Abstract
Background. Hypofractionated radiotherapy potentially offers therapeutic gain for prostate cancer. We investigated the feasibility of hypofractionated proton therapy (PT). Material and methods. Eighty-two patients with biopsy-proven T1-3N0M0 prostate adenocarcinoma and no history of androgen deprivation therapy were randomly assigned to five different dose schedules: Arm 1, 60 CGE (cobalt gray equivalent = proton dose in Gy × 1.1)/20 fractions/5 weeks; Arm 2, 54 CGE/15 fractions/5 weeks; Arm 3, 47 CGE/10 fractions/5 weeks; Arm 4, 35 CGE/5 fractions/2.5 weeks; or Arm 5, 35 CGE/5 fractions/5 weeks. Results. The median follow-up duration was 42 months (11–52 months). The acute GI and GU grade ≥ 2 toxicity rates were 0 and 5%, respectively. The late GI and GU grade ≥ 2 toxicity rates were 16% and 7%, respectively. The best arm for acute GU toxicity was Arm 3, while that for late GI toxicity was Arm 2 in which none had grade ≥ 2 toxicity. The four-year American Society for Therapeutic Radiology and Oncology and Nadir + 2ng/ml BCF free survival (BCFFS) rates were 85% and 86%, respectively. Conclusions. Hypofractionated PT for patients with prostate adenocarcinoma as used in this study is feasible with an acceptable toxicity profile. As the BCFFS rates do not seem to be inferior to those produced using conventional fractionation, the application of hypofractionated PT may save patients time and money.
Prostate cancer has the slowest natural growth rate of all cancers. The average cell doubling time measured before treatment is 40 days (range, 15–> 60 days) [Citation1], compared with about five days for many other types of cancer. Brenner et al. [Citation2] estimated that the α/β ratio of prostate cancer was comparable to that of late-responding normal tissue at 1.5 Gy. By comparison, the α/β ratios for cancer and for late-responding normal tissue are assumed to be 10 and 3 Gy, respectively. The lower α/β ratio for prostate cancer than for the surrounding normal tissue creates the potential for therapeutic gain using hypofractionated radiotherapy (hypo-RT) because hypo-RT damages the former more than the latter. Thus, the effect of a hypofractionated regimen on tumor control would in theory be enhanced. To explore this potential therapeutic gain, we investigated several candidate high-benefit/low-risk hypofractionated regimens and adopted five different hypofractionated dose schedules suggested by Fowler et al. [Citation3]. They suggested a set of hypofractionated dose schedules using the linear quadratic model. This was done by adjusting the dose per fraction and total dose to maintain the risks of late rectal complications constant at the level for 72 Gy of conv-RT (2 Gy × 36 fractions). Fowler et al. [Citation4] also suggested not squeezing hypofractionated schedules into too-short overall times. They used insights gained from mucosal tolerance studies of head and neck RT together with standard linear quadratic modeling. However, how short is too short is unclear.
Due to its unique pattern of dose deposition and spread-out Bragg peak, proton therapy (PT) can deliver highly conformal radiation to tumors located close to critical normal structures. PT has been successfully applied to prostate cancer; the data have demonstrated high rates of biochemical control and low rates of urinary or rectal complications [Citation5]. Our research interest in PT for prostate cancer includes the exploration of hypofractionation, quality of life outcomes, and cost-effectiveness. Our hypothesis was that the hypofractionated PT dose schedules used in the present study would be as effective as 72 Gy of conv-RT in tumor control, if not better, without increasing the risks of acute and late toxicities. If true, our findings would positively affect patients’ time commitment and medical expenses by requiring a smaller number of fractions.
Thus, we conducted a phase II prospective trial using five different dose schedules of PT as shown in . The endpoints were acute and late toxicity, quality of life, and biochemical control.
Table I. Five hypofractionated dose schedules.
Material and methods
Rationale for the dose/fractionation schedules used in this study
We adopted the dose schedules of Fowler et al. [Citation3], and then adjusted the treatment interval from one to four times a week so that acute rectal mucosal toxicity would not exceed the level of a 72 Gy equivalent dose in 2 Gy per fraction (EQD2). Next, five different hypofractionated dose schedules were chosen as follows: Arm 1, 60 CGE (cobalt gray equivalent = proton dose in Gy × 1.1)/20 fractions/4 times a week for 5 weeks; Arm 2, 54 CGE/15 fractions/3 times a week for 5 weeks; Arm 3, 47 CGE/10 fractions/twice a week for 5 weeks; Arm 4, 35 CGE/5 fractions/twice a week for 2 weeks; and Arm 5, 35 CGE/5 fractions/once a week for 4 weeks ().
The biological equivalent dose (BED) was calculated for late effects using equation [Citation1] with α/β = 3 for the rectum. The effect on tumor control was then estimated, assuming α/β = 1.5 for prostate cancer. We did not take the effect of repopulation for prostate cancer during treatment into account because prostate cancer repopulates slowly and no detrimental effects on tumor control were found until the overall treatment times exceeded nine or 10 weeks [Citation6].
As acute rectal mucosal toxicity was a concern due to extreme hypofractionation, the BED was calculated using Equation 2 accounting for repopulation with an assumption of α/β = 10, Tp = 2.5 days [Citation7], and Tk = 7 days [Citation8], which were extrapolated from the oral mucosa. The details of the equations were in the Supplementary Appendix (available online at http://informahealthcare.com/doi/abs/10.3109/0284186X.2013.764011).
summarizes the five hypofractionated dose schedules and the calculated EQD2 for prostate cancer for tumor control and the rectum for acute and late effects.
Patient eligibility
Patients with biopsy-proven androgen-deprivation therapy (ADT)-naive prostate adenocarcinoma and stage T1-3N0M0 were eligible for the trial. The exclusion criteria were as follows: previous curative surgery or RT; evidence of distant metastasis; previous ADT; and failure to provide informed consent. Our institutional review board approved the study protocol.
Proton therapy
Patients were randomly assigned to one of the five different dose schedules. The procedure involved in simulation and treatment planning for PT at our institution has been published elsewhere in detail [Citation9]. In brief, three gold markers were inserted into the prostate and they were used to verify the exact location of the prostate for each treatment session. A balloon was inserted into the rectum and filled with 100 ml of saline for both computed tomography (CT) images and magnetic resonance imaging (MRI) scans for treatment planning. A set of 3-mm-thick contrast CT images and MRI scans were acquired on the same day ().
Figure 1. (A) A set of 3-mm-thick contrast computed tomography images was acquired for treatment planning. Three gold markers were inserted into the prostate. One of the three gold markers is not seen because it was inserted into a different axis. A balloon was inserted into the rectum and filled with 100 ml of saline. (B) Treatment planning was performed using bilateral beams with the Eclipse proton beam planning system (Ver. 8.1; Varian Medical System, Palo Alto, CA, USA). The planning goals included that at least 95% of the PTV received the prescribed dose, and that the volume receiving 50 Gy EQD2 should not exceed 30% for the rectum and bladder.
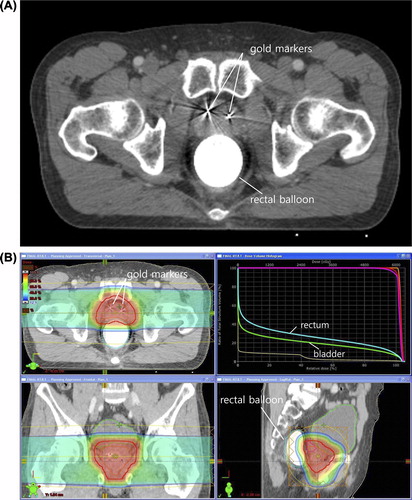
The CT and MRI images were fused by using a registration algorithm, Normalized Mutual Information (The Syntegra software on the Pinnacle TPS Ver. 7.6c; Philips Radiation Oncology Systems, Fitchburg WI). The targets were delineated on the fused images. The clinical target volume (CTV) was defined as the whole prostate and proximal 1 cm of the seminal vesicles. Regional lymph nodes were not included in the target. The planning target volume (PTV) was defined as the CTV plus a 7- to 10-mm margin. Treatment planning was performed using bilateral beams with the Eclipse proton beam planning system (Ver. 8.1; Varian Medical System, Palo Alto, CA, USA). The dose was prescribed to the 100% iso-dose line after heterogeneity correction. The planning goals included that at least 95% of the PTV received the prescribed dose, and that the volume receiving 50 Gy EQD2 should not exceed 30% for the rectum and bladder ().
At every treatment session, digital orthogonal x-ray images were acquired and transferred to the digital image positioning system. Next, the three-dimensional (3D) relative locations of the gold markers in the digital images were compared with those in the reference images. The difference between the two images, if any, was calculated automatically by the system and the treatment couch was adjusted to eliminate any discrepancy greater than 1 mm. All patients were treated using the bilateral two-field technique in a day with PT (Proteus 235; Ion Beam Application, S.A., Louvain-la-Neuve, Belgium).
Statistical analysis
Severe acute toxicity (SAT) is defined as any grade 2 or greater toxicity or any acute toxicity requiring a dose reduction. With a probability of 90% for selecting the best arm with a 30% difference between the best arm and the other four arms in terms of SAT, 18 patients were needed per arm. Assuming a loss to follow-up of 5%, 19 patients were needed per arm [Citation10]. Thus, a total of 95 patients were to be enrolled. Biochemical failure free survival (BCFFS) was determined from the date of the first day of PT. Survival rates were calculated using the Kaplan-Meier actuarial method.
Data collection and follow-up
Follow-up visits were scheduled at one month after the completion of PT, every three months during the next two years, every six months for an additional three years, and annually thereafter. Acute toxicity was rated according to NCI Common Toxicity Criteria (http://ctep.cancer.gov/forms/CTCAEv3.pdf). Late toxicity was rated according to the Radiation Therapy Oncology Group (RTOG)/European Organization for Research and Treatment of Cancer (EORTC) late radiation morbidity scoring system. Biochemical failure (BCF) was assessed using the definition of the American Society for Therapeutic Radiology and Oncology (ASTRO) [three consecutive increases in prostate-specific antigen (PSA)] and the alternate Nadir + 2 ng/ml definition. Patient-reported measures, including the American Urological Association (AUA) score [Citation11], urinary quality of life (QoL) score, sexual dysfunction scale, modified Late Effects Normal Tissue Task Force (LENT)-Subjective, Objective, Management, Analytic (SOMA) genitourinary (GU), and gastrointestinal (GI) toxicity scale [Citation12], were collected. A clinically relevant change in these scores was defined as a difference from baseline to follow-up that exceeded half a standard deviation of the baseline value [Citation13].
Results
Study population
Some concerns regarding tumor control were raised in Arm 5, which used an extremely protracted treatment regimen. Subsequently, accrual to Arm 5 was stopped after 14 patients were enrolled. Thus, the overall accrual goal was changed to 90 patients. Among them, eight patients who declined follow-up were excluded from analysis.
A total of 82 patients were analyzed and the median follow-up duration was 42 months (range, 11–52 months). The characteristics of the patients are summarized in . The median age was 68 years. No significant difference was found in the distribution of the Gleason score, initial PSA, T stage, or risk group (NCCN guideline) among the treatment arms.
Table II. Patient characteristics.
Acute and late toxicities
lists the maximum acute and late toxicities. Grade 1 acute skin toxicity was observed in 57 (70%) patients. Twelve (15%) patients developed an acute grade 1 GI toxicity and none had GI grade ≥ 2 toxicity. Fifty-seven (70%) and four (5%) patients developed grade 1 and 2 acute GU toxicities, respectively. None had a grade ≥ 3 acute toxicity. Late GI toxicities were observed in 53 (65%) patients: grade 1 in 40 (49%) patients, grade 2 in 11 (13%) patients, and grade 3 in two (2%) patients. Late GU toxicities were observed in 28 (34%) patients: grade 1 in 22 (27%) patients and grade 2 in six (7%) patients. Thirty-seven (45%) patients had grade 1 skin pigmentation. In contrast to the other arms, no acute GU grade ≥ 2 toxicity was observed in Arm 3. Regarding late GI toxicities, Arm 2 had no grade ≥ 2 toxicity while the others did. Given these results, we selected Arm 3 as the best arm for severe acute GU toxicity and Arm 2 for severe late GI toxicity.
Table III. Maximum acute and late toxicity.
Patient-reported measures
summarizes the change in patient-reported measures, including AUA score, urinary QoL score, sexual dysfunction scale, and modified LENT-SOMA GU and GI toxicity scale. The AUA score was highest at the end of PT and improved afterwards. The urinary QoL score also improved after PT. The modified LENT-SOMA GU and GI toxicity score showed no significant change except for dysuria and rectal bleeding. Dysuria was at its worst at the end of PT and improved afterwards, while rectal bleeding began to worsen at seven months and improved at 37 months after PT.
Table IV. Patient-reported measures.
BCFFS
demonstrates the BCFFS rates according to ASTRO and Nadir + 2 ng/ml definitions. The four-year ASTRO and Nadir + 2 ng/ml BCFFS rates were 85% and 86%, respectively. The four-year ASTRO BCFFS rates for the low-, intermediate-, and high-risk groups were 93%, 85%, and 75%, respectively (p = 0.29). The corresponding BCFFS rates for the Nadir + 2 ng/ml definition were 92%, 90%, and 75%, respectively (p = 0.56). Although we observed more frequent BCF in Arm 5 than others, there was no statistically significant difference in BCFFS among the five Arms probably because of the small sample size. According to ASTRO definition, the number of patients experiencing BCF were one (5.3%), three (18.8%), two (11.8%), two (11.1%) and three (25%) for Arm 1, 2, 3, 4, and 5, respectively. The corresponding figures according to Nadir + 2 ng/ml definition were one (5.3%), two (12.5%), two (11.8%), one (5.6%) and two (16.7%).
Discussion
The hypofractionated dose schedules used in the present study are feasible with an acceptable toxicity profile compared to the previous studies ().
Table V. Previous studies.
Arcangeli et al. [Citation14,Citation15] performed a randomized trial of 168 patients comparing conv-RT with hypo-RT using 3D conformal RT (3D-CRT). Acute GU grade ≥ 2 toxicity was observed in 40% of the conv-RT (80 Gy EQD2) and 47% of the hypo-RT (62 Gy/20 fractions, 72 Gy EQD2) groups. The corresponding proportions for acute GI grade ≥ 2 toxicity were 21% and 35%, respectively. Kupelian et al. [Citation16] reported the results of 770 patients treated with hypofractionated intensity-modulated RT (IMRT) with 70 Gy/28 fraction/5 weeks (79 Gy EQD2). The acute GU and GI grade ≥ 2 toxicity rates were 19% and 9%, respectively. Martin et al. [Citation17] performed phase II hypo-IMRT and IGRT in 92 patients treated with 60 Gy/20 fractions/4 weeks (74 Gy EQD2); they reported acute GU and GI grade ≥ 2 toxicity rates of 25% and 12%, respectively. To summarize the aforementioned studies using hypofractionated regimens, the acute grade ≥ 2 toxicity rates ranged from 19% to 47% for GU and from 9% to 35% for GI after delivering 72–80 Gy EQD2. Interestingly, Arcangeli et al. [Citation15] reported the highest toxicity rates of 47% for GU and 35% for GI after 3D-CRT, although the lowest EQD2 (72 Gy) was delivered. Thus, treatment techniques seem to affect the risk of toxicity. Compared with these studies, our data showed extremely low rates of acute grade ≥ 2 toxicity (5% for GU and 0 for GI).
Madsen et al. [Citation18] reported the acute GU and GI grade ≥ 2 toxicity rates were 23% and 13%, respectively, following stereotactic hypo-RT with 33.5 Gy/5 fractions/week (57 Gy EQD2). King et al. [Citation19] reported that rectal symptoms were improved in patients treated every other day compared to those treated for five consecutive days (0% vs. 38%, p = 0.0035) following Cyberknife stereotactic hypo-RT of 36.25 Gy/5 fractions (61–63 Gy EQD2). Many factors such as the PTV margins and time/dose/fractionation scheme may have affected the risks of acute toxicity. The PTV margin for CyberKnife [Citation19] was 3 mm for posterior direction and 5 mm for other directions. Madsen et al. [Citation18] used six stationary non-coplanar fields with a 4–5-mm margin from the prostate to the block edge. As these margins were smaller than the 7–10 mm of ours, we could not explain that our lower acute toxicity rates were attributed to the PTV margins. The regimen in these two studies was similar to that in Arms 4 and 5 in our study, but time factor in which the treatment was delivered twice (Arm 4) or once (Arm 5) a week. The corresponding acute toxicity rates for Arms 4 and 5 were of 6% and 8% for GU and 0 and 0 for GI toxicities, respectively. The lower rates of acute toxicity could be attributed, at least in part, to the treatment modality (PT) and carefully designed hypofractionated dose schedule, which limited the EQD2 to below 70 Gy for acute effects.
Pollack et al. [Citation20] and Kuban et al. [Citation21] published the results of a randomized trial comparing conv-RT of 70 Gy/35 fractions with 78 Gy/39 fractions in 301 patients using 3D-CRT. GI grade ≥ 2 toxicity occurred twice as often in the high-dose patients (26% vs. 13%, p = 0.013), although GU grade ≥ 2 toxicity was less common (13% vs. 8%, p ≥ 0.05). Arcangeli et al. [Citation15] reported that the late GU and GI grade ≥ 2 toxicity rates were 11% and 14%, respectively, following conv-RT of 80 Gy and 16% and 17%, respectively, following hypo-RT of 76 Gy EQD2 using 3D-CRT. The toxicity rates were not statistically different between the two arms. Kupelian et al. [Citation16] reported that the rates were 7% and 6%, respectively, with hypo-IMRT of 77Gy EQD2. Martin et al. [Citation17] reported that they were 10% and 6%, respectively, with hypo-IMRT and IGRT of 72 Gy EQD2. Thus, IMRT and IGRT appear to produce lower late toxicity rates. After stereotactic hypo-RT, the late GU and GI grade ≥ 2 toxicity rates ranged from 16% and 9% with 65 Gy EQD2 [Citation18] to 29% and 15% with 74 Gy EQD2 [Citation19], respectively. The latter two studies used extreme hypofractionation and seemed to cause more GU (range, 16%–29%) than GI toxicity (range, 9%–15%). By contrast, the corresponding toxicity rates in Arm 4 and 5 of our study using a similar EQD2 were 7% (2/30 patients) and 13% (4/30 patients), respectively. Whether the difference is due to the treatment technique or treatment volume is unclear. Stereotactic RT, particularly when using a Cyberknife, presumably has more dose inhomogeneity within the target, and subsequently in the urethra, than PT. The overall grade ≥ 2 toxicity rates in the studies above ranged from 7% to 29% for GU and from 6% to 26% for GI. Our corresponding toxicity rates were 7% and 16%, respectively, which are within the ranges produced in these other studies.
The AUA score was the highest at the end of treatment and continued to improve afterwards. The urinary QoL score was also worst at the end of RT and improved afterwards. At 37 months after PT, the urinary QoL was even better than before PT. This finding is consistent with the results of a previous QoL study published by Sanda et al. [Citation22]. Sexual function gradually decreased for the cohorts, which is not unusual after RT. The modified LENT-SOMA GU and GI toxicity scores were not significantly changed, but the rectal bleeding score was worse than the baseline score.
Pollack et al. [Citation20] and Kuban et al. [Citation21] demonstrated that BCFFS (by Nadir + 2 ng/ml) or clinical failure was superior in the 78 Gy arm (78%) compared with the 70 Gy arm (59%) (p = 0.004) at eight years. For the intermediate-risk group, the values were 86% and 76% at eight years, respectively. The corresponding rate in our study was 90% at four years. Kupelian et al. [Citation16] reported the results of hypo-IMRT with 70 Gy (80 Gy EQD2). Approximately 60% of the cohort received neoadjuvant ADT. The ASTRO and Nadir + 2 ng/ml BCFFS values for the intermediate-risk group were 85% and 83% at five years, respectively. The corresponding rates in our study were 85% and 90% at four years, respectively, which are not inferior to those of Kupelian, although neoadjuvant ADT was not given, but the follow-up period was slightly shorter in our study. Madsen et al. [Citation18] reported an ASTRO BCFFS rate of 70% and Nadir + 2 ng/ml BCFFS rate of 90% at four years after IMRT with 77 Gy EQD2. Given that they only included low-risk patients, our treatment outcome for the intermediate-risk group (85% and 90%, respectively) is not inferior to theirs. In summary, our treatment outcome was not inferior to the results of modern high-dose RT in the literature. We could not find any difference in BCFFS among the treatment arms, despite the fact that the EQD2 ranged from 77 to 85 Gy.
A weakness of our study is the small sample size and short follow-up period. Thus, we could not compare the outcomes among the treatment arms. To our knowledge, however, this is the first randomized study using hypofrationationated dose schedules of PT, and we achieved a satisfactory treatment outcome in terms of BCFFS with much less acute toxicity than reported in previous studies.
In conclusion, the hypofractionated dose schedules used in the present study are feasible with an acceptable toxicity profile. As the BCFFS rates do not seem to be inferior to those reported previously, hypofractionated PT could be a viable option to save patients time and money, although further study is warranted to define the optimal dose and fractionation. Thus, a new trial is undergoing to compare biochemical outcomes among the treatment arms.
Supplementary Appendix
Download PDF (1.4 MB)Declaration of interest: The authors report no conflicts of interest. The authors alone are responsible for the content and writing of the paper.
This study was supported by Grant No.1010480 from the National Cancer Center, Korea.
References
- Hausterman K, Fowler JF. A comment on proliferation rates in human prostate cancer. Int J Radiat Oncol Biol Phys 2000;48:303.
- Brenner DJ, Hall EJ. Fractionation and protraction for radiotherapy of prostate carcinoma. Int J Radiat Oncol Biol Phys 1999;43:1095–101.
- Fowler J. What hypofractionated protocols should be tested for prostate cancer? Int J Radiat Oncol Biol Phys 2003; 56:1093–104.
- Fowler J, King CR. Don’t squeeze hypofractionated schedules into too-short overall times. Int J Radiat Oncol Biol Phys 2009;75:323–5.
- Slater JD, Rossi CJ, Jr., Yonemoto LT, Bush DA, Jabola BR, Levy RP, . Proton therapy for prostate cancer: The initial Loma Linda University experience. Int J Radiat Oncol Biol Phys 2004;59:348–52.
- Perez CA, Michalski J, Mansur D, Lockett MA. Impact of elapsed treatment time on outcome of external-beam radiation therapy for localized carcinoma of the prostate. Cancer J 2004;10:349–56.
- Fowler JF, Harari PM, Leborgne F, Leborgne JH. Acute radiation reactions in oral and pharyngeal mucosa: Tolerable levels in altered fractionation schedules. Radiother Oncol 2003;69:161–8.
- Dorr W, Hamilton CS, Boyd T, Reed B, Denham JW. Radiation-induced changes in cellularity and proliferation in human oral mucosa. Int J Radiat Oncol Biol Phys 2002; 52:911–7.
- Yoon M, Kim D, Shin DH, Park SY, Lee SB, Kim DY, . Inter- and intrafractional movement-induced dose reduction of prostate target volume in proton beam treatment. Int J Radiat Oncol Biol Phys 2008;71:1091–102.
- Simon R, Wittes R, Ellenberg S. Randomized phase II clinical trials. Cancer Treat Rep 1985;69:1375.
- Barry MJ, Fowler FJ, Jr., O’Leary MP, Bruskewitz RC, Holtgrewe HL, Mebust WK, . The American Urological Association symptom index for benign prostatic hyperplasia. The Measurement Committee of the American Urological Association. J Urol 1992;148:1549–57; discussion 64.
- LENT SOMA scales for all anatomic sites. Int J Radiat Oncol Biol Phys 1995;31:1049–91.
- Norman GR, Sloan JA, Wyrwich KW. Interpretation of changes in health-related quality of life: The remarkable universality of half a standard deviation. Med Care 2003;41:582–92.
- Arcangeli G, Saracino B, Gomellini S, Petrongari MG, Arcangeli S, Sentinelli S, . A prospective phase III randomized trial of hypofractionation versus conventional fractionation in patients with high-risk prostate cancer. Int J Radiat Oncol Biol Phys 2010;78:11–8.
- Arcangeli G, Fowler J, Gomellini S, Arcangeli S, Saracino B, Petrongari MG, . Acute and late toxicity in a randomized trial of conventional versus hypofractionated three-dimensional conformal radiotherapy for prostate cancer. Int J Radiat Oncol Biol Phys 2011;79:1013–21.
- Kupelian PA, Willoughby TR, Reddy CA, Klein EA, Mahadevan A. Hypofractionated intensity-modulated radiotherapy (70 Gy at 2.5 Gy per fraction) for localized prostate cancer: Cleveland Clinic experience. Int J Radiat Oncol Biol Phys 2007;68:1424–30.
- Martin JM, Rosewall T, Bayley A, Bristow R, Chung P, Crook J, . Phase II trial of hypofractionated image-guided intensity-modulated radiotherapy for localized prostate adenocarcinoma. Int J Radiat Oncol Biol Phys 2007; 69:1084–9.
- Madsen BL, Hsi RA, Pham HT, Fowler JF, Esagui L, Corman J. Stereotactic hypofractionated accurate radiotherapy of the prostate (SHARP), 33.5 Gy in five fractions for localized disease: First clinical trial results. Int J Radiat Oncol Biol Phys 2007;67:1099–105.
- King CR, Brooks JD, Gill H, Pawlicki T, Cotrutz C, Presti Jr JC. Stereotactic body radiotherapy for localized prostate cancer: Interim results of a prospective phase II clinical trial. Int J Radiat Oncol Biol Phys 2009;73: 1043–8.
- Pollack A, Zagars GK, Starkschall G, Antolak JA, Lee JJ, Huang E, . Prostate cancer radiation dose response: Results of the M. D. Anderson phase III randomized trial. Int J Radiat Oncol Biol Phys 2002;53:1097–105.
- Kuban DA, Tucker SL, Dong L, Starkschall G, Huang EH, Cheung MR, . Long-term results of the M. D. Anderson randomized dose-escalation trial for prostate cancer. Int J Radiat Oncol Biol Phys 2008;70:67–74.
- Sanda MG, Dunn RL, Michalski J, Sandler HM, Northouse L, Hembroff L, . Quality of life and satisfaction with outcome among prostate-cancer survivors. N Engl J Med 2008;358:1250–61.