Abstract
Background. To appraise the potential of volumetric modulated arc therapy (VMAT, RapidArc) and proton beams to simultaneously achieve target coverage and enhanced sparing of bone tissue in the treatment of soft-tissue sarcoma with adequate target coverage. Material and methods. Ten patients presenting with soft-tissue sarcoma of the leg were collected for the study. Dose was prescribed to 66.5 Gy in 25 fractions to the planning target volume (PTV) while significant maximum dose to the bone was constrained to 50 Gy. Plans were optimised according to the RapidArc technique with 6 MV photon beams or for intensity modulated protons. RapidArc photon plans were computed with: 1) AAA; 2) Acuros XB as dose to medium; and 3) Acuros XB as dose to water. Results. All plans acceptably met the criteria of target coverage (V95% >90–95%) and bone sparing (D1cm3 <50 Gy). Significantly higher PTV dose homogeneity was found for proton plans. Near-to-maximum dose to bone was similar for RapidArc and protons, while volume receiving medium/low dose levels was minimised with protons. Similar results were obtained for the remaining normal tissue. Dose distributions calculated with the dose to water option resulted ∼5% higher than corresponding ones computed as dose to medium. Conclusion. High plan quality was demonstrated for both VMAT and proton techniques when applied to soft-tissue sarcoma.
Management of soft-tissue sarcomas requires a multidisciplinary approach which consists frequently of combined surgical intervention, radiation therapy and chemotherapy. In particular, radiotherapy can be administered in neo-adjuvant, adjuvant, or exclusive regimens. The chosen modality would depend upon the tumour location and type, the availability of adequate surgical options and the expected efficacy of chemotherapy. Limb-sparing surgery followed by adjuvant radiotherapy is considered the standard of care for most patients with soft-tissue sarcoma of the extremities [Citation1,Citation2]. Side effects of radiotherapy could be joint stiffness (also imputable to prior surgery), oedema and skin toxicity. One of the most common complications is bone fracture, with an increasing incidence to 24% in patients who had periosteal stripping and received adjuvant chemotherapy [Citation2].
The mitigation of the risk of bone fractures, while preserving clinically adequate target coverage, requires sophisticated treatment techniques. These generate homogeneous dose distributions to concave targets typically encompassing radiosensitive structures. In the case of soft-tissue sarcomas of the extremities these are the bones. Intensity modulated radiotherapy (IMRT) was investigated in the treatment of sarcomas of the legs (e.g. [Citation3]) and achieved two objectives: 1) coverage of the target; and 2) minimal involvement of the bones and the remaining surrounding normal soft tissue. In addition, with IMRT it was also possible to avoid the irradiation of the contra lateral leg.
Volumetric modulated arc therapy (VMAT), a recent evolution of IMRT might further contribute to improve the quality of treatments. In fact, VMAT is based on simultaneous optimisation of multi-leaf collimator (MLC) shapes, dose rate and gantry rotation speed [Citation4] and early studies generally demonstrated benefits in healthy tissue sparing together with good target coverage. The studies on the clinical application of VMAT focused on many indications (e.g. [Citation5,Citation6]). In particular, promising results were reported for the cases where critical structures are located very close, if not abutting, to the target. Among these shwannomas or meningiomas [Citation7,Citation8], brain irradiation with hippocampus sparing [Citation9,Citation10] and vertebral metastasis with spinal cord sparing [Citation11,Citation12] suggest that VMAT might be of interest also for soft-tissue sarcomas in the leg with bone sparing.
The radiation treatment planning of advanced techniques for soft-tissue sarcomas adjacent to bony structures implies a relevant dosimetric challenge. The accurate calculation of photon dose distribution in bone tissue is in fact complex from the physics viewpoint. The origin of the problem is the relatively high concentration of calcium in high density tissues such as cortical bone. Dose calculation algorithms should model precisely the chemical composition of materials to avoid discrepancies between calculation and actual delivery in patients [Citation13].
In parallel to the new photon techniques, more and more clinically available, challenging indications are also ideal fields of investigation for protons [Citation14,Citation15]. To strengthen the interest, there is also a moderate increase in the accessibility to treatment facilities. Typical areas of research for proton based treatments are cases where the dose to the surrounding non-target tissue shall be minimised, e.g. paediatric oncology [Citation16,Citation17]. Also sarcomas are of interest and some publications report on the possible clinical benefits of proton treatments [Citation18,Citation19].
The aim of the present report is to summarise investigations on soft-tissue sarcoma patients looking for treatment solutions with maximal bone sparing, in addition to the primary intent of minimising the risk of fractures. The first scope of the study was the comparison of two advanced techniques: VMAT in its RapidArc form, and intensity modulated proton therapy (by means of spot scanning). The second objective was the investigation of the impact, on dose distributions of RapidArc treatments, of two dose calculation algorithms differently accounting for chemical composition of materials. The two algorithms compared were the Anisotropic Analytical Algorithm (AAA) and the Acuros-XB using the two different available options of dose to medium and dose to water calculations.
Material and methods
Ten patients presenting soft-tissue sarcoma of the lower extremities (all treated with RapidArc at our institutes) were included in the study. Patients presented tumours adjacent to the femur. None of them had bone invasion. Target volume longitudinal length was in average 23.8±6.0 cm (range 15.0–37.5 cm). No metastatic spread was recorded at the time of presentation. All patients underwent limb-sparing surgery.
Planning computed tomography (CT) was acquired at the surgical wound healing with adjacent 3 or 5 mm slices. Patient positioning was supine, with immobilisation of the leg using a customised vacuum cushion to ensure daily reproducibility.
The clinical target volume (CTV) was defined as the tumour bed; the planning target volume (PTV) was defined as the CTV with an additional 1 cm margin, except in the bone direction where the bone was excluded from PTV. Toward the skin, the PTV was cropped with an internal margin of 5 mm. Femoral bone tissue was delineated as the entire long bone.
Planning technique
Two plans per patient were optimised by means of the Eclipse treatment planning system (Varian Medical System).
Photon RapidArc plans. Two partial arcs avoiding beam entrance from the contra lateral leg, collimator rotation near or equal to 90° to better spare the femur. Whenever PTV longitudinal extension exceeded 25 cm, two isocentres were used. In this case two arcs were defined per isocentre and an overlapping region of at least 2 cm between the upper and lower arcs was adopted. RapidArc plan optimisation was performed using the Progressive Resolution Optimizer PRO3 (version 10.0) [Citation20]. Photon beams of 6 MV quality from a TrueBeam linac (Varian Medical Systems, Palo Alto, CA, USA) equipped with a Millennium MLC-120 were used for the study.
Proton plans. Intensity modulated plans (IMPT) were optimised with the spot scanning technique. The basic process consisted in the simultaneous optimisation of the energy and weight of each spot inside a cloud describing organs at risk and targets [Citation21,Citation22]. Energy layers were determined to cover proximally and distally the target (plus margins). The maximum energy available was 250 MeV. Spot spacing was set to 3 mm, circular lateral target margins were set to 5 mm, proximal margin to 5 mm and distal margin to 2 mm. Two proton fields were used, whose entrance was chosen case by case according to patient and structures anatomy.
Dose calculation algorithms
For photon RapidArc plans two dose calculation algorithms were evaluated and compared: 1) the Anisotropic Analytical Algorithm, AAA [Citation23], version 10.0.28; and 2) the Acuros-XB® Advanced Dose Calculation [Citation24], version 11.0.03 (pre-clinical), using the two different available options of dose to medium and dose to water calculations [Citation25]. Both methods account for the chemical composition of the media involved in the transport mechanisms. For the latter, the dose to water was defined as the product of the dose to medium times the stopping power ratio between water and medium. Acuros-XB is an explicit solver of the Linear Boltzmann Transport Equation.
RapidArc calculations used the same optimisation result for each case, independent from the final dose calculation: during the optimisation process the tissue inhomogeneity is simply rescaled according to the medium density.
Computed MU was recorded for each dose calculation to assess any possible offset; the percentage differences between MU were reported.
For proton IMPT plans, the Proton Convolution Superposition algorithm, version 10.0, was used. Accuracy of the Eclipse proton calculation algorithm is discussed for example by the group of MD Anderson Cancer Center [Citation26–28], pointing to a difference between calculated and measured point dose for prostate treatment of 0.0±0.7% and a γ-index for 2D measurements of 96.2±2.6% with 3 mm and 3% criteria.
All final dose calculations were performed with a 2.5 mm grid size and individually normalised to the mean dose to PTV. This allows the detection of differences arising from the pure dose calculation algorithm and its inhomogeneity.
Dose prescription and planning objectives
Treatment dose was prescribed to 66.5 Gy in 25 fractions (2.66 Gy/fraction) to the mean PTV. For proton plans the dose is considered as CGE (Cobalt Gy Equivalent, i.e. including the proton Relative Biological Effectiveness of 1.1).
The following planning objectives were used for all plans: 1) PTV: V95% >95% (95% of the PTV should be covered by at least 95% of the prescribed dose). This could be lowered to V95% >90% when large fractions of the PTV surface abutted directly against the bone; and 2) Bone: D1cm3 <50 Gy (doses exceeding 50 Gy should be delivered to a volume smaller than 1 cm3) to minimise the risk of radiation induced fractures [Citation29].
Plan evaluation
Plan evaluation was assessed by dose-volume histograms (DVH) analysis. For PTV the following parameters were studied: Vx with x =90, 95, 110%, Dy with y =2, 98% (D2% and D98% can be seen as maximum and minimum significant doses), standard deviation as index of dose homogeneity. For bones the analysed parameters were D1cm3, D2cm3, Dmax and Vx with x =35, 40, 45, 50 Gy; mean value is reported as a parameter for whole bone irradiation. No other specific organs at risk were analysed. The normal healthy tissue was defined as the entire CT dataset excluding PTV. The following parameters were recorded for it: the low dose bath: V5Gy; the hot spots: V50Gy and V60Gy and the dose integral (defined as the integral of the dose received by a structure with respect to its volume). Mean values (and standard deviations) of the parameters were recorded averaging over the 10 patients. Mean DVHs were built, averaging the relative volumes over all the cases for each dose bin in the histogram.
The inter-patient variability, given by the standard deviation of the mean values of the various parameters reported was forcedly large. This was because of the large variations in the size of the PTVs and in their mutual position with respect to the bone. Some of the reported data should hence be read as relative differences between the techniques or algorithms, more than as absolute values.
Significances were evaluated according to the non-parametric Fisher's sign test.
Results
A typical arc arrangement for the VMAT plan and field arrangement for the proton plan is shown in . this corresponds to the dose distribution presented in .
Figure 1. Arc and beam arrangement for two typical cases (A and B): RapidArc on the left, proton plans on the right.
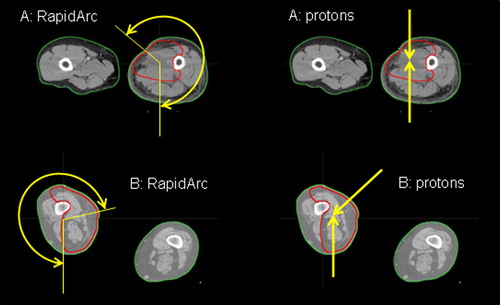
Figure 2. Isodose distributions. First row: RapidArc photon plan, 6 ×, dose to medium calculation; second row: proton plan.
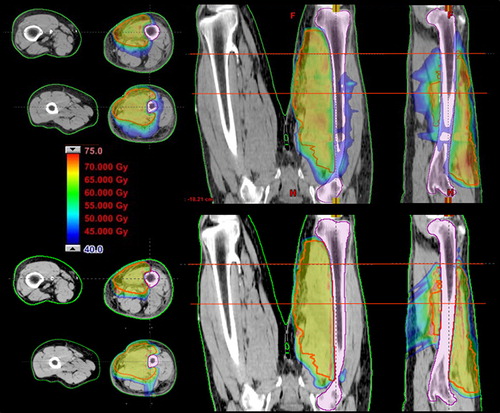
VMAT and proton plan comparison
PTV coverage was within acceptability criteria for all photon and proton plans; the difference in PTV dose distribution between proton and photon plans is clear from the average DVH in . Dose data summarised in present a significant better coverage at 95% of the prescription dose (V95% about 4% higher for protons, p <0.05), in favour of protons with respect to RapidArc photons. Significances were also found for parameters related to dose homogeneity, particularly in terms of the parameter Standard Deviation inside PTV (2.3 Gy for protons, ∼3.0 Gy for photons, p <0.05); minimum dose as D98% (about 2 Gy higher for protons) was conversely not significant.
Figure 3. Average DVHs for PTV and bone for all plans: RapidArc (AAA, Acuros XB dose to medium M, Acuros XB dose to water W), protons. Data refer to plans individually normalised to mean PTV.
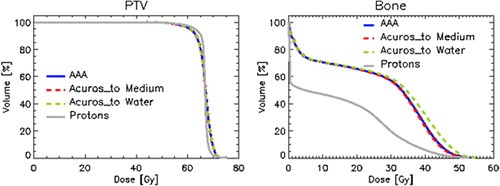
Table I. Statistics summary. For each parameter the mean ± SD over all the 10 plans is reported.
The bone maximum dose objective of 50 Gy to 1 cm3 was met in all cases, with no significant differences (with the exception of photons with dose to water calculation as pointed out below). The largest difference between proton and photon plans in bone tissue is reflected in the medium/low doses, e.g. the V35Gy and V40Gy (p <0.05).
For healthy tissue, the low dose bath was minimised with protons, and the dose integral was about halved with respect to RapidArc plans. On the contrary, no large differences in hot spots as V50Gy (p <0.05) and V60Gy were recorded.
Photon dose calculation comparisons
No clear differences were found in PTV dose distributions between AAA and Acuros XB in both dose to medium and dose to water options.
Dose calculation in bone tissue presented no significant differences between AAA and Acuros XB values for dose to medium option. Bone photon dose was conversely in general about 5% higher when computed as dose to water, for which algorithms all DVH parameters presented highly significant (p <<0.01) differences with respect to AAA or dose to medium Acuros XB.
The calculated MU differences between plans are summarised in . The effect of dose calculation algorithm is shown as a significant lower MU value computed by AAA (1.8% in average) with respect to Acuros XB dose calculations in medium (smaller difference with respect to dose to water). The MU difference between dose to water and dose to medium calculations was shown to be small and not significant.
Table II. MU summary as percentage difference between plans.
Discussion
In the present study two advanced techniques were analysed: VMAT with photons and intensity modulated protons with spot scanning. These were applied to soft-tissue sarcoma cases. In particular, for VMAT dose distributions, AAA and Acuros XB dose calculation algorithms have been evaluated and compared focussing on possible differences arising from the usage of dose to medium or dose to water approaches.
To achieve local control in postoperative soft-tissue sarcoma of the extremities, total prescription dose should be at least 66 Gy. With such high doses, bone fracture probability increases to about 10% with respect to ∼2% for patients receiving 50 Gy to PTV [Citation1]. It is then clear that sophisticated techniques like intensity modulation should be applied to manage the conflicting endpoints. Many groups studied the feasibility of using IMRT techniques [Citation30], showing the dosimetrical and technical possibility of good local control without compromising the neighbouring bones. These suggested that the usage of advanced techniques would be beneficial mostly for patients with the PTV embedding the bone. It was therefore hypothesised that RapidArc or proton therapy would be beneficial to reduce involvement of the bone structure to acceptable dose levels, minimising the bone fracture risk. At the same time, with these techniques it should be possible to avoid any downscaling of the prescription dose, negatively impacting on the probability of local control.
In particular, the usage of protons with the physical characteristics of the distal fall off after the Bragg peak, showed very interesting clinical results in other clinical sites [Citation16]. The advantages of protons are, e.g. described in the paper from Kozak et al. [Citation17], where a comparison between IMRT and proton therapy is presented for paediatric parameningeal rhabdomyosarcoma (malignancy of the same group of soft-tissue sarcoma) to quantify the impact of protons on normal structures irradiation. In their paper the contra lateral irradiation was strongly reduced with protons if compared to photon IMRT (a factor two to four reduction in the mean dose in most of the structures). This range of dose reduction is similar to what was found in the present study, where the medium/low dose levels in the surrounding normal tissue was reduced by a factor up to 3 with IMPT.
To better understand the mutual geometrical position of PTV and bone in conjunction with the required objectives of PTV dose prescription of 66.5 Gy and maximum significant dose to bone of 50 Gy, the ratio between the surface of bone adjacent to PTV and the bone length included in this area was recorded. The ratio represented the lateral spread of the bone adjacent to the PTV. The mean value was 4.2±3.0 cm with a maximum of 9.4 cm (in a case where the PTV was all around the femur). As a rule of thumb, for cases presenting ratios larger than 1–2 cm, the bone convexity would suggest the usage of advanced technique in place of conventional 3DCRT. For ratios of 1–2 cm, the bone volume inside the concavity could be only 3–1%, respectively, of the entire bone volume. One concern for concave targets adjacent to organs at risk is the dose delivered to the target near to the PTV-organ interface. The mean dose delivered to the PTV region adjacent to bone (4 mm thick) has been evaluated in our study, resulting in 56.8 Gy for photon cases, 58.9 Gy for proton plans. The better dose gradient for proton beams could suggest the usage of protons instead of even advanced photon techniques in cases having rather wide ratio between interface area and its length. Considering that the PTV volume of 4 mm thickness toward bone was in average 40 cm3 (range 2–74 cm3), the PTV underdosage necessary to keep the maximum bone dose below the objective of 50 Gy to 1 cm3 has to be evaluated in terms of clinical meaning of such an underdosage. Summarising, the clinical benefit in using RapidArc or proton techniques could be linked to the extension and concavity of the PTV-femur interface.
Concerning the photon dose calculation issues, AAA did not present big differences in relative dose distributions with respect to the more advanced Acuros XB with dose to medium calculations. An overestimation of the dose, due to MU calculation, of about 2% was pointed out. Considering Acuros XB calculations in the presence of inhomogeneities [Citation31–33,Citation25] as more accurate than AAA, a possible underdosage of that amount could be delivered using AAA algorithm. However, this difference should have no visible clinical impact.
A significant difference was conversely found for dose to water calculations with respect to the dose to medium option. This was due to the stopping power ratio between cortical bone and water, being of about 1.11 [Citation34]. The difference between dose to medium and dose to water was however only half of what was expected by the simple product of the dose by the stopping power. To consider the amount of femur having cortical bone density, i.e. less than half of the total femur volume, while the remaining part of the bone is referred as cartilage composition (soft bone), with a stopping power ratio to water of 1.035 [Citation34]. Those considerations can explain the reason for the difference found in this study of about 5% in the dose to bone when dose to water instead of dose to medium is used. The difference between dose to water and dose to medium calculations for Acuros XB compared to AAA computations is also reported in phantom studies [Citation25,Citation31–33], showing results very similar to what was reported in the present clinical planning study.
The non-negligible difference between the dose to medium and dose to water calculations for bony structures highlights the issue of what should be preferably used in clinical practice. ICRU 83 [Citation35] recommends recording dose to water more than dose to medium because dose to water more closely reflects the cellular composition. The dose to medium calculations include the elemental chemical composition of the specific tissue (according to published data as ICRP 23 [Citation36] in the Acuros XB case), yielding the absorbed dose to such a medium (energy deposited in the medium per mass unit) that better translate the actual physics of the interaction in the specific medium using, e.g. better approximations of the cross sections. The step to convert the dose to medium into dose to water through the stopping power ratio between the two materials does not cancel the actual elemental composition of the medium crossed by the radiation, as no change is applied (in terms of cross sections) from the supposed specific tissue composition of the medium to a more related cellular composition. On the other hand, the additional reason of the ICRU suggestion in favour of the dose to water was related to the fact that it should better reflect the past experience in clinical radiotherapy that is based on dose to water calculations. Old dose calculation algorithms, using analytical models, determine dose as in water medium, rescaled for the specific density. Such a dose for cortical bone structures, imperfectly calculated and not including the tissue composition, are more similar in value to dose to medium calculations [Citation25], while a larger difference is found with respect to the dose to water. Summarising, only the understanding of the used calculation model would lead the user to consciously choose one of the two modalities: the dose to water as recommended by ICRU 83, or the dose to medium which better reflects the physical dose to the specific tissue material.
In conclusion, the present study showed the good planning results reached by both RapidArc (with different dose calculation algorithms) and proton therapy for the management of soft-tissue sarcoma of the lower extremities, yielding good and homogeneous target coverage to 66.5 Gy prescription, while keeping the maximum significant dose to the bone below the 50 Gy hence minimising the risk of bone fractures. Protons can give better medium/low dose to surrounding tissues and better gradient at the target to the bone interface.
Declaration of interest: L. Cozzi acts as Scientific Advisor to Varian Medical Systems and is Head of Research and Technological Development at the Oncology Institute of Southern Switzerland, Bellinzona. The authors report no other conflict of interest.
References
- Mundt AJ, Awan A, Sibley GS, Simon M, Rubin SJ, Samuels B, . Conservative surgery and adjuvant radiation therapy in the management of adult soft tissue sarcoma of the extremities: Clinical and radiobiological results. Int J Radiat Oncol Biol Phys 1995;32:977–85.
- Lin PP, Schupak KD, Boland PJ, Brennan MF, Healey JH. Pathologic femoral fracture after periosteal excision and radiation for the treatment of soft tissue sarcoma. Cancer 1998;82:2356–65.
- Hong L, Alektiar KM, Hunt M, Venkatraman E, Leibel SA. Intensity modulated radiotherapy for soft tissue sarcoma of the thigh. Int J Radiat Oncol Biol Phys 2004;59:752–9.
- Otto K. Volumetric modulated arc therapy: IMRT in a single arc. Med Phys 2008;35:310–7.
- Lagerwaard FJ, van der Hoorn EA, Verbakel WF, Haasbeek CJ, Slotman BJ, Senan S. Whole-brain radiotherapy with simultaneous integrated boost to multiple brain metastases using volumetric modulated arc therapy. Int J Radiat Oncol Biol Phys 2009;75:253–9.
- Bignardi M, Navarria P, Mancosu P, Cozzi L, Fogliata A, Tozzi A, . Clinical outcome of hypofractionated stereotactic radiotherapy for abdominal lymph node metastases. Int J Radiat Oncol Biol Phys 2011;81:831–8.
- Fogliata A, Clivio A, Nicolini G, Vanetti E, Cozzi L. Intensity modulation with photons for benign intracranial tumours: A planning comparison of volumetric single arc, helical arc and fixed gantry techniques. Radiother Oncol 2009;89:254–62.
- Lagerwaard FJ, Meijer OW, van der Hoorn EAP, Verbakel WF, Slotman BJ, Senan S. Volumetric modulated arc radiotherapy for vestibular shwannomas. Int J Radiat Oncol Biol Phys 2009;74:610–5.
- Hsu F, Carolan H, Nichol A, Cao F, Nuraney N, Lee R, . Whole brain radiotherapy with hippocampal avoidance and simultaneous integrated boost for 1-3 brain metastases: A feasibility study using volumetric modulated arc therapy. Int J Radiat Oncol Biol Phys 2010;76:1480–5.
- Wiebe E, Cozzi L, Yartsev S, Fogliata A, Clivio A, Vanetti E, . Hippocampus avoidance with fan beam and volumetric arc radiotherapy for base of skull tumours. J Radiother Pract 2010;9:87–98.
- Wu QJ, Yoo S, Kirkpatrick JP, Thongphiew D, Yin FF. Volumetric arc intensity-modulated therapy for spine body radiotherapy: Comparison with static intensity-modulated treatment. Int J Radiat Oncol Biol Phys 2009;75:1596–604.
- Mancosu P, Navarria P, Bignardi M, Cozzi L, Fogliata A, Lattuada P, . Re-irradiation of metastatic spinal cord compression: A feasibility study by volumetric-modulated arc radiotherapy for in-field recurrence creating a dosimetric hole on the central canal. Radiother Oncol 2010;94:67–70.
- Walters BR, Kramer R, Kawrakow I. Dose to medium versus dose to water as an estimator of dose to sensitive skeletal tissue. Phys Med Biol 2010;55:4535–46.
- Ares C, Hug EB, Lomax AJ, Bolsi A, Timmermann B, Rutz HP, . Effectiveness and safety of spot scanning proton radiation therapy for chordomas and chondrosarcomas of the skull base: First long-term report. Int J Radiat Oncol Biol Phys 2009;75:1111–8.
- Song WY, Huh SN, Liang Y, White G, Nichols RC, Watkins WT, . Dosimetric comparison study between intensity modulated radiation therapy and three-dimensional conformal proton therapy for pelvic bone marrow sparing in the treatment of cervical cancer. J Appl Clin Med Phys 2010;11: 3255.
- Timmermann B, Schuck A, Niggli F, Weiss M, Lomax AJ, Pedroni E, . Spot scanning proton therapy for malignant soft tissue tumors in childhood: First experiences at the Paul Scherrer Institute. Int J Radiat Oncol Biol Phys 2007;67: 497–504.
- Kozak KR, Adams J, Krejcarek SJ, Tarbell NJ, Yock TI. A dosimetric comparison of proton and intensity-modulated photon radiotherapy for pediatric parameningeal rhabdomyosarcomas. Int J Radiat Oncol Biol Phys 2009;74:179–86.
- DeLaney TF, Trofimov AV, Engelsaman M, Suit HD. Advanced-technology radiation therapy in the management of bone and soft tissue sarcomas. Cancer Control 2005;12: 27–35.
- Weber DC, Rutz HP, Bosi A, Pedroni E, Coray A, Jermann M, . Spot scanning proton therapy in the curative treatment of adult patients with sarcoma: The Paul Scherrer Institute experience. Int J Radiat Oncol Biol Phys 2007;69: 865–71.
- Vanetti E, Nicolini G, Nord J, Jarkko P, Clivio A, Fogliata A, . On the role of the optimization algorithm of Rapid Arc® volumetric modulated arc therapy on plan quality and efficiency. Med Phys 2011;38:5844–56.
- Ulmer W. Theoretical aspects of energy range relations, stopping power and energy straggling of protons. Radiat Phys Chem 2007;76:1089–107.
- Schaffner B. Proton dose calculation based on in-air fluence measurements. Phys Med Biol 2008;53:1545–62.
- Tillikainen L, Helminen H, Torsti T, Siljamäki S, Alakuijala J, Pyyry J, . A 3D pencil-beam-based superposition algorithm for photon dose calculation in heterogeneous media. Phys Med Biol 2008;53:3821–39.
- Vassiliev O, Wareing T, McGhee J, Failla G, Salehpour M, Mourtada F. Validation of a new grid based Blotzmann equation solver for dose calculation in radiotherapy with photon beams. Phys Med Biol 2010;55:581–98.
- Fogliata A, Nicolini G, Clivio A, Vanetti E, Cozzi L. Dosimetric evaluation of Acuros XB Advanced Dose Calculation algorithm in heterogeneous media. Radiat Oncol 2011;6:82.
- Gillin MT, Sahoo N, Bues M, Ciangaru G, Sawakuchi G, Poenisch F, . Commissioning of the discrete spot scanning proton beam delivery system at the University of Texas M.D. Anderson Cancer Center, Proton Therapy Center, Houston. Med Phys 2010;37:154–64.
- Arjomandy B, Sahoo N, Ciangaru G, Zhu R, Song X, Gillin M. Verification of patient-specific dose distributions in proton therapy using a commercial two-dimensional ion chamber array. Med Phys 2010;37:5831–7.
- Zhu R, Poenisch F, Song X, Johnson JL, Ciangaru G, Taylor B, . Patient-specific quality assurance for prostate cancer patients receiving spot scanning proton therapy using single-field uniform dose. Int J Radiat Oncol Biol Phys 2011;81: 552–9.
- Holt GE, Griffin AM, Pintilie M, Wunder JS, Catton C, O'Sullivan B, . Fractures following radiotherapy and limb salvage surgery for lower extremity soft tissue sarcomas. A comparison of high-dose and low-dose radiotherapy. J Bone Joint Surg Am 2005;87:315–9.
- Stewart AJ, Lee YK, Saran FH. Comparison of conventional radiotherapy and intensity modulated radiotherapy for post operative radiotherapy for primary extremity soft tissue sarcoma. Radiother Oncol 2009;93:125–30.
- Bush K, Gagne IM, Zavgorodni S, Ansbacher W, Beckham W. Dosimetric validation of Acuros XB with Monte Carlo methods for photon dose calculations. Med Phys 2011;38:2208–21.
- Han T, Mikell JK, Salehpour M, Mourtada F. Dosimetric comparison of Acuros XB deterministic radiation transport method with Monte Carlo and model-based convolution methods in heterogeneous media. Med Phys 2011;38: 2651–64.
- Hoffmann L, Jørgensen MBK, Muren LP, Petersen JBB. Clinical validation of the Acuros XB photon dose calculation algorithm, a grid-based Boltzmann equation solver. Acta Oncol (in press)
- Siebers JV, Keall PJ, Nahum AE, Mohan R. Converting absorbed dose to medium to absorbed dose to water for Monte Carlo based photon beam dose calculations. Phys Med Biol 2000;45:983–95.
- ICRU Report 83, Prescribing recording and reporting photon beam intensity modulated radiation therapy (IMRT). Oxford: Oxford University Press; 2010.
- ICRP Report 23. Reference Man: Anatomical, physiological and metabolic characteristics. New York: International Commission on Radiological Protection ICRP; 1975.