Abstract
The tight glycemic control required to attenuate chronic complications in type 1 diabetes mellitus requires multiple daily injections of bolus insulin which cause hyperinsulinemic edema and hypertension due to Na+ retention. Reports indicate that pectin insulin (PI)-containing dermal patches sustain controlled insulin release into the bloodstream of streptozotocin (STZ)-induced diabetic rats. This study investigated whether PI dermal patches can improve the impaired renal function in diabetes. PI patches were prepared by dissolving pectin/insulin in deionized water and solidified with CaCl2. Short-term (five weeks) effects of thrice daily treatments with PI patches on renal function and urinary glucose outputs were assessed in diabetic animals. Blood and kidney samples were collected after five weeks for measurements of selected biochemical parameters. Blood was also collected for insulin measurement 6 h following treatments. The low plasma insulin concentrations exhibited by STZ-induced diabetic rats were elevated by the application of insulin-containing dermal patches to levels comparable with control non-diabetic rats. Untreated STZ-induced diabetic rats exhibited elevated urinary glucose, K+ outputs and depressed urinary Na+ outputs throughout the 5-week period. Treatment with PI dermal patches increased urinary Na+ output and reduced urine flow, urinary glucose and K+ excretion rates in weeks 4 and 5. PI dermal patches increased GFR of diabetic rats with concomitant reduction of plasma creatinine concentrations. Transdermal insulin treatment also decreased the renal expressions of GLUT1 and SGLT1 of STZ-induced diabetic rats. We conclude that PI dermal patches deliver physiologically relevant amounts of insulin that can improve kidney function in diabetes.
Introduction
Hyperglycemia leads to the development and progression of end-stage renal disease (ESRD), the principal cause of death as a result of diabetic nephropathy (DN). Clinical trials suggest that there is no effective treatment for diabetic nephropathy and the prevention of the occurrence and progression of DN have become a serious medical challenge.Citation1 DN is precipitated directly or indirectly via four main molecular mechanisms which include oxidative stress, protein kinase C (PKC), polyol/aldose reductase and advanced glycation end product (AGE)—receptor of AGE (RAGE) pathways and the renin-angiotensin system.Citation2 These pathways metabolize excess glucose to toxic metabolites that perturb intra-renal hemodynamics via glycosylation of intra-renal proteins which induce hyperfiltration and glomerular dysfunction.Citation3–5 Much emphasis on diabetes care and management is on optimal blood glucose control to avert these adverse outcomes. Literature evidence indicates that intensive insulin therapy either via multiple daily injections or continuous subcutaneous insulin (sc insulin) infusion is more effective in protecting renal function than conventional insulin therapy.Citation6,Citation7 The main limitations of these treatments include adherence to diet, particularly as regards to carbohydrate ingestion, the most important measure in avoiding hypoglycemic events. Furthermore, reports indicate that the intensive therapy is associated with increased Na+ retention resulting in hyperinsulinemic edema and hypertension.Citation8 Other therapeutic interventions such as metformin, insulin secretagogues do not achieve glycemic targets and thus the search for new treatment strategies is ongoing. We have reported that insulin-containing dermal patch formulation sustains slow controlled release of insulin into the bloodstream of diabetic rats with a concomitant reduction of blood glucose concentrations.Citation9 The focus of this paper was to determine whether sustained controlled insulin release from insulin-containing dermal patches can improve the impaired renal fluid and electrolyte handling of streptozotocin (STZ)-induced diabetic rats. Several studies have reported compromised renal function in experimental diabetesCitation10,Citation11 and diabetic patients.Citation12–14
The insulin-responsive facilitative glucose transporters, SGLT1 and GLUT1 found in the proximal convoluted tubule are responsible for re-absorbing filtered glucose from the tubule back into circulation.Citation15 These co-transporters are up-regulated in the diabetic kidneyCitation16,Citation17 and result in greater re-absorption of glucose and thus contributing to the pre-existing hyperglycemia. The up-regulation of these transporters has been implicated in the pathogenesis of progressive diabetic nephropathy.Citation18 Accordingly, we determined whether transdermally delivered insulin has any effect on renal expression of glucose transporters of STZ-induced diabetic rats. The study was designed to establish whether application of pectin insulin-containing dermal patches which sustain controlled release of insulin into the bloodstream of STZ-induced diabetic rats can modulate kidney dysfunction associated with diabetes mellitus as assessed by effects on renal fluid and electrolyte handling in STZ-induced diabetic rats.
Materials and methods
Drugs and chemicals
Amidate low-methoxyl pectin with a degree of methoxylation (DM) of 23, degree of amidation (DE) of 24 was donated by Dr Hans-Ulrich Endress of Herbstreith and Fox KG, Neuenburg, Germany. Drugs were sourced from standard pharmaceutical suppliers. All other chemicals which were of analytical grade quality were purchased from standard commercial suppliers.
Pectin insulin (PI) patch preparation
The amidated pectin hydrogel matrix patch was prepared using a previously described protocol described by Musabayane et al. with slight modifications.Citation19 Briefly, amidated low methoxyl pectin was dissolved in deionized water (4 g/100 mL) and mixed with agitation for 30 min. Subsequently, DMSO (3 mL), eucalyptus oil (1.5 mL), vitamin E (1.5 mL) and puromycin (100 µL) were added to the mixture which was left to mix with agitation for another 30 min after which various amounts of insulin (Novo Rapid Pen Refill, Novo Nordisk Pty Ltd., Sandton, South Africa) were added and mixed with agitation in separate beakers in water bath at 37 °C for 15 min. Following this, an aliquot of the mixture (11 mL) was transferred to a petri dish with a known diameter and a 2% CaCl2 solution was added on top of pectin and left to stand at room temperature for 10 min to allow for cross-linking and hence formation of the matrix patch. Preliminary studies indicated that the patches contained 1.20, 2.87 and 5.04 µg of insulin which translated to dosages of 3.99, 9.57 and 16.80 µg/kg, respectively.
Dissolution studies
The pectin hydrogel matrix dermal patches of the same size were dissolved in Sorenson’s phosphate buffer (pH 7.2) to determine the amount of insulin incorporated. The percentage uptake of insulin into the patch was calculated by dividing the theoretical insulin uptake by the actual insulin measured.
Animals
Male Sprague-Dawley rats (250–300 g, bred at the Biomedical Research Unit, University of KwaZulu-Natal) were used in this study. The animals were kept and maintained under standard laboratory conditions of temperature, humidity, 12 h day:12 h night cycle and allowed water ad libitum and given 30 g standard rat chow daily (Meadow Feeds, Pietermaritzburg, South Africa). All animal experimentation was reviewed and approved by the Animal Ethics Committee of the University of KwaZulu-Natal (102/11/Animal).
Induction of diabetes mellitus
Type 1 diabetes mellitus was induced by a single intraperitoneal injection of 60 mg/kg STZ in freshly prepared 0.1 M citrate buffer (pH 6.3). Control group received the vehicle, citrate buffer through the same route. Animals that exhibited glucosuria after 24 h, tested by urine strips (Rapidmed Diagnostics, Sandton, South Africa) were considered diabetic. Seven days later, the blood glucose concentration of STZ-induced diabetic rats greater than 20 mmol/L was considered as indicating stable diabetes.
Application of the hydrogel patch
Rats were shaved on the dorsal region of neck 1–2 days prior to the application of PI hydrogel matrix patches. The dermal patches were secured in place with adhesive hydro film (Hartman-Congo Inc., Rock Hill, South Carolina, USA) and rat jackets (Braintree, Scientific, Inc., Braintree, Massachusetts, USA) which were adjusted for the size of the animal.
Short-term studies
Studies were conducted in groups of STZ-induced diabetic rats housed individually in Makrolon polycarbonate metabolic cages (Tecniplast, Labotec, South Africa) over a 5-week period. Various doses of insulin (3.99, 9.57 and 16.80 µg/kg) were applied topically thrice daily via PI insulin matrices onto the shaved skin area on the back of the neck skin at 09 h00 followed by the same dose at 17 h00 and the 8 h later (01 h00). Animals treated with drug-free pectin and sc insulin (175 µg/kg) acted as negative and positive controls, respectively. The amounts of food and water consumed were recorded daily at 09 h00. The weights of the animals were measured once every week.
Renal studies
Urine volume and urinary concentrations of glucose, creatinine, urea, Na+, K+ and Cl− were determined daily while mean arterial blood pressure (MAP) was monitored every third consecutive day using non-invasive tail cuff method with photoelectric sensors (IITC Model 31 Computerized Blood Pressure Monitor, Life Sciences, Woodland Hills, CA). The unit works with IITC hardware system to measure blood pressure and heart rate in conscious rats. The animals were warmed at ±30 °C in an enclosed chamber (IITC Model 303sc Animal Test Chamber IITC Life Sciences, Woodland Hills, CA) for 30 min before taking blood pressure readings. All measurements were conducted at 09h00. Blood samples were collected by cardiac puncture into individual pre-cooled heparinized containers at the end of the 5-week experimental period for biochemical analysis. Glomerular filtration rate (GFR), as assessed by creatinine clearance (CCr) was calculated using the standard formulae from measurements of the plasma and urinary concentrations of creatinine and urine flow rate in the fifth week.
Urinalysis
Urine flow was determined gravimetrically. Na+, K+, urea and creatinine were analyzed using the Beckman Coulter Counter (Synchron CX3 Clinical Systems, Fullerton, California, USA) with commercial diagnostic kits from Beckman Coulter, Dublin Ireland. Glucose was measured using a glucometer (Bayer’s Glucometer Elite® Elite (Pty) Ltd., Health Care Division, Isando, South Africa).
Laboratory analyses insulin
At the end of 6 h following treatment with various doses of PI patches (3.99, 9.57 and 16.80 µg/kg); animals were sacrificed by exposing to halothane for 3 min via a gas anaesthetic chamber (100 mg/kg). Blood was collected by cardiac puncture into pre-cooled heparinized tubes for insulin determination. The plasma insulin concentrations were measured by ultrasensitive rat insulin ELISA kit (DRG Instruments GmBH, Marburg, Germany). The immunoassay is a quantitative method utilizing two monoclonal antibodies which together are specific for insulin. The lower limit of detection was 1.74 pmol/L. The intra- and inter-assay analytical coefficients of variation ranged from 4.4 to 5.5% and from 4.7 to 8.9%, respectively.
Arginine vasopressin
Blood samples for arginine vasopressin (AVP) measurements were also collected and treated as described for insulin from STZ-induced diabetic rats after five weeks of transdermal treatment with PI patch. Plasma AVP concentrations were determined by standard enzymatic methods using an arg8-vasopressin ELISA Kit (Abcam, Cambridge, Massachusetts, USA). The lower and upper limits of detection were 4 pmol/L and 923 pmol/L, respectively. The intra-assay analytical coefficient of variation ranged from 5.9 to 10.6% and the inter-assay coefficient variation from 6.0 to 8.5%. In all cases, rats treated with insulin free pectin patch or sc insulin (175 µg/kg) acted as untreated and treated positive controls, respectively (n = 6 in each group). Separated plasma was analyzed for AVP, Na+, K+, creatinine and urea concentrations. Glomerular filtration rate (GFR), as assessed by creatinine clearance (CCr) was calculated using the standard formulae from measurements of the plasma and urinary concentrations of creatinine and urine flow rate in the fifth week.
Kidney histology
At the end of the five weeks, control non-diabetic, untreated STZ-induced diabetic rats and treated STZ-induced diabetic rats were killed by exposing to halothane via a gas anaesthetic chamber. Kidneys were excised and fixed in 10% formaldehyde solution, rehydrated in decreasing grades of ethanol and embedded in paraffin wax. These samples (3–5 µm thick) were sectioned with a micro rotary microtome (Robert-Bosch-Straβe, Walldorf, Baden-Württemberg, Germany). The sections were then cleared in xylene and rehydrated in decreasing concentrations of ethanol. Subsequently, the sections were stained with hematoxylin and eosin (H and E), washed and dehydrated in increasing grades of ethanol and cleared in xylene. The processed sections were viewed and captured using a Leica Scanner, SCN400 and Slide Path Gateway LAN software for analysis (Leica Microsystems CMS, Wetzlar, Germany).
SGLT1 and GLUT1
Kidney tissues (0.1 g) were homogenized on ice in isolation buffer (0.5 mm Na2EDTA, 0.1 M KH2PO4, 0.1 mmol dithiothreitol, 0.25 M sucrose) and then centrifuged at 400 × g for 10 min (4 °C) for SGLT1 and GLUT1 analyses. The protein content for all samples was standardized to one concentration (1 mg/mL) was quantified using the Lowry method.Citation20 The proteins were then denatured by boiling in Laemmli sample buffer (0.5 M Tris-HCl, glycerol, 10% sodium dodecyl sulfate (SDS), 2-mercaptoethanol, 1% bromophenol blue) for 5 min. The denatured proteins were loaded (25 µL) on prepared resolving (10%) and stacking (4%) polyacrylamide gels along with molecular weight marker (5 µL). The gel was electrophoresed for 1 h at 150 V in electrode (running) buffer (Trisbase, glycine, SDS, pH 8.3). Following electrophoresis, the resolved proteins were electro-transferred to an equilibrated polyvinylidene difluoride (PVDF) membrane for 1 h in transfer buffer (192 mm glycine, 25 mm tris, 10% methanol). After transfer, the membrane was blocked with 5% non-fat dry milk in Tris-buffered saline with 0.1% Tween 20 (TTBS) (20 mm tris, 150 mm NaCl, KCl, 0.05% Tween-20). The membrane was then immune-probed with antibodies—SGLT1 and GLUT1 (catalogue numbers ab99447, ab15309, respectively, Abcam, Cambridge, United Kingdom, 1:1000 in 1% BSA, Neogen, USA) for 1 h at room temperature. The PVDF membrane was then subjected to five washes (10 min each with gentle agitation) with TTBS. Following which, the membrane was incubated in horse radish peroxidase (HRP)-conjugated secondary antibody (rabbit anti-mouse 1:10,000; Bio-Rad) for 1 h at RT. After further washing, antigen–antibody complexes were detected by chemiluminescence using the Immune-star™ HRP substrate kit (Bio-Rad, Johannesburg, South Africa). Chemiluminescent signals were detected with the Chemi-doc XRS gel documentation system and analyzed using the quantity one software (Bio-Rad, Johannesburg, South Africa). Band intensity analysis was conducted on the resultant bands.
Statistical analysis
Data are presented as the means ± standard error of mean (SEM). Overall statistical comparisons between the control means and experimental groups were performed with GraphPad InStat Software (version 5.00, GraphPad Software, San Diego, California, USA), using one-way analysis of variance (ANOVA), followed by Tukey-Kramer multiple comparison test. A value of p < 0.05 was considered significant. The test which assumes equal variance for the three or more means is generally used to determine whether the means differ significantly in an analysis of variance.
Results
Insulin-loading efficiency
The loading efficiency of insulin in amidated pectin insulin-containing dermal patches ranged from 76% to 94% and patches used contained 1.20, 2.87 and 5.04 µg of insulin, respectively, which translated to a dosage of 3.99, 9.57 and 16.80 µg/kg, respectively.
Short-term effects of PI
Body weight, food and water intake
compares daily weight changes, 24 h food and water intake by control non-diabetic and STZ-diabetic rats and PI-treated STZ-diabetic rats over the 5-week experimental period. Untreated diabetic rats exhibited characteristic signs of diabetes such as severe wasting and increased intake of water. There was no difference in food intake as animals were given a standard amount of food (30 g/day). Treatment with PI hydrogel matrix patches with low doses of insulin (3.99 and 9.57 µg/kg) significantly reduced the weight loss and water intake from week 4 while the highest dose (16.80 µg/kg) and sc insulin (175 µg/kg, sc) effects were observed from week 1.
Table 1. Comparisons of the effects of insulin-containing dermal patches on body weight, food and water intake in STZ-induced diabetic rats with control non-diabetic animals and untreated diabetic rats.
Renal fluid and electrolyte handling
Untreated STZ-induced diabetic rats exhibited high volumes of weekly urine output and increased urinary K+ output throughout the 5-week study compared with non-diabetic control animals (). On the other hand, urinary Na+ output of diabetic rats was significantly reduced (). Application of insulin-containing dermal patches with high insulin doses (16.80 µg/kg) significantly increased urinary Na+ outputs of diabetic rats from week 4. On the other hand PI insulin treatment reduced the volume of urine voided and urinary K+ output of STZ-diabetic rats, but higher than in control non-diabetic animals by the end of the 5th week. The PI-induced loss of Na+ was not reflected in the plasma by the end of the experimental period although plasma K+ concentration was reduced by comparison with untreated diabetic rats (). Urinary creatinine and urea outputs of diabetic rats were significantly (p < 0.05) reduced by comparison with non-diabetic control rats (data not shown). However, plasma creatinine concentrations were significantly (p < 0.05) elevated in control STZ-induced diabetic rats by comparison with untreated non-diabetic rats at the end of the 5-week experimental period (). The PI hydrogel matrix patch with highest insulin (16.80 µg/kg) significantly (p < 0.05) reduced plasma creatinine concentration in STZ-induced diabetic rats concomitant increase in GFR (). The plasma urea concentration after five weeks with the highest dose patch was 82% of that in untreated STZ-induced diabetic rats (p < 0.05). The transdermal PI hydrogel matrix patches (3.99, 9.57 and 16.80 µg/kg) decreased the MAP by the end of the 5-week experimental period ().
Table 2. Comparison of the effects of insulin-containing dermal patches on renal function parameters in STZ-induced diabetic rats with control non-diabetic animals and untreated diabetic rats.
Table 3. Comparisons of the effects on terminal plasma biochemical parameters of insulin-containing dermal patches in STZ-induced diabetic rats with control non-diabetic and untreated diabetic rats.
Terminal hormone measurements
Plasma insulin and AVP concentrations of untreated STZ-induced diabetic rats were significantly low (p < 0.05) at end of the 5-week experimental period by comparison with control non-diabetic animals (). Treatment with insulin-containing dermal patches at various doses over a 5-week period significantly (p < 0.05) elevated plasma insulin concentrations of STZ-induced rats in a dose-independent manner. The application of insulin-containing dermal patch (16.80 µg/kg) as well as insulin (175 µg/kg, sc) restored the concentration of AVP to levels comparable to values of non-diabetic control animals ().
Effects of PI hydrogel patch on urinary glucose
Transdermal application of PI matrix patch at a dose of 16.80 µg/kg for five weeks significantly (p < 0.05) decreased blood glucose and urinary glucose output of STZ-induced diabetic rats (, ). To evaluate whether reduction in urinary glucose output was mediated via modulation of insulin-responsive facilitative glucose transporters, the effects of transdermal insulin on glucose transporters expression, renal GLUT1 and SGLT1 were analyzed for using western blotting in kidney tissues harvested from STZ-induced diabetic rats at the end of 5-week study. The expression of GLUT1 and SGLT1 was significantly increased in STZ-induced diabetic animals in comparison to non-diabetic controls (). Similar to sc insulin, the insulin-containing dermal patch normalized GLUT1 and SGLT1 expression. Kidney mass was not altered by transdermal insulin treatment ().
Figure 1. Comparison of mean weekly urinary glucose outputs of STZ-induced diabetic rats treated with sc insulin and PI hydrogel matrix patch (16.80 µg/kg) thrice daily at 09 h00 followed by the same dose at 17 h00 and the 8 h later (01h00) for five weeks with control non-diabetic rats and untreated STZ-induced diabetic rats. Notes: *Denotes p < 0.05 by comparison with non-diabetic control animals. #Denotes p < 0.05 by comparison with untreated STZ-induced diabetic animals.
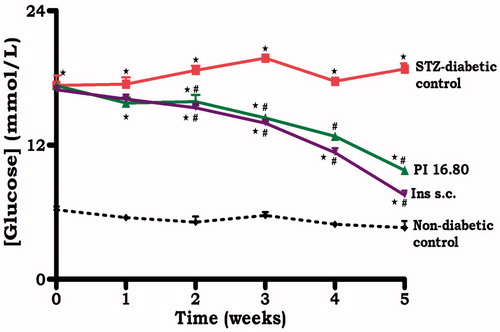
Figure 2. Comparison of the effects of topically applied PI hydrogel matrix patch and sc insulin on GLUT1 and SGLT1 in kidney tissues of STZ-induced diabetic rats, respectively, with untreated non-diabetic as determined by western blotting. Values are expressed as mean ± SEM. Values were obtained from western blots for six preparations. Notes: *Denotes p < 0.05 by comparison with non-diabetic animals. ♦Denotes p < 0.05 by comparison with respective control animals.
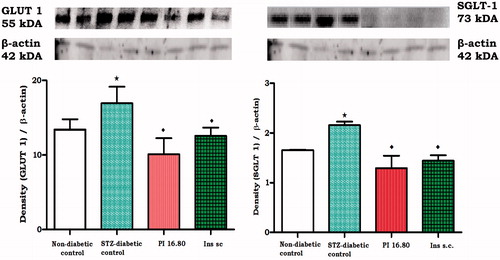
PI effects on kidney histology
Untreated non-diabetic rat kidney sections () exhibited a morphologically normal glomerulus with normal basement membrane, capillaries and proximal convoluted tubule cellularity (). In contrast to the non-diabetic control rats, STZ-induced diabetic rat sections revealed a thickened basement membrane of both the glomerulus and the Bowman’s capsule. In addition, the proximal convoluted tubule hypercellularity was observed following five weeks of study (). Treatment with transdermal insulin and sc insulin for five weeks resulted in attenuation of these anomalies when compared with untreated STZ-diabetic rats (). shows the normal glomerulus of the untreated non-diabetic rat kidney section showing normal glomerular basement membrane (GBM), capillaries (C), nuclei (N), proximal convoluted tubule (PCT), mesangium (M), veins (V) and squamous cells (S). Compared with the untreated non-diabetic control rats (), STZ-induced diabetic rats () showed thickened basement membrane of the Bowmans capsule (TBMBC), thickened glomerular basement membrane (TGBM) and hypercellularity of the proximal tubules (HCPT) after five weeks of the study. Treatment with insulin-containing dermal patches and sc insulin for five weeks however, attenuated these features when compared with the untreated STZ-diabetic rats ().
Figure 3. Photomicrographs (H and E) illustrating the effects of transdermally delivered insulin on the morphology of the kidney in STZ-induced diabetic rats. Photomicrograph (A) represents the normal glomerulus of the untreated non-diabetic rat kidney section showing normal glomerular basement membrane (GMB), glomerular capillaries (C), nuclei (N), proximal convoluted tubule (PCT), mesangium (M), veins (V) and squamous cells (S). Photomicrograph (B) represents the injured glomerulus of the STZ-diabetic rat showing irregular glomerular capillaries, thickened glomerular basement membrane (TGBM), thickened basement membrane of the Bowmans capsule (TBMBC), hypercellularity of the proximal tubules (HPT) and mesangial proliferation (MP). Photomicrograph (C) represents the glomerulus of the transdermal insulin treated rat kidney section showing irregular glomerular capillaries but no basement membrane thickening and mesangial proliferation. Photomicrograph (D) represents the glomerulus of the subcutaneous insulin treated rat kidney section showing irregular glomerular capillaries but no basement membrane thickening (Mag 35 × 100 μm).
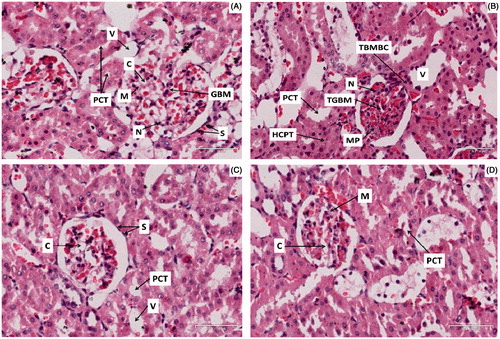
Discussion
The data presented herein show that transdermal application of PI matrix patches ameliorates kidney function of STZ-induced diabetic rats. The findings are clinically relevant considering that the development and progression to ESRD, the principal cause of death as a result of diabetic nephropathy, is associated with a progressive decline in renal function. Several studies have reported compromised renal function in experimental diabetesCitation10,Citation11,Citation21 and diabetic patients.Citation14,Citation22–25 The current therapeutic approach to diabetes using hypoglycemic agents has limited efficacy in averting the development of renal complications. Therefore, the findings are of considerable importance because they indicate that insulin-containing dermal patches may not only eliminate the frequent dosing administration associated with bolus injections, but also improve kidney function.
The effects of insulin over time are dependent on their pharmacokinetic and the mode of administration. Application of insulin-containing dermal patches delivers sustained controlled insulin to probably achieve physiological insulin concentration in the portal vein. The controlled sustained insulin release may reduce systemic hyperinsulinemia and, in the long run, insulin resistance by reversing down regulation of insulin receptors. On the other hand, bolus iv, sc or im insulin injections elicit relatively high plasma levels of insulin which are exposed to peripheral organs and probably cause hyperinsulinemic edema and hypertension.Citation8 The invasive PI dermal patches may offer minimally invasive insulin delivery in clinical applications to perhaps improve insulin bioavailability and patient compliance.
STZ-induced diabetic rats exhibited marked weekly decreases in urinary Na+ excretion and elevated plasma creatinine concentration at the end of five weeks with concomitant reduction in GFR. We suggest that renal insufficiency in diabetic animals was not due to the STZ nephrotoxicity since the dose of STZ (60 mg/kg) used to induce diabetes has been reported to have minimal kidney toxicity in experimental animals.Citation26–28 Na+ retention can also be attributed to diabetes-induced histopathological changes in the kidney particularly thickening of the glomerular basement membrane (Photomicrograph B) which decreases the filtration surface area. These changes are attributed in part to decreased insulin secretion (both type 1 and type 2 diabetes mellitus) or insulin resistance (type 2 diabetes mellitus).Citation29
The kidney of STZ-induced diabetic rats showed structural changes (Photomicrograph C) which was prevented by dermal PI matrix patches treatment indicating protection of diabetes induced kidney damage. Treatment with insulin-containing dermal patches probably reduced renal damage to offset the renal effects associated hyperglycemia by mechanisms through a number of metabolic pathways. Insulin-containing dermal patches increased urinary Na+ excretion of STZ-induced diabetic rats in contrast to hyperinsulinemic edema and hypertension due to increased Na+ retention associated with intensive insulin therapy.Citation8 Our study shows that transdermal PI matrix patches can reverse the previously reported inability of the kidney to excrete Na+ in STZ-diabetes mellitus.Citation30–32 Hyperinsulinemic edema and hypertension associated with intensive insulin therapy have been attributed, at least in part, to Na+retaining action of insulin.Citation8 However, literature evidence on the effects of insulin on kidney function in diabetes is conflicting and remains unclear. It would appear that renal effects of insulin depend on endogenous levels of circulating insulin and on the availability of insulin-binding sites in the kidney.Citation33 Indeed, physiological concentrations of insulin decrease renal Na+ re-absorption and prevent the rise in plasma K+ concentrations in diabeticsCitation34,Citation35 while high doses increase plasma Na+ concentration and cause hypokalemia.Citation36 The results suggest that the pectin hydrogel dermal insulin matrix patches delivered physiologically relevant amounts of pharmacologically active insulin. Indeed, the glycemic control and amelioration of kidney function of STZ-induced rats observed in the present study were achieved with PI hydrogel matrix patches that elicited plasma insulin concentrations within the physiological range. The highest dose of PI hydrogel matrix patch (16.80 µg/kg) increased plasma insulin concentrations to levels comparable with non-diabetic animals and was effective in ameliorating renal fluid and electrolyte handling and expression of glucose transporters as well as reducing the mean arterial blood pressure of STZ-induced diabetic rats. Studies indicate that hyperglycemia is the main cause of the decline in kidney function in diabetic patients.Citation24,Citation25 The changes attenuated by transdermal insulin treatment indicate beneficial effects on renal function in diabetes.
Glycosuria observed in untreated STZ-induced diabetic rats was ameliorated by transdermal insulin treatment as evidenced by the decrease of plasma glucose concentrations measured at the end of the experimental period. We have reported in another study that insulin-containing dermal patch formulation sustains slow controlled release of insulin into the bloodstream of diabetic rats with a concomitant reduction in plasma glucose concentrations.Citation9,Citation37 As assessed by western blotting, PI treatment significantly decreased the renal expression of SGLT1 and GLUT1 to perhaps to reduce glucose re-absorption. These transporters which were unregulated in untreated STZ-induced diabetic rats increased the re-absorption of glucose and thus contributed to the pre-existing hyperglycemia.Citation16,Citation17 Taken together, the studies suggest that insulin-containing dermal patches increase urinary Na+ excretion and decrease renal expression of glucose transporters of STZ-induced diabetic rats.
In summary, the current data suggest that topically administered insulin not only improves glycemic control and kidney function of STZ-induced diabetic rats, but also ameliorates the expression of renal insulin-stimulated facilitative glucose transporters. Therefore, this method of insulin delivery system may gradually progress towards physiological insulin replacement and reduce long-term complications of diabetes mellitus. The major limitations of the current study include lack of measurements of a wide range of humoral factors known to influence kidney function such as aldosterone, atrial natriuretic peptide and angiotensin II which would have enabled us to assess their involvement during the course of the treatment.
Declaration of interest
The authors declare that there is no interest that could be perceived as prejudicing the impartiality of the research reported.
The authors are grateful to the Biomedical Resource Unit, University of KwaZulu-Natal, for the supply of animals.
This study was partly funded by the NRF South Africa and the University of KwaZulu-Natal, Research Division.
References
- Group UKPDS, UK. Prospective diabetes study 16: Overview of 6 years' therapy of type II diabetes: A progressive disease. Diabetes. 1995;44:1249–1258
- Ceriello A. New insights on oxidative stress and diabetic complications may lead to a “causal” antioxidant therapy. Diabetes Care. 2003;26:1589–1596
- Brownlee M. Biochemistry and molecular cell biology of diabetic complications. Nature. 2001;414:813–820
- Sheetz MJ, King GL. Molecular understanding of hyperglycemia's adverse effects for diabetic complications. JAMA. 2002;288:2579–2588
- Rabkin R. Diabetic nephropathy. Clin Cornerstone. 2003;5:1–11
- The Diabetes Control and Complications Trial Research Group. The effect of intensive treatment of diabetes on the development and progression of long-term complications in insulin-dependent diabetes mellitus. The Diabetes Control and Complications Trial Research Group. N Engl J Med. 1993;329:977–986
- Ohkubo YA, Kishikawa H, Araki E, et al. Intensive insulin therapy prevents the progression of diabetic microvascular complications in Japanese patients with non-insulin-dependent diabetes mellitus: A randomized prospective 6-year study. Diabetes Res Clin Pract. 1995;28:103–117
- Bas VN, Setinkaya S, Agladioglu SY, et al. Insulin oedema in newly diagnosed type 1 diabetes mellitus. J Clin Res Pediatr Endocrinol. 2010;2:46–48
- Tufts M, Musabayane CT. Transdermal delivery of insulin using amidated pectin hydrogel patches. Society for Endocrinology BES. 2010;21:173
- Musabayane CT, Ndhlovu CE, Balment RJ. Renal fluid and electrolyte handling in streptozotocin induced diabetic rats. Ren Fail. 1995;17:107–116
- Li Y, Higashiura K, Ura N, et al. Effects of the Chinese medicine, TSJN on insulin resistance and hypertension in fructose-fed rats, hypertension research. J JPN Soc Hypertens. 2000;23:101–107
- Haller H, Drab M, Luft FC. The role of hyperglycemia and hyperinsulinemia in the pathogenesis of diabetic angiopathy. J Clin Nephrol. 1996;46:246–255
- Krishnan R, Izatt S, Bargman JM, Oreopoulos D. Prevalence and determinants of erectile dysfunction in patients on peritoneal dialysis. Int Urol Nephrol. 2003;35:553–556
- Stengel B, Billion S, Van Dijk PC, et al. Trends in the incidence of renal replacement therapy for end-stage renal disease in Europe. Nephrol Dial Transplant. 2003;18:1824–1833
- Abdul-Ghani MA, Defronzo RA. Lowering plasma glucose concentration by inhibiting renal sodium–glucose co-transport. J Int Med. 2014;276:352–363
- Vestri S, Okamoto MM, Freitas HSD, et al. Changes in sodium or glucose filtration rate modulate expression of glucose transporters in renal proximal tubular cells of rat. J Membr Biol. 2001;182:105–112
- Marks J, Carvou NJ, Debnam ES, Srai SK, Unwin RJ. Diabetes increases facilitative glucose uptake and GLUT2 expression at the rat proximal tubule brush border membrane. J Physiol. 2003;553:137–145
- Dominguez JH, Camp K, Maianu L, Feister HW, Garvey TW. Molecular adaptations of GLUT1 and GLUT2 in renal proximal tubules of diabetic rats. Am J Physiol. 1994;266:F238–F290
- Musabayane CT, Munjeri O, Matavire TP. Transdermal delivery of chloroquine by amidated pectin hydrogel matrix patch in the rat. Ren Fail. 2003;25:525–534
- Lowry OH, Rosebrough NJ, Farr AL, Randall RJ. Protein measurement with the Folin phenol reagent. J Biochem. 1951;193:265–275
- Kumar A, RIlavarasan R, Jayachandran T, et al. Anti-diabetic activity of Syzygium cumini and its isolated compound against streptozotocin-induced diabetic rats. J Med Plants Res. 2008;2:246–249
- Krishnan JA, Parce PB, Martinez M, Dietz GB, Brower RG. Caloric intake in medical ICU patients: Consistency of care with guidelines and relationship to clinical outcomes. Chest. 2003;124:297–305
- Remuzzi G, Macia M, Ruggenenti P. Prevention and treatment of diabetic renal disease in type 2 diabetes: The BENEDICT study. J Am Soc Nephrol. 2006;17:S90–S97
- Gnudi L, Thomas SM, Viberti G. Mechanical forces in diabetic kidney disease: A trigger for impaired glucose metabolism. J Am Soc Nephrol. 2007;18:2226–2232
- Bloomgarden ZT. American college of endocrinology pre-diabetes consensus conference: Part three. Diabetes Care. 2008;31:2404–2409
- Kuramochi G, Homma S. Effects of furosemide on renal oxygen consumption after ischemia in normal and streptozotocin diabetic rats. Nephron Physiol. 1993;64:436–442
- Lenzen S. The mechanisms of alloxan- and streptozotocin-induced diabetes. Diabetologia. 2008;51:216–226
- Koulmanda M, Qipo A, Chebrolu S, et al. The effect of low versus high dose of streptozotocin in cynomolgus monkeys (Macaca fascilularis). Am J Transplant. 2003;3:267–272
- Welsh GI, Hale LJ, Eremina V, et al. Insulin signaling to the glomerular podocyte is critical for normal kidney function. Cell Metab. 2010;12:329–340
- Musabayane CT, Ndhlovu CE, Balment RJ. Renal fluid and electrolyte handling in streptozotocin induced diabetic rats. J Ren Fail. 1995;17:107–116
- Musabayane CT, Tufts MA, Mapanga RF. Synergistic antihyperglycemic effects between plant-derived oleanolic acid and insulin in streptozotocin-induced diabetic rats. J Ren Fail. 2010;32:832–839
- Mkhwanazi BN, Serumula MR, Myburg RB, Heerden FRV, Musabayane CT. Antioxidant effects of maslinic acid in livers, hearts and kidneys of streptozotocin-induced diabetic rats: Effects on kidney function. Ren Fail. 2014;36:419–431
- Rostand SG, Watkins JB, Clements RS. The effect of insulin and of anti-insulin serum on handling of sodium by the isolated, perfused kidney of the streptozotocin-diabetic rat. Diabetes. 1980;29:679–685
- Allon M, Copknky C. Albuterol and insulin for treatment of hyperkalemia in hemodialysis patients. Kidney Int. 1990;38:869–872
- Allon M. Treatment and prevention of hyperkalemia in end-stage renal disease. Kidney Int. 1993;43:1197–1209
- Herlitz H, Widgren B, Urbanavicius V, Attvall S, Persson B. Stimulatory effect of insulin on tubular sodium reabsorption in normotensive subjects with a positive family history of hypertension. Nephrol Dial Transplant. 1996;11:47–54
- Hadebe SI, Ngubane PS, Serumula MR, Musabayane CT. Transdermal delivery of insulin by amidated pectin hydrogel matrix patch in streptozotocin-induced diabetic rats: Effects on some selected metabolic parameters. PLoS One. 2014;9:e101461