Abstract
Psychological states of anticipation modulate biological stress responsivity. While researchers generally investigate how subjective distress corresponds to the magnitude of stress reactivity, physiological recovery after acute stressors must also be considered when investigating disease vulnerabilities. This study assessed whether anticipatory stress would correspond to stress reactivity and recovery of salivary cortisol and blood pressure levels in response to a well-validated psychosocial stressor. Thirty participants (63% female; mean ± SEM age 45.4 ± 2.12 years) were exposed to the Trier Social Stress Test (TSST) consisting of a public speech and mental arithmetic. Ten salivary cortisol samples and systolic and diastolic blood pressure recordings were collected at time points spanning 50 min before and up to 50 min after stress exposure. These data were transformed into parameters representing stress reactivity (area under the curve) and stress recovery (percent change). The Primary Appraisal Secondary Appraisal scale assessed anticipatory stress before exposure to the TSST. Our results revealed that increased anticipatory stress predicted increased stress reactivity for cortisol (p = 0.009) but not blood pressure. For stress recovery, increased anticipatory stress predicted greater decrements of cortisol concentration (p = 0.015) and blood pressure (p = 0.039), even when controlling for total systemic “output” by incorporating baseline activity. This efficient shutdown of stress responses would have otherwise been ignored by solely investigating reactive increases. These findings underscore the importance of measuring multiple dynamic parameters such as recovery when investigating physiological stress response patterns as a function of psychosocial factors.
Introduction
Situations that are novel, unpredictable, threatening to the self, and/or uncontrollable additively contribute to physiological stress responses (Mason Citation1968; Dickerson and Kemeny Citation2004; Lupien et al. Citation2006). Under these circumstances, the sympathetic–adrenal–medullary axis releases catecholamines within seconds and the hypothalamic–pituitary–adrenal (HPA) axis secretes glucocorticoids within minutes in order to mobilize metabolic energy, increase cardiac output, and stimulate cytokine defenses necessary to adapt to the demands of the situation (McEwen Citation1998; Sapolsky et al. Citation2000). Individuals must, therefore, ascertain whether situations threaten their well-being and determine whether the demands of the encounter supersede their coping resources (Lazarus and Folkman Citation1984). Much literature focuses on how acute stress responses in artificial settings can be used to identify disease vulnerabilities associated with chronic stress arising in the real world.
Numerous stress-induction paradigms elicit social-evaluative threat to trigger stress responses (Dickerson and Kemeny Citation2004). This occurs specifically in laboratory situations that diminish an individual's control as well as where the prospect of being negatively evaluated, rejected, and shamed is contextually manipulated (Dickerson and Kemeny Citation2004; Andrews et al. Citation2007; Wadiwalla et al. Citation2010). The motivation toward preserving one's social-self vis-à-vis evaluation by others involves complex interactions among cognitive appraisals, affective processes, and physiological responses (Dickerson et al. Citation2004, Citation2008; Gruenewald et al. Citation2004). Those who experience social-evaluative threat more saliently must somehow fundamentally differ in their sensitivity toward the need to preserve their self-esteem or sense of worth. This pattern is deemed deleterious to an individual's health and well-being (Biondi and Picardi Citation1999).
The reactivity hypothesis (Manuck Citation1994) proposes that exaggerated physiological and behavioral reactivity to stressors are risk factors for cardiovascular disease among others (Lovallo and Gerin Citation2003; Lovallo Citation2010). In this perspective, pathophysiological reactivity is discernable by examining the magnitude of stress responses. In spite of this, the reactivity hypothesis has been criticized for often ignoring or dismissing physiological recovery, a period after exposure to stressors that is characterized by much individual variability (Linden et al. Citation1997; Earle et al. Citation1999; Rutledge et al. Citation2000). Instead of focusing solely on increases from baseline to stressor exposure, researchers have been urged to also take into account the duration and prolongation of stress responses after the stressor (Brosschot et al. Citation2005; Brosschot Citation2010). Investigating stable (trait) and situational (state) factors in relation to stress reactivity and recovery profiles might strengthen our understanding of disease trajectories (Cavanagh and Allen Citation2008).
In this vein, the psychological determinants of multi-systemic physiological responses to future psychosocial stressors have recently begun to be investigated in psychoneuroendocrine research on stress. Gaab et al. (Citation2005) have developed the Primary Appraisal Secondary Appraisal (PASA) scale based on Lazarus and Folkman's (Citation1984) transactional definition of stress as a state involving (1) an appraisal of threat and challenge and (2) evaluation of resource capabilities to persevere. The PASA scale also incorporates trait aspects of individual beliefs in competence and control expectancy, specifically as they relate to an anticipated stressor such as the widely used Trier Social Stress Test (TSST; Kirschbaum et al. Citation1993).
Using the PASA scale, studies have shown that anticipatory stress prior to laboratory stressors explained as much as 35% of the variance in increased salivary cortisol secretion (Gaab et al. Citation2005). Subsequent studies have found similar associations among increased anticipatory stress vis-à-vis increased salivary cortisol but not salivary α-amylase levels (Het et al. Citation2009), increased cortisol levels independent of perfectionism (Wirtz et al. Citation2007a), increased expression of plasma proinflammatory cytokines (Wirtz et al. Citation2007b), and increased hypercoagulant D-dimer levels (Wirtz et al. Citation2006a). No PASA scale associations were detected in relation to plasma norepinephrine and salivary cortisol concentrations in overcommitted workers (Wirtz et al. Citation2008) nor when comparing cortisol levels among hypertensives and normotensive individuals (Wirtz et al. Citation2006b). Overall, and pending more research, the PASA scale shows promise as a measure of anticipatory stress strongly predictive of physiological reactivity.
This study investigated whether anticipatory stress as measured using the PASA scale could similarly predict various parameters representative of HPA axis and cardiovascular functioning in response to the TSST vis-à-vis both stress reactivity and recovery. We hypothesized that increased anticipatory stress would be associated with increased stress reactivity as well as prolonged stress recovery.
Methods
Participants
Healthy men (n = 11) and women (n = 19) between 27 and 65 years of age (mode = 47 years, median = 41 years, M = 45.4 years, and SE = 2.12) were recruited through Montreal community-based Internet advertisements in a study first published elsewhere (Juster et al. Citation2011). The sample was predominately Caucasian (89.7%), well-educated (M = 15.97 years, SE = 0.057), and earning annual household incomes (M ≥ $40,000 CAN, SE = 0.55) above the Canadian poverty line (Statistics Canada 2010).
To avoid the confounding effects of sex hormones, women were tested during the follicular phase (days 1–14) rather than during the luteal phase (days 15–28) of their menstrual cycle. Only two women were using oral contraceptives. Exclusionary criteria included use of any glucocorticoid or cardiovascular altering medications (e.g. antidepressants, anti-asthmatics, diuretics, and β-blockers), being a heavy smoker (10+ cigarettes per day), or obese (BMI >30).
All participants provided written informed consent. This study was approved by the Research Ethics Board of the Douglas Mental Health University Institute respecting the Canadian Tri-Council's Policy statement for the ethical conduct for experimentation using humans, guided by the World Medical Association's Declaration of Helsinki.
Protocol
A 2-h afternoon visit scheduled between 13:00 h and 18:00 h to our laboratory involved exposure to the TSST (Kirschbaum et al. Citation1993). The only variation to the original protocol was that the PASA scale had to be administered during the 10-min anticipation phase. Upon completion of the PASA scale while preparing to give their talks, participants were escorted to a separate room where they delivered a 5-min mock job interview presentation, followed by 5 min of mental arithmetic in front of an unseen supposed “behavioral expert” behind a one-way window, while also being video recorded with a camera facing each participant directly. Both parties communicated via an intercommunication device. We previously found that this “panel-out” manipulation results in significant increases in cortisol levels (Lupien et al. Citation1997; Andrews et al. Citation2007; Wadiwalla et al. Citation2010; Juster et al. Citation2011).
A total of 10 saliva samples were collected with Salivettes® (Sarstedt©, tubes Part No. 51.1534 and Salimetrics© oral swabs Part No. 5001.02) at the following time points: − 50, − 30, − 20, − 10 min (anticipation phase), and immediately before the TSST, as well as immediately after, and at +10,+20,+30,+40, and +50 min (). Participants were instructed to avoid eating a major meal, drinking beverages other than water, smoking, or engaging in strenuous physical activity 30 min prior to testing as well as to not brush their teeth or floss 2 h before arriving at the laboratory. Simultaneous with each saliva sample, systolic and diastolic blood pressure was monitored using an electronic sphygmomanometer (LifeSource™ Model UA-767) in a seated position. Once participants were debriefed and compensated with CAN 50$, saliva samples were stored at − 20°C until subsequent analysis for cortisol (μg/dl).
Figure 1. Study timeline. Sampling time points (numbered boxes), pre- and post-stress exposure minutes (time above the timeline), and total minutes (time below timeline) are depicted throughout a 2-h afternoon session. Participants (a) rested for 40 min, (b) were given instructions to begin anticipating the TSST after 50 min at which time they completed the PASA scale, (c) were exposed to the TSST after 60 min, and then (d) recovered during 70–110 min until they were debriefed. The time points representing the recovery phase of salivary cortisol concentration and mean arterial pressure are demarcated at 70 and 80 min, respectively.
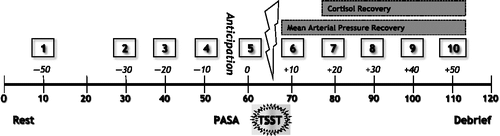
Saliva was analyzed with a high-sensitivity enzyme immunoassay kit (Salimetrics®, State College, PA, USA, Catalogue No. 1-3102). Frozen samples were brought to room temperature to be centrifuged at 15,000 g for 15 min. The standards, controls, and unknowns were placed into a micro-plate pre-coated with monoclonal antibodies to cortisol. The antibodies compete with cortisol bound to horseradish peroxidase (hp) for the binding sites on the plate. After an incubation period, the unbound portion was washed away. Tetramethylbenzidine was added to stain the bound portion blue, then this reaction was stopped with a 3 M solution of sulfuric acid making a yellow color. Within 5 min, the optical density of the yellow reaction was measured on a plate reader at 450 nm with a correction at 490 nm. The intensity of color measured is an indication of the level of hp bound to the plate and is therefore inversely proportional to the concentration of cortisol present. The range of detection for this assay was between 0.012 and 3 μg/dl.
Psychometrics
The 16-item English version of the PASA scale (Gaab et al. Citation2005) measures anticipatory cognitive appraisals prior to TSST exposure using a six-point Likert scale (“totally disagree” to “totally agree”). “The Primary Appraisal sub-scale includes “threat” and challenge statements, while the Secondary Appraisal sub-scale includes “self-concept of own competence” and “control expectancy” conponents. Consistent with Lazarus and Folkman's formulation of transactional stress perception (Lazarus and Folkman 1984), Primary and Secondary appraisals co-dependently determine an individual's perceptions of threat, which forms the “Tertiary Appraisal” scale of “global PASA index”. Participants completed the PASA scale ∼10 min before the TSST during the anticipation phase in which instructions were provided to participants about the upcoming psychosocial stressor.
The original PASA scale psychometrics reported by Gaab et al. (Citation2005) demonstrated acceptable internal consistency for the Primary Appraisal sub-scale (α = 0.80) that includes “threat” (α = 0.83) and “challenge” (α = 0.63) components as well as for the Secondary Appraisal sub-scale (α = 0.74) that includes “self-concept of own competence” (α = 0.81) and “control expectancy” (α = 0.77) components. The Tertiary Appraisal sub-scale is operationally defined as the difference between the Primary Appraisal and Secondary Appraisal sub-scales, whereby increased positive scores indicate greater anticipatory stress.
In this study, internal consistency for the Primary Appraisal sub-scale (α = 0.842) including “threat” (α = 0.921) and “challenge” (α = 0.661) components as well as for the Secondary Appraisal sub-scale (α = 0.590) including “self-concept of own competence” (α = 0.801) and “control expectancy” (α = 0.576) components revealed psychometric properties of strong semblance to the original report. Our sample's descriptive statistics for the Tertiary Appraisal sub-scale used in the main analyses were as follows: mode = − 2.25, median = − 0.625, M = − 0.9583, SE = 0.55, skewness = − 0.438, and kurtosis = − 0.590).
Like the original PASA study by Gaab et al. (Citation2005), we administered the 32-item English version of the Belief in Competence and Control Questionnaire, or FKK (Krampen Citation1989), to measure trait locus of control that was then used in comparison to the PASA sub-scales in order to ascertain validity. Using a six-point Likert scale (“not at all true” to “very true”), the FKK yields four primary FKK scales that include “self-concept of own competence,” “control expectancy: internality,” “control expectancy: powerful others control,” and “control expectancy: chance control.” Our participants completed the FKK questionnaire ∼30 min after the TSST.
Original validation of the PASA scale revealed logical accord to the theoretical assumption that an individual's belief in their competence and control corresponds to anticipatory stress. Validation analyses focusing on the PASA Tertiary Appraisal scale (Gaab et al. Citation2005) revealed the following correlation with primary FKK sub-scales: “self-concept of own competence” (r = − 0.469, p < 0.001), “control expectancy: internality” (r = − 0.314, p < 0.05), “control expectancy: power others control” (r = 0.246, p < 0.05), and “control expectancy: change control” (r = 0.226, p < 0.05).
Similarly in the current sample's results for the PASA Tertiary Appraisal scale, preliminary validation analyses yielded the following correlation coefficients with FKK sub-scales: “self-concept of own competence” (r = − 0.532, p = 0.003), “control expectancy: internality” (r = − 0.521, p = 0.003), “control expectancy: power others control” (r = 0.124, p = 0.514), and “control expectancy: change control” (r = 0.376, p = 0.04). Taking our reliability and validity analyses together, we generally replicated the original psychometrics of Gaab et al. (Citation2005).
It is important to note that test–retest reliability was not originally reported because the PASA is meant to ascertain anticipatory stress prior to the novel social-evaluative threat experienced during TSST exposure, which would render any repeated PASA measurement biased by prior TSST exposure.
Treatment of the data
The 10 measures of systolic and diastolic blood pressure were each transformed into mean arterial blood pressure (MAP) using the following formula: diastolic blood pressure +1/3 (systolic blood pressure–diastolic blood pressure; Zheng et al. Citation2008). Time-dependent stress reactive cortisol and MAP measures were calculated as the area under the curve with respect to increase from basal (AUCi) and with respect to ground (zero; AUCg), using formulae derived from the trapezoid formula (Pruessner et al. Citation2003). The AUCi represents presumed increases in biomarker levels due to the TSST without regard for zero. The AUCg represents total systemic “output” throughout an allotted time period, starting in our study at baseline until just before TSST instructions were given (anticipatory phase).
Stress reactivity was operationally defined as the AUCi for salivary cortisol concentrations and MAP. Stress recovery measures were computed by using a percent change formula [(Time 2 − Time 1/Time 1) × 100] for both cortisol and MAP levels based on the average peak post-TSST levels (Time 1), and levels at the end of the study +50 min after the TSST (Time 2). The cortisol recovery percent change score was based on saliva samples taken 10 min after the TSST (+20 min), as this is the average peak time point according to a meta-analysis (Dickerson and Kemeny Citation2004) consistent with our results. The MAP recovery percent change score was based on blood pressure values assessed immediately after the TSST (+10 min), as this was the average peak for our sample.
For our stress recovery analyses, we used AUCg values (these integrated values are deemed “outputs”) as covariates because gradual decrements in cortisol and MAP levels during recovery depend on the magnitude of systemic “output” prior to stressor exposure. Because the AUCg differs from AUCi in that it represents total systemic “output” rather than only increases, we limited the time period of AUCg values to the first four measurements of cortisol and MAP prior to the anticipation phase of the TSST in order to assess systemic output before stress exposure. illustrates the study's theoretical and methodological underpinnings.
Figure 2. Schematic representing key study constructs illustrated using the mean ( ± SE bars) salivary cortisol and mean arterial pressure values obtained from our sample (N = 30). The PASA scale is here broken down into respective sub-scales representing anticipatory stress prior to TSST exposure. In theory, the TSST induces social-evaluative threat that activates social-self preservation motivations that modulate physiological responses. In our analyses, mean salivary cortisol concentration and mean arterial pressure dynamics throughout the testing session (solid black line) were transformed into stress reactivity and stress recovery measures. In our first regression analyses, we used the area under the curve with respect to increase (AUCi; the area indicated by dotted lines throughout the session) representing stress reactivity from baseline ( − 50 min). For the second analyses, multiple regressions used a recovery percent slope (dashed line indicates recovery) representing linear decreases post-TSST; the area under curve with respect to ground (AUCg) representing total systemic “output” immediately before the TSST onwards was used as a covariate in the prediction of cortisol and mean arterial pressure recovery.
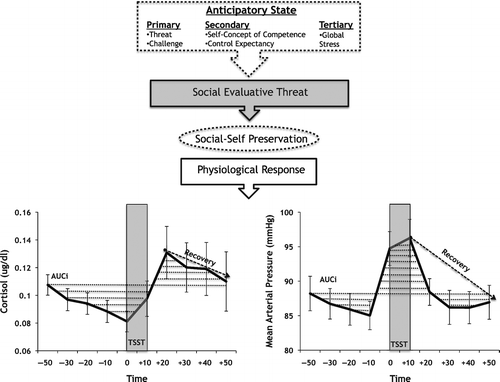
Statistical analysis
Firstly, to assess stress reactivity, linear regressions were executed using the PASA index entered as a predictor of cortisol and MAP AUCi levels. Secondly, to assess stress recovery, multiple regressions were computed in two steps with (1) the PASA index followed by (2) cortisol or MAP AUCg entered as control variables in the prediction of cortisol and MAP recovery percent change.
Results
PASA prediction of salivary cortisol and MAP reactivity
Two separate linear regressions were executed with the PASA indices entered as a predictor of cortisol reactivity and then MAP reactivity to the TSST (). Using the PASA index, increased anticipatory stress before the TSST significantly predicted increased cortisol AUCi levels (r = 0.467, r2 = 0.218, adjusted r2 = 0.190, F(1,28) = 7.823, p = 0.009; ) but not increased MAP AUCi levels (p = 0.71).
Table I. Regression parameters with anticipatory stress PASA prediction of cortisol and mean arterial pressure reactivity AUCi in response to exposure to the TSST.
PASA prediction of salivary cortisol and MAP recovery
Two separate hierarchical linear regressions were executed in two models with (1) the PASA index entered followed by (2) the corresponding AUCg values as a covariate, entered as predictors of cortisol recovery and MAP recovery after TSST exposure ().
Table II. Regression model parameters with anticipatory stress (PASA) in association with cortisol and mean arterial pressure recovery (percent change).
For cortisol recovery, Model 1 revealed that increased anticipatory stress prior to the TSST significantly predicted steeper cortisol recovery after the TSST (r = 0.442 r2 = 0.195, adjusted r2 = 0.166, F(1,28) = 6.790, p = 0.015; ). Model 2 with cortisol AUCg levels added did not contribute additional explanatory power (r = 0.460, r2 = 0.212, adjusted r2 = 0.153, Δr2 = 0.016, ΔF(2,27) = 0.564, Δp = 0.459), nor did it eliminate statistical significance (F(2,27) = 3.624, p = 0.040).
Figure 4. Regression scatterplot illustrating the significant association (p = 0.015) between anticipatory stress measured with the PASA scale and salivary cortisol concentration recovery after exposure to the TSST.
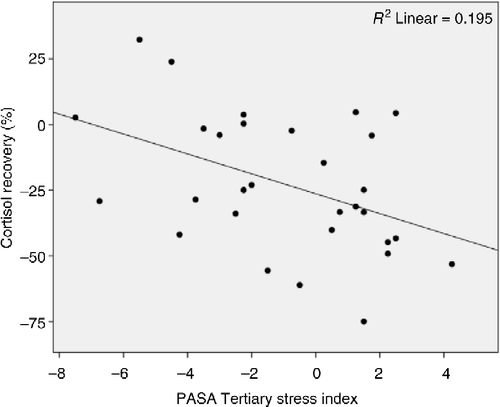
For MAP recovery, Model 1 with the PASA index entered revealed that increased anticipatory stress significantly predicted steeper MAP recovery after the TSST (r = 0.378, r2 = 0.143, adjusted r2 = 0.112, F(1,28) = 4.676, p = 0.039; ). When added to Model 2, increased MAP output (AUCg) significantly added variance as a predictor of increased MAP recovery after the TSST (r = 0.572, r2 = 0.327, adjusted r2 = 0.277, Δr2 = 0.184, ΔF(1,27) = 7.390, Δp = 0.011), rendering the equation even more significant (F(2,27) = 6.566, p = 0.005).
Discussion
This study highlights the importance of measuring time dynamics in various ways when exploring the modulating effects of anticipatory stress on stress responsivity. In summary, we found partial support for our hypothesis that increased stress reactivity, with respect to increased cortisol secretion, would correspond with increased anticipatory stress, although no correspondence was detected for mean arterial pressure. Inconsistent with our hypothesis, participants with increased anticipatory stress had steeper recovery of cortisol and blood pressure levels; however, the latter was also largely explained by total systemic “output” (AUCg) prior to stress exposure.
Had we only focused on stress reactivity, we would have erroneously concluded that participants with increased anticipatory stress were most distressed and vulnerable to stress-related diseases. However, contrary to this assumption, they also showed the greatest decrements in cortisol during the recovery phase. These results force us to consider that the priming effect of anticipatory stress might induce in pre-stress exposure on cognitive states of vigilance and physiological readiness to respond. As our data indicate, these complex processes might indeed trigger intense stress reactivity, but perhaps also a healthy, rapid recovery upon stressor cessation due to elusive individual differences.
Our results using the PASA scale shed further light on the modulating role of anticipatory stress that has on stress responsivity to social-evaluative threat, but not necessarily one that could be deemed deleterious in this cross-sectional study of healthy adults. The PASA scale explained 17–22% of the variance in cortisol dynamics for increased anticipatory stress in association with increased cortisol reactivity (AUCi) and steeper cortisol recovery independently of total systemic HPA axis output (AUCg). Clearly, anticipatory stress is a time-dependent construct that might not necessarily represent dysfunctional emotional regulation in and of itself when we consider recovery as well. Indeed, quite to the contrary, our PASA results indicate that participants who experienced increased anticipatory stress showed intense HPA axis activation and efficient shutdown during recovery.
In terms of cardiovascular recovery, increased anticipatory stress explained about 11–14% of the variance for increased decrements in mean arterial pressure. The effect of the PASA scale held after adjusting for MAP integrated with respect to ground (MAP “output,” AUCg) prior to TSST anticipation, itself accounting for 27–32% of the variance. Nonetheless, there are nuances concerning whether stressful situations are appraised as threatening versus challenging that correspond to respective differences in myocardial or vascular reactions (Tomaka et al. Citation1997, Citation1999) that we could not address.
The similarities among cortisol and blood pressure results during recovery were somewhat unexpected, given that cardiovascular dynamics are often independent of other autonomic nervous system biomarkers (Kamarck and Lovallo Citation2003; Schommer et al. Citation2003). Again, this finding highlights the importance of teasing apart temporal dynamics as they pertain to parameters of stress reactivity and recovery. Future research that incorporates momentary assessments of heart rate variability would be beneficial in elucidating how anticipatory stress mediates or moderates cardiovascular activities during stressful situations. It will also be important to tease apart important factors associated with resiliency such as the personality traits of self-esteem, mastery, extroversion, openness to experience, and others that we regrettably could not address beyond locus of control elements embedded within the PASA scale and in comparison with the FKK scales.
Our study has some key limitations that warrant discussion. Principally, our interpretations are based on only 30 healthy participants varying considerably in age and who were disproportionately female. We are therefore restricted in the conclusions we can make with such low power and heterogeneity. Concerning the overrepresentation of females, our study nevertheless is the only study to our knowledge to use the PASA scale among women not in the luteal phase of their menstrual cycles. A larger sample would have also permitted us to assess associated domains such as social support, health behaviors, and various psychosocial variables. Likewise, it is important for studies of this kind to consider how early life experiences might modulate biological sensitivities to contexts (Boyce and Ellis Citation2005; Ellis et al. Citation2005) and differential susceptibilities to the environment (Ellis and Boyce Citation2011; Ellis et al. Citation2011). Specifically, stress reactivity is not a unitary process, as highly reactive phenotypes can be considered conditionally adaptive given prior historical and neurodevelopmental circumstances that precipitate such sensitivities (Boyce and Ellis Citation2005; Ellis et al. Citation2005).
At a theoretical level that is well beyond the scope of the current study, prolonged physiological arousal during recovery is proposed to co-depend on preservative cognitions (Brosschot et al. Citation2006). These refer to overactivated cognitive representations of stressors manifested in the form of excessive worrying, rumination, and anticipatory stress, which predispose individuals to experiencing exaggerated stress reactivity and prolonged recovery, leading eventually to stress-related conditions such as cardiovascular disease (Brosschot and Thayer Citation2003; Brosschot et al. Citation2005, Citation2006). In addition to emphasizing the importance of exploring features of stress reactivity and recovery together, this perspective suggests that stressors be explored as a function of duration, hence, across different time frames. Researchers are encouraged to transcend retrospective accounts of chronic stress that are the focus of so many questionnaires by developing novel techniques to measure both adaptive and maladaptive cognitive processes that modulate stress responsivity.
In conclusion, we found that increased anticipatory stress predicted increased stress reactivity for cortisol but not blood pressure in healthy adults. For stress recovery, however, increased anticipatory stress predicted greater decrements of cortisol and blood pressure levels. Future studies should consider combining state and trait measures and various time-dependent physiological measures to further understand how synergistic effects can protect health and well-being among resilient as well as vulnerable individuals. Clinical research has demonstrated that cognitive-behavioral group therapy can diminish anticipatory stress and attenuate cortisol responsivity (Gaab et al. Citation2003; Storch et al. Citation2007), which suggests applications well beyond the laboratory that should be further explored among diverse populations.
Acknowledgements
This study was funded by a grant (#134254) from the Canadian Institutes of Health Research to S.J.L. S.J.L. holds a Senior Investigator Chair on Gender and Mental Health from the Canadian Institute of Gender and Health. R.P.J. holds a Doctoral scholarship from the Institute of Aging of the Canadian Institutes of Health Research. We would also like to thank Helen Findlay for meticulously executing our salivary assays. Thanks are also extended to the two anonymous reviewers who helped strengthen our article.
Declaration of interest: The author reports no conflicts of interest. The author alone is responsible for the content and writing of the paper.
References
- Andrews J, Wadiwalla M, Juster RP, Lord C, Lupien SJ, Pruessner JC. 2007. Effects of manipulating the amount of social-evaluative threat on the cortisol stress response in young healthy men. Behav Neurosci. 121:871–876.
- Biondi M, Picardi A. 1999. Psychological stress and neuroendocrine function in humans: The last two decades of research. Psychother Psychosom. 68:114–150.
- Boyce WT, Ellis BJ. 2005. Biological sensitivity to context: I. An evolutionary-developmental theory of the origins and functions of stress reactivity. Dev Psychopathol. 17:271–301.
- Brosschot JF. 2010. Markers of chronic stress: Prolonged physiological activation and (un)conscious perseverative cognition. Neurosci Biobehav Rev. 35:46–50.
- Brosschot JF, Thayer JF. 2003. Heart rate response is longer after negative emotions than after positive emotions. Int J Psychophysiol. 50:181–187.
- Brosschot JF, Pieper S, Thayer JF. 2005. Expanding stress theory: Prolonged activation and perseverative cognition. Psychoneuroendocrinology. 30:1043–1049.
- Brosschot JF, Gerin W, Thayer JF. 2006. The perseverative cognition hypothesis: A review of worry, prolonged stress-related physiological activation, and health. J Psychosom Res. 60:113–124.
- Cavanagh JF, Allen JJ. 2008. Multiple aspects of the stress response under social evaluative threat: An electrophysiological investigation. Psychoneuroendocrinology. 33:41–53.
- Dickerson SS, Kemeny ME. 2004. Acute stressors and cortisol responses: A theoretical integration and synthesis of laboratory research. Psychol Bull. 130:355–391.
- Dickerson SS, Gruenewald TL, Kemeny ME. 2004. When the social self is threatened: Shame, physiology, and health. J Pers. 72:1191–1216.
- Dickerson SS, Mycek PJ, Zaldivar F. 2008. Negative social evaluation, but not mere social presence, elicits cortisol responses to a laboratory stressor task. Health Psychol. 27:116–121.
- Earle TL, Linden W, Weinberg J. 1999. Differential effects of harassment on cardiovascular and salivary cortisol stress reactivity and recovery in women and men. J Psychosom Res. 46:125–141.
- Ellis BJ, Boyce WT. 2011. Differential susceptibility to the environment: Toward an understanding of sensitivity to developmental experiences and context. Dev Psychopathol. 23:1–5.
- Ellis BJ, Essex MJ, Boyce WT. 2005. Biological sensitivity to context: II. Empirical explorations of an evolutionary-developmental theory. Dev Psychopathol. 17:303–328.
- Ellis BJ, Boyce WT, Belsky J, Bakermans-Kranenburg MJ, van Ijzendoorn MH. 2011. Differential susceptibility to the environment: An evolutionary-neurodevelopmental theory. Dev Psychopathol. 23:7–28.
- Gaab J, Blattler N, Menzi T, Pabst B, Stoyer S, Ehlert U. 2003. Randomized controlled evaluation of the effects of cognitive-behavioral stress management on cortisol responses to acute stress in healthy subjects. Psychoneuroendocrinology. 28:767–779.
- Gaab J, Rohleder N, Nater UM, Ehlert U. 2005. Psychological determinants of the cortisol stress response: The role of anticipatory cognitive appraisal. Psychoneuroendocrinology. 30:599–610.
- Gruenewald TL, Kemeny ME, Aziz N, Fahey JL. 2004. Acute threat to the social self: Shame, social self-esteem, and cortisol activity. Psychosom Med. 66:915–924.
- Het S, Rohleder N, Schoofs D, Kirschbaum C, Wolf OT. 2009. Neuroendocrine and psychometric evaluation of a placebo version of the ‘Trier Social Stress Test’. Psychoneuroendocrinology. 34:1075–1086.
- Juster RP, Sindi S, Marin MF, Perna A, Hashemi A, Pruessner JC, Lupien SJ. 2011. A clinical allostatic load index is associated with burnout symptoms and hypocortisolemic profiles in healthy workers. Psychoneuroendocrinology. 36:797–805.
- Kamarck TW, Lovallo WR. 2003. Cardiovascular reactivity to psychological challenge: Conceptual and measurement considerations. Psychosom Med. 65:9–21.
- Kirschbaum C, Pirke KM, Hellhammer DH. 1993. The ‘Trier Social Stress Test’—a tool for investigating psychobiological stress responses in a laboratory setting. Neuropsychobiology. 28:76–81.
- Krampen G. 1989. Fragebogen Zu Kompetenz- und Kontrollüberzeugungen (FKK). Göttingen: Hogrefe.
- Lazarus R, Folkman S. 1984. Stress, appraisal, and coping. New York: Springer Publishing Co..
- Linden W, Earle TL, Gerin W, Christenfeld N. 1997. Physiological stress reactivity and recovery: Conceptual siblings separated at birth?. J Psychosom Res. 42:117–135.
- Lovallo WR. 2010. Cardiovascular responses to stress and disease outcomes: A test of the reactivity hypothesis. Hypertension. 55:842–843.
- Lovallo WR, Gerin W. 2003. Psychophysiological reactivity: Mechanisms and pathways to cardiovascular disease. Psychosom Med. 65:36–45.
- Lupien SJ, Gaudreau S, Tchiteya BM, Maheu F, Sharma S, Nair NP, Hauger RL, McEwen BS, Meaney MJ. 1997. Stress-induced declarative memory impairment in healthy elderly subjects: Relationship to cortisol reactivity. J Clin Endocrinol Metab. 82:2070–2075.
- Lupien SJ, Ouellet-Morin I, Hupbach A, Walker D, Tu MT, Buss C, . 2006. Beyond the stress concept: Allostatic load – a developmental biological and cognitive perspective. In: Cicchetti D. editors. Handbook series on developmental psychopathology784–809.
- Manuck SB. 1994. Cardiovascular reactivity in cardiovascular disease: “Once more unto the breach”. Int J Behav Med. 1:4–31.
- Mason JW. 1968. A review of psychoendocrine research on the sympathetic-adrenal medullary system. Psychosom Med. 30:631–653.
- McEwen BS. 1998. Stress, adaptation, and disease. Allostasis and allostatic load. Ann NY Acad Sci. 840:33–44.
- Pruessner JC, Kirschbaum C, Meinlschmid G, Hellhammer DH. 2003. Two formulas for computation of the area under the curve represent measures of total hormone concentration versus time-dependent change. Psychoneuroendocrinology. 28:916–931.
- Rutledge T, Linden W, Paul D. 2000. Cardiovascular recovery from acute laboratory stress: Reliability and concurrent validity. Psychosom Med. 62:648–654.
- Sapolsky RM, Romero LM, Munck AU. 2000. How do glucocorticoids influence stress responses? Integrating permissive, suppressive, stimulatory, and preparative actions. Endocrinol Rev. 21:55–89.
- Schommer NC, Hellhammer DH, Kirschbaum C. 2003. Dissociation between reactivity of the hypothalamus-pituitary-adrenal axis and the sympathetic-adrenal-medullary system to repeated psychosocial stress. Psychosom Med. 65:450–460.
- Storch M, Gaab J, Kuttel Y, Stussi AC, Fend H. 2007. Psychoneuroendocrine effects of resource-activating stress management training. Health Psychol. 26:456–463.
- Tomaka J, Blascovich J, Kibler J, Ernst JM. 1997. Cognitive and physiological antecedents of threat and challenge appraisal. J Pers Soc Psychol. 73:63–72.
- Tomaka J, Palacios R, Schneider KT, Colotla M, Concha JB, Herrald MM. 1999. Assertiveness predicts threat and challenge reactions to potential stress among women. J Pers Soc Psychol. 76:1008–1021.
- Wadiwalla M, Andrews J, Lai B, Buss C, Lupien SJ, Pruessner JC. 2010. Effects of manipulating the amount of social-evaluative threat on the cortisol stress response in young healthy women. Stress. 13:214–220.
- Wirtz PH, Ehlert U, Emini L, Rudisuli K, Groessbauer S, Gaab J, Elsenbruch S, von Kanel R. Anticipatory cognitive stress appraisal and the acute procoagulant stress response in men. Psychosom Med. 2006a; 68:851–858.
- Wirtz PH, von Kanel R, Mohiyeddini C, Emini L, Ruedisueli K, Groessbauer S, Ehlert U. Low social support and poor emotional regulation are associated with increased stress hormone reactivity to mental stress in systemic hypertension. J Clin Endocrinol Metab. 2006b; 91:3857–3865.
- Wirtz PH, Elsenbruch S, Emini L, Rudisuli K, Groessbauer S, Ehlert U. Perfectionism and the cortisol response to psychosocial stress in men. Psychosom Med. 2007a; 69:249–255.
- Wirtz PH, von Kanel R, Emini L, Suter T, Fontana A, Ehlert U. Variations in anticipatory cognitive stress appraisal and differential proinflammatory cytokine expression in response to acute stress. Brain Behav Immunol. 2007b; 21:851–859.
- Wirtz PH, Siegrist J, Rimmele U, Ehlert U. 2008. Higher overcommitment to work is associated with lower norepinephrine secretion before and after acute psychosocial stress in men. Psychoneuroendocrinology. 33:92–99.
- Zheng L, Sun Z, Li J, Zhang R, Zhang X, Liu S, Xu C, Hu D, Sun Y. 2008. Pulse pressure and mean arterial pressure in relation to ischemic stroke among patients with uncontrolled hypertension in rural areas of China. Stroke. 39:1932–1937.