Abstract
To establish Boron Neutron Capture Therapy (BNCT) for non-resectable liver metastases and for in vitro experiments at the TRIGA Mark II reactor at the University of Mainz, Germany, it is necessary to have a reliable dose monitoring system. The in vitro experiments are used to determine the relative biological effectiveness (RBE) of liver and cancer cells in our mixed neutron and gamma field. We work with alanine detectors in combination with Monte Carlo simulations, where we can measure and characterize the dose. To verify our calculations we perform neutron flux measurements using gold foil activation and pin-diodes. Material and methods. When L-α-alanine is irradiated with ionizing radiation, it forms a stable radical which can be detected by electron spin resonance (ESR) spectroscopy. The value of the ESR signal correlates to the amount of absorbed dose. The dose for each pellet is calculated using FLUKA, a multipurpose Monte Carlo transport code. The pin-diode is augmented by a lithium fluoride foil. This foil converts the neutrons into alpha and tritium particles which are products of the 7Li(n,α)3H-reaction. These particles are detected by the diode and their amount correlates to the neutron fluence directly. Results and discussion. Gold foil activation and the pin-diode are reliable fluence measurement systems for the TRIGA reactor, Mainz. Alanine dosimetry of the photon field and charged particle field from secondary reactions can in principle be carried out in combination with MC-calculations for mixed radiation fields and the Hansen & Olsen alanine detector response model. With the acquired data about the background dose and charged particle spectrum, and with the acquired information of the neutron flux, we are capable of calculating the dose to the tissue. Conclusion. Monte Carlo simulation of the mixed neutron and gamma field of the TRIGA Mainz is possible in order to characterize the neutron behavior in the thermal column. Currently we also speculate on sensitizing alanine to thermal neutrons by adding boron compounds.
In 1936, G. Locher proposed to use the neutron capture properties of the boron isotope 10B for therapeutic purposes [Citation1]. Thus, the concept of boron neutron capture therapy (BNCT) was born. BNCT is a biologically targeted form of radiotherapy, which uses the ability of the isotope 10B to emit an alpha particle and a 7Li recoil ion following capture of a thermal neutron (En < 1 eV). If the 10B can be selectively delivered to cancerous tissue, the short range of the charged particles offers the potential for a targeted irradiation of individual tumor cells while sparing the surrounding healthy tissue. Since the first trials in the USA in the 1950s, a number of interesting clinical approaches have been tested. Most therapies nowadays are focusing on glioblastoma multiforme [Citation2], head and neck cancer [Citation3] or malignant melanoma [Citation4].
Our aim is a curative therapeutic approach for different types of liver cancer. The BNCT project in Mainz is based on the TAOrMINA protocol of the University of Pavia, Italy [Citation5]. For patients suffering from colorectal carcinoma there is a 50 to 80% chance to show liver metastases. For the half of these patients, the dissemination of metastases is restricted to the liver alone (25 to 40% of all cases). Due to their number and inner-hepatic distribution only 25% of these patients (10% of the overall number of patients) can be treated by local or local-ablative surgical actions [Citation6]. For a significant number of the remaining patients, BNCT could be a promising alternative. The intended treatment requires that the liver is explanted before the neutron irradiation and re-implanted afterwards (auto-transplantation). In detail, boron is administered via an infusion of boronphenylalanine (BPA), which is given to the patient over a certain period of time and is required for the boron distribution in the body. The liver is then explanted and irradiated at the TRIGA Mainz. After irradiation, the liver is transported back to the hospital where it is re-implanted.
Before the treatment can become a real alternative to traditional therapies, many parameters have to be determined and combined to create a clinical treatment protocol. Therefore, a multinational research group has been formed to conduct research in BNCT to explore its possibilities for a curative treatment. Additionally, a clinical phase I trial has been started at the University Hospital of Mainz to determine the uptake of the BPA in blood, healthy, and cancerous tissue and to develop a dosimetry protocol. Within this trial, after an infusion of BPA, patients undergo a resection of one liver lobe, which may then be used for further dosimetric experiments. So far four patients have been enrolled.
Furthermore, in vitro experiments are being performed to determine the influence of radiation on tumor cell proliferation compared to conventional irradiation with gamma rays. With the respective results, the relative biological effectiveness (RBE) of different cell lines (HuH7 and HepG2) towards neutron irradiation shall be calculated.
In the in vitro experiments, as well as for the treatment of future patients, it is crucial to have a reliable dosimetry system. So far, it was possible to determine only neutron and gamma fluences by pin-diodes and gold foil activation measurements in different positions in the irradiation channel, but not directly inside tissue or cells, which is why they were entirely calculated using MCNP. To back such calculations, it was necessary to collect reliable experimental data. First attempts with TLDs (thermo-luminescence detectors), which were inserted prior to irradiation into the resected liver lobe were not successful, because the fluences needed in the planned treatment are much higher than TLDs can tolerate.
From previous studies elsewhere [Citation7,Citation8], alanine detectors would appear to be a more reliable option, especially in combination with thermal neutron fluence measurements and Monte Carlo (MC) simulations, in order to establish a system for dosimetry monitoring in the mixed neutron and gamma field in the thermal column of a TRIGA reactor.
The alanine solid state detector has earlier been suggested for dosimetry of mixed radiation fields [Citation7], due to the availability of a response model which describes the reduced relative effectiveness of the detector when exposed to hadronic radiation fields. The Hansen & Olsen model [Citation9–11] is based on the track structure formalism established by Butts and Katz [Citation12], and has recently successfully been applied for forward dose calculation of mixed radiation fields found in antiproton and carbon-ion beams [Citation7,Citation8]. For a given geometry, the Monte Carlo particle transport code FLUKA [Citation13] can calculate the mixed charged particle spectrum found in an alanine pellet. Applying the Hansen & Olsen model, the detector response of that particular alanine pellet can be calculated and can be compared with experimental findings. We think that the high saturation dose of alanine and its tissue equivalent composition makes this detector a very interesting candidate for dosimetry of mixed photon/neutron fields.
Material and methods
The TRIGA Mark II reactor ()
All irradiations take place at the TRIGA Mark II reactor at the University of Mainz. The reactor can be operated in steady state mode with a maximum power of 100 kWth and in pulsed mode with a peak power of 250 MWth for a period of less than 100 ms. The TRIGA has four beam tubes and a thermal column which can be reconstructed for the treatment of an explanted organ and which is used for the alanine dosimetry and in vitro experiments. The thermal column is constructed of graphite and has an aluminium lining. The column can be opened at the exit-facing side and has optionally rectangular channels of different sizes.
Clinical trial and in vitro experiments
The patients selected for our trial undergo a hemi-hepatectomy due to the indication of hepatic metastases caused by colorectal cancer. The surgical protocol remains unchanged except for an additional infusion with the BPA (200 mg/kg body weight) and the taking of blood samples several times during the infusion. After resection, the removed liver lobe is first perfused with an HTK-solution via the portal vein and the hepatic artery, the same way as it is done during transplantation surgery. Following perfusion, tissue samples are taken. The liver specimen is then taken to the TRIGA Mainz reactor for subsequent irradiation in the central channel, 20 × 20 cm2, of the thermal column. In the tissue and blood samples, the boron concentration is determined by Prompt Gamma Ray Spectroscopy (PGRA), Quantitative Neutron Capture Radiography (QNCR), and Inductively Coupled Plasma Mass Spectrometry (ICP-MS).
For in vitro experiments, the cell lines Huh7 and HepG2 are used. They are irradiated in the same channel of the thermal column.
Alanine dosimetry
The alanine pellets have a diameter of 5 mm and a thickness of 2.2–2.3 mm, consisting of 90% finely grained crystalline alanine powder and 10% paraffin wax. When alanine is irradiated with ionizing radiation, it forms the stable radical CH3-H-COOH. Using an electron spin resonance (ESR) spectrometer, the unpaired electron at the carbon atom can be detected. The value of the ESR signal correlates directly to the number of radicals. All alanine pellets which we have used in our experiments are read out at the primary standard laboratory at the National Physical (NPL), UK, due to long time experience with alanine dosimetry in photon fields and well established readout protocols [Citation14].
The signal in each pellet correlates to an equivalent 60Co gamma dose by a factor called the relative effectiveness (RE). To determine the RE values and to predict the dose for each pellet, we use the Hansen & Olsen alanine detector response model together with FLUKA, a multipurpose transport Monte Carlo code able to treat particle interactions up to 10 000 TeV. The only information needed for our calculations is the neutron and gamma spectrum for the TRIGA Mainz [Citation15] and the buildup of the thermal column []. For the simulations performed in this study, we implemented a two dimensional (2D) surface source of photons and neutrons, located perpendicular in the thermal column, 63 cm away from the centre of the core. The graphite of the column is surrounded by the aluminum lining and 1 m concrete in every direction for the calculation geometry.
To validate the MC results, thermal neutron fluence measurements using gold foil activation and a pin-diode are used. The irradiated gold foils were analyzed using a standard high-purity germanium (HPGe) gamma spectrometry system (Canberra/Genie™). The induced activities of gold were computed from the photopeak areas of the 411 keV gamma peak of gold. The system efficiencies based on calibrations of the spectrometer using the PTB, Germany standards and a mixed calibration source. PTB is the abbreviation of Physikalisch-Technische Bundesanstalt, which is the national institute for natural and engineering sciences. The activation correlates to the absolute integral of the thermal neutron fluence. The pin-diode is augmented by a lithium fluoride foil. This foil converts the neutrons into alpha and tritium particles which are products of the 7Li(n,α)3H reaction. These particles are detected by the diode and the measured amount correlates to the neutron fluence. As the pin-diode is an online detector, it can be used for online dosimetry, once it has been calibrated in the respective radiation field, i.e. using gold foils.
Results and discussion
Both gold foil activation and the pin-diode are reliable neutron fluence measurement systems for the TRIGA reactor. At the core-facing side of the thermal column, the thermal neutron flux is around 1010 n/cm2·s, at the exit-facing side around 108 n/cm2·s, measured absolutely by gold foil activation. The pin-diode was calibrated for several positions in the thermal column. A position at the exit-facing end of the column is advantageous because it prevents saturation effects at high neutron fluxes. shows an exemplary measured calibration curve for the diode. With such curves it is possible to correlate the diode signal at the exit-facing end with low neutron flux in the column to the neutron flux at the other end or in between both positions.
With the neutron and gamma spectra, in addition to the geometry of the reactor, it is possible to simulate the neutron and gamma field in the thermal column. shows the simulated fields for the thermal column. The simulation gives for each point of the geometry the particle flux normalized per unit volume and unit primary particle (neutron or photon). Because of this, it is necessary to multiply the result with a certain factor to get the real flux. For the shown data a gold foil measurement (performed in the central channel 104 cm from the core) was used to calculate this factor. Additional gold foil measurements are shown in compared to the FLUKA results for the corresponding points and former MCNP calculations. shows that it is possible to use FLUKA to simulate the neutron behavior in our thermal column.
Figure 3. a) Neutron flux in the thermal column. b) Photon flux in the thermal column (Flux dimensions in n cm−2 s−1 (a) respectively p cm−2 s−1 (b)).
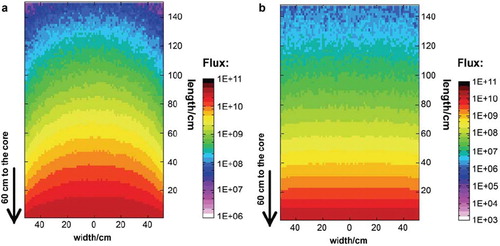
With these data, we are able to calculate the alanine detector response and the corresponding RE values using a combination of FLUKA and the Hansen & Olsen model. The first comparisons of alanine measurements with these calculations are promising, but further experiments with alanine detectors in different phantoms are in progress to confirm our results.
Another result of the simulations is that the largest part of the alanine dose is due to the gamma radiation, which is generated in the core and its surroundings. The thermal neutrons do not lead to a direct dose. This dose originates from secondary particles such as protons or electrons. These particles occur due to the interaction between neutrons and material they pass through.
These results are a substantial improvement in calculations of the dose in healthy and tumor tissue. From the boron concentration measurements, it will now be possible to calculate all the weighted dose values, using the corresponding RBE values from the in vitro experiments.
Conclusion
With the application of FLUKA it is possible to simulate neutron fluences and the dose for the alanine pellets. With further investigation, it will be possible to develop a reliable dosimetry monitoring system for a liver treatment and in vitro experiments at the TRIGA Mainz.
To increase the sensitivity of the alanine to thermal neutrons, we are planning to modify the alanine in the detectors with boron to get a higher thermal neutron dose into the pellet, which would lead to even more detailed dosimetry results.
Acknowledgements
The authors acknowledge support in part by the Danish Cancer Society (www.cancer.dk), the Lundbeck Foundation Centre for Interventional Research in Radiation Oncology (www.cirro.dk), the Boehringer Ingelheim Foundation, the National Science Foundation under grant CBET-0853157 and from the European Union in form of a Marie Curie International Incoming Fellowship grant # PIIF-GA-2009-234814.
Declaration of interest: The authors report no conflicts of interest. The authors alone are responsible for the content and writing of the paper.
References
- Locher GL. Biological effects and therapeutic possibilities of neutrons. Am J Roentgenol 1936;36:1–13.
- Yamamoto T, Nakai K, Matsumura A. Boron neutron capture therapy for glioblastoma. Cancer Lett 2008;262: 143–52.
- Kato I, Ono K, Sakurai Y, Ohmae M, Maruhashi A, Imahori Y, . Effectiveness of BNCT for recurrent head and neck malignancies. Topics in Neutron Capture Therapy: Proceedings of the Eleventh World Congress on Neutron Capture Therapy (ISNCT-11). App Radiat Isot 2004;61:1069–73.
- Menéndez PR, Roth BMC, Pereira MD, Casal MR, González SJ, Feld DB, . BNCT for skin melanoma in extremities: Updated Argentine clinical results. 13th International Congress on Neutron Capture Therapy BNCT: A new option against cancer. Appl Radiat Isot 2009;67: 50–3.
- Zonta A, Prati U, Roveda L, Ferrari C, Zonta S, Clerici AM, . Clinical lessons from the first applications of BNCT on unresectable liver metastases. J Phys: Conference Series 2006; 41:484–95.
- Kemeny N, Fata F. Arterial, portal, or systemic chemotherapy for patients with hepatic metastasis of colorectal carcionoma. J Hepatobiliary Pancreat Surg 1999;1:39–49.
- Bassler N, Hansen J.W, Palmans H, Holzscheiter MH, ovacevic S. The antiproton depth-dose curve measured with alanine detectors. Nuc Instr Meth Phys Res B 2008;26:929–36.
- Herrmann R. The response of alanine in a therapeutic carbon ion beam. Fakultät für Physik und Astronomie, Ruprecht-Karls-Universität Heidelberg, Germany 2009; Master's Thesis.
- Hansen JW, Olsen KJ. Theoretical and experimental radiation effectiveness of the free radical dosimeter alanine to irradiation with heavy charged particles. Radiat Res 1985;104: 15–27.
- Hansen JW, Olsen KJ, Wille M. the alanine radiation detector for high and low LET Dosimetry. Radiat Prot Dosimetry 1987;19:43–7.
- Hansen JW, Waligorski MPR, Byrski E. Intercomparison of gamma ray X-ray and fast neutron dosimetry using alanine detectors. Radiat Prot Dosimetry 1989;27:85–92.
- Butts JJ, Katz R. Theory of {RBE} for heavy ion bombardment of dry enzymes and viruses. Radiat Res 1967;30: 855–71.
- Battistoni G, Muraro S, Sala PR, Cerutti F, Ferrari A, Roesler, . The FLUKA code: Description and benchmarking. Proceedings of the Hadronic Shower Simulation Workshop 2006; AIP Conference Proceedings 2007;896: 31–49.
- Sharpe P, Sephtan J. An automated system for the measurement of alanine/EPR dosimeters. Appl Radiat Isot 2000;52: 1185–8.
- Wortmann B. Auslegung und Optimierung einer Bestrahlungseinrichtung für die Bor Neutronen Einfang Therapie an autotransplantierten Organen; Fakultät Maschinenwesen, TU Dresden, Germany, 2008, PhD Thesis.