oncol Biol Phys 2005;63:164–9.