Abstract
The incidence of type 2 diabetes mellitus has markedly increased worldwide over the past decades. Pancreatic β-cell dysfunction as well as central and peripheral insulin resistance appears to be elementary features in the pathophysiology of type 2 diabetes mellitus. Major environmental conditions predisposing to the development of type 2 diabetes are excessive food intake and sedentary life-style on the background of a genetic predisposition. Recent genome-wide association studies identified several novel type 2 diabetes risk genes, with impaired pancreatic β-cell function as the underlying mechanism of increased diabetes risk in the majority of genes. Many of the novel type 2 diabetes risk genes, including MTNR1B which encodes one of the two known human melatonin receptors, were unexpected at first glance. However, previous animal as well as human studies already pointed to a significant impact of the melatonin system on the regulation of glucose homeostasis, in addition to its well known role in modulation of sleep and circadian rhythms.
This brief review aims to give an overview of how alterations in the melatonin system could contribute to an increased diabetes risk, paying special attention to the role of melatonin receptors in pancreatic β-cell function.
Abbreviations | ||
cAMP | = | cyclic adenosine monophosphate |
cGMP | = | cyclic guanosine monophosphate |
GLP-1 | = | glucagon-like peptide-1 |
GWA | = | genome-wide association |
IP3 | = | inositol-1,4,5-trisphosphate |
MTNR1A | = | melatonin receptor 1A |
MTNR1B | = | melatonin receptor 1B |
MTNR1C | = | melatonin receptor 1C |
Key messages
MTNR1B was recently added to the growing list of diabetes genes.
Similar to the majority of novel diabetes genes, variants in MTNR1B confer pancreatic β-cell dysfunction.
Melatonin receptors appear to be involved in insulin secretion, though their role especially in human pancreatic β-cells is only incompletely understood, and the literature regarding this is partly controversial.
Introduction
The incidence of type 2 diabetes mellitus has markedly increased worldwide over the past decades, reaching epidemic proportions with major health consequences at an individual as well as a public health level (Citation1). Although the underlying mechanisms of type 2 diabetes are not completely understood, pancreatic β-cell dysfunction as well as central and peripheral insulin resistance appears to be elementary features in the pathophysiology of type 2 diabetes mellitus (Citation2,Citation3). Major environmental conditions that predispose to the development of type 2 diabetes are excessive food intake and sedentary life-style on the background of a genetic predisposition (Citation4).
Recent genome-wide association (GWA) studies identified a series of new risk loci for type 2 diabetes (Citation5–19). Many of the novel type 2 diabetes risk genes, including MTNR1B which encodes one of the two known human melatonin receptors, were unexpected at first glance. However, previous animal as well as human studies already pointed to a significant impact of the melatonin system on the regulation of glucose homeostasis, in addition to its well known role in modulation of sleep and circadian rhythms (Citation20). In humans, insulin secretion rates and serum insulin concentrations underlie circadian changes, with increasing insulin secretion during the day and decreasing during the night (Citation21). Plasma melatonin levels, a major regulator of the circadian rhythm, behave in the opposite manner, with lowest values when insulin secretion rates peak and vice versa (Citation21). Disturbances of sleep and circadian rhythms, typically found in shift-work and jet lag, can lead to significant changes in energy metabolism and represent, therefore, important mechanisms in the development of obesity and type 2 diabetes (Citation22). In accordance, a simulated phase shift significantly alters pancreatic β-cell responses, postprandial glucose levels, and lipid metabolism (Citation23), and circadian misalignment results in postprandial glucose responses in the range characteristic of a prediabetic state (Citation24).
This brief review aims to give an overview of how alterations in the melatonin system could contribute to an increased diabetes risk, paying special attention to the role of melatonin receptors in pancreatic β-cell function.
Effects of MTNR1B type 2 diabetes risk variants on β-cell function
Several variants within the MTNR1B gene locus, i.e. rs1387153, rs2166706, rs10830962, rs4753426 (all located in the 5′-flanking region), rs10830963, rs3781638 (both located in intron 1), and rs8192552 (located in exon 1, resulting in the non-synonymous mutation G24E)—localization within the locus depicted in —were found to associate with impaired fasting glucose (Citation16,Citation25–35) and increased risk of type 2 diabetes (Citation16,Citation26–29,Citation32,Citation34) in both adult and child populations of different ethnicity. All studied single nucleotide polymorphisms (SNPs) confer impaired fasting glucose, whereas an increased risk of type 2 diabetes was described for rs1387153, rs2166706, and rs10830963 (). Similar to other diabetes risk genes, the odds ratio for presence or later development of type 2 diabetes was moderate, ranging from 1.09 to 1.23 (Citation16,Citation26–29,Citation32,Citation34), supporting the multifactorial genesis of type 2 diabetes with complex interactions of a large quantity of susceptibility genes. The MTNR1B variant rs10830963 that shows considerable consistency over these studies is located within the single 11.5 kb intron of the MTNR1B gene () without interfering with consensus transcription factor binding or alternative splicing (Citation16).
Figure 1. Genomic region of human chromosome 11 harbouring the MTNR1B gene locus and linkage disequilibrium (LD) data of representative single nucleotide polymorphisms (SNPs) within this region (HapMap data). The MTNR1B gene consists of 2 exons and spans 13,160 bases from nucleotide 92,342,437 to nucleotide 92,355,596. The locations of the genotyped representative SNPs are indicated by arrows. The HapMap minor allele frequencies (MAF) are given below the SNP numbers. The Haploview LD colour scheme ‘r-squared’ was chosen to visualize LD data. Within the diamonds, the r2 values are given. SNP rs8192552 (G24E) is not covered by the HapMap data and was added, therefore, on the right side of the diamonds. No linkage data are available for this SNP.
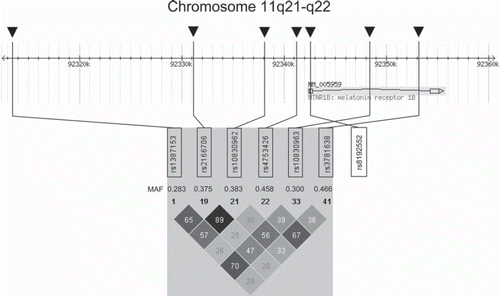
Table I. Associations of single nucleotide polymorphisms within or in proximity to the MTNR1B gene locus with prediabetes phenotypes and risk of type 2 diabetes.
MTNR1B is one of the recently identified 13 diabetes risk genes that confer an impaired pancreatic β-cell function (Citation16,Citation25,Citation26,Citation29,Citation30,Citation33,Citation36–38). MTNR1B variants rs10830962, rs4753426, rs10830963, and rs3781638 associated with reduced insulin secretion, whereas one SNP, i.e. rs3781638, affected insulin sensitivity (Citation25) and one SNP, i.e. rs8192552 (G24E), associated with changes in measures of obesity (Citation35) (). In the latter study, the minor allele of rs8192552 was associated with increased body mass despite decreased fasting plasma glucose levels. The authors hypothesized that these discrepant results may be due to differential regulation of the melatonin receptor in specific target tissues (Citation35).
Given that the described polymorphisms in MTNR1B affect different aspects of prediabetes and that they are only in moderate linkage disequilibrium (LD), as depicted in , these variants probably do not reflect all the same association signal.
Though the underlying mechanisms by which common genetic variation within these loci affects β-cell function are not completely understood, risk variants may alter glucose-stimulated insulin secretion, proinsulin conversion, incretin sensitivity or incretin secretion. Impairments of the following measures of β-cell function were found to be associated with MTNR1B variants: homeostasis model assessment of insulin secretion (Citation16,Citation33), insulin release after oral and intravenous glucose challenges (Citation25,Citation29,Citation36), and, in particular, the early insulin response to both oral and intravenous glucose (Citation26,Citation30,Citation37,Citation38). In carriers of MTNR1B risk variants, faster deterioration of glucose-stimulated insulin secretion during a 7-year follow-up period was reported (Citation26). Furthermore, MTNR1B was one of eight type 2 diabetes genes, summation of which was associated with reduced first-phase glucose-stimulated insulin secretion during hyperglycaemic clamps (Citation39). In a very recent study, the risk allele of the MTNR1B SNP rs10830963 associated with increased insulin responses towards glucagon-like peptide-1 (GLP-1) and arginine stimulation, despite reduced insulin secretion after an oral glucose challenge (Citation36). This finding surprises in so far as incretins, including GLP-1, mediate, at least to some extent, the insulin secretion after oral glucose intake.
Expression and function of melatonin receptors in pancreatic β-cells
MTNR1B (melatonin receptor 1B; OMIM entry no. 600804) encodes one of two known human melatonin receptors, which are MTNR1A, also known as Mel1A or MT1, and MTNR1B, also known as Mel1B or MT2 (Citation40). In amphibia and birds, with MTNR1C, also known as Mel1C or MT3, a third melatonin receptor subtype has been identified (Citation41). MTNR1B is located on the long arm of chromosome 11, contains two coding exons that span about 10 kb, and shares about 60% nucleotide sequence homology with MTNR1A (Citation40). Both receptors belong to the G protein-coupled receptor family which is characterized by seven transmembrane domains, an extracellular N-terminal domain, and an intracellular C-terminal domain. While the MTNR1A subtype is expressed in the pars tuberalis of the pituitary gland, the suprachiasmatic nuclei of the hypothalamus, and the paraventricular thalamus, the MTNR1B subtype is present in rodent suprachiasmatic nuclei and hippocampus as well as in human retina and, to a lesser extent, brain (Citation42).
Furthermore, melatonin receptors have been detected in neonatal rat pancreas (Citation43), in the insulin-secreting rodent insulinoma cell lines INS-1 and MIN-6 (Citation44,Citation45), as well as in rat and human islets and pancreatic β-cells (Citation46,Citation47). Early studies showed a significantly lower MTNR1B expression in comparison with MTNR1A expression in human and rat islets as well as in the murine MIN-6 cell line (Citation46,Citation47). Analysis of MTNR1A mRNA content at the single cell level showed its expression in human pancreatic α-cells, but not in β-cells (Citation47). In a very recent study, the occurrence of MTNR1A and MTNR1B in human islets as well as in clonal β-cells was confirmed (Citation26). However, in contrast to previous studies (Citation46,Citation47), both melatonin receptors were almost equally expressed in human islets (Citation26). While MTNR1B was predominantly detected in β-cells in both human and rodent islets, MTNR1A was present only in a population of peripherally located β-cells in human, mouse, and rat islets (Citation26).
In the vast majority of in-vitro studies, treatment of pancreatic β-cells with melatonin attenuated insulin secretion (Citation43–45,Citation48,Citation49), though a limited number of studies in pancreatic islets did not reveal inhibitory (Citation50) but even stimulatory effects (Citation47) of melatonin on β-cell function. A potential explanation for the discrepant results is that the stimulatory action of melatonin on insulin release that has been observed in one study most probably resulted indirectly from melatonin-induced glucagon secretion (Citation47). In agreement with the negative actions of melatonin on β-cell function in vitro, in animal studies, pinealectomy caused severe hyperinsulinaemia (Citation51) and a significant insulin hypersecretion in isolated pancreatic islets (Citation49,Citation52), whereas exogenous application of melatonin decreased insulin plasma levels (Citation53). Besides, very recently insulin secretion from isolated islets of MTNR1A, MTNR1B, or MTNR1A and MTNR1B double-knockout animals was found to be enhanced compared to wild-type islets (Citation54). In line with these findings, over-expression of melatonin receptors has been found in islets from patients with type 2 diabetes in comparison with non-diabetic subjects (Citation55).
The two melatonin receptors, MTNR1A and MTNR1B, on pancreatic β-cells are linked to three highly divergent signalling pathways, each with different impact on insulin secretion. The adenylyl cyclase/cyclic adenosine monophosphate (cAMP) pathway predominates regarding insulin liberation from pancreatic β-cells (Citation56). MTNR1A as well as MTNR1B are coupled to the pertussis toxin-sensitive inhibitory Giα protein that can inhibit cAMP-dependent signalling and subsequently insulin secretion (Citation40,Citation49). The importance of pertussis toxin-sensitive inhibitory G proteins as regulators of insulin secretion has been underscored by a recent mouse study showing that pancreatic β-cell-specific inactivation of the inhibitory G proteins (Gi/o) resulted in constitutive hyperinsulinaemia, increased insulin secretion in response to glucose, and resistance to diet-induced hyperglycaemia (Citation57). This study identified several highly expressed Gi/o-coupled receptors, such as α2A-adrenoceptor, glucagon-like peptide-1 (GLP-1) receptor, and glucose-dependent insulinotropic peptide (GIP) receptor, in pancreatic β-cells, though MTNR1B was not among those. While acute treatment with melatonin reduced the forskolin- and GLP-1-stimulated cAMP formation and insulin secretion in pancreatic β-cells (Citation44,Citation45), after prolonged exposure to melatonin treatment cAMP-mediated responses to forskolin and GLP-1 were enhanced (Citation45) due to the well known G protein-coupled receptor desensitization mechanisms in which G protein-coupled receptor kinases are involved (Citation58). Inhibition of the guanylate cyclase/cyclic guanosine monophosphate (cGMP) pathway following activation of the MTNR1A (but not MTNR1B) is a further mechanism for inhibitory effects of melatonin on insulin secretion (Citation59). Though less relevant than the aforementioned pathways, melatonin exerts its biological effects on the pancreatic β-cell also via MTNR1A-dependent, but Giα-independent activation of phospholipase C and release of its second messenger inositol-1,4,5-trisphosphate (IP3). Binding of IP3 to its cognate receptor results in opening of calcium channels and an increase in intracellular calcium, thereby facilitating insulin secretion from pancreatic β-cells (Citation60). Thus, the actions of melatonin on pancreatic β-cells and on insulin release result from a complex interplay of intracellular signal transduction cascades, which comprise the cAMP-, cGMP-, and IP3-signalling pathways. Furthermore, effects of melatonin on pancreatic β-cells appear to be species-specifically regulated, given that in one study melatonin did not alter the cAMP content in human islets, whereas melatonin treatment reduced cAMP formation and, subsequently, insulin secretion in murine MIN-6 cells (Citation47).
In this context it is important to note that the use of nocturnal animals as model organisms is limited, in so far that in these animals melatonin levels are high during their active period, in contrast to humans where they are highest during the non-active period. These differences could explain some of the controversial data on melatonin and glucose metabolism found in humans and in murine animal models.
Although in-vitro studies have clearly shown direct effects of melatonin on pancreatic β-cell function, it cannot be excluded that there are also other effects of melatonin that indirectly influence β-cell function and glucose homeostasis. This assumption is supported by the expression of the melatonin receptors in tissues with crucial functions in carbohydrate metabolism, such as adipose tissue, skeletal muscle, liver, and hypothalamus (Citation61,Citation62). Previous studies in human brown adipocytes pointed to an important role of melatonin in adipocyte physiology, including glucose transporter 4 expression and glucose uptake, through MTNR1B activation (Citation63). The impact of melatonin through melatonin receptor activation on early events of the insulin-dependent cascade, such as the insulin receptor substrate-1 (IRS-1)/phosphatidylinositol 3-kinase (PI3K) pathway, as well as on end-points of insulin action, including glucose transport, have been also described in murine skeletal muscle cells (Citation64). In line with this, in a very recent mouse study, functional knock-out of MTNR1A significantly impaired glucose metabolization, probably due to increased insulin resistance (Citation65). Melatonin-induced activation of insulin signalling pathways, such as the IRS-1/PI3K or the IRS-1/mitogen-activated protein kinase (MAPK) pathways, most likely via MTNR1B, were also found in rat hypothalamic suprachiasmatic nucleus which directly controls the circadian rhythm of plasma glucose concentration (Citation66). As shown in hepatic HepG2 cells, melatonin modulates central mechanisms of insulin action in the liver, such as glycogen synthesis, via a protein kinase C (PKC)-ζ/Akt/glycogen synthase kinase 3β (GSK3-β) pathway (Citation67). In agreement with these preclinical data, two recent genetic studies showed associations of MTNR1B variants with measures of insulin sensitivity (Citation25) and body mass (Citation35).
Future outlook
In light of the inhibiting actions of melatonin on insulin secretion in pancreatic β-cells (Citation68), the increased expression of the melatonin receptor MTNR1B in islets of subjects with type 2 diabetes (Citation26,Citation69), and the association between common variants in MTNR1B and type 2 diabetes risk (Citation26–29,Citation31,Citation32), MTNR1B appears to be a potential target for the development of anti-diabetic therapies. Treatment with melatonin receptor antagonists, such as the non-selective melatonin receptor antagonist luzindole (N-acetyl-2-benzyltryptamine) as well as the MTNR1B-specific antagonist 4P-PDOT (4-phenyl-2-propionamidotetraline), reversed the inhibiting effect of melatonin on insulin secretion in pancreatic β-cells (Citation68). Both melatonin receptor antagonists proved effectively to ameliorate melatonin actions also in animal models (Citation66). However, while melatonin agonists, such as agomelatine (Valdoxan™), have become mainstays in the current treatment of depression (Citation70), preclinical studies on melatonin receptor antagonists are not yet at the point where clinical trials can be recommended.
Conclusions
MTNR1B was recently added to the growing list of diabetes genes. Variants within the MTNR1B gene locus were found to associate with impaired fasting glucose and increased risk of type 2 diabetes in both adult and child populations of different ethnicity. Similar to the majority of novel diabetes genes, variants in MTNR1B confer pancreatic β-cell dysfunction. These associations appear highly plausible, in light of substantial evidence in human and animal studies linking disturbances of circadian rhythms and sleep to metabolic disorders, such as diabetes and obesity, the significant involvement of the melatonin system in the regulation of glucose homeostasis, and the, though not completely understood, role of the melatonin receptors in pancreatic β-cell function and, in particular, insulin secretion.
Declaration of interest: The authors state no conflicts of interest and have received no payment in preparation of this manscript.
References
- Wild S, Roglic G, Green A, Sicree R, King H. Global prevalence of diabetes: estimates for the year 2000 and projections for 2030. Diabetes Care. 2004;27:1047–53.
- Kahn SE. The relative contributions of insulin resistance and beta-cell dysfunction to the pathophysiology of Type 2 diabetes. Diabetologia. 2003;46:3–19.
- DeFronzo RA, Bonadonna RC, Ferrannini E. Pathogenesis of NIDDM. A balanced overview. Diabetes Care. 1992; 15:318–68.
- Lieberman LS. Dietary, evolutionary, and modernizing influences on the prevalence of type 2 diabetes. Annu Rev Nutr. 2003;23:345–77.
- Sladek R, Rocheleau G, Rung J, Dina C, Shen L, Serre D, . A genome-wide association study identifies novel risk loci for type 2 diabetes. Nature. 2007;445:881–5.
- ; Diabetes Genetics Initiative of Broad Institute of Harvard and MIT, Lund University, and Novartis Institutes of BioMedical ResearchSaxena R, Voight BF, Lyssenko V, Burtt NP, de Bakker PI, Chen H, . Genome-wide association analysis identifies loci for type 2 diabetes and triglyceride levels. Science. 2007;316:1331–6.
- Zeggini E, Weedon MN, Lindgren CM, Frayling TM, Elliott KS, Lango H, . Replication of genome-wide association signals in UK samples reveals risk loci for type 2 diabetes. Science. 2007;316:1336–41.
- Scott LJ, Mohlke KL, Bonnycastle LL, Willer CJ, Li Y, Duren WL, . A genome-wide association study of type 2 diabetes in Finns detects multiple susceptibility variants. Science. 2007;316:1341–5.
- Steinthorsdottir V, Thorleifsson G, Reynisdottir I, Benediktsson R, Jonsdottir T, Walters GB, . A variant in CDKAL1 influences insulin response and risk of type 2 diabetes. Nat Genet. 2007;39:770–5.
- Frayling TM, Timpson NJ, Weedon MN, Zeggini E, Freathy RM, Lindgren CM, . A common variant in the FTO gene is associated with body mass index and predisposes to childhood and adult obesity. Science. 2007;316:889–94.
- Gudmundsson J, Sulem P, Steinthorsdottir V, Bergthorsson JT, Thorleifsson G, Manolescu A, . Two variants on chromosome 17 confer prostate cancer risk, and the one in TCF2 protects against type 2 diabetes. Nat Genet. 2007;39:977–83.
- Sandhu MS, Weedon MN, Fawcett KA, Wasson J, Debenham SL, Daly A, . Common variants in WFS1 confer risk of type 2 diabetes. Nat Genet. 2007;39:951–3.
- Zeggini E, Scott LJ, Saxena R, Voight BF, Marchini JL, Hu T, . Meta-analysis of genome-wide association data and large-scale replication identifies additional susceptibility loci for type 2 diabetes. Nat Genet. 2008;40:638–45.
- Unoki H, Takahashi A, Kawaguchi T, Hara K, Horikoshi M, Andersen G, . SNPs in KCNQ1 are associated with susceptibility to type 2 diabetes in East Asian and European populations. Nat Genet. 2008;40:1098–102.
- Yasuda K, Miyake K, Horikawa Y, Hara K, Osawa H, Furuta H, . Variants in KCNQ1 are associated with susceptibility to type 2 diabetes mellitus. Nat Genet. 2008;40:1092–7.
- Prokopenko I, Langenberg C, Florez JC, Saxena R, Soranzo N, Thorleifsson G, . Variants in MTNR1B influence fasting glucose levels. Nat Genet. 2009;41:77–81.
- Rung J, Cauchi S, Albrechtsen A, Shen L, Rocheleau G, Cavalcanti-Proença C, . Genetic variant near IRS1 is associated with type 2 diabetes, insulin resistance and hyperinsulinemia. Nat Genet. 2009;41:1110–5.
- Dupuis J, Langenberg C, Prokopenko I, Saxena R, Soranzo N, Jackson AU, . New genetic loci implicated in fasting glucose homeostasis and their impact on type 2 diabetes risk. Nat Genet. 2010;42:105–16.
- Qi L, Cornelis MC, Kraft P, Stanya KJ, Linda Kao WH, Pankow JS, . Genetic variants at 2q24 are associated with susceptibility to type 2 diabetes. Hum Mol Genet. 2010;19:2706–15.
- Peschke E. Melatonin, endocrine pancreas and diabetes. J Pineal Res. 2008;44:26–40.
- Boden G, Ruiz J, Urbain JL, Chen X. Evidence for a circadian rhythm of insulin secretion. Am J Physiol. 1996; 271:E246–52.
- Laposky AD, Bass J, Kohsaka A, Turek FW. Sleep and circadian rhythms: key components in the regulation of energy metabolism. FEBS Lett. 2008;582:142–51.
- Hampton SM, Morgan LM, Lawrence N, Anastasiadou T, Norris F, Deacon S, . Postprandial hormone and metabolic responses in simulated shift work. J Endocrinol. 1996;151:259–67.
- Scheer FA, Hilton MF, Mantzoros CS, Shea SA. Adverse metabolic and cardiovascular consequences of circadian misalignment. Proc Natl Acad Sci U S A. 2009;106:4453–8.
- Staiger H, Machicao F, Schafer SA, Kirchhoff K, Kantartzis K, Guthoff M, . Polymorphisms within the novel type 2 diabetes risk locus MTNR1B determine beta-cell function. PLoS One. 2008;3:e3962.
- Lyssenko V, Nagorny CL, Erdos MR, Wierup N, Jonsson A, Spégel P, . Common variant in MTNR1B associated with increased risk of type 2 diabetes and impaired early insulin secretion. Nat Genet. 2009;41:82–8.
- Bouatia-Naji N, Bonnefond A, Cavalcanti-Proenca C, Sparsø T, Holmkvist J, Marchand M, . A variant near MTNR1B is associated with increased fasting plasma glucose levels and type 2 diabetes risk. Nat Genet. 2009;41:89–94.
- Ronn T, Wen J, Yang Z, Lu B, Du Y, Groop L, . A common variant in MTNR1B, encoding melatonin receptor 1B, is associated with type 2 diabetes and fasting plasma glucose in Han Chinese individuals. Diabetologia. 2009;52:830–3.
- Sparso T, Bonnefond A, Andersson E, Bouatia-Naji N, Holmkvist J, Wegner L, . G-allele of intronic rs10830963 in MTNR1B confers increased risk of impaired fasting glycemia and type 2 diabetes through an impaired glucose-stimulated insulin release: studies involving 19,605 Europeans. Diabetes. 2009;58:1450–6.
- Langenberg C, Pascoe L, Mari A, Tura A, Laakso M, Frayling TM, . Common genetic variation in the melatonin receptor 1B gene (MTNR1B) is associated with decreased early-phase insulin response. Diabetologia. 2009; 52:1537–42.
- Reiling E, van't Riet E, Groenewoud MJ, Welschen LM, van Hove EC, Nijpels G, . Combined effects of single-nucleotide polymorphisms in GCK, GCKR, G6PC2 and MTNR1B on fasting plasma glucose and type 2 diabetes risk. Diabetologia. 2009;52:1866–70.
- Chambers JC, Zhang W, Zabaneh D, Sehmi J, Jain P, McCarthy MI, . Common genetic variation near melatonin receptor MTNR1B contributes to raised plasma glucose and increased risk of type 2 diabetes among Indian Asians and European Caucasians. Diabetes. 2009;58:2703–8.
- Kelliny C, Ekelund U, Andersen LB, Brage S, Loos RJ, Wareham NJ, . Common genetic determinants of glucose homeostasis in healthy children: the European Youth Heart Study. Diabetes. 2009;58:2939–45.
- Takeuchi F, Katsuya T, Chakrewarthy S, Yamamoto K, Fujioka A, Serizawa M, . Common variants at the GCK, GCKR, G6PC2-ABCB11 and MTNR1B loci are associated with fasting glucose in two Asian populations. Diabetologia. 2010;53:299–308.
- Andersson EA, Holst B, Sparso T, Grarup N, Banasik K, Holmkvist J, . The MTNR1B G24E variant associates with BMI and fasting plasma glucose in the general population in studies of 22,142 Europeans. Diabetes. 2010;59: 1539–48.
- Simonis-Bik AM, Nijpels G, van Haeften TW, Houwing-Duistermaat JJ, Boomsma DI, Reiling E, . Gene variants in the novel type 2 diabetes loci CDC123/CAMK1D, THADA, ADAMTS9, BCL11A, and MTNR1B affect different aspects of pancreatic beta-cell function. Diabetes. 2010;59:293–301.
- Stancakova A, Kuulasmaa T, Paananen J, Jackson AU, Bonnycastle LL, Collins FS, . Association of 18 confirmed susceptibility loci for type 2 diabetes with indices of insulin release, proinsulin conversion, and insulin sensitivity in 5,327 nondiabetic Finnish men. Diabetes. 2009;58:2129–36.
- Ingelsson E, Langenberg C, Hivert MF, Prokopenko I, Lyssenko V, Dupuis J, . Detailed physiologic characterization reveals diverse mechanisms for novel genetic loci regulating glucose and insulin metabolism in humans. Diabetes. 2010;59:1266–75.
- 't Hart LM, Simonis-Bik AM, Nijpels G, van Haeften TW, Schäfer SA, Houwing-Duistermaat JJ, . Combined risk allele score of eight type 2 diabetes genes is associated with reduced first-phase glucose-stimulated insulin secretion during hyperglycemic clamps. Diabetes. 2010;59:287–92.
- Reppert SM, Godson C, Mahle CD, Weaver DR, Slaugenhaupt SA, Gusella JF. Molecular characterization of a second melatonin receptor expressed in human retina and brain: the Mel1b melatonin receptor. Proc Natl Acad Sci U S A. 1995;92:8734–8.
- Reppert SM, Weaver DR, Cassone VM, Godson C, Kolakowski LF Jr. Melatonin receptors are for the birds: molecular analysis of two receptor subtypes differentially expressed in chick brain. Neuron. 1995;15:1003–15.
- von Gall C, Stehle JH, Weaver DR. Mammalian melatonin receptors: molecular biology and signal transduction. Cell Tissue Res. 2002;309:151–62.
- Peschke E, Fauteck JD, Musshoff U, Schmidt F, Beckmann A, Peschke D. Evidence for a melatonin receptor within pancreatic islets of neonate rats: functional, autoradiographic, and molecular investigations. J Pineal Res. 2000;28:156–64.
- Peschke E, Muhlbauer E, Musshoff U, Csernus VJ, Chankiewitz E, Peschke D. Receptor (MT(1)) mediated influence of melatonin on cAMP concentration and insulin secretion of rat insulinoma cells INS-1. J Pineal Res. 2002;33:63–71.
- Kemp DM, Ubeda M, Habener JF. Identification and functional characterization of melatonin Mel 1a receptors in pancreatic beta cells: potential role in incretin-mediated cell function by sensitization of cAMP signaling. Mol Cell Endocrinol. 2002;191:157–66.
- Muhlbauer E, Peschke E. Evidence for the expression of both the MT1- and in addition, the MT2-melatonin receptor, in the rat pancreas, islet and beta-cell. J Pineal Res. 2007;42:105–6.
- Ramracheya RD, Muller DS, Squires PE, Brereton H, Sugden D, Huang GC, . Function and expression of melatonin receptors on human pancreatic islets. J Pineal Res. 2008;44:273–9.
- Peschke E, Peschke D, Hammer T, Csernus V. Influence of melatonin and serotonin on glucose-stimulated insulin release from perifused rat pancreatic islets in vitro. J Pineal Res. 1997;23:156–63.
- Picinato MC, Haber EP, Cipolla-Neto J, Curi R, Oliveira Carvalho CR, Carpinelli AR. Melatonin inhibits insulin secretion and decreases PKA levels without interfering with glucose metabolism in rat pancreatic islets. J Pineal Res. 2002;33:156–60.
- Frankel BJ, Strandberg MJ. Insulin release from isolated mouse islets in vitro: no effect of physiological levels of melatonin or arginine vasotocin. J Pineal Res. 1991;11:145–8.
- Nishida S. Metabolic effects of melatonin on oxidative stress and diabetes mellitus. Endocrine. 2005;27:131–6.
- Gorray KC, Quay WB, Ewart RB. Effects of pinealectomy and pineal incubation medium and sonicates on insulin release by isolated pancreatic islets in vitro. Horm Metab Res. 1979;11:432–6.
- Rasmussen DD, Boldt BM, Wilkinson CW, Yellon SM, Matsumoto AM. Daily melatonin administration at middle age suppresses male rat visceral fat, plasma leptin, and plasma insulin to youthful levels. Endocrinology. 1999;140:1009–12.
- Muhlbauer E, Gross E, Labucay K, Wolgast S, Peschke E. Loss of melatonin signalling and its impact on circadian rhythms in mouse organs regulating blood glucose. Eur J Pharmacol. 2009;606:61–71.
- Peschke E, Stumpf I, Bazwinsky I, Litvak L, Dralle H, Muhlbauer E. Melatonin and type 2 diabetes—a possible link? J Pineal Res. 2007;42:350–8.
- Peschke E, Bach AG, Muhlbauer E. Parallel signaling pathways of melatonin in the pancreatic beta-cell. J Pineal Res. 2006;40:184–91.
- Regard JB, Kataoka H, Cano DA, Camerer E, Yin L, Zheng YW, . Probing cell type-specific functions of Gi in vivo identifies GPCR regulators of insulin secretion. J Clin Invest. 2007;117:4034–43.
- Gainetdinov RR, Premont RT, Bohn LM, Lefkowitz RJ, Caron MG. Desensitization of G protein-coupled receptors and neuronal functions. Annu Rev Neurosci. 2004;27:107–44.
- Petit L, Lacroix I, de Coppet P, Strosberg AD, Jockers R. Differential signaling of human Mel1a and Mel1b melatonin receptors through the cyclic guanosine 3′-5′-monophosphate pathway. Biochem Pharmacol. 1999;58:633–9.
- Bach AG, Wolgast S, Muhlbauer E, Peschke E. Melatonin stimulates inositol-1,4,5-trisphosphate and Ca2+ release from INS1 insulinoma cells. J Pineal Res. 2005;39:316–23.
- Sallinen P, Saarela S, Ilves M, Vakkuri O, Leppaluoto J. The expression of MT1 and MT2 melatonin receptor mRNA in several rat tissues. Life Sci. 2005;76:1123–34.
- Sanchez-Hidalgo M, Guerrero Montavez JM, Carrascosa-Salmoral MP, Naranjo Gutierrez MC, Lardone PJ, de la Lastra Romero CA. Decreased MT1 and MT2 melatonin receptor expression in extrapineal tissues of the rat during physiological aging. J Pineal Res. 2009;46:29–35.
- Brydon L, Petit L, Delagrange P, Strosberg AD, Jockers R. Functional expression of MT2 (Mel1b) melatonin receptors in human PAZ6 adipocytes. Endocrinology. 2001;142: 4264–71.
- Ha E, Yim SV, Chung JH, Yoon KS, Kang I, Cho YH, . Melatonin stimulates glucose transport via insulin receptor substrate-1/phosphatidylinositol 3-kinase pathway in C2C12 murine skeletal muscle cells. J Pineal Res. 2006;41:67–72.
- Contreras-Alcantara S, Baba K, Tosini G. Removal of melatonin receptor type 1 induces insulin resistance in the mouse. Obesity (Silver Spring). 2010 Feb 18 (Epub ahead of print).
- Anhe GF, Caperuto LC, Pereira-Da-Silva M, Souza LC, Hirata AE, Velloso LA, . In vivo activation of insulin receptor tyrosine kinase by melatonin in the rat hypothalamus. J Neurochem. 2004;90:559–66.
- Shieh JM, Wu HT, Cheng KC, Cheng JT. Melatonin ameliorates high fat diet-induced diabetes and stimulates glycogen synthesis via a PKCzeta-Akt-GSK3beta pathway in hepatic cells. J Pineal Res. 2009;47:339–44.
- Stumpf I, Muhlbauer E, Peschke E. Involvement of the cGMP pathway in mediating the insulin-inhibitory effect of melatonin in pancreatic beta-cells. J Pineal Res. 2008;45:318–27.
- Peschke E, Frese T, Chankiewitz E, Peschke D, Preiss U, Schneyer U, . Diabetic Goto Kakizaki rats as well as type 2 diabetic patients show a decreased diurnal serum melatonin level and an increased pancreatic melatonin-receptor status. J Pineal Res. 2006;40:135–43.
- Dubovsky SL, Warren C. Agomelatine, a melatonin agonist with antidepressant properties. Expert Opin Investig Drugs. 2009;18:1533–40.