Abstract
The use of positron emission tomography (PET) using F18 labeled fluorodeoxyglucose (FDG) for both oncology disease staging and radiation therapy target volume delineation has steadily increased over the last decade, and FDG-PET is today readily available in all major medical centers. The goal of anti tumor treatment, including chemotherapy and/or radiation therapy is to diminish a tumor cell population, ideally to the state of total eradication. Reducing the number of viable tumor cells can lead to a reduction in anatomical tumor size, and may also be correlated with decreased FDG uptake. Efforts to assess tumor response to therapy have attempted to describe and quantify changes in glucose utilization, also referred to as metabolic tumor response. In this review, an attempt is made to present and discuss methodologies to assess and quantify tumor metabolic response to radiation therapy or chemoradiation treatment courses.
The use of positron emission tomography (PET) using F18 labeled fluorodeoxyglucose (FDG) for both oncology disease staging and radiation therapy target volume delineation has steadily increased over the last decade, and FDG-PET is today readily available in all major medical centers. Both uses take advantage of increased glucose transport and hexokinase levels in tumor cells, resulting in disproportionably high intracellular trapping of FDG-6-phosphate when compared to normal healthy tissues. In malignant tumors, FDG uptake has been shown to belated with both proliferative activity as well as the number of viable cells [Citation1–6]. The goal of anti tumor treatment, including chemotherapy and/or radiation therapy is to diminish a tumor cell population, ideally to the state of total eradication. Reducing the number of viable tumor cells can lead to a reduction in anatomical tumor size, and may also be correlated with decreased FDG uptake. However, tumor response to therapy does not always lead to significant volume reductions assessable by CT and/or MRI. In fact, anatomically stable tumor volume and partial tumor response by trans-sectional imaging create particular challenges in the assessment of the persistence of residual viable tumor. Thus, it comes as no surprise that efforts to assess tumor response to therapy have attempted to describe and quantify changes in glucose utilization, also referred to as metabolic tumor response. An analysis of the National Oncologic PET Registry published in 2008 revealed that 19% of registered scans were acquired for treatment monitoring [Citation7], a number that is certain to increase in coming years.
In this review, an attempt is made to present and discuss methodologies to assess and quantify tumor metabolic response to radiation therapy or chemoradiation treatment courses. This review appears necessary as a variety of measures to quantify regional tissue glucose metabolism have been established, or recommended. While exemplary published clinical data are referenced in the context of critically assessing quantitative approaches to response assessment, this overview is not intended to comprehensively summarize the available literature.
Quantitative assessment of FDG uptake in tissues
Rationale for quantitative analysis
For both oncology disease staging and response assessment following a therapeutic intervention, visual assessment of a focus of pathologic uptake, and its reduction or normalization after therapy may arguably suffice. In fact for response assessment for hematological malignancies standardized in the International Harmonization Project in Lymphoma, complete remission is assumed if FDG uptake of lymphomas by visual assessment does not exceed the uptake in the mediastinal blood pool [Citation8]. However, if FDG uptake following treatment is visually reduced, yet still above background metabolic activity, only quantitative analysis will allow to meaningfully assessing the amount of change.
Standardized uptake value (SUV): Concept and limitations
The uptake of F18 FDG in tissue can be expressed as an activity concentration value such as Bq/cm3, a value that can be directly derived from the PET image-date. More commonly, FDG uptake is expressed as a standardized uptake value (SUV). SUV is calculated as an index derived from the FDG concentration in a particular tissue divided by the ratio of the injected dose to the subject's body weight. A PET image can thus be understood as a SUV map, composed of image volume elements (voxels) that each carry a distinct SUV.
Potentially limiting the value of using SUV to quantify FDG uptake is the fact that a particular SUV cannot be understood as a singular objective value, in fact SUV has to be considered a semiquantitative assessment. Any measured SUV is depended on a number of factors, with time after radiolabeled glucose injection and equipment used being the most critical to allow comparison between studies in general, as well as baseline and follow-up studies. Ideally PET imaging of the FDG uptake is acquired when the activity concentration in tissue is linearly correlated with net FDG phosphorylation [Citation9]. In clinical practice this state is often arbitrarily assumed at 50 to 70 minutes after injection. However, in most tumor types changes in SUV (mostly increases) can be measured well beyond this time, making standardized protocols for serial PET measurements to assess a particular change a necessity [Citation10].
Factors such as unrecognized FDG extravasation at the site of injection, and high blood glucose levels in diabetic patients or a postprandial study can lead to erroneous low tumor SUV [Citation11,Citation12]. Other limitations to the use of SUV as a quantitative measure are related to the impact of the subject's body habit, mainly the ratio of total body fat relative to overall body weight. White body fat participates little in FDG metabolism, creating the possibility of an artificially high tumor and normal organ SUV [Citation13,Citation14]. While this may be predominantly an issue for inter-person comparisons, and less so for serial assessments in the same person, this observation has still potential relevance for intra-individual repeat assessments in the field of oncology, as side effects from therapeutic measures often cause weight loss, and thus body fat loss. Ways to account for body composition and its effects on SUV, is to calculate a SUL (standardized uptake normalized to lean body mass), or normalization to body surface. While SUL is favored by select investigators, SUV remains the most commonly used clinical metric to report uptake of FDG in tissues.
SUVmax
In clinical practice, the FDG uptake in a ROI such as a tumor is most often reduced to a single number, the maximum measured SUV value or SUVmax. A tumor SUVmax represents the uptake within a single voxel inside an arbitrary ROI, such as a sphere encompassing a lesion or an automatic threshold rendered volume over the tumor. Often, the “search” for a lesion SUVmax is based on a visual assessment of a maximum intensity projection (MIP) PET reconstruction, as visualized in and , during which an uptake anomaly is identified. The user then places a sphere of variable size over the ROI to include the grossly abnormal uptake region, and a vendor provided algorithm determines the highest SUV within the selected region (). Different SUVmax can be reported for multiple lesions.
Figure 1. Display of a maximum intensity projection (MIP) in coronal view (A), as well as a transverse PET slice reconstructed by CT attenuation correction. A solitary, FDG avid mass is recognized in projection onto the left lung. Corresponding pathology represents a NSCLC. Tumor stage by imaging and biopsy sampling of mediastinal nodes was T1N0M0.
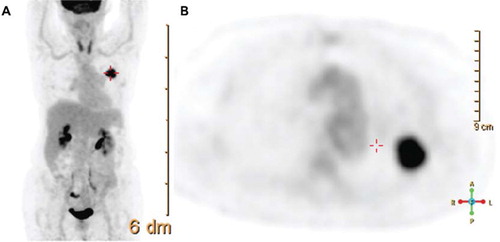
Figure 2. Different approaches to assessing the glucose metabolic activity of a lung lesion (biopsy confirmed NSCLC). Assessment by SUVmax (A) by fitting a sphere of 23.5 mm diameter to the lesion detects maximum uptake of 9.5. Mean uptake in a 10 mm diameter circular area around the location of SUVmax reveals a peakSUV of 7.4 (B). Automated threshold rendering (C) based on a minimum SUV of 3 allows der iving SUVmax, SUVmean, and a volume with an uptake higher than selected threshold (here 8.1 cm3). This threshold rendered structure may be exported to a radiation therapy planning software for objective structure generation.
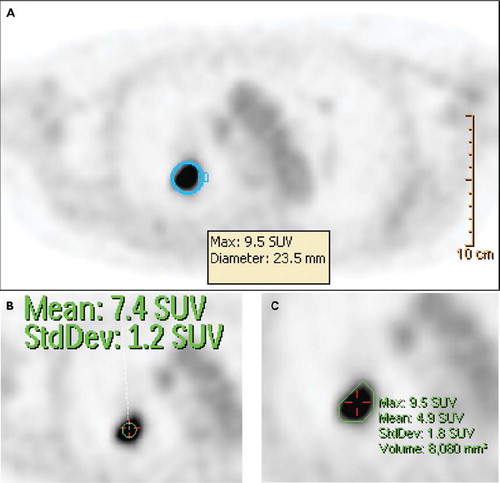
While relatively robust and quick to assess, criticisms of rendering a SUVmax are based on concerns that data noise may affect the accuracy of this maximum uptake reading. In serial assessments, SUVmax values are readily produced as long as post-treatment FDG uptake remains pathologic. Upon reduction or normalization of pathologic FDG uptake in a prior lesion, an anatomically different uptake region that may not represent the treated lesion may be visually selected in error to derive a SUVmax, unless correlation with anatomic computed tomography (CT) imaging is available.
Peak SUV
To overcome the limitations of noise issues associated with reporting the SUVmax based on a single voxel, a peakSUV may be generated. The peakSUV represents the mean or average SUV over an area in one imaging slice surrounding the location of the SUVmax. Ideally a circle with a diameter of 10 mm is positioned over the SUVmax (). This means of measuring FDG uptake is favored for prospective clinical assessments by the American College of Radiology Imaging Network (ACRIN). A typical trial to use peakSUV as the primary outcome measure is ACRIN 6668/RTOG 0235. This prospective clinical trial has the primary purpose to determine the role FDG PET acquired shortly after the end of a course of definitive chemoradiation treatment for locally advanced non small-cell lung cancer in predicting long-term clinical survival. As with reporting SUVmax, serial assessments are reliable as long as pathologic uptake can be visually assessed. Normalization of uptake within a tumor carries risk for false comparisons, unless registered CT data is used for comparative assessment.
Volume of tissue encompassed by a SUV threshold
Most vendor supplied PET imaging processing software platforms allow rendering a ROI or structure based on a user selected absolute SUV threshold or a percentage of maximum SUV (). By placing a cursor inside a target region, all voxels above the selected threshold are rendered as an analysis structure. In oncology applications SUV thresholds higher than 2.5 or 3 are often considered to be representative of tumor metabolic activity. Aside from enabling quantification of a mean SUV within such a threshold region, the volume encompassed by a set SUV threshold may also be valuable for serial assessment. Such threshold derived structures are often exported as DICOM structures into radiation therapy treatment planning software for radiation therapy planning structure generation. Any treatment effect may impact on both the mean SUV and the volume of tissue measuring with a FDG uptake above a chosen threshold, and may as such provide the basis for metabolic response assessment.
Glucose Metabolic Rate Mapping
Assessing the kinetic of FDG uptake after injection instead of waiting about one hour to start image acquisition allows calculating a ROI glucose metabolic rate (MRglu), a parameter that is not time dependent and may be more objective than assessing a SUV. MRglu depends on a rate constant for free FDG transport from plasma into tissue, a rate constant for transport of free FDG back into plasma, and a rate constant for phosphorylation of FDG inside of cells. If conducted as a voxel by voxel analysis, an MRglu map can be generated which may again be used to derive structures for analysis or for radiation therapy planning. Challenges to the widespread use of MRglu are based on the fact that the patient will need to lay on the PET scanner table for the entire uptake time (about 60 minutes) in addition to typical diagnostic scan times, and the need for repeated arterial blood glucose sampling. Tumor response to therapy may affect the MRglu, making MRglu an alternate, yet more resource intense and more invasive methodology to assess metabolic tumor response.
Assessment of metabolic response
Dimension of change constituting a metabolic response
In order to be useful for therapy response assessment, any change in FDG metabolism must be larger than potential physiologic FDG uptake fluctuations within tumor tissues. Those fluctuations have been documented to be typically within an approximate 10% range, when FDG PET scans are repeated within few days without interim therapeutic interventions [Citation15–19]. No generally accepted criteria for establishing tumor response to treatment have been established. While a consensus paper based on a 2005 Cancer Imaging Program workshop sponsored by the US National Cancer Institute was issued, the panel of experts also recognized that no agreed upon standard for judging the significance of response based on FDG uptake reduction has been identified [Citation20]. Aside from a visual assessment of PET images, which is highly subjective and discouraged for use in clinical trials, SUVmax are most often used to document treatment response. Arbitrary recommendations include a minimum 20% change in SUVmax, or an absolute change in SUV of at least 0.9 [Citation17]. An objective metabolic response has been proposed in the 1999 EORTC PET response criteria as complete resolution of FDG uptake within tumor volume so that it was indistinguishable from surrounding normal tissues [Citation21] (an example for complete FDG uptake resolution is shown in ).
Figure 3. Metabolic tumor response of a biopsy confirmed NSCLC treated by Stereotactic Body Radiation Therapy (SBRT, 60 Gy in 5 fractions). Upper left figure represents PET-CT fusion at baseline; the upper right figure represents metabolic response at three months post completion of SBRT. By visual inspection, the uptake has resolved. The lower figures represent two ways of plotting data gathered by deformable registration-based voxel to voxel mapping. The follow up PET scan was mapped onto the baseline PET to derive voxel specific response data which can be plotted in individual uptake distribution graphs for the two PET scans (lower left), or a scatter plot which tracks uptake changes on a voxel by voxel basis (lower right). The lower left graphs confirm that FDG uptake at three months post SBRT (display in green) is much reduced compared to baseline (red), with SUVmax reduction from 10.7 to less than 2. The lower right scatter plot shows all voxels in blue in which the SUV has decreased by more than 10%. Voxels which show an increase or decrease of 10% over baseline are rendered in green. Voxels that would have an increase in SUV by more than 10% would have been rendered in red in the upper left aspect of the plotted area. In this particular case, virtually all voxels with an initial SUV of 2.5 or higher have decreased to SUV values of 2 or lower. Only voxels with low initial SUV have retained their baseline uptake (green).
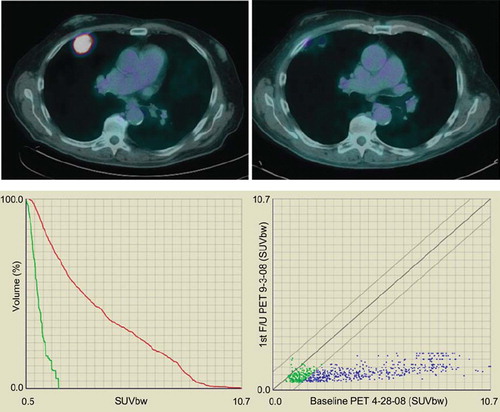
Timing of repeat assessment
Unlike metabolic response to chemotherapy, where changes in tumor metabolism may be assessed within days or very few weeks post treatment administration, metabolic tumor repose to radiation therapy appears more delayed, with robust response assessment being feasible after a minimum follow-up of six to eight weeks. It has been recognized that FDG decline measured early after a therapeutic intervention may be smaller than declines assessed at later time points (example shown in ) [Citation21,Citation22]. Prospective trials assessing the optimal timing of post radiation therapy treatment response are sparse. Increasingly, early response assessments are conducted during treatment or within few weeks after completion of neoadjuvant treatment courses for rectal and esophageal cancer, often showing decline in metabolic tumor activity, but with residual tumor FDG uptake [Citation10,Citation23,Citation24]. Similar data has been shown for cervical cancer patients undergoing chemoradiation, with a subset of cases showing complete metabolic response already during treatment delivery [Citation25]. Longer delays between baseline assessment and follow-up scans (12 weeks) may help distinguishing between treatment-associated inflammatory response and residual viable tumor tissue.
Figure 4. Upper figures represent PET-CT fusion images of a recurrent endometrial cancer at baseline, three months post completion of hypofractioned radiation therapy (45 Gy in 18 fractions), and at six months post radiation therapy. At three months of follow-up a significant response can be assessed visually, but minor uptake remains in a paraaortic tumor nodule. This uptake has resolved at six months, without evidence of tumor recurrence. The lower left figure shows graphs of SUV uptake distribution at baseline (red), first follow-up (green) and second PET-CT follow-up (blue). Not only is SUVmax decreased from a baseline value of 20.7 to 4.6 at three months, and to 3.2 at six months, but also the SUV distribution in general is further decreased with additional follow-up. The lower right figures show voxel by voxel response scatter plots, which indicate a significant response at three months, with further SUV declines later in follow-up. Notable here is again the excellent response of initial high SUV voxels, but also the observation that select voxels with initial low SUV show an increase at early follow-up (red dots; mid figure), with some normalization at later imaging (left figure). This increase in FDG uptake in initial low SUV voxels is preliminarily interpreted to represent inflammatory response with unknown prognostic significance.
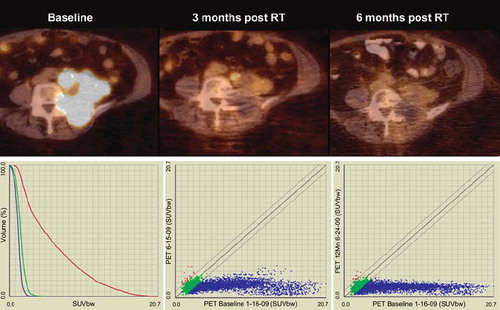
Protocol and technical considerations for response assessment
Based on the above discussed limitations associated with using SUV as the key clinical parameter to report tissue glucose metabolism, particular attention needs to be paid to assuring that baseline and follow-up studies are acquired in comparable ways, as otherwise, variability associated with image data acquisition protocols may be larger than the treatment effects to be measured. The most critical parameter aside from dose injected and patient preparation is the timing of image data acquisition after F18 FDG injection [Citation10]. Standardized institutional protocols are strongly suggested. In multi-center prospective clinical trials, standardization of image-acquisition parameters and certification through a central data quality committee or core laboratory are critical (a good example is ACRIN6668/RTOG 0235). While it is generally advisable to conduct both baseline and follow-up scans in the same institution, it is also strongly suggested that in facilities with multiple PET units, baseline and follow-up PET scans be acquired on the same unit or at least on a same model scanner.
Assessment metric
Clinical FDG PET scans are most often evaluated visually, with SUVmax data reported for the most prominent lesions. NCI PET guidelines as well as prominent investigators favor SUL and body surface normalized uptake data for serial assessments. “Peak” measurement approaches appear most robust, and have been integrated as primary endpoint into prospective cooperative group trials (ACRIN and RTOG). Short of an expert consensus, it appears prudent to suggest reporting parameters that include at least SUVmax and/or SULmax, as well as respective peak values.
Proposals for novel metabolic response assessment methods
Deformable registration based voxel to voxel mapping
Advanced means of image fusion allow for deformable registration of follow-up CT and PET data onto a baseline scan and afford serial voxel to voxel mapping of metabolic activity (). In serial assessments, multiple time points can be assessed and compared against baseline. Conceptually, this approach is similar to tracking contrast media inflow over time in a ROI in dynamic contrast enhanced CT and MRI studies (DCE CT and DCE MRI). Obviously, while DCE CT and MRI studies are based on an analysis of multiple images acquired in a single session over one anatomical region, serial voxel to voxel mapping of follow-up PET onto baseline PET data is not only complicated be a time delay of weeks or months between image acquisitions, but also needs to compensate for potential anatomical changes as a consequence of interim tumor directed treatment. However, deformable registration of anatomic follow-up CT data of a PET-CT data set onto a baseline CT from a comparable baseline PET-CT dataset can be performed using dedicated institutional or commercial software co-registration or fusion platforms such as the Velocity AI software (Velocity, Atlanta, Georgia, USA). The associated PET data are then deformed according to the CT deformation matrix. This process allows to compare uptake values between baseline and follow-up study and to plot them into graphs for visualization and data analysis (Figure 5). Such assessments are obviously much more data rich than measurement of SUVmax or peak SUV, but their usefulness needs to be validated in clinical studies.
PERCIST
PERCIST, or Positron Emission Response Criteria in Solid Tumors, is a recent proposal to standardize metabolic response assessment to anti-tumor therapy [Citation26]. The rationale to develop such a novel standardized approach to tumor response assessment was based on the finding that the biological or metabolic prediction of tumor response during or at the end of a treatment course may be superior to using anatomical measurements based on the widely accepted Response Evaluation Criteria in Solid Tumors (RECIST, and RECIST 1.1) [Citation27]. Measurement criteria for this proposed method address most criticisms and concerns in quantization of PET images. Wahl and colleagues propose to report a SULpeak in the hottest target region. The volume measured is a sphere of 12 mm diameter, equal to a volume of 1 cm3, centered on the “hottest” area in a PET scan. For a baseline SULpeak to be considered meaningful, the value reported would need to be 1.5 times the value of a 3 cm diameter right liver lobe SUL+2SD. Owing to having been proposed most recently, no clinical data have been reported using those criteria.
Summary
Undoubtedly, measurement and quantization of tumor glucose metabolism at baseline and in follow-up is an attractive yet incompletely explored aspect of oncology care and radiation oncology follow-up specifically. At this point in time, it has become clear that baseline, pretreatment FDG uptake can yield prognostic information for some tumor types but not others. FDG uptake change in response to chemotherapy is often more dramatic and may be assessed at earlier time-points than response to radiation therapy, and is now used to prognosticate outcomes and to stratify treatment selection and intensity for select hematological malignancies. While, despite critical discussion, FDG PET is increasingly used in the process of delineating radiation therapy target volumes [Citation28–33], serial assessment of tumor response following a course of radiation therapy is still in its infancy. Ongoing and future prospective clinical trials need to clarify optimal timing of repeat FDG PET assessment, and a level of metabolic uptake reduction predictive of long-term outcomes for specific tumor types. It is actually likely that optimal timing of response assessment and prognostic level of uptake reduction may differ dramatically for different underlying pathologies. Ablative radiation treatments such as stereotactic treatment approaches in the body may yield more dramatic and earlier metabolic uptake normalization than conventionally fractionated radiation regimens. Concurrent administration of chemotherapy may also result in earlier metabolic tumor response than radiation monotherapy. Aside from correlations with pathologic tumor response, afforded in neoadjuvant treatment concepts, a more standardized approach to assessing metabolic tumor response is warranted. As such, aside from institutional preferences, the NCI consensus guidelines, as well as the recently proposed PERCIST criteria may serve in helping to design future prospective clinical trials.
Declaration of interest: The authors report no conflicts of interest. The authors alone are responsible for the content and writing of the paper.
References
- Kubota K. From tumor biology to clinical Pet: A review of positron emission tomography (PET) in oncology. Ann Nucl Med 2001;15:471–86.
- Bos R, van Der Hoeven JJ, van Der Wall E, van Der Groep P, van Diest PJ, Comans EF, . Biologic correlates of (18)fluorodeoxyglucose uptake in human breast cancer measured by positron emission tomography. J Clin Oncol 2002;20: 379–87.
- Avril N. GLUT1 expression in tissue and (18)F-FDG uptake. J Nucl Med 2004;45:930–2.
- Higashi K, Clavo AC, Wahl RL. Does FDG uptake measure proliferative activity of human cancer cells? In vitro comparison with DNA flow cytometry and tritiated thymidine uptake. J Nucl Med 1993;34:414–9.
- Haberkorn U, Strauss LG, Reisser C, Haag D, Dimitrakopoulou A, Ziegler S, . Glucose uptake, perfusion, and cell proliferation in head and neck tumors: Relation of positron emission tomography to flow cytometry. J Nucl Med 1991;32:1548–55.
- Vesselle H, Schmidt RA, Pugsley JM, Li M, Kohlmyer SG, Vallires E, . Lung cancer proliferation correlates with [F-18]fluorodeoxyglucose uptake by positron emission tomography. Clin Cancer Res 2000;6:3837–44.
- Hillner BE, Siegel BA, Liu D, Shields AF, Gareen IF, Hanna L, . Impact of positron emission tomography/computed tomography and positron emission tomography (PET) alone on expected management of patients with cancer: Initial results from the National Oncologic PET Registry. J Clin Oncol 2008;26:2155–61.
- Juweid ME, Stroobants S, Hoekstra OS, Mottaghy FM, Dietlein M, Guermazi A, . Use of positron emission tomography for response assessment of lymphoma: Consensus of the Imaging Subcommittee of International Harmonization Project in Lymphoma. J Clin Oncol 2007;25:571–8.
- Huang SC. Anatomy of SUV. Standardized uptake value. Nucl Med Biol 2000;27:643–6.
- Janssen MH, Ollers MC, van Stiphout RG, Riedl RG, van den Bogaard J, Buijsen J, . Blood glucose level normalization and accurate timing improves the accuracy of PET-based treatment response predictions in rectal cancer. Radiother Oncol 2010;95:203–8.
- Torizuka T, Clavo AC, Wahl RL. Effect of hyperglycemia on in vitro tumor uptake of tritiated FDG, thymidine, L-methionine and L-leucine. J Nucl Med 1997;383:382–6.
- Diederichs CG, Staib L, Glatting G, Beger HG, Reske SN. FDG PET: Elevated plasma glucose reduces both uptake and detection rate of pancreatic malignancies. J Nucl Med 1998;39:1030–3.
- Sugawara Y, Zasadny KR, Neuhoff AW, Wahl RL. Reevaluation of the standardized uptake value for FDG: Variations with body weight and methods for correction. Radiology 1999;213:521–5.
- Zasadny KR, Wahl RL. Standardized uptake values of normal tissues at PET with 2-[fluorine-18]-fluoro-2-deoxy-D-glucose: Variations with body weight and a method for correction. Radiology 1993;189:847.
- Nakamoto Y, Zasadny KR, Minn H, Wahl RL. Reproducibility of common semi-quantitative parameters for evaluating lung cancer glucose metabolism with positron emission tomography using 2-deoxy-2-[18F]fluoro-D-glucose. Mol Imaging Biol 2002;4:171–8.
- Minn H, Zasadny KR, Quint LE, Wahl RL. Lung cancer: Reproducibility of quantitative measurements for evaluating 2-[F-18]-fluoro-2-deoxy-D-glucose uptake at PET. Radiology 1995;196:167–73.
- Weber WA, Ziegler SI, Thödtmann R, Hanauske AR, Schwaiger M. Reproducibility of metabolic measurements in malignant tumors using FDG PET. J Nucl Med 1999;40: 1771–7.
- Nahmias C, Wahl LM. Reproducibility of standardized uptake value measurements determined by 18F-FDG PET in malignant tumors. J Nucl Med 2008;49:1804–8.
- Krak NC, Boellaard R, Hoekstra OS, Twisk JW, Hoekstra CJ, Lammertsma AA. Effects of ROI definition and reconstruction method on quantitative outcome and applicability in a response monitoring trial. Eur J Nucl Med Mol Imaging 2005;32:294–301.
- Shankar LK, Hoffman JM, Bacharach S, Graham MM, Karp J, Lammertsma AA, . Consensus recommendations for the use of 18F-FDG PET as an indicator of therapeutic response in patients in National Cancer Institute Trials. J Nucl Med 2006;47:1059–66.
- Young H, Baum R, Cremerius U, Herholz K, Hoekstra O, Lammertsma AA, . Measurement of clinical and subclinical tumour response using [18F]-fluorodeoxyglucose and positron emission tomography: Review and 1999 EORTC recommendations. European Organization for Research and Treatment of Cancer (EORTC) PET Study Group. Eur J Cancer 1999;35:1773–82.
- Henderson MA, Hoopes DJ, Fletcher JW, Lin PF, Tann M, Yiannoutsos CT, . A pilot trial of serial 18F-fluorodeoxyglucose positron emission tomography in patients with medically inoperable stage I non-small-cell lung cancer treated with hypofractionated stereotactic body radiotherapy. Int J Radiat Oncol Biol Phys 2010;76:789–95.
- Janssen MH, Ollers MC, van Stiphout RG, Buijsen J, van den Bogaard J, de Ruysscher D, . Evaluation of early metabolic responses in rectal cancer during combined radiochemotherapy or radiotherapy alone: Sequential FDG-PET-CT findings. Radiother Oncol 2010;94:151–5.
- Jingu K, Kaneta T, Nemoto K, Takeda K, Ogawa Y, Ariga H, . (18)F-fluorodeoxyglucose positron emission tomography immediately after chemoradiotherapy predicts prognosis in patients with locoregional postoperative recurrent esophageal cancer. Int J Clin Oncol 2010;15:184–90.
- Schwarz JK, Lin LL, Siegel BA, Miller TR, Grigsby PW. 18-F-fluorodeoxyglucose-positron emission tomography evaluation of early metabolic response during radiation therapy for cervical cancer. Int J Radiat Oncol Biol Phys 2008;72:1502–7.
- Wahl RL, Jacene H, Kasamon Y, Lodge MA. From RECIST to PERCIST: Evolving considerations for PET response criteria in solid tumors. J Nucl Med 2009;50(Suppl 1):122S–50S.
- Therasse P, Arbuck SG, Eisenhauer EA, Wanders J, Kaplan RS, Rubinstein L, . New guidelines to evaluate the response to treatment in solid tumors. European Organization for Research and Treatment of Cancer, National Cancer Institute of the United States, National Cancer Institute of Canada. J Natl Cancer Inst 2000;92:205–16.
- Grégoire V, Haustermans K, Geets X, Roels S, Lonneux M. PET-based treatment planning in radiotherapy: A new standard? J Nucl Med 2007;48S:68–77.
- van Baardwijk A, Baumert BG, Bosmans G, van Kroonenburgh M, Stroobants S, Gregoire V, . The current status of FDG-PET in tumour volume definition in radiotherapy treatment planning. Cancer Treat Rev 2006; 32:245–60.
- El-Bassiouni M, Ciernik IF, Davis JB, El-Attar I, Reiner B, Burger C, . [18FDG] PET-CT-based intensity-modulated radiotherapy treatment planning of head and neck cancer. Int J Radiat Oncol Biol Phys 2007;69:286–93.
- Hong R, Halama J, Bova D, Sethi A, Emami B. Correlation of PET standard uptake value and CT window-level thresholds for target delineation in CT-based radiation treatment planning. Int J Radiat Oncol Biol Phys 2007;67:720–6.
- Muijs CT, Beukema JC, Pruim J, Mul VE, Groen H, Plukker JT, . A systematic review on the role of FDG-PET/CT in tumour delineation and radiotherapy planning in patients with esophageal cancer. Radiother Oncol 2010 (in press).
- Hanna GG, Carson KJ, Lynch T, McAleese J, Cosgrove VP, Eakin RL, . (18)F-Fluorodeoxyglucose positron emission tomography/computed tomography-based radiotherapy target volume definition in non-small-cell lung cancer: Delineation by radiation oncologists vs. joint outlining with a PET radiologist? Int J Radiat Oncol Biol Phys 2010 (in press).