Abstract
Purpose: Oxidative renal injury is the mainstay in patients with spinal cord injury (SCI) and it may eventuate to chronic renal failure. In our study, we investigated the potential of α2-adrenoreceptor agonist Dexmedetomidine (Dex) in ameliorating SCI provoked oxidative renal assault. Methods: Complete SCI was generated by surgical transaction of the cord at the T10–12 level. Dex administration (50 mcg/kg, b.wt, intraperitoneally) was initiated 12 h after the surgery for 10 days. Then, blood was collected and kidneys were removed to evaluate the efficacy of Dex on post-SCI renal complications. Results: Dex treatment significantly attenuated elevated serum creatinine and blood urea nitrogen in SCI rats to normalcy. Further in SCI rats elevated level of MDA, protein carbonyl and myeloperoxidase (MPO) were observed and Dex treatment significantly attenuated these toxic manifestations to normalcy. Besides in SCI rats, the antioxidants (SOD, CAT, Gpx and GST and GSH) levels were significantly diminished and Dex treated rats significantly restored the antioxidants level in renal tissue to normalcy. Notably, in our study the protein expression of inflammatory cytokines (TNF-α and IL-6) and cleaved caspase-3 were upregulated in renal tissue of SCI rats. Fascinatingly, Dex treatment downregulated the protein expression of TNF-α, IL-6 and cleaved caspase-3 by anti-inflammatory, antiapoptotic mechanism. Furthermore, SCI rats displayed upregulated protein expression of kidney of SCI rats. Dex treatment diminished the renal apoptosis by downregulating the cleaved caspase-3 expression. Conclusion: Taken together, these results accentuate that Dex may be a beneficial clinical agent to combat post-SCI renal complications.
Introduction
Globally, spinal cord injury (SCI) is an austere traumatic healthcare condition resulting from wide array of etiological factors such as traffic collisions. Clinically, SCI is bifurcated into a two staged process: one, the acute phase due to tissue compression and hemorrhage to a specific region of the spinal cord and the secondary injury phase encompassing a series of noxious pathological events, which culminate to life threatening conditions.Citation1 Assortment of research reports indicate that apoptosis, elevated amino acids level and exaggerated accumulation of free radicals with concurrent lipid peroxidation (LPO) orchestrate a predominant role in SCI provoked secondary damage/complications.Citation2–5 Furthermore, spinal cord collapse leads to the stimulation of inflammatory cells within the circulation, this systemic response may also impair the organs like liver, lung and kidney. In SCI patients, organ failure proffers significant morbidity and mortality and the lung, kidney are cardinal cellular structures vulnerable to SCI-mediated inflammatory response.Citation6–9 However, the kidney is highly prone to free radical encounter due to high oxygen utilization (10% of total oxygen consumption by the body) than the other vital tissues. Hence, rampant production of reactive oxygen species (ROS) occurs prominently in the kidney.Citation10 Renal failure in people with SCI eventually leads to a stage where hemodialysis is needed and is a major cause of death. When it comes to renal failure, the damage is irreversible, however, the protection of the kidneys may prevent or retard their deterioration.
Dexmedetomidine (Dex) is an effective lipophilic α-2 adrenoceptor agonist with an imidazole structure.Citation11 It exerts wide array of biological effects like sedative, analgesic, hemodynamic stabilizing, anti-inflammatory and diuretic effects.Citation12 A wealth of work has shown that Dex has tissue protective effects, mitigating cerebral, cardiac, intestinal and renal injury which can be abolished by atipamezole, an α2-adrenoreceptor antagonist.Citation13–18 Multiple studies indicate the protective of Dex in spinal cord-mediated injury and its complications.Citation19–21 In this backdrop, the present study was designed to determine whether spinal cord trauma elicits oxidative renal injury and Dex could attenuate these toxic manifestations. As far as we are aware, this is the first study to report the efficacy of Dex in ameliorating SCI provoked renal complications.
Materials and methods
Chemicals
Dex and commercial diagnostic kits were obtained from Sigma Chemical Co. (St. Louis, MO). All other chemicals were of the highest available commercial grade.
Animals
Sprague-Dawley rats weighing 170–200 g were obtained from our animal facility Qingdao University Medical College, China. The animals were maintained under standard laboratory conditions of relative humidity (55 ± 5%), temperature (25 ± 2 °C), and light (12 h light/12 h dark). They were fed standard diet pellets and water was provided ad libitum.
Animal model of SCI
The rats were anesthetized by administration of xylazine + ketamine (10 + 75 mg/kg, b.wt; i.p.). Then, the dorsum of the animals was shaved and sterilized an incision was performed from posterior to the lower thoracic region. Laminectomy (thoracic T10–T12 vertebrae) was performed to expose the spinal cord alone (sham group). After the laminectomy, lower thoracic cord was subsequently completely transected with fine sterilized microscissors (SCI group) under the magnifier. Both stumps of the spinal cord were gently lifted away to create a 1–2 mm gap, which was filled with sponge gel. The muscle fascia and skin were sutured, and the rats were returned to their home cages. After completion of surgery, the animals received a bolus of Lactate Ringers solution (5 mL, i.p.) to compensate for blood loss, and antibiotic cover (systemic, gentamicin 50 mg/kg b.wt; weight, i.m.; local Neosporin ointment) was provided. Furthermore, the SCI rats received daily assistance in bladder emptying until spontaneous miction recovered.
Evaluation of locomotor functions
The quality of locomotion was assessed by Basso, Beattie and Bresnahan locomotor rating score.Citation22
Study protocol
Sprague-Dawley rats were divided into four groups (n = 10) as follows:
Group I – sham-operated rats (Sham).
Group II – SCI rats (SCI).
Group III – sham-operated rats received Dex (50 mcg/kg, b.wt) intraperitoneally for 10 days (Sham + Dex).
Group IV – SCI rats received Dex (50 mcg/kg, b.wt) intraperitoneally for 10 days (SCI + Dex).
The kidney homogenate, 10% (w/v), was prepared in ice-cold 0.1 M Tris–HCl buffer, pH 7.5 using a Branson sonifier (250, VWR Scientific, Radnor, PA) at 4 °C followed by centrifugation at 10,000 rpm for 10 min. The supernatant was kept at −20 °C till further analysis.
Biochemical estimation
Assessment of serum renal markers
The serum creatinine was measured colorimetrically using a diagnostic kit that based on the reaction with picric acid in alkaline media. The serum urea was measured colorimetrically using a diagnostic kit that based on the reaction with the diacetylmonoxime reagent. The units were expressed as mg/dL.
Estimation of enzymic antioxidants
The kidney superoxide dismutase (SOD) was estimated by the method of Misra and Fridovich.Citation23 The ability of SOD to inhibit the auto-oxidation of epinephrine to adrenochrome at pH 10.2 has been used as the basis for the assay. One unit of SOD corresponds to the 50% inhibition of epinephrine to adrenochrome transition by the enzyme. The catalase (CAT) activity in the kidney homogenate was analyzed by the method of Sinha,Citation24 wherein the breakdown of H2O2 is measured at 240 nm. The CAT activity was expressed as nmol H2O2 consumed/min/mg of protein. The renal glutathione peroxidase (GPx) level was assayed by the method of Rotruck et al.Citation25 The assay system was based on the reaction between a measured amount of enzyme preparation H2O2 and reduced glutathione (GSH) for a specified time period. Then, the unreacted GSH was measured by reaction with 5,5′-dithiobis(2-nitrobenzoic acid (DTNB). The GPx activity was expressed as nmol of GSH oxidized/min/mg of protein. Glutathione-S-transferase (GST) was estimated by the protocol of Misra et al.Citation23 The increase in absorbance was measured at 340 nm using 1-chloro-2,4-dinitrochlorobenzene (CDNB) as substrate. The enzyme unit of GST was expressed nmol of GSH–CDNB conjugate formed/min/mg of protein. Protein content in the kidney homogenate was estimatedCitation26 using bovine serum albumin as a standard.
Measurement of non-enzymic antioxidants
The renal reduced GSHCitation27 levels were determined by the method of Moron et al.Citation27 The non-protein sulfhydryl content of cells is in the form of reduced GSH. DTNB is a disulfide compound that is readily reduced by sulfhydryl compounds forming a highly colored yellow anion. The optical density of this yellow substance is measured at 412 nm.
Measurement of LPO and protein carbonyl content
The malondialdehyde (MDA) an index of LPO level in the kidney homogenate was measuredCitation28 according to the method of Ohkawa et al.Citation28 The final colored end product was assayed spectrophotometrically at 532 nm. The MDA level was expressed as the nmol/g of tissue.
The protein carbonyl content in the kidney homogenate was determined as previously described, by the reaction with DNPH and HCl and finally with guanidine hydrochloride.Citation29
Assay of MPO
Tissue MPO is employed to assess the neutrophil infiltration in inflamed tissues. Briefly, 100 mg of left kidney tissue was homogenized in ice-cold potassium phosphate buffer (50 mM – K2HPO4, pH 6) containing hexadecyltrimethylammonium bromide (0.5%, w/v) to get 10% homogenate. The homogenate was allowed for three freeze and thaw cycles and then centrifuged at 41,400 g for 10 min at 4 °C. MPO activity was estimated by measuring the H2O2-dependent oxidation of o-dianisidine at 460 nm. The enzyme activity was expressed as U/g of tissue.
Assay of pro-inflammatory cytokines
Concentrations of IL-6, and TNF-α were determined in serum using commercially available ELISA kits (USCN LIFE, Wuhan EIAab Science Co., Ltd., Wuhan, China).
Western blot analyses
Frozen renal tissues from different treatment groups were homogenized in ice-cold RIPA buffer (Pierce Biotechnology, Rockford, IL) containing 1% protease inhibitor cocktail (Sigma-Aldrich, St. Louis, MO) to get total protein extracts. The contents were centrifuged at 12,000 g for 20 min at 4 °C, the supernatant was collected and used for analysis of cleaved caspase-3 expression. Similarly, nuclear extracts were prepared by using NEPER nuclear and cytoplasmic extraction kit (Pierce Biotechnology, Rockford, IL) containing 1% protease inhibitor cocktail (Sigma-Aldrich) according to the manufacturer's protocol and used for TNF-α and IL-6 protein expression. The protein contents were measured by using biscinchonic acid kit (Pierce Biotechnology) against bovine serum albumin (BSA) as standard.
Briefly, the samples (40 μg/lane) were loaded and separated by 10% sodium dodecyl sulfate–polyacrylamide gel electrophoresis (SDS–PAGE), and then transferred to polyvinylidene difluoride membranes. The membranes were blocked with 3% BSA for 1 h and incubated with primary antibodies overnight at 4 °C against cleaved caspase-3 (rabbit monoclonal 1:1000, Cell Signaling Technology, Danvers, MA), TNF-α (mouse monoclonal 1:1000, Cell Signaling Technology), IL-6 (rabbit monoclonal 1:1000, Cell Signaling Technology), followed by horseradish peroxidase-conjugated secondary antibodies (1:3000) for 1 h and visualization with the enhanced chemiluminescence system (Pierce Biotechnology). β-Actin was used as the loading control. Densitometric analysis of immunoblots was performed with the Image J software (NIH, Bethesda, MD).
Statistical analysis
The data were represented as the mean ± standard deviation (SD). To test for statistically significant differences among various groups for a given parameter, one way analysis of variance with Tukey’s post hoc test comparison procedure. All analyses were performed using the Statistical Package for the Social Sciences (SPSS) software (Version 13.0; SPSS, Inc., Chicago, IL). p-Value < 0.05 was considered statistically significant.
Results
The renal function markers parameters such as blood urea nitrogen (BUN), creatinine and kidney to body weight ratios were estimated in all the groups. SCI rats displayed significant (p < 0.05) increase in level of creatinine and BUN in serum as that of the sham-operated rats. Treatment with Dex significantly (p < 0.05) diminished the altered level to normalcy as that of the SCI rats ().
Figure 1. Effect of Dex on serum renal markers in SCI-induced renal injury. (A) BUN (mg/dL) and (B) creatinine (mg/dL). Values are mean ± SD for 10 rats in each group. Comparisons are made between: (a) sham and SCI; (b) SCI and SCI + Dex. *Statistically significant (p < 0.05).
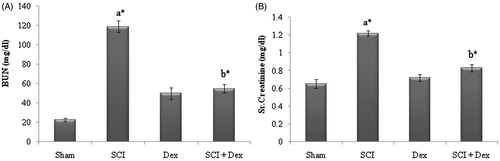
In the present study, MDA, a reliable indicator of LPO and protein carbonyl content, as an index of protein oxidation were significantly (p < 0.05) elevated in SCI rats. However, animals treated with Dex significantly (p < 0.05) abolished the elevated MDA and protein carbonyl content in renal tissues as that of the SCI induced rats ().
Figure 2. Effect of DEX on SCI-induced changes in LPO and protein oxidation markers in kidney tissues of rats. (A) MDA (nmol/g tissue) and (B) protein carbonyl content (nmol/mg protein). Values are mean ± SD for 10 rats in each group. Comparisons are made between: (a) sham and SCI; (b) SCI and SCI + Dex. *Statistically significant (p < 0.05).
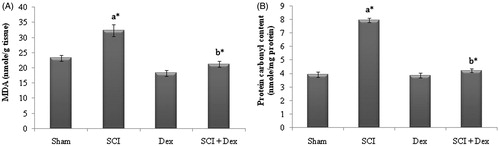
The experiment revealed that renal MPO levels were significantly (p < 0.05) higher in SCI group compared to sham. However, Dex treatment significantly (p < 0.05) diminished the MPO level to normal and thus confers protection to renal tissue ().
Figure 3. Effect of SCI and Dex on MPO level in kidney homogenate. The MPO was expressed as U/g of tissue. Values are mean ± SD for 10 rats in each group. Comparisons are made between (a) sham and SCI and (b) SCI and SCI + Dex. *Statistically significant (p < 0.05).
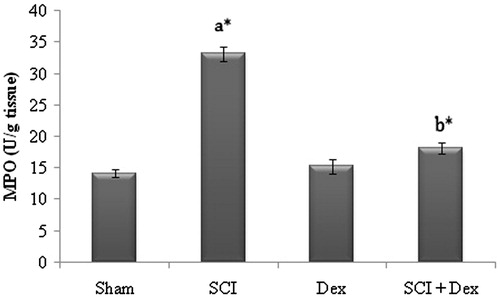
In our study, SCI rats displayed significant (p < 0.05) reduction in the level of non-enzymic antioxidant GSH in renal tissue as a result of excessive LPO when compared to the sham-operated rats, while treatment with Dex significantly (p < 0.05) elevated the GSH content in renal tissue as that of the SCI rats ().
Figure 4. Effect of SCI and Dex on non-enzymic antioxidant level in kidney tissue. The GSH level was expressed µg/mg of tissue. Values are mean ± SD for 10 rats in each group. Comparisons are made between (a) sham and SCI and (b) SCI and SCI + Dex. *Statistically significant (p < 0.05).
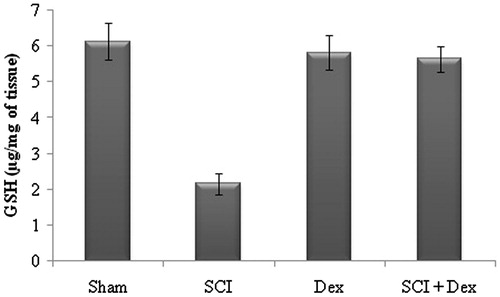
SCI insulted rats elicited significant (p < 0.05) decrease in the level of enzymic antioxidants SOD, CAT, GPx and GST in renal tissue when compared to the sham operated rats. However, administration of Dex significantly (p < 0.05) restored the diminished enzymic antioxidants level to normalcy ().
Table 1. Effect of SCI and Dex on renal enzymic antioxidant levels.
In the present study, serum inflammatory cytokines (TNF-α and IL-6) level were significantly (p < 0.05) elevated in SCI rats compared to sham. Treatment with Dex significantly (p < 0.05) diminished the elevated level of TNF-α and IL-6 to normal ().
Figure 5. Levels of inflammatory cytokines in serum. (A) TNF-α (pg/mL) and (B) IL-6 (pg/mL). Values are mean ± SD for 10 rats in each group. Comparisons are made between: (a) Sham and SCI and (b) SCI and SCI + Dex. *Statistically significant (p < 0.05).
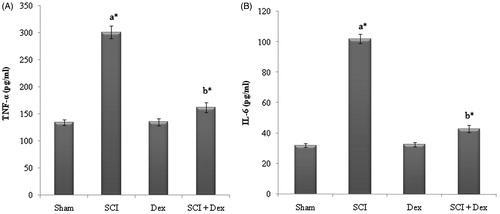
Furthermore, the expression levels of TNF-α and IL-6 were extensively studied in terms of their involvement in acute and chronic inflammations. As shown in , SCI rats displayed significant increase in the expression level of TNF-α and IL-6 in kidneys as compared with sham rats (p < 0.05). However, treatment with Dex significantly (p < 0.05) downregulated the TNF-α and IL-6 expression level to normal.
Figure 6. Western blot analysis of inflammatory cytokines in renal tissues. (A) TNF- α and IL-6 protein expression; (B) relative fold change of TNF-α and IL-6. β-Actin served as an internal control. Values are mean ± SD for five rats in each group. Comparisons are made between: (a) Sham and SCI and (b) SCI and SCI + Dex. *Statistically significant (p < 0.05).
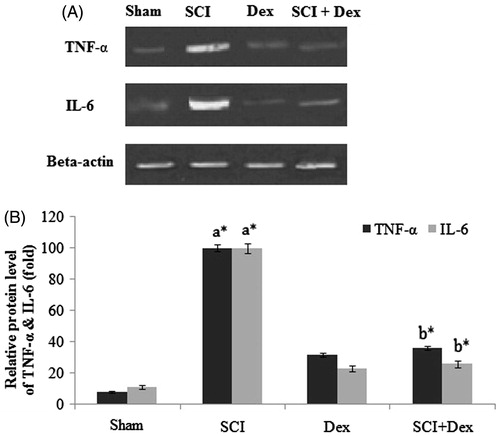
To further investigate the effect of renal cell apoptosis in SCI renal injury, western blot analysis was performed to determine the expression of cleaved caspase-3. In our study, SCI rats displayed significant (p < 0.05) upregulation of cleaved caspase-3 expression as that of sham-operated rats. However, Dex treatment significantly (p < 0.05) reduced the cleaved caspase-3 expression when compared to the SCI rats ().
Figure 7. Western blot analysis of apoptotic marker in renal tissues. (A) Cleaved caspase 3 protein and (B) relative fold change of cleaved caspase 3. β-Actin served as an internal control. Values are mean ± SD for five rats in each group. Comparisons are made between: (a) Sham and SCI and (b) SCI and SCI + Dex. *Statistically significant (p < 0.05).
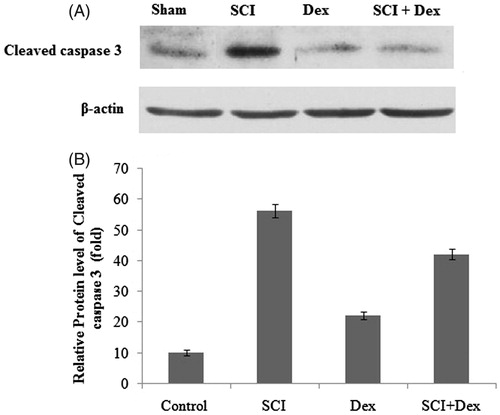
Discussion
SCI is an irreversible chronic pathological state bolsters systemic inflammation and elicits multiorgan failure and dysfunction apart from neurological insults. Mounting evidences corroborate that SCI displays significant structural, physiological and molecular alterations in the urinary bladder.Citation30,Citation31 In clinical utility, Dex has been portrayed as an effective, safe adjunct in many clinical applications. Previous studies documented the effect of Dex on renal system, which may boost urine output by revamping of cardiac output, blocking vasopressin release and augment the renal blood flow and glomerular filtration.Citation32–34 Furthermore, Dex proffers renoprotective to the ischemic kidney as a result of oxidative injury, which may overture to exaggerate acute kidney damage.
In the present study, SCI animals displayed a significant elevation of BUN and creatinine levels in serum which is an indicative of deteriorated renal function. However, treatment with Dex significantly diminished the elevated levels of BUN and creatinine and restored the renal function markers to normalcy. Post-SCI mediated ROS generation and free radical provoked oxidative organ insult is one of the vital injury secondary injury mechanisms in acute SCI.Citation35–37 Free radical-mediated LPO is the prime sequel in the destruction cellular membranes which are composed of polyunsaturated fatty acids (PUFA). During this process the PUFA are vulnerable to the attack of LPO which lead to structural changes in membrane fluidity, integrity and permeability. These toxic events prelude to enhanced protein degradation and finally cell destruction. Furthermore, research reports advocate that LPO is one of the cardinal event in post-SCI-mediated tissue destruction.Citation38 During oxidative assault, carbonylated protein serves as a reliable marker to assess irreversible oxidative damage disease-derived protein dysfunction.Citation39,Citation40 Post-SCI elevated levels of NO react with superoxide anion () to form highly potent oxidant peroxynitrite.Citation41,Citation42 Research reports indicate that peroxynitrite orchestrate wide array of noxious chemical reaction including protein carbonylation.Citation39,Citation40,Citation43 In our study, SCI rats elicited elevated MDA and protein carbonyl content in renal tissue and treatment with Dex significantly attenuated the extent of LPO and protein carbonylation. Previous studies explore the antilipid peroxidative effect of Dex and it may be mediated through free radical quenching mechanism.Citation44,Citation45 Furthermore, the inhibition of protein carbonylation in our study may be due to the NO scavenging activity of Dex which is in line with previous report.Citation46
Reduced GSH guards the cells against ROS and mounting evidences suggest that oxidative tissue injury provoked by noxious stimuli is coupled with GSH depletion.Citation47–49 Thus, GSH orchestrates a pivotal role in cell metabolism, differentiation, proliferation and apoptosis.Citation50 In this scenario, strategies targeting augmentation of GSH level may be implicated in the maintenance of cellular integrity. The present findings demonstrated that SCI rats displayed diminished GSH content in renal tissue and treatment with Dex boosted the level of GSH and this effect may be due to the indirect antioxidant activity by blocking the activity of inflammatory enzymes or by upholding the synthesis of GSH. Furthermore, GSH modulation effects of DEX may be due to the inhibition of NO production and downregulation of mRNA and protein inducible nitric oxide synthase (iNOS) expression.Citation51
Cells are integrated with exclusive assortment of antioxidant defense system to encounter the free radicals. The present communication shows that the SCI-mediated elevated LPO is accompanied by concomitant decline in the activities of cellular enzymic antioxidants SOD, CAT, GPX and GST. The diminished levels of antioxidant enzymes are mainly attributed due to the interaction of ROS produced during the spinal cord trauma with sulfhydryl group of enzymes. In our study, SCI rats displayed significant reduction of antioxidant enzymes in renal tissue and Dex administration elicited effective protection against SCI induced changes in antioxidant enzyme levels by restoring them to normal. Preclinical studies indicate that Dex ameliorates oxidative injury in wide array of tissue encompassing brain, kidney and heart.Citation13,Citation17,Citation18
MPO orchestrates a central role in neutrophil-mediated free radical production and serves as a reliable marker for neutrophil infiltration in organs in the event oxidative injury. In the current study, elevated level of MPO in SCI animals clearly exemplifies that SCI provoked renal injury may be neutrophil-dependent. ROS stimulated neutrophils release MPO, which in turn releases noxious HOCl and oxidizes proteins, lipids, carbohydrates and nucleic acids. A growing body of research studies indicates that neutrophils release chemotactic molecules and aid the process of neutrophil migration to organs where it activates the neutrophil and further enhances oxidative damage.Citation52 In our study, treatment with Dex significantly attenuated the elevated MPO level via anti-inflammatory mechanism which is line with previous report.Citation53
Cytokines play a crucial role in SCI-mediated renal injury and estimation of its level is of highly significant to assess the severity of oxidative stress. Elevated levels of TNF-α and IL-6 have been proven to orchestrate cellular imbalance caused by oxidative, nitrosative and apoptotic mediator.Citation54 Furthermore, SCI trauma triggers local inflammatory response which is attributed due to infiltration of inflammatory cells, release of inflammatory mediators and stimulation of endothelial cells leading to vascular permeability, edema, necrosis and tissue destruction at the site of trauma.Citation55 Interleukins, a key cytokine molecule, play a vital role in inflammatory network to elicit necrosis, apoptosis and leukocyte infiltration. Downregulation of cytokines has been reported to accelerate renoprotective effects. In the present investigation, SCI rats displayed elevated level of TNF-α and IL-6 in serum and further upregulated the expression of TNF-α and IL-6 in renal tissue. Treatment with Dex significantly reduced the serum level of TNF-α and IL-6 and downregulated protein expression of TNF-α and IL-6. In our present study, the anticytokine potential of Dex remains unclear; however, mounting evidences displayed the role of Dex and α2 adrenergic receptor agonists on cytokines.Citation56–59
Apoptosis in renal tissues have been endorsed as one of the major mechanisms SCI induced renal damage. Among the apoptotic mediators, activated caspase-3 acts as a final executor, as it regulates the extrinsic and intrinsic pathways of apoptosis. Furthermore, caspase-3-mediated cell apoptosis has been demonstrated to be essential in SCI-induced organ damage.Citation60–62 The present finding displayed that administration of Dex notably inhibited the protein level of cleaved caspase-3, (the activated form of caspase-3) within the renal tissues. Moreover, the activity of caspase-3 was downregulated as a result of Dex administration. Therefore, it is hypothesized that Dex may protect renal cells against SCI provoked apoptosis by suppressing caspase-3. Assorment of research reports authenticate that Dex potentially minifies the brain, intestine, heart, testis, neutrophils and kidney in the event of oxidative insult.Citation13–18
In conclusion, the findings of the current study substantiate that renoprotective effect of Dex in post-SCI rats might be due to the antioxidant, anti-inflammatory and antiapoptotic mechanism, suggesting an additional agent to be further studied for its consideration to be included in the combined therapeutic interventions for limiting SCI-induced secondary tissue degeneration.
However, further molecular studies are highly warranted to explore the renoprotective effect of DEX and to establish its feasible use in clinical setup as an adjunct candidate to spinal cord trauma.
Declaration of interest
The authors report no conflicts of interest. The authors alone are responsible for the content and writing of the paper.
References
- Beattie MS. Inflammation and apoptosis: Linked therapeutic targets in spinal cord injury. Trends Mol Med. 2004;10:580–583
- Balentine JD. Pathology of experimental spinal cord trauma. The necrotic lesion as a function of vascular injury. Lab Invest. 1978;39:236–253
- Wrathall JR, Teng YD, Choiniere D. Amelioration of functional deficits from spinal cord trauma with systemically administered NBQX, an antagonist of non-N-methyl-daspartate receptors. Exp Neurol. 1996;137:119–126
- Bao F, John SM, Chen Y, Mathison RD, Weaver LC. The tripeptide phenylalanine-(d)glutamate-(d) glycine modulates leukocyte infiltration and oxidative damage in rat injured spinal cord. Neuroscience. 2006;140:1011–1022
- Liu NK, Zhang YP, Titsworth WL, et al. A novel role of phospholipase A2 in mediating spinal cord secondary injury. Ann Neurol. 2006;59:606–619
- Acosta JA, Yang JC, Winchell RJ. Lethal injuries and time to death in a level I trauma center. J Am Coll Surg. 1998;186:528–533
- DeVivo MJ, Krause JS, Lammertse DP. Recent trends in mortality and causes of death among persons with spinal cord injury. Arch Phys Med Rehabil. 1999;80:1411–1419
- O' Connor PJ. Survival after spinal cord injury in Australia. Arch Phys Med Rehabil. 2005;86:37–47
- Pickett GE, Campos-Benitez M, Keller JL, Duggal N. Epidemiology of traumatic spinal cord injury in Canada. Spine. 2006;31:799–805
- Ozer MK, Parlakpinar H, Vardi N, Cigremis Y, Ucar M, Acet A. Myocardial ischemia/reperfusion-induced oxidative renal damage in rats: Protection by caffeic acid phenethyl ester (CAPE). Shock. 2005;24:97–100
- Coursin DB, Coursin DB, Maccioli GA. Dexmedetomidine. Curr Opin Crit Care. 2001;7:221–226
- Hall JE, Uhrich TD, Barney JA, Arain SR, Ebert TJ. Sedative, amnestic and analgesic properties of small-dose dexmedetomidine infusions. Anesth Analg. 2000;90:699–705
- Engelhard K, Werner C, Eberspächer E, et al. The effect of the alpha 2-agonist dexmedetomidine and the N-methyl-d-aspartate antagonist S(+)-ketamine on the expression of apoptosis-regulating proteins after incomplete cerebral ischemia and reperfusion in rats. Anesth Analg. 2003;96:524–531
- Sanders RD, Sun P, Patel S, Li M, Maze M, Ma D. Dexmedetomidine provides cortical neuroprotection: Impact on anaesthetic-induced neuroapoptosis in the rat developing brain. Acta Anaesthesiol Scand. 2010;54:710–716
- Sanders RD, Xu J, Shu Y, et al. Dexmedetomidine attenuates isoflurane-induced neurocognitive impairment in neonatal rats. Anesthesiology. 2009;110:1077–1085
- Zhang XY, Liu ZM, Wen SH, et al. Dexmedetomidine administration before, but not after, ischemia attenuates intestinal injury induced by intestinal ischemia-reperfusion in rats. Anesthesiology. 2012;116:1035–1046
- Yoshitomi O, Cho S, Hara T, et al. Direct protective effects of dexmedetomidine against myocardial ischemia-reperfusion injury in anesthetized pigs. Shock. 2012;38:92–97
- Gu J, Sun P, Zhao H, et al. Dexmedetomidine provides renoprotection against ischemia-reperfusion injury in mice. Crit Care. 2011;15:R153. doi: 10.1186/cc10283
- Goyagi T, Tobe Y. Dexmedetomidine improves the histological and neurological outcomes 48 h after transient spinal ischemia in rats. Brain Res. 2014;1566:24–30
- Bell MT, Puskas F, Bennett DT, et al. Dexmedetomidine, a α-2a adrenergic agonist, promotes ischemic tolerance in a murine model of spinal cord ischemia-reperfusion. J Thorac Cardiovasc Surg. 2014;147:500–506
- Gul S, Hanci V, Bahadir B, et al. The effectiveness of dexmedetomidine in experimental spinal cord injury compared to methylprednisolone in rats. J Clin Neurosci. 2010;17:490–494
- Basso DM, Beattie MS, Brenham JC. Graded histological and locomotor outcomes after spinal cord contusion using the NYU weight-drop device versus transection. Exp Neurol. 1996;139:244–256
- Misra HP, Fridovich I. The role of superoxide anion in the autoxidation of epinephrine and a simple assay for superoxide dismutase. J Biol Chem. 1972;247:3170–3175
- Sinha AK. Colorimetric assay of catalase. Anal Biochem. 1972;47:389–394
- Rotruck JT, Pope AL, Ganther HE, Swanson AB, Hafeman DG, Hoekstra WG. Selenium: Biochemical role as a component of glutathione peroxidase. Science. 1973;179:588–590
- Lowry OH, Rosebrough NJ, Farr AL, Randall RJ. Protein measurement with the Folin phenol reagent. J Biol Chem. 1951;193:265–275
- Moron MS, Dipierre JW, Mannervik B. Levels of glutathione reductase and glutathione S-transferase activities in rat lung and liver. Biochem Biophy Acta. 1979;582:67–68
- Ohkawa H, Ohishi N, Yagi K. Assay for lipid peroxidation in animal tissues by thiobarbituric acid reaction. Anal Biochem. 1979;95:351–358
- Dalle-Donne I, Rossi R, Giustarini D, Milzani A, Colombo R. Protein carbonyl groups as biomarkers of oxidative stress. Clin Chim Acta. 2003;329:23–38
- Toosi KK, Nagatomi J, Chancellor MB, Sacks MS. The effects of long-term spinal cord injury on mechanical properties of the rat urinary bladder. Ann Biomed Eng. 2008;36:1470–1480
- Juan J, Herrera Ricky JL, Haywood-Watson II, Grill RJ. Acute and chronic deficits in the urinary bladder after spinal contusion injury in the adult rat. J Neurotrauma. 2010;27:423–431
- Villela NR, do Nascimento Júnior P, de Carvalho LR, Teixeira A. Effects of dexmedetomidine on renal system and on vasopressin plasma levels. Experimental study in dogs. Rev Bras Anestesiol. 2005;55:429–440
- Billings FT, Chen SW, Kim M, et al. Alpha2-adrenergic agonists protect against radio contrast-induced nephropathy in mice. Am J Physiol Renal Physiol. 2008;295:741–748
- Chi OZ, Hunter C, Liu X, Weiss HR. The effects of dexmedetomidine on regional cerebral blood flow and oxygen consumption during severe hemorrhagic hypotension in rats. Anesth Analg. 2011;113:349–355
- Juurlink BHJ, Paterson PG. Review of oxidative stress in brain and spinal cord injury: Suggestions for pharmacological and nutritional management strategies. J Spinal Cord Med. 1998;21:309–334
- Hall ED. Antioxidant therapies for acute spinal cord injury. Neurotherapeutics. 2011;8:152–167
- Bao F, Omana V, Brown A, Weaver LC. The systemic inflammatory response after spinal cord injury in the rat is decreased by a4b1 integrin blockade. J Neurotrauma. 2012;29:1626–1637
- Liu J, Tang T, Yang H. Protective effect of deferoxamine on experimental spinal cord injury in rat. Injury. 2011;42:742–745
- Berlett BS, Stadtman ER. Protein oxidation in aging, disease, and oxidative stress. J Biol Chem. 1997;272:20313–20316
- Dalle-Donne I, Aldini G, Carini M, Colombo R, Rossi R, Milzani A. Protein carbonylation, cellular dysfunction, and disease progression. J Cell Mol Med. 2006;10:389–406
- Bao F, Liu D. Peroxynitrite generated in the rat spinal cord induces neuron death and neurological deficits. Neuroscience. 2002;115:839–849
- Yune TY, Chang MJ, Kim SJ, et al. Increased production of tumor necrosis factor-alpha induces apoptosis after traumatic spinal cord injury in rats. J Neurotrauma. 2003;20:207–219
- Szabo C. Multiple pathways of peroxynitrite cytotoxicity. Toxicol Lett. 2003;140:105–112
- Hanci V, Ero B, Bektaş S, et al. Effect of dexmedetomidine on testicular torsion/detorsion damage in rats. Urol Int. 2010;84:105–111
- Kiliç K, Hanci V, Selek S, et al. The effects of dexmedetomidine on mesenteric arterial occlusion-associated gut ischemia and reperfusion-induced gut and kidney injury in rabbits. J Surg Res. 2012;178:223–232
- Uysal HY, Cuzdan SS, Kayıran O, et al. Preventive effect of dexmedetomidine in ischemia-reperfusion injury. J Craniofac Surg. 2012;23:1287–1291
- Ballatori N, Krance SM, Notenboom S, Shi S, Tieu K, Hammond CL. Glutathione dysregulation and the etiology and progression of human diseases. Biol Chem. 2009;390:191–214
- Erşahin M, Özdemir Z, Özsavcı D, et al. Melatonin treatment protects against spinal cord injury induced functional and biochemical changes in rat urinary bladder. J Pineal Res. 2012;52:340–348
- Toklu HZ, Hakan T, Celik H, et al. Neuroprotective effects of alpha-lipoic acid in experimental spinal cord injury in rats. J Spinal Cord Med. 2010;33:401–409
- Matés JM, Segura JA, Alonso FJ, Márquez J. Intracellular redox status and oxidative stress: Implications for cell proliferation, apoptosis, and carcinogenesis. Arch Toxicol. 2008;82:273–299
- Chang Y, Huang X, Liu Z, et al. Dexmedetomidine inhibits the secretion of high mobility group box 1 from lipopolysaccharide-activated macrophages in vitro. J Surg Res. 2013;18:308–314
- Kettle AJ, Winterbourn CC. Myeloperoxidase: A key regulator of neutrophil oxidant production. Redox Rep. 1997;3:3–15
- Can M, Gul S, Bektas S, et al. Effects of dexmedetomidine or methylprednisolone on inflammatory responses in spinal cord injury. Acta Anaesthesiol Scand. 2009;53:1068–1072
- Lee SM, Yune TY, Kim SJ, et al. Minocycline reduces cell death and improves functional recovery after traumatic spinal cord injury in the rat. J Neurotrauma. 2003;20:1017–1027
- Popovich PG, Stokes BT, Whitacre CC. Concept of autoimmunity following spinal cord injury: Possible roles for T lymphocytes in the traumatized central nervous system. J Neurosci Res. 1996;45:349–363
- Straub RH, Herrmann M, Berkmiller G, Frauenholz T, Lang B, Schölmerich Falk SW. Neuronal regulation of interleukin 6 secretion in murine spleen: Adrenergic and opioidergic control. J Neurochem. 1997;68:1633–1639
- Maes M, Lin A, Kenis G, Egyed B, Bosmans E. The effects of noradrenaline and alpha-2 adrenoceptor agents on the production of monocytic products. Psychiatry Res. 2000;96:245–253
- Szelényi J, Kiss JP, Vizi ES. Differential involvement of sympathetic nervous system and immune system in the modulation of TNF-alpha production by alpha2- and beta-adrenoceptors in mice. J Neuroimmunol. 2000;103:34–40
- Venn RM, Bryant A, Hall GM, Grounds RM. Effects of dexmedetomidine on adrenocortical function, and the cardiovascular, endocrine and inflammatory responses in post-operative patients needing sedation in the intensive care unit. Br J Anaesth. 2001;86:650–656
- Dasari VR, Spomar DG, Cady C, Gujrati M, Rao JS, Dinh DH. Mesenchymal stem cells from rat bone marrow downregulate caspase-3-mediated apoptotic pathway after spinal cord injury in rats. Neurochem Res. 2007;32:2080–2093
- Barut S, Unlü YA, Karaoğlan A, et al. The neuroprotective effects of z-DEVD.fmk, a caspase-3 inhibitor, on traumatic spinal cord injury in rats. Surg Neurol. 2005;64:213–220
- Knoblach SM, Huang X, Van Gelderen J, Calva-Cerqueira D, Faden AI. Selective caspase activation may contribute to neurological dysfunction after experimental spinal cord trauma. J Neurosci Res. 2005;80:369–380
- Habig WH, Pabst MJ, Jakoby WB. Glutathione S-transferases. The first enzymatic step in mercapturic acid formation. J Biol Chem. 1974;249:7130–7139