Abstract
Background. To review treatment toxicity for patients with pancreatic and ampullary cancer treated with proton therapy at our institution. Material and methods. From March 2009 through April 2012, 22 patients were treated with proton therapy and concomitant capecitabine (1000 mg PO twice daily) for resected (n = 5); marginally resectable (n = 5); and unresectable/inoperable (n = 12) biopsy-proven pancreatic and ampullary adenocarcinoma. Two patients with unresectable disease were excluded from the analysis for reasons unrelated to treatment. Proton doses ranged from 50.40 cobalt gray equivalent (CGE) to 59.40 CGE. Results. Median follow-up for all patients was 11 (range 5–36) months. No patient demonstrated any grade 3 toxicity during treatment or during the follow-up period. Grade 2 gastrointestinal toxicities occurred in three patients, consisting of vomiting (n = 3); and diarrhea (n = 2). Median weight loss during treatment was 1.3 kg (1.75% of body weight). Chemotherapy was well-tolerated with a median 99% of the prescribed doses delivered. Percentage weight loss was reduced (p = 0.0390) and grade 2 gastrointestinal toxicity was eliminated (p = 0.0009) in patients treated with plans that avoided anterior and left lateral fields which were associated with reduced small bowel and gastric exposure. Discussion. Proton therapy may allow for significant sparing of the small bowel and stomach and is associated with a low rate of gastrointestinal toxicity. Although long-term follow-up will be needed to assess efficacy, we believe that the favorable toxicity profile associated with proton therapy may allow for radiotherapy dose escalation, chemotherapy intensification, and possibly increased acceptance of preoperative radiotherapy for patients with resectable or marginally resectable disease.
Pancreatic cancer is the fourth leading cause of cancer death in the US [Citation1]. In 2010 it was estimated that 43 000 people would be diagnosed and that there would be 36 000 deaths [Citation2]. Although only 15–20% of patients diagnosed with pancreatic cancer are considered for surgical resection, the median five-year survival rate for patients undergoing surgery is only 20% [Citation3]. Median survival expectation for patients with localized disease treated non-operatively is in the range of 9–13 months [Citation4]. Radiotherapy is commonly utilized in the management of non-metastatic pancreatic adenocarcinoma: as definitive therapy for unresectable disease, as neoadjuvant therapy for resectable or marginally resectable disease, and in the adjuvant postoperative setting. The toxicity of upper-abdominal photon-based radiotherapy, however, is not trivial. The Radiation Therapy Oncology Group (RTOG) 9704 trial demonstrated a 59% incidence of grade 3 non-hematologic toxicity in patients receiving postoperative adjuvant chemoradiation to a dose of 50.40 Gy with concomitant fluorouracil (5-FU) chemotherapy [Citation5]. Chauffert et al. reported similarly high rates of non-hematologic toxicity in the 2000–2001 Federation Francophone de Cancerologie Digestive and Societe Francaise de Radiotherapie Oncologique (FFCD/SFRO) trial for patients with unresectable pancreatic cancer receiving 60 Gy with infusional 5-FU and intermittent cisplatin [Citation6]. Loehrer et al. reported grade 3 or higher toxicity in 28 of 34 patients receiving 50.40 Gy with concurrent gemcitabine using 3-dimensional conformal radiotherapy (3DCRT) [Citation7]. Although many flaws have been identified in the study design [Citation8,Citation9], the results of the European Study Group for Pancreatic Cancer-1 (ESPAC-1) trial suggest that the toxicity of radiotherapy in the postoperative setting may outweigh its potential benefits and may, in fact, be associated with a nominal survival decrement [Citation10,Citation11].
A promising report from the University of Maryland suggests that intensity-modulated radiotherapy (IMRT) can reduce grade 3 toxicity in the postoperative adjuvant setting with concomitant 5FU-based chemotherapy. In this series, the rate of grade 3 diarrhea was only 4%, although grade 2 diarrhea was 20% [Citation12,Citation13]. Other series utilizing IMRT in the treatment of pancreatic cancer, however, have demonstrated higher rates of toxicity [Citation14–16].
While IMRT appears to represent a meaningful improvement in the therapeutic ratio compared to conventional 3DCRT, it is reasonable to believe that further treatment intensification—either with higher radiotherapy doses or more potent chemotherapy (i.e. gemcitabine)—will require further refinements in radiotherapy technique.
Dosimetric studies have suggested that proton therapy may allow for the delivery of standard radiotherapy doses to upper-abdominal targets while significantly reducing exposure to surrounding critical normal tissues. Hsiung-Stripp et al. [Citation17] reported the planning comparison between 3DCRT and 130–180 MeV protons for four patients with unresectable pancreatic adenocarcinoma. Compared to similarly effective photon plans, the proton plans significantly reduced dose to the spinal cord (p = 0.003), left kidney (p = 0.025), right kidney (p = 0.059), and liver (p = 0.061). The authors argue that this reduction in normal-tissue exposure might allow for radiotherapy dose escalation. Bouchard et al. [Citation18] compared 3DCRT with IMRT and protons in the delivery of 72 Gy to unresectable tumors. The authors argue that protons are superior to photons for tumors where small bowel is located anterior to the tumor. Kozak et al. [Citation19] demonstrated the dosimetric feasibility of hypofractionated proton radiotherapy for neoadjuvant pancreatic cancer treatment using anatomical data from nine patients. This study provided the basis for a subsequent phase I clinical trial. Compared with IMRT, protons offered a significant reduction of dose to the liver, kidneys, and small bowel, particularly in the low-dose regions. A three-field technique [anterior posterior oblique (APO): posterior anterior oblique (PAO): left lateral oblique] was used in the dosimetric study; however, protons were not associated with a reduced dose to the stomach or duodenum compared to IMRT. By the time the clinical trial was implemented [Citation20], the investigators had modified their technique to deliver most of the dose with PAO and right-lateral oblique fields. Nichols et al. [Citation21] compared postoperative IMRT versus protons for eight patients with resected pancreatic cancers. By delivering the majority of the dose with one or two posterior fields and a lesser dose through a right-lateral or lateral-oblique field, the investigators were able to significantly reduce the median small bowel V20Gy from 47.0% with IMRT to 15.4% with protons (p = 0.0156), the gastric V20Gy from 20.0% to 2.3% (p = 0.0313), and the median right kidney V18Gy from 50.5% to 27.3% (p = 0.0156).
With regard to clinical data supporting the use of proton therapy in the treatment of pancreatic cancers, Hong et al. [Citation20] published the results of a phase I trial of hypofractionated preoperative proton therapy with concomitant capecitabine (825 mg/m2 twice daily). Fifteen patients were treated with escalating radiotherapy doses. The final dose level—25 cobalt gray equivalent (CGE) in 5 fractions over one week—was tolerated. No dose-limiting toxicities were identified and there were no unexpected 30-day postoperative complications. Terashima et al. reported on the outcomes for 50 patients with locally advanced pancreatic cancers treated with proton therapy and concurrent gemcitabine [Citation22]. The majority of patients received a dose of 67.5 Gray Equivalent in 25 fractions using a field-within-a-field technique. With a median follow-up of 12.5 months, 10% of patients experienced grade 3 or higher late gastric hemorrhage. The one-year freedom from local-progression, progression-free, and overall survival rates were 81.7%, 64.3%, and 76.8%, respectively.
Herein we review our preliminary experience in the treatment of pancreatic and ampullary adenocarcinoma using conventionally fractionated proton therapy with concurrent capecitabine.
Material and methods
From March 2009 through April 2012, 22 patients were treated with proton therapy and concomitant oral capecitabine (1000 mg twice daily) for resected (n = 5), marginally resectable (n = 5), and unresectable/inoperable (n = 12) biopsy-proven pancreatic or ampullary adenocarcinoma. Patients were consented and enrolled on either the institutional review board-approved University of Florida Proton Therapy Institute (UFPTI) PC01 protocol for unresectable/inoperable disease (n = 7), the UFPTI PC02 protocol for patients with marginally resectable disease (n = 4), or the UFPTI outcomes tracking protocol (OTP) (n = 11). The OTP is an IRB-approved process which allows for retrospective review of patients treated with protons outside of a formal prospective study. The PC01 (unresectable) and PC02 (marginally resectable) protocols were written with strict entry criteria to allow for reliable analysis of survival outcomes. Not all patients seen in consultation were eligible for these prospective protocols, although it was believed that they would have been likely to benefit from proton therapy. Since the current analysis primarily addresses the toxicity of proton-based upper abdominal radiotherapy, we have pooled the protocol patients with the off-protocol (OTP) patients. The definitions for ‘marginal resectability’ were based on the NCCN guidelines as interpreted by the pancreatic surgeon participating in the patient's care.
One patient with unresectable disease (on the OTP) demonstrated clinical deterioration unrelated to treatment early on in his course and did not complete therapy. Another patient with unresectable disease (on PC01) died of a gunshot wound five days after starting treatment. Preradiotherapy chemotherapy was a function of the referral pattern. Several patients had been started on chemotherapy prior to seeing us. When this was the case, our approach was to allow treatment to be completed prior to staring chemoradiotherapy. The pretreatment characteristics of the 20 evaluable patients are shown in the Supplementary Table I to be found online at http://www.informahealthcare.com/doi/abs/10.3109/0284186X.2012.762997.
4D treatment planning computed tomography (CT) scans were used to account for breathing motion. Patients were simulated with intravenous and oral contrast. Normal tissues including the spinal cord, liver, kidneys, stomach and the entire small bowel space (as opposed to individual loops of bowel) were contoured from the contrast scan. For patients with measurable gross disease, an internal gross tumor volume (IGTV) was established using the contrast enhanced simulation scan along with other available imaging including positron emission tomography (PET) and magnetic resonance imaging (MRI). For patients treated postoperatively, an internal clinical target volume (ICTV) was established, based on the RTOG 9704/US Intergroup guidelines using the above imaging modalities. Target volumes for patients with marginally resectable disease consisted of the IGTV plus a 1.5-cm clinical target volume (CTV) expansion and a 1-cm planning target volume (PTV) expansion. Target volumes for patients with unresectable disease consisted of the IGTV plus a 0.3- to 1.0-cm CTV expansion and a 0.5- to 1.0-cm PTV expansion. Patients with resected disease were treated postoperatively to a median target dose of 54.0 (range 54–55.8) CGE using the standard 1.1 relative biological effectiveness (RBE) conversion factor. Patients with marginally resectable disease received 50.4 CGE. Patients with unresectable disease received a median dose of 59.4 CGE (range 55.8–59.4 CGE). Patients were treated at 1.8 CGE per fraction Monday through Friday for five days per week. The smaller target expansion for the unresectable patients (who received a dose of 59.40 CGE vs. 50.40 CGE for the preoperatively treated patients) was chosen out of concern for normal bowel exposure to compensate for the higher target dose delivered. Conversely, the larger target expansions were used in the marginally resectable patients based on the lower dose delivered and the assumption that the exposed duodenum would be removed at pancreaticoduodenectomy.
At least 95% of PTV volumes received 100% of the prescribed target dose and 100% of the PTV volumes received at least 95% of the target dose while maintaining acceptable doses to the spinal cord, kidneys, liver, bowel, and stomach. Conventional normal-tissue constraints were utilized [Citation23]. The spinal cord was absolutely limited to 46 CGE; the liver V30CGE was absolutely limited to < 60%; and 50% of the functioning nephrons (by nuclear imaging) received a dose below 18 CGE. Although our goal was to limit the small bowel and stomach V20CGE to < 50%, V45CGE to < 15%, V50CGE to < 10%, and V54CGE < 5%, in several situations, particularly for patients with large-volume unresectable disease, we exceeded these constraints.
CT images and structures were imported into the Eclipse Treatment Planning System (Varian Medical Systems, Palo Alto, CA, USA). A CT-Hounsfield unit to proton relative stopping-power conversion curve was used for proton-range calculations. The distal and proximal margins for each treatment field were estimated to be 2.5% of the beam range to the distal/proximal PTV plus 1.5 mm. These margins, thus calculated, typically had ranges of 6–9 mm. Field apertures were designed to conform to the PTV with an aperture margin adequate to account for the beam penumbrae. The range compensators were calculated with smearing margins that accounted for patient setup uncertainties and intra- and inter-fraction target motion, typically at 6–9 mm as well. A standard border-smoothing margin of 1 cm was used for all range-compensator calculations. Gantry and table angles were selected so that proton-beam paths minimally intersected high-uncertainty tissue volumes, such as the diaphragm and bowels. Early patients in our series were treated with combinations of anterior, posterior, right, and left lateral fields. We found that the use of anterior and left lateral fields was associated with higher rates of nausea and diarrhea, which led us to modify our field selection. This consideration typically led to the use of one or two heavily weighted posterior oblique fields that passed through the spine while minimally intersecting the kidneys. These fields were supplemented by a lateral oblique field with a significantly lower beam weight, often through the liver, to minimize the dosimetric effects of organ filling and motion uncertainties ().
Figure 1. Typical optimized proton plans later in our series utilized a posterior or posterior-oblique field delivering approximately 75% of the prescribed dose in conjunction with a right lateral or lateral oblique field delivering the remaining 25%. As proton beams are associated with no exit dose beyond the target, significant sparing of anterior small bowel and stomach tissues could be achieved. No patient treated in this fashion experienced grade 2 or higher gastrointestinal toxicity. Based on this favorable toxicity profile, all patients with pancreatic cancer at UFPTI are treated using this field configuration.
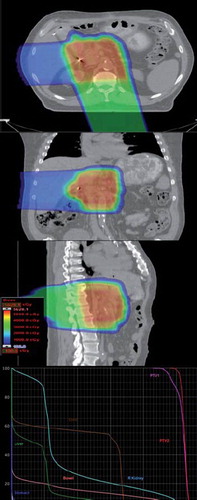
Patients were treated daily after eating a small meal to recreate gastric filling equivalent to that achieved at simulation. Patients were set up with daily orthogonal imaging to boney anatomy. Respiratory gating was not utilized. Fiducial markers were not used for patient positioning due to a concern that patient shifts to ostensibly improve tumor coverage might simultaneously bring a critical normal structure (such as the spinal cord) into a high-dose volume. CT scans were performed in the treatment position every one to two weeks to confirm coverage of target volumes.
SAS and JMP software were utilized for all statistical calculations (SAS Institute, Cary, NC, USA). Grade 2 gastrointestinal toxicity rates between those treated with and without anterior or left lateral fields was assessed with Fisher's exact test. Levels of weight loss between these two treatment methods was assessed with the Wilcoxon signed rank test.
Results
Field configuration, dose, normal-tissue exposures, follow-up duration, and toxicity for all patients are shown in .
Table I. Patient-specific field configurations, doses, normal-tissue exposures, follow-up durations, and most severe toxicities.
The median follow-up for all patients is 11 months (range 5–36 months) from the initiation of treatment. No patient required a treatment interruption due to toxicity. Proton therapy did not interfere with chemotherapy delivery. All patients were prescribed oral capecitabine at 1000 mg twice daily to be taken during radiotherapy. Of the 10 patients enrolled on the PC01 or PC02 protocols (for whom formal drug logs were maintained), a median of 99% of the prescribed doses were taken (range 90–100%). One patient with reduced (90%) chemotherapy compliance was unable to obtain the capecitabine due to insurance issues for the first three days of radiotherapy. Of the patients treated on OTP, one patient discontinued capecitabine after one week against medical advice without experiencing any drug or treatment-related toxicity. The remaining nine patients on OTP informed us during their weekly on-treatment visits that they were taking the chemotherapy as prescribed although a formal drug log was not maintained in the radiotherapy record. Toxicity was measured using the Common Terminology Criteria for Adverse Events (CTCAE), version 4.
No patient experienced any grade 3 toxicity during treatment or at any time during the follow-up period. Three of 20 patients reported grade 2 acute gastrointestinal toxicities including vomiting (n = 3) and diarrhea (n = 2). All three of these patients were treated with fields that delivered a portion of the dose through the small bowel (anterior field) or stomach (left lateral field). The patients who experienced grade 2 gastrointestinal toxicity are described below:
Patient #1 is a 70-year-old female presenting with a T4N0 adenocarcinoma of the pancreatic head. She was deemed to be marginally resectable based on superior mesenteric artery (SMA) abutment (without arterial encasement) and enrolled on the PC02 preoperative proton therapy protocol. She was treated with equally weighted right posterior oblique (RPO), right anterior oblique (RAO), and left lateral (L) fields to a PTV dose of 50.40 CGE. She began to experience grade 2 abdominal pain and vomiting starting in the second week of therapy. Post-treatment imaging showed disease progression after completion of proton therapy and she was not offered curative surgery. She died with local progression and distant metastases 11 months after treatment initiation.
Patient #3 is a 53-year-old male presenting with a T4N1 unresectable (based on hepatic artery encasement) adenocarcinoma of the pancreatic head. He was enrolled on the OTP because the PC01 protocol for patients with unresectable disease was under IRB review at the time of his consultation. He was treated with a combination of posterior anterior (PA), right lateral (RL) and left lateral (L) fields with approximately a 2:1:1 weighting to a PTV dose of 59.40 CGE. He began to experience grade 2 fatigue in week number 2, grade 2 vomiting and diarrhea in week number 4, and grade 2 weakness and abdominal pain in week number 6. He died with local disease progression and distant metastases five months after treatment initiation.
Patient #6 is a 54-year-old female presenting with a T4N0 unresectable (based on superior mesenteric artery encasement) adenocarcinoma of the uncinate process. She was treated with a combination of PA and anterior superior oblique (ASO) fields with approximately a 3:2 weighting. She was enrolled on the OTP for the same reason described for Patient #3. The initial plan was to deliver a PTV dose of 59.40 CGE; however, toxicity (grade 2 nausea and vomiting in week 4, grade 2 diarrhea and anorexia in week 5, and grade 2 abdominal pain in week 6) prompted a discontinuation of treatment after a dose of 55.80 CGE. She died of distant metastases without evidence of local progression seven months after treatment initiation.
Of the 17 patients who were treated without anterior or left lateral fields—sparing entry dose through the small bowel and stomach—no patient experienced grade 2 vomiting or diarrhea. The elimination of grade 2 gastrointestinal toxicity with this field configuration was statistically significant at the p = 0.0009 level.
The median weight loss for all 20 patients over the course of radiotherapy was 1.3 kg (range, a gain of 4.7 kg to a loss of 9.6 kg). Patients treated without anterior or left lateral fields demonstrated a median 0.5-kg weight loss (range, a gain of 4.7 kg to a loss of 6.4 kg). The three patients treated with anterior or left lateral fields demonstrated a median 9.0 kg weight loss (range, a loss of 1.6 kg to a loss of 9.6 kg). On the basis of percentage weight loss, this difference was statistically significant at the p = 0.0390 level.
With regard to local and regional tumor control, distant metastasis, and survival, the best outcomes are seen in the patients treated preoperatively for marginally resectable disease (median survival, 14 months) followed by the patients treated postoperatively (median survival, 11 months) and the unresectable/inoperable patients (median survival, 8.8 months).
One of the five patients treated postoperatively is alive without evidence of local-regional recurrence or distant metastasis 14 months after treatment. One patient is alive with distant metastases (liver) without local failure at 27 months. Three patients died with both a local recurrence and distant metastases (at 8, 9, and 11 months).
One of the five patients (with a T3N0 primary tumor) treated for marginally resectable disease refused surgery after proton therapy and is alive without measurable disease or toxicity 36 months after proton therapy. One patient (T3N0) underwent a curative R0 resection but demonstrated liver metastases at nine months after treatment. She is alive without evidence of treatment-related toxicity 18 months after treatment. Two patients did not undergo surgery and died with both local and distant disease at 11 and 13 months after proton therapy. One patient with a T3 tumor of the pancreatic tail achieved a complete response at the primary site but demonstrated hepatic metastases prior to planned surgery and died 11 months after proton therapy ().
Figure 2. Patient #5 presented with a marginally resectable adenocarcinoma of the pancreatic tail (top three images). He achieved a virtual complete radiographic response (bottom three images) after 50.40 CGE in 28 fractions with oral concomitant capecitabine at 1000 mg twice daily on treatment days. He did not undergo surgery due to the emergence of multiple liver metastases shortly after completion of proton therapy.
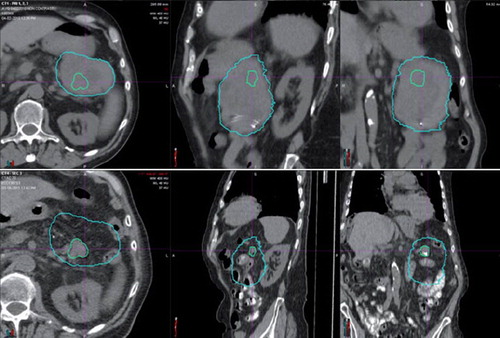
Of the 10 patients treated for unresectable (T4) or inoperable (T3) disease, eight have died of disease with distant metastases with a median survival of 8.4 months. Two patients (both with T4 presentations), however, achieved significant radiographic responses to proton therapy and were taken to surgery for pancreaticoduodenectomy. One patient underwent an R2 resection and is without disease progression at 10 months after proton therapy. One patient was found to have achieved a pathologic compete response and is now alive without disease nine months after proton therapy.
Discussion
To our knowledge, the current series is the first published study of clinical outcomes for pancreatic cancer patients treated with conventionally fractionated proton therapy. While it is not possible to offer a meaningful comparison of disease control with conventional radiotherapy or IMRT, the modest gastrointestinal toxicity observed suggests that proton therapy may have the potential to improve the therapeutic index for patients receiving radiotherapy for pancreatic malignancy.
One weakness of this report includes the short median follow-up of 11 months for surviving patients. While the 12 patients followed for less than 12 months can be evaluated for acute toxicity (i.e. weight loss during treatment, interruptions of therapy due to nausea or diarrhea), it is not possible to rule out the possibility that some of these patients may go on to suffer duodenal or other small bowel toxicity. The eight patients followed for more than 12 months may be unlikely to manifest small bowel or duodenal toxicity given the absence of toxicity up to the point of last follow-up. It is, however, not possible to rule out the emergence of a late toxicity, such as billiary stricture, which could occur several years after radiotherapy.
Of the eight patients with more than 12 months of follow-up, three were treated for unresectable disease to a dose of 59.40 CGE; one received 54.00 CGE in the postoperative setting; and four received 50.40 CGE as preoperative therapy. While late small bowel or duodenal toxicity would generally not be expected for patients receiving the dose of 50.40 CGE, or even 54.00 CGE, the lack of such toxicity in the surviving patients receiving 59.40 CGE is encouraging. Nevertheless, it is fair to point out that two of these three patients underwent duodenal bypass surgery prior to proton therapy.
An additional weakness of the report includes the heterogeneity of the doses delivered as well as the patient population treated, which included postoperatively treated, medically inoperable, locally advanced inoperable, and marginally resectable patients. This heterogeneity certainly clouds any interpretation of the local control and survival data. On the other hand, the main focus of the report is the toxicity of upper abdominal proton therapy to doses ranging from 50.40 to 59.40 CGE delivered with concomitant capecitabine. We believe that our findings substantiate the limited toxicity of this approach.
Currently at UFPTI we are accruing patients to three clinical trials utilizing proton therapy and concomitant chemotherapy for patients with non-metastatic pancreatic carcinomas. Our protocol for patients with unresectable tumors delivers 59.40 CGE to gross disease with concomitant capecitabine (1000 mg twice daily). Our protocol for patients with marginally resectable tumors delivers 50.40 CGE to gross disease with concomitant capecitabine (1000 mg twice daily). Our protocol for patients with resected pancreatic head tumors prescribes 50.40 CGE for patients with R0 resections, 54.00 CGE for patients with R1 resections, and 59.40 CGE for patients with R2 resections. All patients in this third protocol receive concomitant weekly gemcitabine at 300 mg/m2.
Supplementary Table 1
Download PDF (29 KB)Declaration of interest: The authors report no conflicts of interest. The authors alone are responsible for the content and writing of the paper.
Presented at the International Symposium on Pancreas Cancer 2012, Kyoto, Japan October 6, 2012.
References
- Jemal A, Siegel R, Ward E, Hao Y, Xu J, Thun MJ. Cancer statistics, 2009. CA: A cancer journal for clinicians. 2009;59:225–49.
- SEER Cancer Statistics Review, 1975–2007. Bethesda, MD: National Cancer Institute; 2010. [cited 27 May 2011]. Available from: http://seer.cancer.gov/csr/1975_2007/.
- Sohn TA, Yeo CJ, Cameron JL, Koniaris L, Kaushal S, Abrams RA, . Resected adenocarcinoma of the pancreas-616 patients: Results, outcomes, and prognostic indicators. J Gastrointest Surg 2000;4:567–79.
- Gunderson LL, Haddock MG, Burch P, Nagorney D, Foo ML, Todoroki T. Future role of radiotherapy as a component of treatment in biliopancreatic cancers. Ann Oncol 1999;10(Suppl 4):291–5.
- Regine WF, Winter KA, Abrams RA, Safran H, Hoffman JP, Konski A, . Fluorouracil vs gemcitabine chemotherapy before and after fluorouracil-based chemoradiation following resection of pancreatic adenocarcinoma: A randomized controlled trial. JAMA 2008;299:1019–26.
- Chauffert B, Mornex F, Bonnetain F, Rougier P, Mariette C, Bouche O, . Phase III trial comparing intensive induction chemoradiotherapy (60 Gy, infusional 5-FU and intermittent cisplatin) followed by maintenance gemcitabine with gemcitabine alone for locally advanced unresectable pancreatic cancer. Definitive results of the 2000–01 FFCD/SFRO study. Ann Oncol 2008;19:1592–9.
- Loehrer PJ, Sr. Feng Y, Cardenes H, Wagner L, Brell JM, Cella D, . Gemcitabine alone versus gemcitabine plus radiotherapy in patients with locally advanced pancreatic cancer: An Eastern Cooperative Oncology Group trial. J Clin Oncol 2011;29:4105–12.
- Abrams RA. Radiotherapy in the adjuvant management of pancreatic adenocarcinoma. Semin Oncol 2005;32(6 Suppl 9):S30–2.
- Koshy MC, Landry JC, Cavanaugh SX, Fuller CD, Willett CG, Abrams RA, . A challenge to the therapeutic nihilism of ESPAC-1. Int J Radiat Oncol Biol Phys 2005;61:965–6.
- Neoptolemos JP, Dunn JA, Stocken DD, Almond J, Link K, Beger H, . Adjuvant chemoradiotherapy and chemotherapy in resectable pancreatic cancer: A randomised controlled trial. Lancet 2001;358:1576–85.
- Neoptolemos JP, Stocken DD, Friess H, Bassi C, Dunn JA, Hickey H, . A randomized trial of chemoradiotherapy and chemotherapy after resection of pancreatic cancer. N Engl J Med 2004;350:1200–10.
- Yovino S, Poppe M, Jabbour S, David V, Garofalo M, Pandya N, . Intensity-modulated radiation therapy significantly improves acute gastrointestinal toxicity in pancreatic and ampullary cancers. Int J Radiat Oncol Biol Phys 2011;79:158–62.
- Regine WF. Personal Communication. 2010.
- Milano MT, Chmura SJ, Garofalo MC, Rash C, Roeske JC, Connell PP, . Intensity-modulated radiotherapy in treatment of pancreatic and bile duct malignancies: toxicity and clinical outcome. Int J Radiat Oncol Biol Phys 2004;59:445–53.
- Abelson JA, Murphy JD, Minn AY, Chung M, Fisher GA, Ford JM, . Intensity-modulated radiotherapy for pancreatic adenocarcinoma. Int J Radiat Oncol Biol Phys 2012;82:e595–601.
- Ben-Josef E, Schipper M, Francis IR, Hadley S, Ten-Haken R, Lawrence T, . A phase I/II trial of intensity modulated radiation (IMRT) dose escalation with concurrent fixed-dose rate gemcitabine (FDR-G) in patients with unresectable pancreatic cancer. Int J Radiat Oncol Biol Phys 2012;84:1166–71.
- Hsiung-Stripp DC, McDonough J, Masters HM, Levin WP, Hahn SM, Jones HA, . Comparative treatment planning between proton and X-ray therapy in pancreatic cancer. Med Dosim 2001;26:255–9.
- Bouchard M, Amos RA, Briere TM, Beddar S, Crane CH. Dose escalation with proton or photon radiation treatment for pancreatic cancer. Radiother Oncol 2009;92:238–43.
- Kozak KR, Kachnic LA, Adams J, Crowley EM, Alexander BM, Mamon HJ, . Dosimetric feasibility of hypofractionated proton radiotherapy for neoadjuvant pancreatic cancer treatment. Int J Radiat Oncol Biol Phys 2007;68:1557–66.
- Hong TS, Ryan DP, Blaszkowsky LS, Mamon HJ, Kwak EL, Mino-Kenudson M, . Phase I study of preoperative short-course chemoradiation with proton beam therapy and capecitabine for resectable pancreatic ductal adenocarcinoma of the head. Int J Radiat Oncol Biol Phys 2011;79:151–7.
- Nichols RC, Jr., Huh SN, Prado KL, Yi BY, Sharma NK, Ho MW, . Protons offer reduced normal-tissue exposure for patients receiving postoperative radiotherapy for resected pancreatic head cancer. Int J Radiat Oncol Biol Phys 2012;83:158–63.
- Terashima K, Demizu Y, Hashimoto N, Jin D, Mima M, Fujii O, . A phase I/II study of gemcitabine-concurrent proton radiotherapy for locally advanced pancreatic cancer without distant metastasis. Radiother Oncol [Clinical Trial, Phase I Clinical Trial, Phase II] 2012;103:25–31.
- Huguet F, Goodman KA, Azria D, Racadot S, Abrams RA. Radiotherapy technical considerations in the management of locally advanced pancreatic cancer: American-French consensus recommendations. Int J Radiat Oncol Biol Phys 2012;83:1355–64.