Abstract
Background and Purpose. Few studies have explored the potential clinical advantages of dose escalation and integrated boosts for patients with non-resectable locally advanced rectal cancer. The possibility of escalating dose to non-resectable regions in these patients was the aim of this study. Patients and methods. Seven patients with locally very advanced rectal tumours (sacrum overgrowth or growth into pelvic side walls) were evaluated. Intensity modulated photon and pencil beam scanning proton plans with simultaneously integrated boosts (45 Gy to elective lymph nodes, 50 Gy to tumour and 62.5 Gy to boost area in 25 fractions) were compared. Results. Target coverage was achieved with both photon and proton plans. Estimated risks of acute side effects put the two patients with the largest tumours at unacceptable risk for intestinal toxicity, regardless of modality. The remaining five patients had beneficial sparing of dose to the small intestine with protons. Conclusions. Adding boost to areas where rectal tumours infiltrate adjacent non-resectable organs is an attractive option which appears possible using both photon and proton irradiation. Proton plans reduced dose to organs at risk. Integrated peripheral boosts should be considered more frequently in these very advanced tumours.
Colorectal cancer is a common malignancy worldwide, and locally advanced rectal cancers (LARC) pose special difficulties for physicians. Especially challenging are those tumours that are so advanced at presentation that surgery is not an up-front option due to high risk of non-radical surgery or local failure. Surgery may not be possible even after preoperative chemoradiotherapy (CRT) due to overgrowth to structures difficult to resect. Complete eradication of all tumour cells with CRT is rarely seen in these frequently large tumours [Citation1].
CRT given preoperatively is considered state- of-the-art treatment for patients with LARC. There is major disagreement in the literature on what is considered LARC. The terminology of the European Rectal Cancer Consensus (EURECA) project [Citation2,Citation3] is used, dividing rectal cancer into three groups: early, intermediate and locally advanced (also designated “T4 non-resectable”). The terms “good”, “bad” and “ugly” have also been used for these groups [Citation4]. The intermediate group is popularly termed “locally advanced” and is the group included in most recent trials evaluating different CRT protocols.
The LARC group comprises about 10–15% of all newly diagnosed rectal cancers in Sweden [Citation5]. Local control can be achieved in about 82% of the LARC patients provided they are treated with preoperative CRT consisting of 5-fluorouracil and 50 Gy followed by attempts at radical surgery [Citation6]. A subgroup of these patients has very advanced tumours with overgrowth to, e.g. the lateral pelvic side walls and upper part of the sacrum. Such tumours cannot be resected without mutilating surgery such as hemipelvectomy. These patients, usually excluded from clinical trials, may potentially benefit from increased radiation dose to areas that cannot be resected.
Studies have indicated that increased radiotherapy (RT)-dose is advantageous [Citation7], and some groups have tried to find strategies for giving total doses of 66 Gy in 2 Gy fractions or more without compromising organs at risk (OAR) [Citation8–11]. The pelvis and perineum are especially difficult areas to irradiate because of the proximity of the urinary bladder, small intestine and internal genital organs, and risk for both acute and late toxicity must be considered. The irradiated volume of small bowel may be reduced by creating a conformal dose distribution that tightly matches the usually horseshoe-shaped planning-target volume (PTV) of rectal cancer, using techniques such as intensity-modulated RT [Citation12–14]. Proton therapy has the potential to become an attractive option when there is a need for higher RT-doses in the pelvis. Protons have special physical characteristics that allow the dose to be delivered precisely in the culmination of their so-called Bragg peak, after which the dose is close to zero.
The aim of this study was to explore the possibility of dose escalation to non-resectable regions in patients with exceptionally locally advanced rectal tumours without increasing toxicity above a level which is acceptable. The intent of dose escalation is firstly to increase the chance that the tumour becomes resectable and secondly to increase the chance of local control if the tumour remains non-resectable.
Patients and methods
Patients
Between November 2007 and December 2009, 77 consecutive patients diagnosed with LARC at two Swedish university hospitals (Karolinska in Stockholm and Akademiska in Uppsala) were included in a positron emission tomography (PET)-delineation study [Citation15]. LARC was defined as clinical stage T4 with overgrowth to adjacent organs or structures (cT4a, American Joint Committee on Cancer (AJCC) TNM5), cT3 with mesorectal fascia involvement (MRF+) or any TN+ with lateral node involvement. Out of 77 patients, seven had locally very advanced tumours (all cT4a) with overgrowth to non-resectable structures (pelvic side walls including lumbo-sacral plexus or sacrum above S3) and were therefore selected for this dose escalation study. All evaluations were done at a multidisciplinary team conference. Patient characteristics are displayed in .
Table I. Patient characteristics.
All patients were planned for treatment following the standard guidelines of the actual institution, independent of inclusion in this study. Some patients went through preoperative CRT (50.4 Gy to the tumour with concomitant capecitabine, 45 Gy to non-involved lymph nodes at risk) and some started up-front combination chemotherapy because of synchronous metastases.
Image acquisition
Magnetic resonance imaging (MRI) with 1.5 Tesla was performed at the referring hospital. All MRI protocols included at least transversal and sagittal T2-weighted fast spin-echo sequences and transversal T1-weighted images of the pelvic area. Whole-body FDG (2-18F-fluoro-2-D-deoxyglucose)-PET/computed tomography (CT) scanning was performed with a Siemens Biograph 64 (Siemens Medical Solutions) or a GE Discovery STE (GE Medical Systems). All PET/CT datasets included transversal, sagittal, and coronal reformations of the PET, CT and fused PET/CT images with a slice thickness from 3.75 to 5 mm.
Definition of target volumes
Target volume definition and OARs were outlined by the same radiation oncologist on the same PET/CT-scan for both photon and proton planning.
Gross tumour volume (GTV) was defined as the gross extent of tumour as shown on MRI and PET/CT. Next, regions with tumour overgrowth to S1-S2-vertebrae, or into the lateral pelvic side walls outside of the MRF were identified, and delineated as GTV-boost within a peripheral part of the GTV-tumour. Clinical target volume (CTV)-tumour was defined as GTV-tumour plus 10–15 mm 3D margin with manual readjustment for bony structures and MRF where necessary. CTV-regional was delineated according to Radiation Therapy Oncology Group-guidelines [Citation16] and also included CTV-tumour. PTVs were automatically generated by adding a 7 mm margin to the GTV-boost (PTV-boost), the CTV-tumour (PTV-tumour) and the CTV-regional (PTV-regional).
OARs were defined as body (lower abdomen), femoral head and neck, sacral nerve roots from L5 to S2, S1 to S5 vertebrae, urinary bladder and intestinal cavity. The latter was defined according to Sanguineti and Fiorino [Citation17,Citation18]. Fiorino et al. studied small bowel toxicity in clinically localised prostate cancer patients who received whole pelvic irradiation both with 3D conformal RT (3DCRT) and IMRT. The dose delivered to the pelvic lymph nodes was at least 50.4 Gy in 1.8 Gy fractions, much like the irradiation in rectal cancer when given with a fluoropyrimidine. The intestinal cavity delineation was found to be robust with respect to defining bowel movement. We thus prefer to use intestinal cavity rather than small bowel loops as it is difficult to account for intestinal movement. Intestinal cavity was contoured superiorly from 1 cm above the most cranial part of PTV, anteriorly to the abdominal/pelvic anterior wall, laterally to the pelvic wall and inferiorly to the rectum and urinary bladder.
Treatment planning
Photon and proton plans with simultaneously integrated boost were optimised to deliver 45 Gy to the lymph nodes, 50 Gy to the tumour and 62.5 Gy to the boost-areas in 25 fractions.
The proton beam was of spot scanning type; with energy range 70–235 MeV, which gives a range of the protons from 4 to 30 cm in water. The distance between energy layers was 0.5 cm and the distance between spots was 0.5 cm in a hexagonal pattern. Two posterior beams with gantry angles 140° and 220° were used in all proton plans (). In two of the plans an additional beam at 180° with a range shifter was used due to a shallow target. Single field uniform doses were used to increase robustness. The gantry angles were chosen to minimise the volume of irradiated intestinal cavity and the overall integral dose.
Figure 1. Beam orientation in proton plan (top) and starting angles for photon arcs (bottom) for patient no. 3.
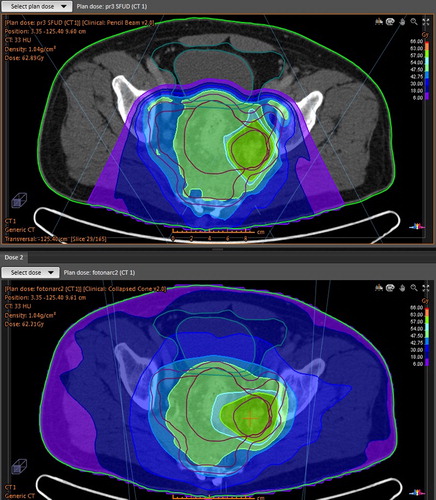
Photon plans were made with volume modulated arc therapy (VMAT) technique using two arcs with 90 control points each, i.e. 4° between control points. The collimator was positioned at 15° and had a maximum field size 40 cm × 40 cm with the leaves 1 cm wide at isocentre. Interdigitising was not allowed.
The planning aim was to cover PTV with 95% of the prescribed dose whilst minimising the dose outside PTV as recommended by Fiorino et al. [Citation18]. The relative biological effect of the proton beam was assumed to be 1.1 uniformly. Boost doses were 2.5 Gy per fraction, which is slightly higher than other groups have given. This dose was chosen as controlling tumour growth quickly in these advanced cases was important. The total dose to PTV-boost of 62.5 Gy corresponds to 68.8 Gy if α/β = 3 or 65.1 Gy if α/β = 10 for the same BED in 2 Gy-fractions (no time correction). Conformity index was defined as the patient volume that received at least 95% of the target dose divided by the total target volume.
A new treatment planning system was used, Raystation [Citation19]. In the system several linear accelerators and proton machines were available. For the photon plans an Elekta accelerator was used and for the proton plans an IBA machine.
To estimate the robustness of the plans for changes in intestinal gas filling, the gas in the PTV was delineated for all patients. Both proton and photon plans were then recalculated with the gas replaced by a water equivalent material.
Results
The target criteria were met by all plans. Proton and photon plans reached equally good conformity to the three PTVs ().
Table II. Planning target volume (PTV) coverage and doses (VMAT stands for photon plan and P+ stands for proton plan).
Intestinal cavity volumes (mean 913 cm3, range 615–1157 cm3) and doses are shown in and the DVHs in . The critical doses and volumes for acute grade 2–3 toxicity from RT alone and CRT are included in the figure.
Table III. Organs at risk (OAR) volumes and doses (VMAT stands for photon plan and P+ stands for proton plan).
Figure 2. Dose volume histogram (DVH) for intestinal cavity for protons (top) and photons (bottom) with critical volumes recommended by Fiorino et al. (18) and Roeske et al. (30) mapped.
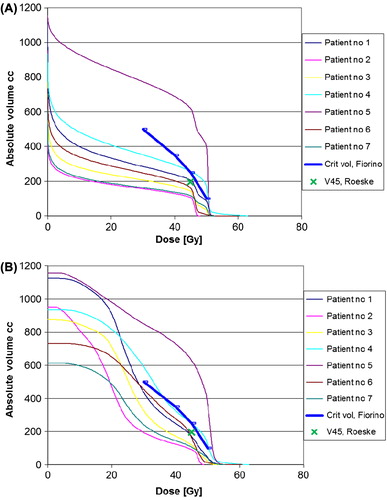
One patient (no. 5, with the largest tumour and inguinal metastases, where PTV included more than 40% of the intestinal cavity) had unacceptably high doses to the intestinal cavity at all measured levels. Patient no. 4, with the GTV-boost adjacent to the intestinal cavity, also had high doses to the intestinal cavity with V45 around 250 cm3 for both plans and above maximum acceptable dose in the photon plan with 53.6 Gy. The rest of the patients’ intestinal cavity doses were acceptable, with proton plans generally being superior for doses below 45 Gy.
In a separate calculation the boost was removed in two patients to see if intestinal cavity doses were substantially reduced. Removing the boost lowered the small bowel dose slightly in one patient whose target was close to the intestinal cavity whereas it had no effect in the other patient ().
Figure 3. Dose volume histogram (DVH) for intestinal cavity showing mean values for patient 3 and 6, critical volumes recommended by Fiorino et al. (18) and Roeske et al. (30) mapped.
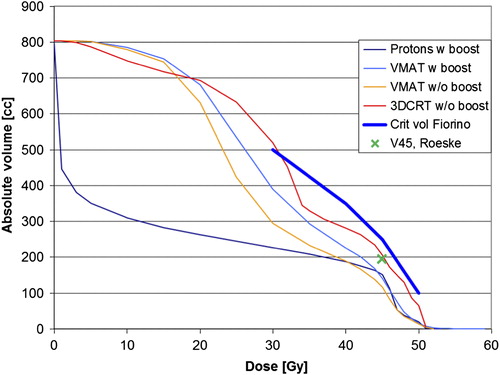
The volumes and doses to the different OARs are shown in . The proton plans spared the bladder better than the photon plans; with a maximum of 56.3 Gy for the photon plan in patient no. 5. Advantages of protons were seen also for the femoral heads with a maximum dose of 48.5 Gy for the photon plan in patient no. 5. The integral doses (i.e. deposited energy) to the body, with PTV-regions excluded, estimated by multiplying the average dose with the volume were 109 J for proton plans and 222 J for photon plans.
Nerve roots and S1-S5 vertebrae doses were higher in patients with growth into S1-S2, which was expected, with a maximum of 61.7 Gy and 63.1 Gy, respectively. Photons gave a slight advantage compared to protons in these areas.
The mean volume of intestinal gas in the CT planning studies was 19 cm3, range 2–35 cm3. When the gas was replaced by water in the treatment planning system the targets were, depending on the size and location of the replaced gas, no longer covered with the 95%-isodose line in all proton plans. Photon plans seems to be more robust in this sense (no statistical differences) ().
Figure 4. Differences in target coverage – intestinal gas influence in patient no 3. Photon plan to the left and proton plan to the right. Upper row: Dose distribution in the planning CT with gas present. Lower row: Dose distribution when gas is replaced by water in 25 of 25 fractions. It is the distance the protons have to cross in the gas cavity that is important for the robustness of the proton plan.
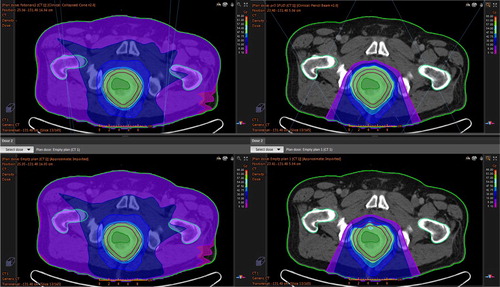
Discussion
Fortunately, the patients used in this study are rarely seen. In our original study material of 77 patients with LARC (all “ugly”), these “ugly-ugly” (with overgrowth to non-resectable structures) constituted about 10% of the patients. In the randomised trial with 209 LARC patients [Citation6], between 10% and 15% had “ugly-ugly” tumours at a retrospective evaluation of the patient cohort. Even though the patients are rare, the consequences can be dire if local control is not achieved.
While it is perfectly valid in many tumour types where RT is the sole or main treatment to boost cellular or hypoxic volumes, usually somewhere in the centre of the tumour, this is not particularly relevant when the main tumour is removed by surgery, as is the case in rectal cancer. Rather, the purpose of RT is to eradicate all cancer cells in the periphery, and not primarily those which are later removed by surgery. Thus, if a boost is required to further improve local control, it should be directed against a critical volume not (easily) removed surgically. In rectal tumours growing extensively into the prostate, uterus, urinary bladder or levators (cT4b according to TNM7), adequate removal of the infiltrated organs will result in local control. In order to avoid a pelvic exenteration (usually resulting in two stomas), a boost to that particular organ would be advantageous, alone or together with partial resection of the infiltrated organ if the infiltration is not too extensive. In the patients included in this study, surgery would likely have resulted in hemipelvectomy, together with removal of the organs in the pelvis, if it would even have been possible. An alternative would be to boost intraoperatively the volume containing tumour cells not possible to resect by an electron boost (IORT) or using brachytherapy [Citation20]. Neither of these techniques is “evidence-based” [Citation21] and none are widely practiced in Sweden.
In this study we report that for the seven patients with the most locally advanced rectal cancers seen during a recent two-year period in the Stockholm-Uppsala regions, both photons and protons could have been used for the treatment including the peripheral boost. Protons gave similar coverage of treatment target whilst sparing the small intestine for five of seven patients. The remaining two patients had very large tumour volumes and their respective risk of acute treatment toxicity was high regardless of whether photons or protons had been used. It must be emphasised that the cases included in this model study are more extensive than most tumours that are at presentation classified as cT4.
Since the 1990s, efforts have been made to explore if protons can be utilised in clinical RT of patients with rectal cancer [Citation22–25]. One problem with protons as a treatment modality in rectal cancer is the changeability of the target volume due to internal organ motion, organ filling and bowels containing gas. The relative biological effectiveness at the end of the Bragg peak extends the effective range of the protons [Citation26] which in the case of intestinal gas means that the distal edge of the Bragg peak will be located in the bowels and increase the risk of toxicity. The finite range of protons makes the proton plans more sensitive than photon plans if the density distribution in the patient changes from that of the planning CT. Gas in the bowels will not be present at the same location, or with the same volume, in all 25 fractions. We cannot exclude the possibility that a small degradation of the target coverage could occur. This scenario should be investigated in more detail to assess its likeliness. With this uncertainty, it is possible that further research should be done to evaluate if interventions such as placing a catheter in the rectum, or even considering a colostomy for these patients, will translate into better treatment effects.
Severe diarrhoea and other gastrointestinal toxicity can be a cause of undesired breaks or early termination of CRT. Several authors have reported toxicity of the small bowel at the low to medium dose ranges 5–30 Gy [Citation27–29]. Derived models to calculate the probability for severe diarrhoea involve large uncertainties [Citation24,Citation28] but can give a hint of the complications to expect. That it is impossible to predict intestinal movement adds to this difficulty. Another problem when using models to calculate toxicity risks is that they do not take into account the additive and sometimes radio-sensitising effect of chemotherapy. In Uppsala we are aiming at limiting the doses to the small bowel as described by Fiorino et al. [Citation18] and Roeske et al. [Citation30], which is also recommended in the QUANTEC rapport [Citation31]. Fiorino et al. find a clear impact of reducing the fraction of intestinal cavity receiving a high dose (V40–V50), a finding that was in agreement with Roeske et al. who use peritoneal space as OAR in cervix cancer for both RT and CRT. Fiorino et al. also found that V15 was the best predictor of toxicity in patients treated with 3DCRT, but it had no biological meaning for patients treated with IMRT (higher values in these patients with fewer cases of toxicity), where V40–V50 were more important.
Dose to sacral nerves and bone has been studied with regards to acute and late toxicity in spine sarcoma patients [Citation32]. With both photon and proton plans they found that nerve tissue could tolerate doses up to 77 Gy in 1.8 Gy fractions, which is reassuring as none of our plans would have given doses in excess of 61.7 Gy in 2.5 Gy fractions to nerve roots.
Late toxicity is of greatest relevance when RT is given, but was not modelled in this study. Reasons for this are the lack of validated parameter values for combinations of chemotherapy and RT and the use of intestinal cavity rather than small bowel loops. Since the physical doses to various risk organs did not increase substantially by adding a boost, it is not likely that late toxicity will be a greater concern than in standard preoperative CRT; rectal toxicity is no longer a concern if successful surgery can be performed. The doses to the nerves are increased in the cases where the tumours grow adjacent to/or into the nerves, but still below what generally cause severe late toxicity.
All patients in this study would have been treated with CRT in order to maximise their chances of becoming resectable, since concomitant chemotherapy to RT improves local control [Citation6,Citation33,Citation34]. Literature on proton treatment of rectal cancer is scarce, and of protons in combination with chemotherapy it is virtually non-existent. Wolff et al. have suggested in a planning study for LARC that since proton plans were superior to photon plans, patients should be better equipped to cope with possible toxicity of combined CRT [Citation25]. No prospective study evaluating in vivo toxicity and tissue tolerance of CRT with protons has yet been done; rather, indications of possible advantages of protons in this setting are derived from planning studies similar to this one.
The patients recruited for this planning study came from a study in which PET was done for all patients. For delineation of these very advanced tumours MRI is generally considered sufficient and was the most important radiological examination for delineation purposes in this study with a boost to the periphery of the tumour. It could be stressed that if the purpose is to avoid or minimise surgery (so called organ preservation), boost doses should be against the tumour volumes with the highest number of (resistant) tumour cells. In these cases, PET-imaging may be more relevant.
For patients with locally very advanced rectal cancers who are not only primarily but likely also secondarily non-resectable, adding a boost to the area of organ infiltration is possible using either intensity-modulated photon or proton rays. In our study sample of seven patients, using protons gave five of seven patients an advantage with regards to dose in OAR. Finding ways to overcome disadvantages with protons such as lack of robustness, availability and treatment expense will be important if this treatment is to become more widespread.
Acknowledgements
The authors thank RaySearch laboratories for help regarding the planning software. Financial support was provided through the regional agreement on medical training and clinical research (ALF) in Stockholm.
The study was also supported by the Swedish Cancer Society and the Stockholm Cancer Society.
Declaration of interest: The authors report no conflicts of interest. The authors alone are responsible for the content and writing of the paper.
References
- Hughes R, Harrison M, Glynne-Jones R. Could a wait and see policy be justified in T3/4 rectal cancers after chemo-radiotherapy? Acta Oncol 2010;49:378–81.
- Valentini V, Aristei C, Glimelius B, Minsky BD, Beets-Tan R, Borras JM, . Multidisciplinary rectal cancer management. Radiother Oncol 2009;92:148–63.
- Valentini V, Glimelius B. Rectal cancer radiotherapy: Towards European consensus. Acta Oncol 2010;49:1206–16.
- Blomqvist L, Glimelius B. The ‘good’, the ‘bad’, and the ‘ugly’ rectal cancers. Acta Oncol 2008;47:5–8.
- Bjerkeset T, Morild I, Mork S, Soreide O. Tumor characteristics in colorectal cancer and their relationship to treatment and prognosis. Dis Colon Rectum 1987;30:934–8.
- Braendengen M, Tveit KM, Berglund A, Birkemeyer E, Frykholm G, Pahlman L, . Randomized phase III study comparing preoperative radiotherapy with chemoradiotherapy in nonresectable rectal cancer. J Clin Oncol 2008;26:3687–94.
- Mohiuddin M, Regine WF, John WJ, Hagihara PF, McGrath PC, Kenady DE, . Preoperative chemoradiation in fixed distal rectal cancer: Dose time factors for pathological complete response. Int J Radiat Oncol Biol Phys 2000; 46:883–8.
- Steele GD, Jr., Herndon JE, Bleday R, Russell A, Benson A, 3rd, Hussain M, . Sphincter-sparing treatment for distal rectal adenocarcinoma. Ann Surg Oncol 1999;6:433–41.
- Russell AH, Harris J, Rosenberg PJ, Sause WT, Fisher BJ, Hoffman JP, . Anal sphincter conservation for patients with adenocarcinoma of the distal rectum: Long-term results of Radiation Therapy Oncology Group protocol 89-02. Int J Radiat Oncol Biol Phys 2000;46:313–22.
- Jingu K, Ariga H, Kaneta T, Takai Y, Takeda K, Katja L, . Focal dose escalation using FDG-PET-guided intensity-modulated radiation therapy boost for postoperative local recurrent rectal cancer: A planning study with comparison of DVH and NTCP. BMC Cancer 2010;10:127.
- Caravatta L, Picardi V, Tambaro R, Padula GD, Macchia G, Deodato F, . Neoadjuvant accelerated concomitant boost radiotherapy and multidrug chemotherapy in locally advanced rectal cancer: A dose-escalation study. Am J Clin Oncol Epub2011 May 6.
- Duthoy W, De Gersem W, Vergote K, Boterberg T, Derie C, Smeets P, . Clinical implementation of intensity-modulated arc therapy (IMAT) for rectal cancer. Int J Radiat Oncol Biol Phys 2004;60:794–806.
- De Ridder M, Tournel K, Van Nieuwenhove Y, Engels B, Hoorens A, Everaert H, . Phase II study of preoperative helical tomotherapy for rectal cancer. Int J Radiat Oncol Biol Phys 2008;70:728–34.
- Engels B, De Ridder M, Tournel K, Sermeus A, De Coninck P, Verellen D, . Preoperative helical tomotherapy and megavoltage computed tomography for rectal cancer: Impact on the irradiated volume of small bowel. Int J Radiat Oncol Biol Phys 2009;74:1476–80.
- Braendengen M, Hansson K, Radu C, Siegbahn A, Jacobsson H, Glimelius B. Delineation of gross tumor volume (GTV) for radiation treatment planning of locally advanced rectal cancer using information from MRI or FDG-PET/CT: A prospective study. Int J Radiat Oncol Biol Phys 2011;81:e439–45.
- Myerson RJ, Garofalo MC, El Naqa I, Abrams RA, Apte A, Bosch WR, . Elective clinical target volumes for conformal therapy in anorectal cancer: A radiation therapy oncology group consensus panel contouring atlas. Int J Radiat Oncol Biol Phys 2009;74:824–30.
- Sanguineti G, Little M, Endres EJ, Sormani MP, Parker BC. Comparison of three strategies to delineate the bowel for whole pelvis IMRT of prostate cancer. Radiother Oncol 2008;88:95–101.
- Fiorino C, Alongi F, Perna L, Broggi S, Cattaneo GM, Cozzarini C, . Dose-volume relationships for acute bowel toxicity in patients treated with pelvic nodal irradiation for prostate cancer. Int J Radiat Oncol Biol Phys 2009;75:29–35.
- RaySearch. RayStation planning system. Stockholm, Sweden: RaySearch Laboratories AB; 2012.
- Martínez-Monge R, Nag S, Martin EW. Three different intraoperative radiation modalities (electron beam, high-dose-rate brachytherapy, and iodine-125 brachytherapy) in the adjuvant treatment of patients with recurrent colorectal adenocarcinoma. Cancer 1999;86:236–47.
- Wilking N. Intraoperative radiotherapy (IORT) – A critical review of the literature. Acta Oncol 2003;42:384–5.
- Tatsuzaki H, Urie MM, Willett CG. 3-D comparative study of proton vs. x-ray radiation therapy for rectal cancer. Int J Radiat Oncol Biol Phys 1992;22:369–74.
- Isacsson U, Montelius A, Jung B, Glimelius B. Comparative treatment planning between proton and X-ray therapy in locally advanced rectal cancer. Radiother Oncol 1996;41: 263–72.
- Johansson J. Comparative treatment planning in radiotherapy and clinical impact of proton relative biological effectiveness [Doctoral thesis]. Uppsala: Uppsala University; 2006.
- Wolff HA, Wagner DM, Conradi LC, Hennies S, Ghadimi M, Hess CF, . Irradiation with protons for the individualized treatment of patients with locally advanced rectal cancer: A planning study with clinical implications. Radiother Oncol 2012;102:30–7.
- Tilly N, Johansson J, Isacsson U, Medin J, Blomquist E, Grusell E, . The influence of RBE variations in a clinical proton treatment plan for a hypopharynx cancer. Phys Med Biol 2005;50:2765–77.
- Baglan KL, Frazier RC, Yan D, Huang RR, Martinez AA, Robertson JM. The dose-volume relationship of acute small bowel toxicity from concurrent 5-FU-based chemotherapy and radiation therapy for rectal cancer. Int J Radiat Oncol Biol Phys 2002;52:176–83.
- Tho LM, Glegg M, Paterson J, Yap C, MacLeod A, McCabe M, . Acute small bowel toxicity and preoperative chemoradiotherapy for rectal cancer: Investigating dose-volume relationships and role for inverse planning. Int J Radiat Oncol Biol Phys 2006;66:505–13.
- Robertson JM, Lockman D, Yan D, Wallace M. The dose-volume relationship of small bowel irradiation and acute grade 3 diarrhea during chemoradiotherapy for rectal cancer. Int J Radiat Oncol Biol Phys 2008;70:413–8.
- Roeske JC, Bonta D, Mell LK, Lujan AE, Mundt AJ. A dosimetric analysis of acute gastrointestinal toxicity in women receiving intensity-modulated whole-pelvic radiation therapy. Radiother Oncol 2003;69:201–7.
- Kavanagh BD, Pan CC, Dawson LA, Das SK, Li XA, Ten Haken RK, . Radiation dose-volume effects in the stomach and small bowel. Int J Radiat Oncol Biol Phys 2010;76(3 Suppl):S101–7.
- DeLaney TF, Liebsch NJ, Pedlow FX, Adams J, Dean S, Yeap BY, . Phase II study of high-dose photon/proton radiotherapy in the management of spine sarcomas. Int J Radiat Oncol Biol Phys 2009;74:732–9.
- Bosset JF, Collette L, Calais G, Mineur L, Maingon P, Radosevic-Jelic L, . Chemotherapy with preoperative radiotherapy in rectal cancer. N Engl J Med 2006;355: 1114–23.
- Gerard JP, Conroy T, Bonnetain F, Bouche O, Chapet O, Closon-Dejardin MT, . Preoperative radiotherapy with or without concurrent fluorouracil and leucovorin in T3–4 rectal cancers: Results of FFCD 9203. J Clin Oncol 2006;24: 4620–5.